4
Networks and Mobile Platforms
The NEXRAD network radar-site selection was based upon a simple criterion—the aerial coverage at an altitude of 10,000 feet above the site level. A more comprehensive evaluation of radar coverage (NRC, 1995) measured the effective range of coverage as a function of specific weather phenomena. The 1995 NRC study illustrated convincingly that radar coverage by the NEXRAD network was generally far better than that of the network it had replaced. A few exceptions were found, and the agencies subsequently took remedial action.
By almost any measure, the network has proved its worth, serving the nation well and setting a new international standard for coordinated weather radar observations in a national context. Although the NEXRAD network was originally envisioned as an integral part of a complex and comprehensive integrated system in the modernized weather service, fully comprehensive integration of the radar information with other observational data in sophisticated interpretive algorithms and in data assimilation schemes with numerical models has yet to be realized. Much developmental work of this nature will certainly continue in the years to come, and weather forecasts will certainly be improved as a consequence of the NEXRAD data. There are, however, certain physical limitations that cannot be overcome with the existing deployment strategy. In the design of the successor network, these factors need to be considered seriously. However, it remains a fundamental assumption that the successor to NEXRAD will remain but one component in an integrated observing system. In addition to the primary radar network, complementary observations may include supplemental short-range radars, other government and private sector radars, mobile radars, space-based radars, and other observational systems.
AUXILIARY SHORT-RANGE RADARS
Limitations of individual, long-range radars are that at distant ranges, the beam overshoots the lower altitudes because of the earth’s curvature, and the crossbeam resolution degrades progressively with increasing range.
A denser network of radars would provide solutions to many of these problems. Overshoot reduction and resolution enhancements are the obvious benefits. If the radars were close enough to provide coverage above their nearest neighbors, then the cone-of-silence voids would be eliminated. Because of the directional dependence of anomalous propagation, a dense network of radars might also provide some mitigation of these data corruption problems, provided that there is a data-level integration of the base data throughout the radar network. In addition to filling data voids, multiple viewing angles in overlap regions could provide multiple-Doppler resolution of the wind field. It is extremely important to investigate cost-effective ways to achieve a sufficiently dense radar network.
Thus, there are many reasons to consider a future radar system comprised of a network of long-range advanced radars operating at S-band and subnetworks of smaller radars operating at higher frequencies such as X-band. The latter would be used, for example, to cover the lower elevations below the radar horizon of the longer-range S-band radars, particularly in tornado-prone areas (where tornadoes have frequently been found to form at lower levels) (Trapp et al., 1999) and in mountainous regions. In addition, low-level boundaries such as coastal, seabreeze, gust, and some synoptic-scale fronts may also be below the radar horizon. These boundaries are often the sites of significant weather events. Such short-range radars would also serve a variety of other functions. In particular, polarimetric X-band systems can possibly measure hydrologically and climatologically important light to moderate rainfall more accurately than is possible with S-band systems (Martner et al., 2001). These radars may also be useful in detecting conditions in clouds prior to the onset of lightning where electric fields align crystals vertically, leading to regions of negative differential phase shift that are readily detected by polarimetric radars (Caylor and Chandrasekar, 1996; Carey and Rutledge, 1998).
These X-based radars would be smaller and much less expensive than the larger S-band systems and would operate unattended with control from a central command office. Spread spectrum coding of the individual radar waveforms would allow them to operate in the same band with minimal interference. A relatively small fixed phased array, perhaps cylindrical or lampshade-shaped, could be used in order to allow coverage in all azimuths for a limited sector of elevations. Furthermore, line-of-sight attenuation measurements between the individual sensors over ranges of 20–50 km may provide additional information on precipitation rates or water vapor content using differential absorption techniques.
Anticipated advances in networking technology in the next decade will bring the cost and bandwidth of wide-area networking to levels that would make a
high-density, highly coupled network of radars possible. Functionally, the radars would be sensors equipped with signal processing and display capabilities. The signals would be sent to a central location by fiber optic networks. The central facility would collect all the data in real time, process the data, and create the three-dimensional meteorological fields needed for forecasting and warning. Thus, with appropriate networking, a single radar could be divided into a system of smaller radars with short-range coverage that would enable new capabilities (Chandrasekar et al., 2001).
