IV Process of Dose Reconstruction in NTPR Program
This chapter discusses methods of dose reconstruction for atomic veterans that have been used in the NTPR program. Consistent with discussions on the principles and process of dose reconstruction in Section I.C, this chapter is organized as follows: Section IV.A discusses development of exposure scenarios; Section IV.B discusses methods used to estimate external dose from exposure to photons, neutrons, and beta particles; Section IV.C discusses methods used to estimate internal dose from intakes of radionuclides; Section IV.D discusses dose reconstructions for occupation forces in Japan; Section IV.E discusses methods used to account for uncertainties in estimates of external and internal dose; Section IV.F discusses the approach to estimating total dose from external and internal exposure, taking into account all radiation types and exposure pathways of concern; and Section IV.G discusses documentation of dose reconstructions and quality assurance. The committee’s evaluations of these aspects of the NTPR dose reconstruction program are presented in Chapters V and VI.
As discussed in the standard operating procedures (DTRA, 1997) and in 32 CFR Part 218, the goal of the NTPR program is to obtain upper-bound estimates of dose to atomic veterans, consistent with the policy of giving the veterans the benefit of the doubt in estimating their doses (see Section I.C.3.2). More specifically, the goal is to obtain at least a 95th percentile upper bound of possible doses, taking into account uncertainties in estimating dose. That is, the NTPR program intends that a reported dose should exceed the true dose in at least 95% of all cases and that there should be no more than a 5% chance that the true dose to an individual is higher than the reported value. As discussed in more detail in Sec-
tions IV.B and IV.C, it is the current policy of the NTPR program to report a central (“best”) estimate of external dose from exposure to photons along with an estimated 95th percentile upper bound, and the same approach has been taken in estimating external dose from exposure to neutrons in dose reconstructions for participant groups. However, only a single estimate of dose is reported when a beta dose to the skin or lens of the eye or an internal dose from intakes of radionuclides is calculated, and this estimate is intended to be at least a 95th percentile upper bound.1
IV.A EXPOSURE SCENARIOS
Development of exposure scenarios for participants in the nuclear-weapons testing program involves consideration of assumptions about the locations of the participants of concern, their activities at those locations, and the time spent at each location and assumptions about the radiation environment at the assumed locations of the participants during the time spent at those locations (see Section I.C.2.1). Approaches to development of exposure scenarios used in the NTPR program are described in the standard operating procedures (DTRA, 1997) and in 32 CFR Part 218.
The dose reconstruction process requires that the analyst first determine whether a veteran’s records support his qualifying as a “participant” according to the definition in applicable laws and regulations. In this initial stage, military records are used to confirm that the veteran was present at the Nevada Test Site (NTS) or the Pacific test sites during designated intervals before and after tests of nuclear devices, was present in Hiroshima or Nagasaki during the occupation of Japan, or was a prisoner of war near Hiroshima or Nagasaki at the time of the atomic bombings. The burden of proof in establishing a veteran’s status as a participant is stricter if a veteran’s claim is filed for a presumptive disease under 38 CFR 3.309 than for a nonpresumptive disease under 38 CFR 3.311 (see Section I.B.4).
Once a veteran’s participation status has been confirmed and a dose reconstruction has been requested (usually by the Department of Veterans Affairs [VA] in response to a claim filed for alleged radiogenic health conditions), a government contractor (currently JAYCOR) undertakes extensive historical research based on archival records to reconstruct the movements and activities of the veteran during the period of participation.
IV.A.1 Unit-Based Dose Reconstructions
If nothing in the historical research suggests that the veteran was involved in unusual activities (that is, activities different from those of the other members of his unit), a “unit-based” dose reconstruction may be carried out. An example is participants in units who observed detonations at the NTS from trenches close to ground zero (see, for example, Figure IV.A.1). This one-size-fits-all strategy assigns the same dose to everyone in a given unit, with an upper bound assigned to allow for uncertainty in the estimated dose.
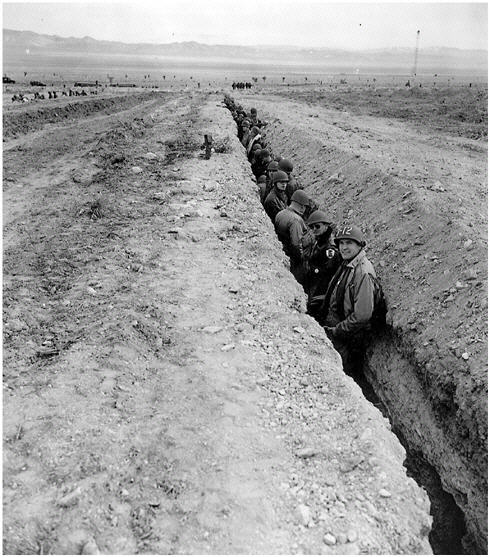
FIGURE IV.A.1 Observers in the trench from which they observed a nuclear detonation.
If no badge records are available, a unit-based dose reconstruction can be based on radiation-monitoring data that were obtained at the time of an operation as part of the test itself. With computer models, the measurements are interpolated and smoothed across space and time (allowing for the physics of radioactive decay) and then combined with historical summaries of the activities of the unit, including the likely path of the unit through the radiation environment, to reconstruct the dose for the unit. If the exact times spent in various locations are not known, assumptions are sometimes applied on the basis of the presumption that radiation-safety policies in force at the time of the test were followed.
IV.A.2 Individualized Dose Reconstructions
If a participant was involved in unusual activities, an individualized dose reconstruction is required. In such instances, there may have been complete or nearly complete badging during the entire time of participation.2 If so, and if the issue and turn-in dates for the badges of record are complete and cover the veteran’s entire time at the site, the badge readings are simply summed, and their variances are combined with a method called quadrature, in which the variance (error) of the summed dose is taken to be the sum of the variances of the individual readings, assuming independence of errors. The per-badge biases and variances are based on modifications of methods proposed in a previous National Research Council report on film badge dosimetry in atmospheric nuclear tests (NRC, 1989).
One issue that often arises in the dose reconstruction process is related to the fact that participants often had a “permanent” badge, which was supposed to be worn throughout their entire time in an operation, plus occasional “mission” badges, which were issued on particular occasions when radiation safety personnel determined that a participant was likely to encounter an unusual potential for exposure. If the “permanent” badge was not worn on such occasions, the proper way to combine the two types of badge readings would be to sum them. If, instead, the two badges were worn contemporaneously, the mission badges can be ignored because any additional dose experienced on a particular mission presumably was already captured by the permanent badge. A dose reconstruction policy requiring the benefit of the doubt to be given to the veteran would require summing the two, and this was sometimes done.
Because badging often was not complete or uncertainties remained (for example, because the issue or turn-in dates were missing—a common problem), an individualized dose reconstruction is required for some intervals of the veteran’s time of participation. The analyst must reconstruct the particular activities and locations of activities that the veteran would have undertaken in the
assumed radiation environment and apply modeled radiation levels to those activities and locations. Difficulty often arises in the reconstruction of the veteran’s experiences.
In some cases, “cohort” film badging is used to assign a unit-based dose. When only a few members of the unit wore a badge during an operation, the mean of those few badge readings can be assigned to all members of the same cohort. The uncertainty in such dose assignments is computed from the variability in the badge measurements.
Dose assignments based on film-badge data are discussed in more detail in Section IV.B.1 and IV.E.2.
IV.A.3 Individualized Reconstruction of Scenarios
The sources of historical information that can be used to reconstruct exposure scenarios include, in addition to such official documents as morning reports and ship logs, narratives written at the time, such as reports of unexpected changes in wind or fallout that complicated the management of radiation exposure for participants at specific tests. Individual information about a veteran’s job type or specific mission responsibilities is sometimes available. Other documents can contribute information on a person’s exposure scenario, such as questionnaires filled out by the veteran (especially early in the NTPR Program) or statements the veteran provided in support of his claim. Occasionally, other people are consulted to clarify uncertainties in what was experienced by a particular veteran, such as his commanding officer or others in the same unit. Surviving widows or children are sometimes contacted when the veteran is deceased, although they usually do not provide much detail. Some of the veterans had been sworn to secrecy for national-security reasons and never described their experiences even to their spouses.
