3
Technology Development
Historically, the fields of solar and space physics were founded on ground-based observation of the Sun and the near-Earth space environment. Great advances occurred during and after the International Geophysical Year 1957-1958, when globally coordinated, ground-based observations and measurements made by balloons, sounding rockets, and orbiting spacecraft became available. Many of the most fundamental discoveries in solar and space physics were made with these early systems, which employed “borrowed” technology such as captured V2 rockets and intermediate-range ballistic missiles. As time went by, dramatic advances in spacecraft technology, and particularly in scientific instrumentation, led to the rapid and complete reconnaissance of geospace and the identification of dynamic features of the solar corona that are closely connected with geomagnetic activity. Studies of coronal activity benefited greatly from the deployment of solar imagers on the Skylab space station. In 1973-1974, for example, these imagers verified and extended earlier rocket measurements identifying coronal holes as the source of high-speed solar wind streams. Rapid development of both ground- and space-based solar imagers has enabled multispectral imaging of the Sun with ever higher resolution, now in the sub-arcsecond range. These observations, together with theory and numerical simulations, have come closer than ever to revealing how electromagnetic energy is explosively converted to plasma kinetic and heat energy in the solar corona.
During the more than four decades of the space age, the magnetic and gaseous environments of eight of the planets and of several planetary satellites and comets, along with different regions of the heliosphere, have been explored to varying degrees. Prior to the first dedicated dual-spacecraft mission in 1977, simultaneous measurements at several locations in geospace had been achieved on a hit-or-miss basis since the mid-1960s. The ability to make more than single-point measurements enabled research-
ers to establish the characteristic structure and motions of various plasma boundaries and shocks in geospace. Understanding of the global dynamics of Earth’s magnetosphere was spurred by the advent of global auroral imaging in 1981 and more recently (2000) by the comprehensive imaging of inner magnetospheric plasmas. Targeted in the future is the three-dimensional dynamic “imaging” of magnetic fields and charged particle populations in large parts of the magnetosphere using constellations of many tiny spacecraft.
Theoretical understanding of solar and space physics has been aided greatly by the rapid development of computing technology, which has allowed investigations of the global magnetohydrodynamic behavior of space plasmas as well as of their kinetic behavior on a local scale. Large data sets are being collected, and the assimilation of these data into physics-based models is an important intermediate goal in the search for an ultimate predictive understanding of planetary space environments.
While the above-mentioned “borrowed” technologies have made possible many of the advances in solar and space physics, the development of new technologies is needed to enable the solar and space physics research communities to address some of the key scientific questions set forth in Chapter 1. The committee has identified seven main areas in which focused technology development, based on the immediate and projected needs of solar and space physics research, is required:
-
Sending spacecraft to the planets and beyond as efficiently as possible;
-
Developing highly miniaturized spacecraft and advanced spacecraft subsystems for missions involving constellations of multiple spacecraft;
-
Developing highly miniaturized sensors of charged and neutral particles and photons;
-
Gathering and assimilating the data from multiple platforms;
-
Integrating large space-physics databases into physics-based numerical models;
-
Deploying reliable, unmanned, ground-based ionospheric and geomagnetic measurement stations; and
-
Developing a high-resolution, ground-based solar imager.
Progress in each of these areas will require (1) recognition of the limitations of present technologies; (2) development of new technologies such as in-space propulsion, miniaturization, and low-power techniques for spacecraft systems and more capable ground-based instrument arrays; and (3)
investment in existing technological capabilities to maximize productivity and efficiency. The committee expands on each of these issues below.
TRAVELING TO THE PLANETS AND BEYOND
New propulsion and power technologies are needed to enable further planetary and heliospheric missions. An important part of any space mission is the ability to loft a spacecraft into space and propel it to its intended orbit or destination. At present this task is achieved by low- or moderate-technology propulsion systems operated by highly sophisticated avionics. Limited options are provided by the fleet of launch vehicles in the United States, and the frequency of their use for solar and space physics is insufficient to reduce costs below the levels that the solar and space physics research community has come to accept. There is also no new, groundbreaking technology that promises to change this constraint. It should be recognized, therefore, that for small- and moderate-class missions, launch costs will remain a significant fraction of the overall mission costs. However, as discussed in Chapter 7, there is a new DOD program to develop launch systems that could potentially provide very-low-cost access to low Earth orbit for small (75-100 kg) spacecraft.