Figure 4–1 shows the concept of adding networks of small radars, similar to the concept of cell definition in wireless communication (Chandrasekar and Jayasumana, 2001). These smaller radars could be at a higher frequency and transmit less power than the S-band NEXRAD in use today. For the anticipated shorter range of coverage, the beamwidth could be wider while still maintaining a specific spatial resolution, since the coverage area would be reduced.
These multiple networked radars would be individually less expensive than the longer-range radar, but the total network cost might be comparable, even after including the added cost of developing the protocol and infrastructure for merging data from multiple radars. This concept can be extended to regions where terrain blockage is a problem. If a higher frequency were to be selected, then corresponding issues of attenuation correction would need to be addressed. Polarimetric techniques have been used successfully to correct the radar echoes for attenuation in rain (Bringi and Chandrasekar, 2001). However, the algorithms have yet to be demonstrated in regions of mixed-phase precipitation and have yet to be fully explored at X-band (and higher) frequencies. Because these smaller, higher-frequency radars would be expected to provide coverage over shorter ranges, the impact of path-integrated attenuation is not as significant as in longer-range systems. Therefore, the use of extensive networking to integrate large S-band phased array systems with smaller, short-range, short-wavelength radar, along with implementation of attenuation correction, could solve two critical problems of current-day systems. Specifically, the near-surface boundary layer coverage would be more uniform, and polarimetric capability would allow improved rainfall estimation at lower rain rates. These short-range radars could first be located in important rainfall and drainage basins, near airports, and near cities or other areas where boundary layer coverage is important.
Recommendation—Far-Term
The potential for a network of short-range radar systems to provide enhanced near-surface coverage and supplement (or perhaps replace) a NEXRAD-like network of primary radar installations should be evaluated thoroughly.
Some of the gap-filling function can be provided by more effective use of other operational weather radars. For example, the Terminal Doppler Weather Radar (TDWR) operated by the FAA and located at 45 major airports around the
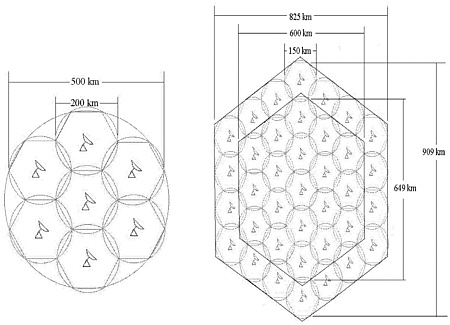
FIGURE 4–1. Comparison of widely spaced array of large radars (left) and closed spaced array of small, short-range radars (right) (Adapted from Chandrasekar and Jayasumana 2001)
nation provide superb low-level coverage in areas often not covered as well by the NEXRAD. There are also more than 100 weather radars operated by private sector organizations, primarily television stations that also could provide useful data.
Recommendation—Near-Term
The potential value and technology to assimilate data from complementary radar systems to provide a more comprehensive description of the atmosphere should be investigated.
RADAR PROFILERS
In the future, an integral part of the weather observing networks will be the radar profilers (operating at around 400 or 900 MHz) with radio acoustic sounding (RASS) for nearly continuous wind and temperature soundings. (The choice of radar frequency will depend upon frequency allocations.) The continuous
measurement of winds and temperature is essential for a broad range of forecasting applications and for data assimilation into numerical models.
The lower-frequency profilers can also provide winds through the stratosphere. These have recently been found to have extended-range predictive value (Baldwin and Dunkerton, 2001).
Profilers have also been used extensively in vertical sounding of precipitation microphysics and kinematics (Atlas et al., 1999; Tokay et al., 1999). By providing a measure of the vertical air motions and the drop size distribution, the profilers allow one to improve microphysical models without the crude parameterizations that have been necessary in the past. Such information is also of considerable value in a variety of other contexts such as providing appropriate physically based Z-R relations. It can also be used as input to larger-scale models, and as means of calibrating conventional scanning radar. The profiling radars are a critical element of the future radar system.