IV.B ESTIMATION OF EXTERNAL DOSE
All estimates of external dose to participants are based on film-badge readings or surveys with field instruments. If the participant wore a film badge and the data could be located, the external gamma dose of record is generally based on those data. If no acceptable film-badge data are available or if the film-badge data do not cover all potential exposures, the external dose for these exposures is based on a “scientific” dose reconstruction that relies on survey data. For most participants, the reconstructed gamma and neutron dose from external exposure is based on a generic dose reconstruction performed for their particular units’ activities during a given test series, modified as appropriate to conform to a participant’s duties and period of exposure.
It is important to note that the method used in the NTPR program to estimate external doses changed over time as shown below (Schaeffer, 2001a).
Year |
Change in Methodology |
1978 |
Individual services (Army, Navy, Air Force, and Marines Corps) report external doses based on film-badge dosimetry |
1980 |
Neutron dose reconstruction added for unit dose reconstructions |
1984 |
Statistical application of military-unit film-badge readings used when a veteran’s film-badge readings are missing |
1988 |
Dose reconstruction applied to periods of incomplete film-badge coverage |
1989 |
Upper-bound doses for individual film-badge data applied |
1990 |
Doses from damaged film badges superseded by reconstructed doses |
1992 |
Total upper-bound doses coupling the estimated uncertainty from film-badge data and reconstructed doses are calculated |
1996 |
Upper-bound doses included in all reports in response to VA claims |
1998 |
Skin-dose calculations added for all skin-cancer claims in response to a VA request |
When changes in policy or method are adopted, there is no systematic way to review earlier dose reconstructions or to apply the changes retroactively; this is discussed in more detail in Section VI.E. The method currently used in the NTPR program to estimate the most likely external gamma dose based on film-badge dosimetry, most likely gamma dose based on a scientific dose reconstruction, most likely neutron dose, and upper-bound beta dose to skin or lens of the eye are discussed in the following paragraphs. The method used to estimate upper bounds of gamma and neutron doses is discussed in Section IV.E.2.
IV.B.1 External Dose Estimation from Film-Badge Data
It is the policy of the NTPR program that if a film badge was issued and worn and valid film-badge data can be located, the film-badge reading is to be considered the dose of record for the period when the badge was worn (see Brady and Nelson, 1985). Thus, the policy for reconstructing a dose to a test participant is first to search for film-badge data. However, during the earlier test series, only a small fraction of test participants were badged. Attempts were made beginning in the 1956 Operation REDWING in the Pacific and the 1957 Operation PLUMBBOB in Nevada to badge all participants. During the earlier test series, mission badges were issued to participants to be worn when some radiation exposure was expected to occur because of the particular duties to be performed, such as maintenance on contaminated aircraft or recovery of contaminated equipment from displays. Civilian and military participants at Operation CROSSROADS numbered about 43,000, but only about 7,000 film badges were issued to the persons thought most likely to receive radiation exposure. Because multiple badges were issued to many of those personnel, only a small percentage of CROSSROADS participants had film-badge records. Often, one or more members of a unit per-
forming similar duties would be issued a “cohort” film badge. For example, only one or two members of each platoon participating in maneuvers during tests at the NTS were badged (for example, see Frank et al., 1982). The data from this cohort badge would provide an estimate of the external dose to the entire group.
Even if permanent or mission badges were issued (see Figure IV.B.1), often the badges or the data from them can no longer be located. For example, although many film badges were issued during Operation UPSHOT-KNOTHOLE in 1953, most of the data from them were lost, and only summary data can be located (for example, see Edwards et al., 1985).
When film-badge data are reported but the data are considered suspect, the NTPR program requests a re-examination of the film by the Department of Energy. If the film is still on file, it is re-examined by a health physicist, and a determination is made as to whether the reading is questionable or highly suspect. Often, film was damaged by heat, water, or humidity, particularly in the Pacific during the REDWING and DOMINIC test series. That was particularly the case if a badge was worn for more than a few weeks (NRC, 1989). Problems with calibration errors also caused film data to be suspect (NRC, 1989). According to current policy of the NTPR program (Schaeffer, 1995; 2002b; 2002e), suspect film-badge data are discarded in favor of a scientific dose reconstruction.
In a 1989 report, a committee of the National Research Council reviewed the method used in the NTPR program to analyze film-badge data (NRC, 1989). The
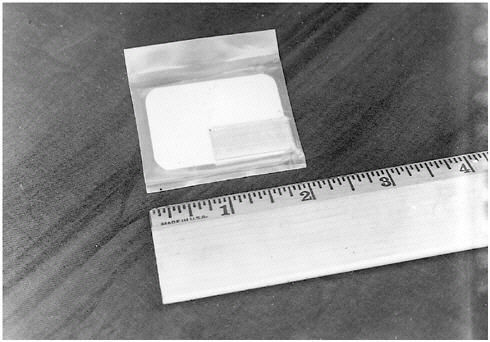
FIGURE IV.B.1 Example of film badges worn by participants at atomic tests.
review examined possible calibration errors and reported heat and water damage, and it recommended that bias and uncertainty factors be applied for each test series. It also recommended how the film-badge data should be reported and how estimates of uncertainty in individual readings and sums of multiple readings should be treated. The NTPR program claims in its letters to VA and the veterans that its reported film-badge data conform to the recommendations of the National Research Council report. However, the NTPR program does not follow the recommendations exactly but has modified them somewhat. In particular, the report recommended that a reported film-badge reading be divided by a bias factor to convert to a whole-body equivalent dose in rem. Most of the bias factor is intended to convert a film-badge measurement of exposure in air in roentgens (R) to a whole-body equivalent dose in rem. However, the NTPR program assumes that the film-badge exposure in R is a direct estimate of the shielded whole-body dose in rem (Klemm, 1989; Flor, 1992).3 Furthermore, all badge readings, rather than the bias-corrected doses inferred from them, are summed to get the total dose. The NTPR program asserts that that is done to preserve a one-to-one correlation with the film-badge record so that a veteran can see evidence that original records are being used in the dose reconstruction and to avoid the perception that the program is lowering recorded doses (Flor, 1992; Schaeffer, 2002b). Estimates of film-badge doses used in dose reconstructions thus are higher, by a factor of about 1.3 or more, than if recommendations by the National Research Council committee had been followed precisely.
In assigning doses based on film-badge data, the NTPR program usually assumes that if a participant was issued both a mission badge and a permanent badge encompassing the same interval, the badges were worn concurrently, as required. Thus, doses recorded by mission badges were generally assumed to be included in the permanent-badge reading. However, if the sum of all mission-badge doses is greater than the permanent-badge reading, the higher value is to be used (Flor, 1992). Similarly, when mission badges were issued but no permanent badge was issued, the mission-badge data generally were adjusted by subtracting the reconstructed dose from routine exposure to fallout during the period that the mission badge was supposed to have been worn. This procedure is used because that dose presumably would have been included in the mission-badge reading.
IV.B.2 External Gamma-Dose Estimation Based on Dose Reconstruction
Because only a fraction of participants were issued film badges during the earlier test series and the time when badges were worn often covered only a portion of the time of potential exposure, methods have been developed to recon
struct the external gamma dose to unbadged participants on the basis of available monitoring data and physical models.
Generic average external doses have been estimated for all major units participating in each test series. The results of the generic assessments and the methods used to estimate external dose have been published in a series of reports issued mainly in the 1980s. Examples of such unit dose reconstructions include calculations for observers at NTS tests (Barrett et al., 1987), maneuver troops at NTS tests (Edwards et al., 1983; 1985), garrisons on the headquarters islands at the Pacific test sites on Enewetak and Bikini atolls (Thomas et al., 1982; 1983a; 1983b; 1984), sailors on support ships (Weitz et al., 1982; Thomas, 1983a; 1983b), boarding parties on target vessels (Weitz et al., 1982), and occupation troops at Hiroshima and Nagasaki (McRaney and McGahan, 1980). The published unit-dose reports referred to above are supplemented by internal memoranda in which daily doses are estimated for specific ships and islands and for smaller units at NTS tests (Frank, 1982; Ortlieb, 1991; 1995; Phillips, 1983; Thomas, 1985; Weitz, 1995a; 1995b; 1997). For example, the Ortlieb (1995) memorandum gives daily dose tables for seven support units at Operation UPSHOT-KNOTHOLE: the 505th Signal Services, the 412th Engineer Construction Battalion, the 3623rd Ordinance Company, the 77th Army Band, the 93rd Army Band, the 371st Evacuation Hospital, and the 163rd Quartermaster Laundry Company.