Near-Earth missions slated for launch in the relatively near term will use hydrazine thrusters to provide impulsive changes in velocity for station keeping—required, for example, for magnetospheric multiprobe missions— and for excursions to low altitudes by the Geospace Electrodynamic Connections mission. However, many future missions require satellite trajectories that are not possible without continuous thrust capability. NASA has therefore created the In-Space Propulsion (ISP) program within the Office of Space Science to research and develop advanced propulsion and associated power systems that will supply the needed continuous thrust capability. Two propulsion technologies that are receiving particular attention within the ISP program are solar sails and nuclear electric propulsion.
Solar sails (see Figure 3.1) have long been envisioned as a simple, inexpensive means of propulsion that could provide access to and maintenance of unstable orbits that would otherwise require large, expensive propulsion systems. The potential of solar sails is being explored for a number of space physics missions proposed or now under study, including Solar Wind Sentinels, ESA’s Solar Orbiter, the Solar Polar Imager, and the Interstellar Probe. The Planetary Society is sponsoring a solar-sail demonstration, with a target launch date of 2003. NASA’s Office of Space Science has issued a solicitation for in-space propulsion technology (ROSS-2002)

FIGURE 3.1 Artist’s concept of a solar-sail-powered spacecraft. Solar sails, which use the radiation pressure of sunlight for propulsion or station keeping, are being considered for an Interstellar Probe and, within the Living With a Star program, for a multispacecraft “sentinel” mission to study the evolution of coronal mass ejection-driven disturbances and other solar wind structures as they propagate through the inner heliosphere. Courtesy of NASA Marshall Space Flight Center.
that includes a request for the development of an engineering model of a prototype solar sail system and for the development of a simulation package to validate sail performance. Because of the time required for development, testing, and validation, use of solar sails for actual solar and space physics missions is likely to be some 7 to 10 years in the future.
Missions to the outer planets and beyond, including an Interstellar Probe, require propulsion capabilities that significantly exceed those of the present fleet of launch vehicles. Nuclear electric propulsion (NEP) is being considered as a promising solution to the problem of providing the high-performance propulsion capabilities that such deep-space missions will need. The NASA 2003 budget submitted to Congress contains funding to support the study of NEP technology, and the ROSS-2002 NASA research announcement solicits proposals for propulsion and power studies that will lead eventually to the development of an NEP capability. When such a propulsion technology becomes available, there is little doubt that an Interstellar Probe will become one of the most exciting of the future missions that could reach beyond the solar system.
Although recent advances in high-efficiency solar arrays can support small- and moderate-scale missions to Jupiter, radioisotope thermoelectric generators (RTGs) are needed for most missions to the outer solar system. They might also simplify the design of a Solar Probe mission and make multiple passes into ~4 solar radii a possibility. Pioneers 10 and 11, Voyagers 1 and 2, and the Ulysses, Galileo, and Cassini missions have all used RTGs as the source of their electrical power, with the Voyager and Pioneer missions having demonstrated long-term (>25-year) reliability. The 2003 NASA budget provides funding for the renewed production of RTGs.
Recommendation: NASA should assign high priority to the development of advanced propulsion and power technologies required for the exploration of the outer planets, the inner and outer heliosphere, and the local interstellar medium. Such technologies include solar sails, space nuclear power systems, and high-efficiency solar arrays. Equally high priority should be given to the development of lower-cost launch vehicles for Explorer-class missions and to the reopening of the radioisotope thermoelectric generator (RTG) production line.
These advanced technologies include solar sails, space nuclear power systems, and high-efficiency solar arrays. Equally high priority should be given to the development of lower-cost launch vehicles for Explorer-class missions and to reopening the RTG production line.