OTHER COMPLEMENTARY OBSERVATIONS IN AN INTEGRATED NETWORK
Observing system networks currently contain a host of different approaches for making a variety of atmospheric measurements. Fundamental to these networks are in situ surface and radiosonde observations, the most basic of measurements used in numerical weather prediction (NWP) models and used for many short-term forecasts. Cloud-to-ground lightning flashes are now routinely monitored nationally, thus augmenting substantially severe weather and aviation forecasts. Polar orbiting satellite data are valuable in numerical weather prediction, and geostationary satellite data provide continuous images of clouds, water vapor, temperature, and other variables along with wind data. The increasing availability and sophistication of digital photographic and video equipment, coupled with the emphasis on increasing the data transmission bandwidth available to nearly everyone, means that a wide variety of visual imagery provided by amateurs as well as professionals may play a role in the future observing system. SuomiNet, a national network (Ware et al., 2000) is using ground-based and inexpensive global positioning system (GPS) receivers to measure water vapor fields. Further in the future, Constellation Observing System for Meteorology, Ionosphere, and Climate (COSMIC)—a constellation of low-orbiting satellites with GPS receivers (Rocken et al., 1997; Kuo et al., 2000)—will make thousands of soundings daily of atmospheric refractivity, which will be highly resolved in the vertical axis, and relatable to temperature and water vapor.
To date, however, the broad range of network data has not been exploited in a fully integrated fashion. In weather forecast offices, such supplemental observations are taken into consideration by individual forecasters, but usually without the benefit of advanced data assimilation analyses or high-resolution NWP guidance. Similarly, Doppler radar data are only now being evaluated for their
potential to better initialize and improve the performance of high-resolution and national mesoscale models. In addition, a network of ground-based GPS receivers would provide horizontal fields of vertically integrated precipital water vapor. However, there is little doubt that research in the next decade will achieve considerable progress in the field of data assimilation and high-resolution NWP modeling for at least three reasons (Droegemeier et al., 2000). First, this is a topic receiving great emphasis in the U.S. Weather Research Program. Second, the topic is of considerable intellectual interest and is attracting outstanding people to the field. Third, digital computing technology is advancing so rapidly that the tools necessary for advanced operational modeling will be available and affordable (More detailed descriptions of these issues are found in Chapter 5 and in NRC 1999).
MOBILE RADAR
In recent years mobile and airborne radars have been used successfully in studies of severe storms and have been obtaining unprecedented high-resolution observations of tornadoes during their birth and evolution. Wurman and Gill (2000) made such observations with an X-band radar (Doppler on Wheels, or DOW); Bluestein and Pazmany (2000) provided equally impressive measurements with a mobile 3-mm-wavelength radar. In addition, Wakimoto and Liu (1998) used the X-band airborne Electra Doppler Radar (ELDORA) to document storm-scale motions in a super-cell while a tornado formed. All such observations have revealed significant aspects of tornadoes and their genesis not previously resolved. Richardson et al. (2001) have also obtained complete flow fields using dual-Doppler observations from two DOW systems. With the aid of local severe storm forecasts, groups from the University of Oklahoma have been able to position mobile Doppler radars near tornadoes as they developed and moved (Bluestein and Pazmany, 2000). This achievement suggests that it may be feasible for mobile radar units to be used to provide data to assist in storm warnings and to supplement warnings that are based solely upon the fixed-network radars. For example, real-time wind speed estimates in tornadoes and the precise location of the tornadoes could be relayed to the National Weather Service (NWS) and local communities.
Mobile Doppler radars could also be used to set up multiple-radar networks on the coastline or inland near to but a safe distance from land-falling hurricanes. In the past, the DOWs have been transported to coastal regions of North Carolina, the central Gulf, and Florida with adequate time to be set up in advance of hurricane landfall. Data from mobile radar networks could be used to determine more accurately the boundary layer wind field so that nowcasts and forecasts of surface wind speeds could be improved.
Ad hoc mobile Doppler radar networks could also be used near and downwind from sites of nuclear, biological, chemical, and other accidents or acts of
terrorism. Multiple-Doppler wind fields could be used to determine areas most affected by contaminants, and they could also be used as input for very small-scale numerical models, which might be able to provide short-term forecasts of contaminant fields.