On the basis of those unit dose reconstructions, the NTPR program assigned a generic dose to all participants in the units. Unless a formal dose reconstruction is requested as a consequence of a VA claim or a specific participant request to the Defense Threat Reduction Agency, the participant’s dose of record is generally based on either a film-badge measurement or the assigned average dose for the participant’s unit. The applicable unit dose reconstructions are usually the starting point for a scientific dose reconstruction for a specific person.
IV.B.2.1 Unit Dose Reconstructions at the NTS
External doses to military units participating as observers or in maneuvers at NTS tests were based on estimates of the location of troops versus time, as obtained from documented unit activity histories, mission plans, and rehearsals (Goetz et al., 1979; 1980; 1981). Shielding provided by vehicles, trenches, and so on, was estimated from radiation transport calculations (Edwards et al., 1983). Computerized interpolation schemes were used to estimate dose rates at various grid locations and times from the available exposure-rate data (Edwards et al., 1985). Separate dose estimates have been made for direct exposure to prompt gamma and neutron radiation in trenches, from exposure to fallout and from overhead debris clouds during observation of a shot from trenches or during post-shot maneuvers, from exposure to fallout-contaminated fields from previous shots during pre-shot rehearsals, and from observation of contaminated displays set up
to study blast and radiation effects. For example, Table IV.B.1 summarizes calculated unit doses and upper and lower confidence limits of a 90% confidence interval for maneuver troops participating in tests during the UPSHOT-KNOTHOLE series at the NTS (Edwards et al., 1985). As indicated in the table, external doses from prompt radiation during observation of the tests from trenches were generally smaller than doses received from residual gamma radiation during post-shot maneuvers and touring of contaminated display areas (see Figures IV.B.2 and IV.B.3). The estimated confidence intervals are discussed further in Section IV.E.2.
TABLE IV.B.1 Summary of unit dose reconstruction for maneuver troops at Operation UPSHOT-KNOTHOLE (Edwards et al., 1985); BCT = Battalion Combat Team. Estimated lower and upper confidence limits shown are doses to add or subtract from tabulated dose to obtain 5th and 95th percentiles of distribution, respectively.
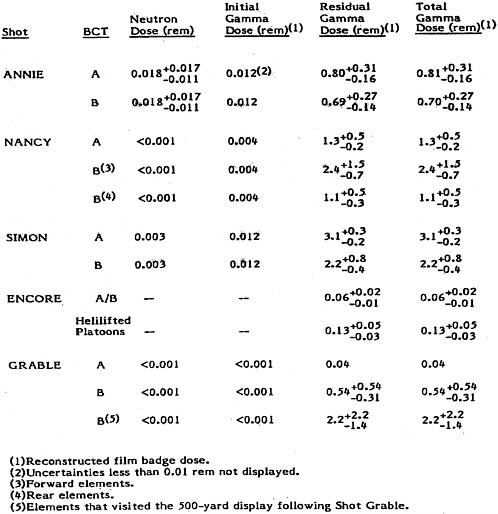
IV.B.2.2 Unit Dose Reconstructions for Pacific Test Sites
Average generic doses to military units at the Pacific test sites are based on estimates of radiation exposure rate versus time. Those estimates are often based on spare data from post-shot ship and island surveys and from radiation monitors accompanying maneuver troops (see Figure IV.B.4). The total time-integrated dose is estimated from the initial exposure rates and estimated or measured decay rates. Tables of daily doses received by sailors were constructed for each ship participating in tests and for participants on various islands. Doses to ship crews were estimated from exposure to fallout on deck (topside), from direct exposure to contaminated seawater topside, from radiation below decks due to contamination of piping and engineering spaces, and from direct exposure during approach toward contaminated target ships (Weitz et al., 1982; Thomas et al., 1984). Doses to engineering crews were generally estimated to be higher than doses to average crew members because of greater proximity to pipes and condensers contaminated by radionuclides in the seawater below decks. Thus, radiation exposure to some naval participants, such as in Operation CROSSROADS, was actually higher to crew members when below decks than when on decks.
Models were developed to describe doses due to contamination of piping and seawater and to proximity to contaminated vessels (Weitz et al., 1982). Generic estimates of time spent indoors vs outdoors or above versus below decks on ships and generic estimates of shielding provided by housing on islands or ship structures are used to modify the free-in-air exposure-rate estimates for sailors on ships or for island-based personnel. Generally, the reconstructions assume that sailors are topside for an average of 40% of the time during three periods daily and below decks the remainder of the time. It is assumed that exposure below decks is minimal because of shielding by steel decks and structures that reduces the exposure rate to an average of about 10% of the topside rate (Thomas et al., 1982; 1984). It is generally assumed that personnel billeted on islands spent 60% of the time outdoors and 40% indoors and that shielding provided by a building structure reduced indoor doses by an average of 50% compared with the outdoor dose (Thomas et al., 1982; 1984).
Daily dose rates from gamma rays for each ship or island were calculated by decaying the reported mean of initial survey data for a particular ship or island for each fallout episode and applying the generic outdoor and indoor fractions and shielding factors discussed above. A generic decay factor of t−1.2 (where t is the time, in hours, after the time of the blast) was generally assumed unless actual data were available. If there were multiple fallout episodes, the residual fallout level from the previous episode was subtracted from the mean of the new survey data to calculate later dose rates in the days after the new event. Thus, the doses from each fallout event are calculated separately for each day and then added. The total dose for any person is calculated either by summing the daily doses during documented periods of potential exposure based on military records (if
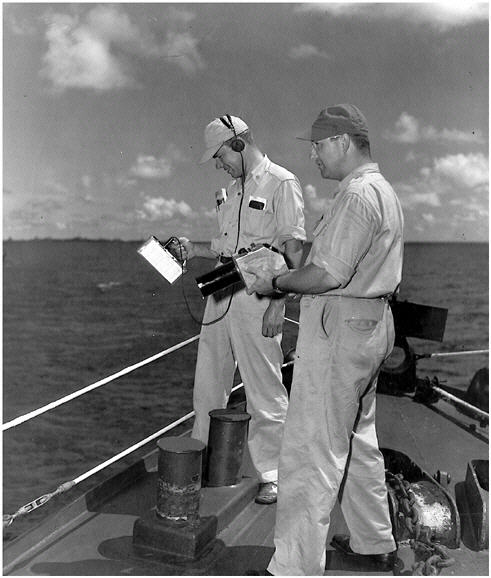
FIGURE IV.B.4 Participants conducting radiation survey on deck of a ship.
from multiple events) or by integrating over the period of exposure by using the nominal t−1.2 decay rate or the actual decay rate if it is measured. The tabulated daily dose rates for later times are considered “high-sided,” in that any decontamination or weathering after the radiation survey usually was not considered in making the estimates.
The 1946 CROSSROADS test series was one of the largest with respect to the number of participants and potential exposures. More than 36,000 persons on
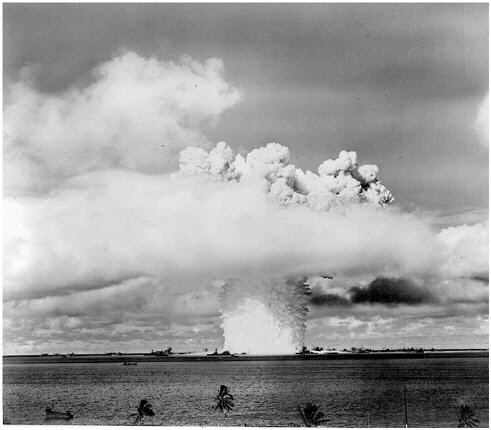
FIGURE IV.B.5 Photograph of underwater Shot BAKER in Bikini Lagoon.