ADVANCED SPACECRAFT SYSTEMS
The development of highly miniaturized spacecraft and advanced spacecraft subsystems is needed to enable planned and proposed missions that involve constellations of multiple spacecraft. NASA has studied several
science missions that require multiple distributed satellites, including the Magnetospheric Constellation (MagCon) mission. MagCon is an example of a science mission that is driving “nanosatellite” technology development. Meeting the objectives of this mission will require multiple simultaneous in situ observations from 50 to 100 spacecraft, which in turn is driving technology on many fronts. Especially important is the need to minimize spacecraft mass, size, power, and cost. Each satellite subsystem function must be examined to determine if reductions are possible, or if it can be combined with other functions or even eliminated. In addition, to generate such a fleet of nanosatellites on a normal development schedule requires that they be mass-produced, a process that would be facilitated by highly integrated instrumentation and spacecraft subsystems. Several of the technologies required for MagCon will be flight-tested by the New Millennium Program’s Space Technology 5 mission, which is planned for launch in 2004 and will place three nanosatellites (mass = 19 kg) into geostationary orbit (see Figure 3.2).
To reduce the mass and power requirements of satellite subsystems means that some emphasis must be placed on new components and technologies, such as microelectromechanical systems (MEMS) and micro-thrusters (thrusters on a chip) for attitude control.1 It also means developing much denser, more capable, and lower-power satellite data processing and control subsystems. Much effort is currently being put into developing low-power, high-density, high-speed application-specific integrated circuits, which cover the gamut of analog, digital, and hybrid analog/digital devices. It is hoped that they will achieve a significant reduction in satellite mass and power resource requirements as well as in the overall cost of the systems.
Other areas of technological concern include the injection of a large number of spacecraft in pre-determined orbits from one or a few “dispenser payloads”2 and station keeping to maintain optimum spacecraft positioning.
Recommendation: NASA should continue to give high priority to the development and testing of advanced spacecraft technologies through such programs as the New Millennium Program and its advanced technology program.
ADVANCED SCIENCE INSTRUMENTATION
The development of highly miniaturized sensors of charged and neutral particles and photons will remain a critical and continuing focus of space-
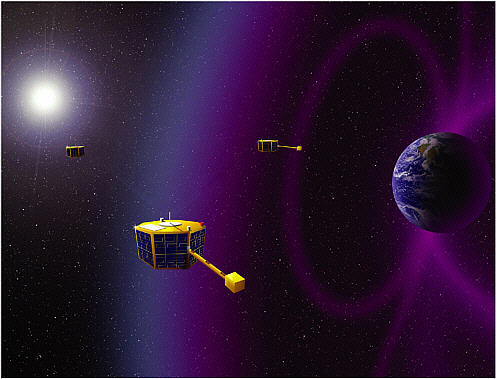
FIGURE 3.2 The New Millennium Program’s Space Technology 5 (ST 5) mission, planned for launch in 2004, will test advanced spacecraft technologies required for future space physics missions involving constellations of miniaturized satellites. Lessons learned from ST 5 are expected to be incorporated in the Magnetospheric Constellation mission, a Solar Terrestrial Probe mission that will deploy 50 to 100 small satellites to study the structure and dynamics of Earth’s magnetotail. Courtesy of NASA Goddard Space Flight Center.
research-related technology development during the coming decade. Advances in instrumentation generally provide increases in capability with a reduction in the required resources. Space science instrument development both drives advanced technology and utilizes emerging technologies at the forefront of materials science. Miniature particle multipliers and counters and charge-coupled device arrays for photon detection are two examples of emerging technologies that have significantly advanced space instrument capability. MEMS approaches can be used for adaptive apertures that
extend the dynamic range of detectors. Miniaturization of micro-channel plate detectors and amplifiers on silicon substrates will allow low-power, high-sensitivity particle detectors to be constructed. Microcalorimeters (superconducting tunnel junctions and transition edge sensors) may be fabricated as pixel arrays, allowing unprecedented spatial and spectral resolution at wavelengths ranging from the infrared to the hard x ray. Doppler imaging of atmospheric emissions, allowing large-scale descriptions of charged and neutral dynamics, is currently being examined. Carbon annotates are being investigated to produce a low-power source of cold electrons for mass spectrometry and other applications.