While mobile, short-wavelength radars could be used for special purposes in limited areas, transportable NEXRAD replacements could be used near NEXRAD sites at which the local network operational radars are not working for extended periods of time.
Recommendation—Near-Term
The potential of operational mobile radar systems to contribute to the nation’s weather surveillance system for emergency response and for improved short- term forecasts should be evaluated.
AIRBORNE RADARS
The use of radar on aircraft as a weather detection and avoidance tool goes back approximately 50 years, when the airlines, particularly United and Northwest Airlines, worked with several manufacturers to develop the first airborne storm avoidance radars. In time, a requirement for on-board weather radar became a federal (and international) requirement. The military has developed several airborne radars that have weather detection capabilities, which have been used occasionally. Today, many of the commercial airborne radars have upgraded with Doppler capability, primarily to detect turbulence aloft.
Within the past several years, unmanned aerospace vehicles (UAVs) have become prominent, especially in the military, which has an operational need to “see” enemy activities in “denied” areas where more conventional observations of military operations are difficult. Another major use of UAVs has been the collection of weather data in remote, typically oceanic areas. It is envisioned that during the coming years, there will be two applications of airborne radar heretofore not addressed. During the coming years, unmanned aircraft operating at a variety of altitudes may be able to carry weather radars and view from above regions not accessible to the fixed ground-based network. For this to occur, UAVs, which proved to be critically important tools in Afghanistan, must be modified to include airborne radars; consequently, a strong case can be made for such a development. The current payload would be a difficult barrier to overcome. The solar-powered NASA HELIOS, developed by Dr. Paul MacCready (who first achieved human-powered flight with the Gossamer Condor), has been tested at altitudes above 80,000 feet and is expected to be able to gain 100,000 feet. The current system has such a limited payload (thought to be 150 pounds) that radar remote sensing would be prohibited, but the emphasis on UAVs may allow for an increase of payload, or unique ways may be found to develop very
small radars suited to the platform. Some of these UAVs operate at low altitude, and small radar could be used. High-altitude UAVs like the jet-powered Northrop Grumman Global Hawk (a long endurance aircraft that flies above 60,000 feet) could be an excellent platform.
To fill vital gaps in precipitation observations over the oceans and to provide calibration of satellites over the oceans, consideration should also be given to the use of existing and new radars and radiometers on board intercontinental commercial aircraft. The data from existing weather radars on these aircraft would serve to fill in the data-void regions until precipitation satellites are able to cover the globe with adequate time and space resolution. Calibration of the airborne radars would also provide a transfer calibration to the space-borne systems.
There are many cases in which transcontinental aircraft fly above significant weather that goes unreported. At such times it would be useful to switch the radar to a vertically pointing antenna below the aircraft. In the near future it is expected that airborne radars will be coherent and capable of Doppler measurements. By beam switching the antenna fore and aft and left and right, it would be possible to obtain excellent measures of the wind profile as well as quantitative measurements of the precipitation.
It is also worthwhile to consider equipping commercial aircraft with synthetic aperture radar (SAR) and scatterometers. An airborne C-band scatterometer is in operation on the Gulfstream aircraft operated by the National Oceanic and Atmospheric Administration (NOAA) Office of Aircraft Operations (OAO). This latter system provides both measurements of the near sea-surface winds and velocity azimuth display (VAD) of the winds above the surface.
Recommendation—Visionary
The capabilities of Unmanned Aerospace Vehicles and piloted aircraft to carry weather radar payloads should be monitored for their potential to provide weather surveillance over the continental United States and over the oceans.
SPACE-BASED RADAR
The first satellite dedicated to the measurement of rainfall—the Tropical Rainfall Measurement Mission (TRMM)—was launched in November 1997 and is expected to maintain observations until 2007, thus establishing an important climatological record. TRMM has performed beyond all expectations and has contributed in many ways that were not anticipated prior to launch:
-
TRMM has greatly enhanced estimates of the zonal distribution of rain over the tropics,
-
Assimilation of data on rainfall and total precipitable water into TRMM has improved predictions of the hydrological cycle, clouds, and radiation in global computer analyses,
-
TRMM has aided in the monitoring of the evolution of precipitation from the 1998 El Niño to the 1999 La Niña and the associated changes in storminess and precipitation around the tropics,
-
TRMM has provided a single transfer standard for calibrating ground-based radars around the world.