154 support ships participated in this exercise (Weitz et al., 1982). Most of the radiation dose was from Shot BAKER, an underwater test conducted in Bikini Lagoon (see Figure IV.B.5). During that test, a fleet of 88 unmanned target ships was anchored in the lagoon and was heavily contaminated by the wave of contaminated water generated by the blast and by heavy fallout. Personnel of support ships entering the lagoon after the shot were exposed to contaminated seawater, which also contaminated ship internal piping, as discussed above. Other personnel were exposed while serving on boarding parties sent to inspect damage on the target ships or to decontaminate and re-man some of the ships. The NTPR program has constructed curves of daily doses for each support and target ship (Weitz et al., 1982, Volumes 2 and 3) on the basis of monitoring data and decay extrapolations. An estimated decay rate of t−1.3 was adopted for the dose rates on target ships; this rate was based on measurements on various target ships and is higher than the nominal t−1.2 decay rate normally assumed. The plotted daily doses are means for topside, below decks, and amidships, and often they are based on very sparse data. An example of the average daily dose rate on one such
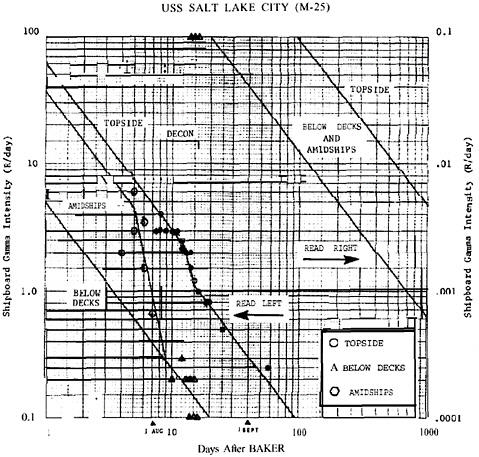
FIGURE IV.B.6 Average daily doses on USS Salt Lake City at Operation CROSSROADS, Shot BAKER (Weitz et al., 1982, Vol. 2).
target ship, the USS Salt Lake City, is shown in Figure IV.B.6. The daily doses to most nonbadged people serving on a particular support ship or boarding a particular target ship were calculated from these curves by using the onboard times that are documented in the support-ship logs. Some daily-dose estimates for particular people were based on cohort film-badge data, if available.
IV.B.3 Estimation of Neutron Doses
Estimates of dose due to external exposure to neutrons produced in a detonation (mainly for some close-in observers at NTS shots and crews of cloud-sampling aircraft) are based on calculations with radiation-transport models. The models calculate the free-in-air exposure in air as a function of distance from ground zero and estimate the shielding provided by trenches and aircraft to calcu
late a dose in tissue in rad (Goetz et al., 1985). The whole-body equivalent dose in rem is then calculated by applying an estimated spectrum-weighted quality factor (QF) of 13 (Goetz et al., 1985); QF accounts for the biological effectiveness of neutrons relative to that of gamma rays in inducing stochastic radiation effects, such as cancer. However, a QF of 8.5 was used for participants at the PLUMBBOB test series (Goetz et al., 1979). The dose to any organ or tissue from external exposure to neutrons is assumed to be the same as the equivalent dose to the whole body.
IV.B.4 Estimation of Beta Dose to Skin and Lens of the Eye
Energetic electrons are emitted by most fission and activation products and were an intrinsic component of the radiation to which atomic veterans were exposed. Most of the electrons are beta particles, which are electrons emitted as a direct result of a radioactive decay process in which a neutron transmutes to a proton and an electron is discharged. As beta particles from sources outside the body enter tissue, the dose falls off rapidly with depth, and tissues and organs lying deeper than 10 mm in the body are unaffected. Thus, beta particles are appropriately ignored in considering external dose to most tissues and organs, which lie deeper than 10 mm, and for them the appropriate quantities are gamma dose and neutron dose. The two exceptions are the skin, with its sensitive component (basal cells) at a depth of 0.07 mm, and the eye, with its sensitive component (lens) at a depth of 3 mm. The potential contribution from beta particles should be considered whenever the dose to skin or the lens of the eye is assessed.
The current method used in the NTPR program to assess beta-particle doses from sources outside the body is described by Barss (2000). Before 1998, skin doses were not estimated in the NTPR program except on a case-by-case basis (Schaeffer, 2002c). Before the 2000 publication of the Barss report, beta doses were computed by using information from references cited in the publication (Schaeffer, 2002c), principally the user’s manual for the CEPXS radiation transport code (Lorence et al., 1989) and a report that specified the beta and gamma energy spectra as functions of time after a detonation (Finn et al., 1979). Those two references were often cited in the individual beta-dose reconstructions before 2000. Although the data used in calculations have changed, the general method has remained substantially the same since routine assessment of skin dose began in 1998. Accordingly, the Barss report can be considered to generally document the method used in 1998 and 1999 and to present the method used since January 2000.
In the Barss (2000) report, external beta dose from standing on a contaminated surface, from being in contaminated air and water, and from contaminated skin is considered in some detail. The report describes models for calculation and, for the case of external beta dose from standing on contaminated ground, makes numerous comparisons with other methods of calculation and with measurement data. Pertinent information from the report is summarized below.
IV.B.4.1 External Beta Dose from Standing on Contaminated Surface
External beta doses from standing on contaminated ground or other surfaces are calculated by applying a beta-to-gamma dose ratio to an estimated upper-bound gamma dose, which is determined from film-badge data or dose reconstruction. Doses to the skin or lens of the eye are then calculated by adding the beta and gamma doses. The fundamentals of the method for estimating external dose to the skin and eye from standing on contaminated ground are summarized as follows:
-
Beta dose to the skin or lens of the eye from external sources is accrued with the gamma dose from radioactive fallout, contamination, or neutron-induced radionuclides. As a result, the beta dose is proportional to the gamma dose, and its relative magnitude can be expressed by a beta-to-gamma dose ratio.
-
The beta-to-gamma dose ratio depends on radionuclide decay and distribution and on geometric relationships between the exposed individual and the radiation source. Gamma and beta energy spectra are interdependent functions of time. Consequently, the beta-to-gamma dose ratio depends on time since detonation.
-
Because of the attenuation characteristics of electrons in matter, beta dose assessments depend more critically than gamma dose assessments on geometry and the shielding material between the radioactive source and the exposed individual. Consequently, the nature of specific job- or task-related activities and their associated protective measures entails special attention and evaluation in determining a beta dose component.
-
Beta doses to skin are evaluated at the anatomic location where a skin cancer has been diagnosed. The depth for evaluation is 0.07 mm, the conventional depth of the basal-cell layer of the skin, which is assumed to be the tissue at risk for skin cancer. Beta doses to the lens of the eye are assessed at a depth of 3 mm below the front surface of the eye, where the tissue at risk for posterior subcapsular cataract development is assumed to be.
-
A beta energy greater than 0.07 MeV is required to penetrate the dead epidermal layer, so beta particles with energies less than that are not included in dose assessments.
-
Skin and eye doses are assessed as the sum of the applicable “high-sided” beta and “high-sided” gamma doses (neutron doses presumably are included if they are significant).
-
Fallout deposited on a surface is considered to be a semi-infinite plane isotropic source, and decontamination activities are considered in evaluating beta doses. Assessments of skin doses are simplified by ignoring attenuation of electrons by large fallout particles that contain volume-distributed activity, particle and photon scattering due to surface roughness, particle and photon attenuation due to penetration into a radioactive surface, and radioactive-source depletion due to weathering, chemical dissociation, or environmental transport (concentra-
-
tion or dispersion). Those simplifications have the effect of making the calculated doses overestimates (“high-siding”).
Barss (2000) provides methods and tables useful for assessing beta dose. They include separate tables of beta-to-gamma dose ratios from exposure to fission products on the ground as a function of time since detonation for NTS and Pacific tests (in the Pacific, one table applies to Operation CASTLE, Shot BRAVO fallout, and a second table applies to all other shots). A separate table of beta-to-gamma dose ratios is provided for activation products in soil. The beta-to-gamma dose ratios are calculated from published beta-particle and gamma-ray spectra. For illustration, the beta-to-gamma dose ratios from the table for Pacific tests are plotted in Figure IV.B.7. The figure illustrates the substantial variation of beta-to-gamma dose ratios with time after detonation and height above ground. The beta-to-gamma dose ratios for the lens of the eye are much smaller because the greater depth of the sensitive tissue (3 mm for lens vs 0.07 mm for skin) leads to much more attenuation.