Several programs within NASA’s Office of Space Science fund instrument definition and development activities. The Planetary Instrument Definition and Development Program (PIDDP) supports studies of instrument concepts that will lead to the development of flight instruments to be proposed for specific planetary missions. While most of the instruments that have come out of this program are designed for the investigation of planetary atmospheres and surfaces, some space plasma physics instruments have been developed with PIDDP support as well, e.g., the energetic neutral atom component of the Magnetospheric Imaging Instrument on the Cassini Saturn Orbiter and the “hockey puck” energetic particle detector to be flown on MESSENGER. Such examples are few, however. Until recently, a program equivalent to the PIDDP did not exist for instrumentation for solar and space physics applications. This situation changed with the NRA 02-OSS-01, which provides for both a Sun-Earth Connection instrument development program and a Living With a Star geospace instrument development program.
Recommendation: NASA should continue to assign high priority, through its recently established new instrument development programs, to supporting the development of advanced instrumentation for solar and space physics missions and programs.
GATHERING AND ASSIMILATING DATA FROM MULTIPLE PLATFORMS
Missions involving constellations of spacecraft will place challenging requirements on operations and data acquisition systems. The need to resolve the spatial and temporal scales that describe the dominant physical processes in solar system plasmas has now become of paramount importance. The description of future flight missions inevitably includes the concept of multipoint measurements in the regions of interest. Such multi-
point measurements, whether in the outer magnetosphere, the solar wind, or low-Earth orbit, offer unique challenges to spacecraft command and data-handling systems. Previous missions generally involved single autonomous spacecraft with data retrieval that was dependent only on scheduling a suitable contact time from a single ground station. Future missions will involve operation of many satellites that may be triggered by information from just one, and data transfer to and from the ground must accommodate multiple satellites in the same orbit with conflicting contact times. Such operational scenarios already exist, for example, in satellite telephone systems, but they have yet to be implemented in NASA science missions. The success of such future initiatives will require examination of the effectiveness of the Deep Space Network for many near-Earth missions. The construction of medium-range antennas might be more appropriate for such missions, and an appropriate distribution of more modestly sized antennas would allow some flexibility in the data acquisition plans. Finally, the growing number of receiving stations for missions in low Earth orbit will require coordination so that data from many sources can be consolidated and become transparent to the end user.
Recommendation: NASA should accelerate the development of command-and-control and data acquisition technologies for constellation missions.
MODELING THE SPACE ENVIRONMENT
The coming decade will see the availability of large space physics databases that will have to be integrated into physics-based numerical models. Data assimilation techniques were first used in numerical weather prediction. Meteorologists were confronted with having to solve an initial value problem without the correct initial data. Specifically, synoptic observations were insufficient to initiate a model run that could be used to predict the weather. To overcome this obstacle, meteorologists developed a methodology that is now called “data assimilation.” This methodology uses data obtained at various places and times, in combination with a physics-based (numerical) forecast model, to provide an essentially time-continuous “movie” of the atmosphere in motion. During the last 40 years, meteorologists have continually improved their ability to predict the weather, as a result of both model improvements and a large infusion of new satellite and ground-based data. Following the example set by meteorologists, oceanographers began to use data assimilation techniques about 20 years ago,
reaching the point where they were able to successfully predict the coming of the last El Niño.
Owing primarily to the relatively limited number of measurements of the near-Earth space environment, the solar and space physics community has not until recently had to address the issue of data assimilation as seriously as have the meteorologists. However, this situation is changing rapidly, particularly in the ionospheric arena. Within 10 years, it is anticipated that vast arrays of data sets will be available for assimilation into specification and forecast models such as those to be developed in the NASA Living With a Star theory and modeling initiative. The data include in situ and imaging data from numerous satellites, altitude profiles from a large network of ground-based ionosondes, and line-of-sight total electron content (TEC) and optical measurements between constellations of low-Earth-orbit and geostationary satellites and between the satellites and thousands of ground stations.