Space-borne weather observations have the same advantages as satellite-borne platforms for all disciplines—coverage and communication. TRMM has proved the technology of space-borne weather radar in terms of stability, coverage, range/azimuth resolution, and observation window. However, the azimuthal swath is quite narrow (220 kilometers), so that it only overlaps about a third of the swath of the on-board microwave radiometer. This creates problems when one tries to “extend” the rain profile derived from the precipitation radar out to regions beyond the radar swath. Also, the long intervals between successive observations limit the utility of space-borne observations for weather prediction.
In addition to providing rainfall measurements, TRMM has also provided high quality measurements of the ocean surface cross section; the latter are also well related to the near surface winds. We therefore anticipate that a future radar-radiometric satellite will provide data on both precipitation and ocean surface winds.
It is expected that TRMM will be followed by the global precipitation mission (GPM), the orbit of which orbit will extend to extratropical latitudes (±60°). It is planned that GPM will carry a dual-wavelength radar system for more accurate precipitation measurements. In addition GPM will fly a constellation of microwave radiometers to permit a three-hour revisit time, thus allowing the use of the data for weather prediction.
Significant advances in micro-satellite technology combined with radio frequency (RF), power, and receiver subsystems for space may provide an opportunity to bring space-borne surveillance radar within affordable levels in the next two decades. Indeed, Cloud Sat is another planned space-borne radar mission intended for launch in 2005.
Among the various technological advances will be the deployment of numerous micro-satellites that can fly in low earth orbits and can selectively provide continuous coverage for North America. This assumes implementation of other technologies that are under development for space applications: distributed-aperture systems, adaptive deployment, and spot beam or electric scanning. The COSMIC system, discussed earlier, is a nonradar example of such a constellation.
Multiple low earth orbiting satellite systems provide continuous coverage, whereas distributed aperture systems provide narrow footprints at subrun levels.
Spot beam and electronic scanning technology enable adaptive deployment. Although there are many advantages to satellite-borne systems, they still suffer a basic limitation of contamination by ground clutter at near-surface observations. In summary, space technologies may advance to a point where affordable space-borne weather surveillance radars can play an ancillary role in conjunction with ground-based radars.
Measurements of surface winds over the oceans by space-borne scatterometers and microwave radiometers have been made possible by satellite systems such as the NASA Scatterometer (NSCAT), by the scatterometers on the European Remote Sensing satellites (ERS-1 and 2), by QuickSat, and by the Special Sensor Microwave/Imager (SSM/I) on the Defense Meteorological Satellite Program (DMSP). The impact on global and regional forecast skill has been assessed by several organizations and is reviewed by Atlas et al. (2001). The key portions of their summary follow.
Scatterometer data over the oceans are able to delineate precise locations and structures of significant meteorological features, including cyclones, anticyclones, fronts, and cols. As such, their use by marine forecasters can result in improved analyses, forecasts, and warnings for ships at sea and other marine interests. The use of Scatterometer observations in data assimilation systems can extend their usefulness substantially and lead to improved sea level pressure analyses, improved upper air analyses of both wind and geopotential, and improved short and extended-range numerical weather forecasts. Typically, forecast improvements are skewed toward the Southern Hemisphere extratropics, with the length of a useful forecast extended by as much as 24 h in some Data Assimilation Systems. In the Northern Hemisphere extratropics forecast impact is close to neutral or slightly positive, but occasionally the depiction of an individual storm is improved substantially, leading to a more skillful forecast.
Finally, observations of the ocean surface by airborne and space-borne SAR have revealed heretofore unobservable atmospheric processes by virtue of the effects of the winds on the development of the short surface waves. The nature, intensity, and direction of storms have been deduced from the pattern of SAR echoes on the sea. SAR has also observed cyclonic circulations in the lower atmosphere prior to the development of hurricanes.
Recommendation—Visionary
The capabilities of future space-based radar systems to supplement ground- based systems should be determined.