Activation products are distributed with depth in soil because they originate primarily by interactions with neutrons that penetrate into the soil rather than in deposition from the atmosphere. Thus, beta-to-gamma dose ratios of activation products are small because most of the activation products are deeper in the soil than the range of the emitted beta particles.
Dose to skin is reduced by clothing, and Barss (2000) provides a method for determining the reduction as a function of clothing thickness. Many other factors affect beta dose, such as sizes and shapes of the source materials, position of the body relative to the source (for example, standing, sitting, or kneeling), whether the person is above or below deck on a ship, and whether the person is in an aircraft. Barss provides separate tables to guide adjustment of beta dose when skin is covered by light clothing or denser materials (see Tables 13 and 14 in that report).
The simplest calculation is for a short-term exposure to beta and gamma radiation. The total beta plus gamma dose to the skin or lens of the eye is estimated as
Doseskin/lens = [D(t)γ/ub/fall × Rβ/γ(x,t) × M(x,t)] + D(t)γ/ub/total
where
D(t)γ/ub/fall |
= upper-bound gamma dose due to external exposure to fallout or other beta radiation field, |
Rβ/γ(x,t) |
=β/γ ratio = bare-skin or lens beta-to-gamma dose ratio at distance x and time t, |
M(x,t) |
= combined modifying factor that accounts for differences from the simple case of standing on contaminated ground with bare skin (for example, attenuation by clothing, position of the body, and location above or below decks on |
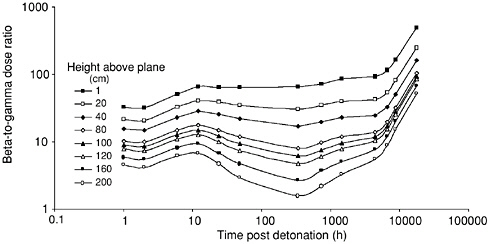
FIGURE IV.B.7 Beta-to-gamma dose ratios for contaminated surfaces used at Pacific tests [see Barss (2000), Table 5].
|
a ship or in an aircraft) [see Barss (2000), Appendix C, Table 12], and |
D(t)γ/ub/total |
= upper-bound gamma dose from all sources. |
If an exposure extends over a period sufficient for any term in the equation to change significantly, the individual beta doses must be summed or integrated to obtain a total beta dose. Alternatively, if M(x,t) is constant, an average value of Rβ/γ(x,t) may be calculated and then multiplied by D(t)γ/ub/fall and M(x,t) to obtain total beta dose. In some cases, Rβ/γ(x,t) may be zero, such as when gamma dose is accrued in uncontaminated interior spaces of a ship.
In an effort to “high-side” the total skin or eye dose, the beta-to-gamma dose ratio is applied to the upper-bound gamma dose from exposure to fallout or other beta-radiation field to calculate beta dose, and the upper-bound gamma dose from all sources is then added to the beta dose.
IV.B.4.2 External Beta Dose from Immersion in Contaminated Air or Water
Immersion in a descending fallout-debris cloud was a less frequent circumstance than exposure to fallout after deposition on the ground or other surface. However, several potential applications for this exposure condition are identified by Barss (2000), such as ground troops advancing toward ground zero at the NTS immediately after a detonation, naval personnel outside on ship weather decks when fallout began, or contaminated air penetrating into the interior of an aircraft during cloud sampling or tracking activities. Some participants on residence islands in the Pacific also were immersed in descending fallout.
Beta-to-gamma dose ratios, such as those used to estimate beta dose from exposure to a contaminated ground surface, are not used in cases of exposure to descending fallout. Instead, beta dose is estimated by using calculated dose coefficients, which give equivalent dose rates from electrons per unit concentration of radionuclides in air,4 combined with the duration of exposure and composite beta-spectrum radiation energies associated with a reconstructed gamma exposure or film-badge reading. The calculated beta dose is added to the upper-bound gamma dose for the corresponding period. The approach is to be tailored case by case for any person exposed to descending fallout. The dose coefficients assume uniform suspension of radioactive material in an infinite air source, and the current method is said to overestimate the corresponding beta dose because it includes no provision for shielding from electrons or for self-attenuation by fallout particles.
Composite energy spectra from mixed fission-product beta particles were calculated as a function of time after a detonation, and the resulting beta energies were binned and combined with calculated beta-particle dose coefficients for air immersion (Kocher and Eckerman, 1981). The resulting composite dose coefficients for the assumed mixtures of radionuclides were calculated as a function of time after the detonation in units of rem y−1 per μCi cm−3 of air. The dose coefficients were tabulated by Barss (2000) for specific periods after detonation, because descending fallout typically lasted anywhere from less than a few hours to 1 day. The composite dose coefficients for assumed mixtures of fission products in descending fallout are illustrated graphically in Figure IV.B.8. Multiplication of the composite beta dose coefficient by the corresponding total activity concentration results in an initial beta dose rate that can be integrated over the time of exposure to give the total beta dose. The resulting beta dose is summed with the upper-bound gamma dose over the same period of time to yield the skin dose due to exposure in air. Clothing modification factors can be applied as appropriate. Barss also describes special methods for the case of being in contaminated air in an aircraft performing cloud sampling or tracking activities and provides an approach for the case of immersion in water. For aircraft, it is appropriate to account for beta-particle backscatter from the interior of the fuselage. The approach for submersion in water is similar to that for air, but it accounts for the different densities of water and air.
IV.B.4.3 Skin Contamination
Barss (2000) correctly indicates that for skin contamination (for example, from fallout or resuspended radioactive soil), the film-badge gamma dose is a
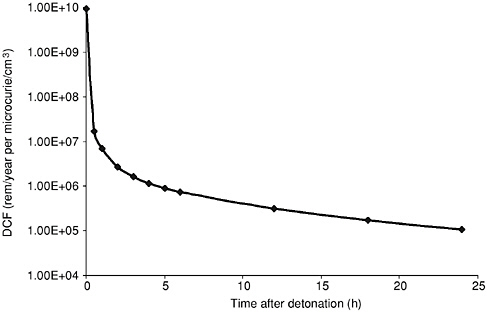
FIGURE IV.B.8 Composite beta dose coefficient for immersion in fallout-contaminated air based on data in Table 16 of Barss (2000).
highly inaccurate indicator of skin dose, so beta-to-gamma ratios are not appropriate for such applications. As in the method used for surface deposition and exposure in air, the beta energy spectrum due to radioactive material on the surface of the skin can be determined as a function of time after detonation, and this allows beta doses to be directly calculated by using dose coefficients from Kocher and Eckerman (1987). The dose coefficients are based on radionuclides deposited on or near the skin surface, and they are nearly constant for average beta energies greater than 0.1 MeV. Hence, it is possible to estimate an average dose coefficient for skin of about 9 rem h−1 (beta plus gamma) per μCi cm−2 of skin, which includes a 30-35% overestimate due to the potential presence of an external backscatter surface (for example, a contaminated surface or tool) and a gamma contribution of about 5%. For contaminated gloves, a dose-reduction factor of 0.5 is assumed.
Barss (2000) recommends that levels of skin contamination be based on measurements when they are available and provides information to guide estimates of skin contamination based on measurements expressed in terms of dose or exposure rate. The report also indicates that the VARSKIN code (Durham, 1992) can be used for additional calculations of skin dose, presumably for source geometries where an assumption of uniform large-area contamination is inappropriate. Barss does not discuss contamination estimates that might be made for troops that are potentially contaminated by resuspended fallout while marching
or performing other work, but there are some early articles on the subject (Schwendiman, 1958; Black, 1962).
IV.C ESTIMATION OF INTERNAL DOSE
IV.C.1 Introduction
This section discusses methods that have been used in the NTPR program to estimate internal doses to atomic veterans. In general, participants at the NTS, in the Pacific, or in Japan could have received internal doses as a result of intakes of radionuclides by inhalation, ingestion, or absorption through the skin or open wounds (see Section I.C.2.2.2). Intakes by inhalation are expected to be the most important for most participants, and the most important exposure scenarios usually involve inhalation of descending fallout or fallout that was deposited on the ground or other surfaces and then resuspended into the air.