An increasing emphasis is being placed on understanding space science from a systems perspective, which ultimately requires access to reliable geophysical and solar-heliospheric parameters without regard to their origin or the instrumental details that underlie their derivation. These data will appear in a variety of formats and apply to quite disparate regions of space, and yet they must be accessible in a format that allows scientists to view and/or manipulate them in a manner dictated by the analysis procedures employed. The increased use of physics-based data assimilation will also require that renewed attention be given to data quality and to the generation of uncertainty estimates. The planned constellation-type missions, for example, will require parallel data assimilation techniques through which the spacecraft data can be directly injected into global magnetohydrodynamic models of the magnetosphere.
Recommendation: Existing NOAA and DOD facilities should be expanded to accommodate the large-scale integration of space- and ground-based data sets into physics-based models of the geospace environment.
OBSERVING GEOSPACE FROM EARTH
A global network of tended and autonomous ground-based ionospheric and geomagnetic measurement stations will continue to be an invaluable source of data on Earth’s space environment during the next decade. Since the International Geophysical Year, ground-based observatory networks,
particularly at high geographic latitudes, continue to be important. Several programs, such as the Canadian Auroral Network for the OPEN Program Unified Study (CANOPUS), have provided long-term observation facilities that are sometimes widely distributed in latitude, but nearly always localized in longitude.3 Coordination provided by international programs such as the International Solar-Terrestrial Physics program has allowed for more widespread coverage by probes such as ionospheric radars and riometers,4 magnetometers, all-sky cameras, and meridian-scanning photometers. In the near future it would be extremely beneficial for the United States to have such a network that is operating continuously and providing data in real time.
Tomography has been used extensively by the medical community for several decades, but it was not until about 1988 that this technique was first applied to the ionosphere. With radio tomography, transmissions from a low-Earth-orbiting satellite (or satellites, such as the planned Republic of China Satellite (ROCSAT) array) are received along a chain of stations with the intent to determine the TEC along the ray paths. Optical tomography works in a similar way, but uses airglow emissions instead of TECs. Currently, tomography chains in the United States, South America, Europe, Russia, and Asia provide information about ionospheric weather in these local regions. During the next decade, tomography is anticipated to play an important role in elucidating ionospheric weather features.
While the large radar facilities still require human tending, many of the smaller sensor systems and arrays have been deployed autonomously in the polar regions. However, the severe environments of temperature and moisture and the wildly varying solar insolation5 have posed a serious reliability problem for these systems, to the point that their existence is now threatened.
Recommendation: The relevant program offices in the NSF should support comprehensive new approaches to the design and maintenance of ground-based, distributed instrument networks, with proper regard for the severe environments in which they must operate.
OBSERVING THE MAGNETIC SUN AT HIGH RESOLUTION
Developing the capabilities needed for the high-priority Advanced Technology Solar Telescope (ATST) requires that several important technical issues be addressed. Knowledge of the Sun has reached the point where a new, large-aperture solar telescope is needed to make observations of small-
scale interactions between magnetic fields and plasmas.6 Recent break-throughs in adaptive optics have eliminated the major technical impediments to making observations with sufficient resolution to measure the pressure scale height and the photon mean free path length, the fundamental length scale in the solar atmosphere. The proposed ATST is a 4-m-class adaptive optics system that is designed to make the required measurements.
Development of the ATST presents several challenges not faced with large nighttime telescopes. The enormous flux of energy from the Sun makes thermal control a paramount consideration, both to remove the heat without degrading telescope performance and to control mirror seeing. To achieve diffraction-limited performance, a powerful adaptive optics system is required that operates at wavelengths from the visible to the infrared using solar structure as the wavefront sensing target. Low scattered light is essential for observing the corona and for measuring accurately the physical properties of small structures in sunspots, flux tubes, and magnetic pores.
While these challenges are well understood, a systematic program of technology development will be required in support of a timely development of the ATST. Progress in this direction is already under way as the National Solar Observatory (funded mostly by the NSF) has invested substantial resources in demonstrating the first solar adaptive optics system, which works with solar granulation as the wavefront sensing target.
Recommendation: The NSF should continue to fund the technology development program for the Advanced Technology Solar Telescope.