Estimation of internal doses to atomic veterans is inherently more difficult than estimation of external doses. External doses usually can be estimated directly on the basis of measurements of external exposure with film badges worn by participants or measurements of external exposure rates at various locations and times in a participant’s exposure environment with field instruments (see Sections IV.B.1 and IV.B.2). In general, however, internal dose cannot be measured directly but must be estimated based on models and other assumptions.
Ideally, estimates of internal dose should be based on relevant monitoring data that were obtained at the time of exposure or shortly thereafter. For example, intakes by inhalation can be monitored on the basis of measured concentrations of radionuclides in air during times of exposure, and intakes by any pathway can be monitored on the basis of measured activities of radionuclides excreted in urine or feces at known times after exposure. Given such data, internal doses can be estimated by using mathematical models that describe the behavior of radionuclides in the human body over time after an intake and the doses delivered to various organs and tissues.
In practice, however, suitable monitoring data to estimate intakes of radionuclides by atomic veterans generally were not obtained. The inhalation hazard posed by plutonium was a concern during the period of atomic testing, especially at the NTS (for example, see Dick and Baker, 1961, and Luna et al., 1969). There also were efforts at some tests to monitor intakes with urinanalysis (NRC, 1985b), and urinanalysis was used to estimate intakes of 131I by participants who received very high doses from exposure to fallout on Rongerik Atoll in the Pacific (Goetz et al., 1987). However, in all dose reconstructions reviewed by the committee, no information was provided on airborne concentrations of radionuclides or contemporaneous measurements of radionuclides in urine or feces that could be used to estimate internal doses. Therefore, indirect methods based on other data are required to estimate intakes of radionuclides.
Section IV.C.2 describes methods and databases that have been used to estimate inhalation doses to atomic veterans. Since the middle 1980s, methods documented by Barrett et al. (1986) and incorporated into the FIIDOS computer code (Egbert et al., 1985) have been used; the committee did not review methods used earlier. The approach to addressing uncertainty in estimates of inhalation dose is described in Section IV.E.4. Section IV.C.3 discusses methods used to estimate ingestion doses to atomic veterans in rare cases in which ingestion is considered, and Section IV.C.4 discusses the possibility of internal doses due to absorption through the skin or open wounds. The committee’s evaluation of methods used in the NTPR program to estimate internal doses to atomic veterans and to account for uncertainty in the estimates is presented in Section V.C. The so-called low-level internal dose screen, which was developed to assess the potential importance of inhalation exposures of participant groups (Barrett et al., 1986), and a bioassay program, which was recently undertaken in an effort to assess internal exposures to plutonium on the basis of urinanalysis, are discussed in Section VI.C and VI.D, respectively.
IV.C.2 Methods of Estimating Inhalation Dose
Inhalation is the only pathway of radionuclide intake normally considered in dose reconstructions for atomic veterans. Four basic scenarios of inhalation exposure have been defined and used in dose reconstructions (Barrett et al., 1986):
-
Inhalation of radioactive material in fallout that was deposited on the ground or other surfaces and then resuspended into the air by mechanical or natural disturbances;
-
Inhalation of neutron-induced radioactive material in soil that was lofted (suspended) into the air by mechanical or natural disturbances;
-
Inhalation of radioactive material in descending fallout;
-
Inhalation of radioactive material in an atmospheric cloud.
In any scenario, the dose from inhalation of a particular radionuclide is estimated by using the following equation in the notation of Barrett et al. (1986):
D = AA × BR × T × DF, (IV.C-1)
where
D |
= equivalent dose to organ or tissue of concern (rem),5 |
AA |
= activity concentration of radionuclide in air (Ci m−3), |
BR |
= breathing rate (m3 h−1), |
5 |
In the NTPR program, the dosimetric quantity calculated in dose reconstructions is called “dose equivalent,” but the term “equivalent dose” is used in this report (see Note on Units and Section I.C.2.3). |
T |
= duration of exposure (h), |
DF |
= equivalent dose to organ or tissue of concern per unit activity intake of the radionuclide inhaled (rem Ci−1).6 |
Equation IV.C-1 gives the dose from inhalation of a single radionuclide. However, any scenario for inhalation exposure of atomic veterans involves intakes of mixtures of radionuclides, and the dose to an organ or tissue of concern is the sum of the doses from intakes of all of them. Thus, composite dose coefficients that apply to assumed mixtures (activity concentrations) of radionuclides are used in all dose reconstructions. Concentrations of radionuclides in air and the associated composite dose coefficients generally are time-dependent because of radioactive decay.
When a veteran does not file a claim for compensation for a specific disease (such as a cancer in a particular organ) but a dose reconstruction is requested, the quantity often calculated and reported to the veteran to represent inhalation dose is the effective dose equivalent, rather than the equivalent dose to a specific organ or tissue. The effective dose equivalent is a weighted average of equivalent doses to several organs and tissues that was developed for use in radiation protection (ICRP, 1977).
A breathing rate of 1.2 m3 h−1 normally is assumed in estimating inhalation doses (Egbert et al., 1985). That value was recommended for use in assessing inhalation doses to average adult workers engaged in light activity (ICRP, 1975), and it represents the breathing rate during walking on a flat surface at about 3 mph (4.8 km h−1) (TGLD, 1966). A higher breathing rate of 1.5 or 2 m3 h−1 may be assumed if veterans engaged in moderate or heavy activity, respectively (Egbert et al., 1985).7
The following sections discuss, first, methods that have been used to estimate concentrations of radionuclides in air in the different scenarios of inhalation exposure listed above and, second, equivalent doses to specific organs or tissues per unit activity intake of inhaled radionuclides (inhalation dose coefficients).
IV.C.2.1 Methods of Estimating Concentrations of Radionuclides in Air
Concentrations of radionuclides in air generally were not measured at locations and times of exposure of atomic veterans. Therefore, indirect methods of estimating them must be used in dose reconstructions. Methods and data used in the NTPR program to estimate airborne concentrations of radionuclides in each
of the four scenarios of inhalation exposure listed above are described in the following sections.
IV.C.2.1.1 Scenario involving inhalation of resuspended fallout
A four-step procedure is used to estimate concentrations of radionuclides in air due to resuspension of fallout particles that were deposited on the ground or other surfaces after a particular nuclear detonation.
First, the relative activities of radionuclides produced in a detonation are estimated on the basis of radiochemical data obtained from sampling of the atmospheric cloud soon after the detonation and calculations of the activities of different fission and activation products that result from an assumed number of fissions in the weapon type of concern. Radionuclides considered in the analysis are fission products, radionuclides produced by neutron activation of plutonium or uranium and other components of the weapon and its support systems, and plutonium or uranium that did not undergo fission or activation. Given the estimated relative activities of radionuclides at the time of detonation, the relative activities at later times, including activities of any radioactive decay products, are calculated on the basis of the half-lives of the radionuclides produced in the detonation and their decay products.
Second, an assumption is made about the relative activity concentrations of radionuclides in fallout deposited on the ground or other surfaces compared with the estimated relative activities in the atmospheric cloud. In all shots, chemical and physical separation of different radionuclides, a process referred to as fractionation and discussed below, is assumed not to occur before deposition, except that noble-gas radionuclides (isotopes of krypton and xenon) in the cloud are removed from fallout. Thus, except for the effects of radioactive decay and the exclusion of noble gases, the relative activity concentrations of radionuclides in fallout are assumed to be the same as the estimated relative activities in the atmospheric cloud.8
Third, the estimated relative activity concentrations of radionuclides in fallout deposited on the ground or other surface are renormalized (scaled) to obtain an estimate of the absolute activity concentrations (Ci m−2). Renormalization is based either on measurements of total photon exposure in air above the surface in roentgens (R) with film badges worn by participants who were present in the fallout field at known times and locations or on measurements of photon exposure rates (R h−1) at various locations and times with field instruments, combined with calculations of the exposure rate in air per unit activity concentration of each
radionuclide on the surface (R h−1 per Ci m−2). That is, estimated relative activity concentrations of radionuclides in deposited fallout obtained in the first two steps are adjusted to give a calculated total exposure or exposure rate above ground that matches measurements obtained by using film badges or field instruments, taking into account radioactive decay and the known times of measurement and exposure. Mathematically, the activity concentration on the surface is (SA/I) × I, where I is the exposure rate and SA/I is the reciprocal of the calculated exposure rate per unit concentration on the surface. Exposure rates per unit concentration of radionuclides on the surface are calculated on the basis of known energies and intensities of photons emitted by the radionuclides assumed to be present in fallout, an assumption that radionuclides are distributed uniformly on a surface of infinite extent, and theoretical considerations of photon transport from the source region on the surface to the assumed height of a film badge or field instrument above the surface and the resulting exposure in air, taking into account the shielding effect of ground roughness.9 An important condition in applying this method is that exposures measured with film badges or field instruments must have been due primarily to deposited fallout.
Fourth, the activity concentrations of radionuclides in air resulting from resuspension of deposited fallout are estimated by using a simple resuspension-factor model given by
AA = SA × K, (IV.C-2)
where AA is the activity concentration of a radionuclide in air (Ci m−3); SA is the activity concentration on the ground or other surface (Ci m−2), as estimated in the first three steps described above; and K is the resuspension factor (m−1). The resuspension factor is assumed to be the same for all radionuclides on a surface, and it is estimated on the basis of relevant experimental studies. Resuspension factors that are used in different exposure scenarios are described later.
Thus, the equivalent dose to an organ or tissue from inhalation of resuspended fallout, D, is represented by:
D = I × (SA/I) × K × BR × T × DF, (IV.C-3)
where
I |
= measured photon exposure rate in fallout field (R h−1), adjusted for radioactive decay between time of measurement and time of exposure; |
SA/I |
= reciprocal of calculated exposure rate per unit activity concentration of radionuclides on the ground or other surface (Ci m−2 per R h−1); |
K |
= resuspension factor (m−1); |
BR |
= breathing rate (m3 h−1); |
T |
= duration of exposure (h); and |
DF |
= inhalation dose coefficient (rem Ci−1). |
As in Equation IV.C-1, calculation of inhalation dose is more complicated than indicated by the representation in Equation IV.C-3 because the quantities SA/I and DF are estimated on the basis of an assumed mixture of radionuclides in fallout and I, SA/I, and DF are time-dependent. The dependence of the photon exposure rate (I) on time usually is assumed to be t−1.2, where t is the time after detonation in hours.
In some dose reconstructions for participants at a particular shot, estimates of inhalation dose due to resuspension of deposited fallout also take into account the presence of fallout that was deposited in the same area after previous shots. Inhalation doses due to resuspension of previously deposited fallout are estimated with the same methods described above, with radioactive decay since the times of the previous shots taken into account. Dose reconstructions for participants on residence islands in the Pacific consider resuspension of all fallout that was present during the time of residence, but only during times when the measured exposure rate exceeded background. At the NTS, exposures of participants in areas where fallout from previous shots occurred are often a concern. Shots at the NTS at which inhalation of resuspended fallout from previous shots has been taken into account in dose reconstructions (Barrett et al., 1986) are listed in Table IV.C.1. In most of these cases, the previous shot occurred relatively recently in the same test series. Fallout that occurred after shots in previous test series usually is not taken into account, because it is assumed that the resuspension factor would have diminished by at least a factor of 10 through weathering, including penetration into surface soil and attachment to large soil particles (Barrett et al., 1986).
To recapitulate, airborne concentrations of radionuclides due to resuspension of previously deposited fallout are estimated in the NTPR program in the following way. Estimates of relative activities of radionuclides produced in a nuclear detonation combined with an assumption of no fractionation in fallout, except for removal of noble gases, are used to estimate relative activities of radionuclides in fallout deposited on the ground or other surfaces. Activity concentrations of radionuclides in deposited fallout (Ci m−2) are then estimated by using measured photon exposures or exposure rates at the locations of fallout and calculations of the exposure rate per unit activity concentration of each radionuclide on the surface, which is assumed to be uniformly contaminated and infinite in extent. Finally, activity concentrations of radionuclides in air (Ci m−3) due to resuspension are estimated by using the estimated concentrations in deposited fallout
TABLE IV.C.1 Shots at Nevada Test Site Where Inhalation of Resuspended Fallout from Previous Shots Is Taken into Account in Dose Reconstructionsa
Operation |
Shot of concern in dose reconstruction (date) |
Previous shot accounted for in dose reconstruction (date) |
BUSTER-JANGLE |
UNCLE (11/29/51) |
SUGAR (11/19/51) |
TUMBLER-SNAPPER |
FOX (05/25/52) |
EASY (05/07/52) |
UPSHOT-KNOTHOLE |
RAY (04/11/53) |
NANCY (03/24/53) |
|
BADGER (04/18/53) |
NANCY (03/24/53) |
HARRY (05/19/53) |
BADGER (04/18/53), SIMON (04/25/53) |
|
CLIMAX (06/04/53) |
BADGER (04/18/53) |
|
TEAPOT |
HORNET (03/12/55) |
MOTH (02/22/55) |
|
APPLE I (03/29/55) |
TURK (03/07/55) |
POST (04/09/55) |
TESLA (03/01/55) |
|
PLUMBBOB |
LASSEN (06/05/57) |
BOLTZMANN (05/28/57) |
|
WILSON (06/18/57) |
BOLTZMANN (05/28/57) |
OWENS (07/25/57) |
BOLTZMANN (05/28/57) |
|
SMOKY (08/31/57) |
BOLTZMANN (05/28/57), DIABLO (07/15/57), SHASTA (08/18/57) |
|
WHEELER (09/06/57) |
BOLTZMANN (05/28/57), SMOKY (08/31/57) |
|
CHARLESTON (09/28/57) |
BOLTZMANN (05/28/57), SMOKY (08/31/57) |
|
MORGAN (10/07/57) |
SMOKY (08/31/57)b |
|
aSee Section 2.2 of Barrett et al. (1986). In addition, impact of fallout from TEAPOT Shot TESLA at location of PLUMBBOB Shots LASSEN, WILSON, HOOD, OWENS, WHEELER, CHARLESTON, and MORGAN is taken into account, except resuspension factor applied to TESLA fallout 2 years later is factor of 10 lower than value applied in other cases. bImpact of fallout from PLUMBBOB Shot BOLTZMANN on location of Shot MORGAN—which was at same ground zero as earlier PLUMBBOB Shots LASSEN, WILSON, OWENS, WHEELER, and CHARLESTON—apparently was considered to be insignificant. |
and an assumed resuspension factor. Radioactive decay is taken into account from the time of detonation to the time exposure is assumed to occur. That method is applied in all cases of inhalation of resuspended fallout that was deposited on the ground surface, on exterior or interior surfaces of ships, or on surfaces of airplanes used in cloud sampling.
Further discussion that focuses on the intention of the NTPR program that the method should result in overestimates of airborne concentrations of radionuclides in resuspended fallout is presented in Section IV.C.2.1.7.
IV.C.2.1.2 Fractionation of radionuclides
The term fractionation refers to the chemical and physical separation of radionuclides produced in a detonation. As noted in the previous section, an
assumption about fractionation is needed to estimate concentrations of radionuclides in fallout deposited on the ground or other surfaces on the basis of estimated relative activities of radionuclides in the atmosphere after a detonation. The following description of fractionation and its effect on the radionuclide composition of fallout is based mainly on a discussion by Hicks (1982).
Chemical separation of radionuclides in the atmosphere occurs in the first few minutes after a detonation as a result of differences in the boiling points of the various elements that make up the radioactive materials. Initially, all materials in the fireball are vaporized, because of the extreme temperatures. As the fireball cools to about 3000°C, iron oxides and soil materials that make up most of the mass in the fireball form liquid droplets in which so-called refractory elements are dissolved. Refractory elements have relatively high boiling points, comparable to or greater than the melting points of materials that form the liquid droplets; examples are barium, cerium, uranium, and plutonium. As the liquid droplets continue to cool, they solidify at about 1500°C, and over the next few minutes, as the solid particles cool to the ambient temperature of about 50°C, volatile elements that have boiling points lower than the melting points of the particles condense on particle surfaces. Examples of volatile elements are strontium, iodine, and cesium. As a result of the chemical separation of radionuclides between those which are dispersed mainly throughout the volume of solid particles (refractory elements) and those which mainly condense on the surface (volatile elements), and taking into account that the surface area-to-volume ratio of a particle is about 1/r (where r is the radius), smaller particles contain a relatively high proportion of volatile radionuclides, compared with the initial composition of the atmospheric cloud, and larger particles contain a relatively high proportion of refractory radionuclides.
Physical separation of radionuclides then occurs as a result of differences in the fall velocities of small and large particles in the atmosphere. Larger particles fall to Earth more rapidly than small particles, and this results in a physical separation by particle size along the path of travel of the atmospheric cloud. Thus, fallout close to ground zero consists mostly of relatively large particles that contain a higher proportion of refractory radionuclides, whereas fallout at more distant locations consists mostly of relatively small particles that contain a higher proportion of volatile radionuclides.
Another factor that contributes to fractionation of radionuclides in fallout is the presence of the noble gases krypton and xenon among the fission products. Separation of noble gases from other materials begins as soon as liquid droplets form and continues as the droplets solidify and begin to fall to Earth. As noted previously, this type of fractionation is taken into account by the NTPR program in estimating concentrations of radionuclides in fallout deposited on the ground or other surfaces at the NTS and in the Pacific. In addition, differences in half-lives of noble-gas radionuclides can result in fractionation of chemically similar elements. A case in point involves the important fission products 90Sr and 137Cs,
both of which are volatile. Those radionuclides are produced mainly by decay of shorter-lived fission products in the mass 90 and 137 decay chains, which include noble-gas radionuclides with substantially different half-lives (90Kr with a half-life of 32 s, and 137Xe with a half-life of 3.84 min). As a result, condensation of 90Sr and 137Cs on surfaces of solid particles tends to occur at different times, and fractionation occurs as particles containing 90Sr are physically separated from 137Xe before 137Cs is produced in the atmospheric cloud. That effect often results in a severe depletion of 137Cs, compared with the amounts of 90Sr and other volatile radionuclides, in fallout close to ground zero. Fractionation of 90Sr and 137Cs also can be important in detonations just below the ground surface.10 In that type of shot, longer-lived 137Xe can vent to the atmosphere to a much greater extent than 90Kr, and this results in increased 137Cs in fallout near ground zero compared with 90Sr, in contrast to the depletion of 137Cs relative to 90Sr that often occurs in aboveground shots.
As noted previously, in estimating inhalation doses to atomic veterans, the NTPR program generally assumes no fractionation of radionuclides in fallout except for removal of noble gases. On the basis of the foregoing discussions, that assumption normally should result in overestimates of the concentrations of volatile radionuclides deposited on the ground or other surfaces but underestimates of the concentrations of refractory radionuclides, because participants at the NTS and in the Pacific usually were exposed at locations where fallout was dominated by larger particles that contained a high proportion of refractory radionuclides. The method also does not account for fractionation of the volatile radionuclides 90Sr and 137Cs, which is due to the difference in half-lives of their noble-gas precursors.
IV.C.2.1.3 Resuspension of radionuclides in deposited fallout
The resuspension factor that should be applied to fallout deposited on the ground or other surfaces is recognized to be a highly uncertain parameter that depends on the nature of the activity causing the resuspension (Egbert et al., 1985; Barrett et al., 1986). On the basis of a review of available data and the use of subjective judgment, different resuspension factors are used in the NTPR program to estimate airborne concentrations of radionuclides in various exposure scenarios. Resuspension factors commonly used in dose reconstructions at the NTS (Barrett et al., 1986) and on ships in the Pacific (Phillips et al., 1985) are summarized in Tables IV.C.2 and IV.C.3, respectively. A resuspension factor of 10−5 m−1 often is used in estimating inhalation exposures on residence islands in the Pacific, with the additional assumption that inhalation of resuspended fallout occurs only during the fraction of the time spent outdoors but not during time
spent indoors. In cases of exposure in an airplane whose interior was contaminated during cloud sampling, a resuspension factor of 10−4 m−1 is used.
Resuspension factors different from those summarized in Tables IV.C.2 and IV.C.3 and described above have been used in some cases. At Operation UPSHOT-KNOTHOLE, Shot GRABLE, for example, a higher resuspension factor of 10−2 m−1 was assumed in dose reconstructions for participant groups that encountered a severe dust storm in an area where neutron activation of soil occurred (Goetz et al., 1981; Edwards et al., 1985). But the resuspension factor often is reduced by a factor of 10, compared with the standard assumption of 10−5 m−1 used in many dose reconstructions, or to zero in cases of exposure to aged fallout that was deposited well before the time of exposure; this is especially the case on residence islands in the Pacific.
TABLE IV.C.2 Resuspension Factors Normally Assumed for Various Activities of Atomic Veterans in Contaminated Areas at NTSa
TABLE IV.C.3 Resuspension Factors Normally Assumed for Various Activities of Participants on Contaminated Ships in Pacifica
Thus, in general, judgment has been applied by the NTPR program in estimating resuspension factors used in dose reconstructions for particular exposure scenarios that involve previously deposited fallout. As discussed further in Section IV.C.2.1.7, the intent of the NTPR program has been to select resuspension factors that result in overestimates of airborne concentrations of radionuclides in resuspended fallout to which participants at the NTS and in the Pacific were exposed.
IV.C.2.1.4 Scenario involving inhalation of suspended neutron activation products in soil
The method of estimating airborne concentrations of radionuclides due to suspension of radioactive material that was produced by neutron activation in soil, which occurred only at the NTS, is essentially the same as the method used in the scenario involving resuspension of deposited fallout described above (Barrett et al., 1986). The method is summarized as follows.
Relative activities of activation products in soil are estimated on the basis of field measurements of known activation products, known elemental compositions of soil, calculations of neutron transport in air and soil, and calculations of neutron capture in nuclei of stable elements in soil. Activities of radionuclides per unit volume of surface soil (Ci m−3) then are estimated by renormalization (scaling) of the estimated relative activities to give a calculated photon exposure in air above ground or exposure rate at a particular time that matches available measurements with film badges or field instruments, taking radioactive decay into account. Finally, a resuspension factor is applied to the estimated concentrations of radionuclides in surface soil to obtain an estimate of the concentrations in
air. Radionuclide activities per unit volume of surface soil are converted to equivalent surface concentrations (Ci m−2) by assuming that the top 1 cm of soil can be suspended in the air. As in the scenario involving resuspension of deposited fallout, an important condition in applying this method is that the film-badge or instrument readings must be due primarily to activation products in soil.
Resuspension factors that are applied to activation products in surface soil at the NTS are the same as those that are applied to deposited fallout (see Table IV.C.2). That is, a resuspension factor of 10−5 m−1 often is assumed, and higher values are assumed when suspension is caused by an unusually vigorous disturbance of surface soil. Again, the intent of the NTPR program has been to select resuspension factors that result in overestimates of airborne concentrations of activation products in soil to which participants at the NTS were exposed.
IV.C.2.1.5 Scenario involving inhalation of descending fallout
Airborne concentrations of radionuclides in descending fallout are estimated on the basis of estimates of concentrations on the ground or other surfaces that resulted from deposition of the fallout. The basic concept underlying the method used in the NTPR program is that activities of radionuclides per unit area on a surface resulted from deposition of the activities per unit volume in air averaged over the height of the atmospheric cloud produced by the detonation.
With reference to Equations IV.C-1 and IV.C-3, the concentration of a radionuclide in air (AA) can be represented as I × (SA/I) × (AA/SA), where I is the maximum photon exposure rate in air above the ground or other surface due to the deposited fallout at the time after detonation, t (in hours), when fallout is complete, and SA/I is the reciprocal of the calculated exposure rate per unit activity concentration on the surface (SA) at time t. Again, SA/I is based on an assumption that the surface is uniformly contaminated and infinite in extent, and the calculation accounts for the shielding effect of ground roughness. Because the airborne concentration (AA) persists for a time T during which fallout is assumed to descend with a velocity V at the location of concern, the activity concentration on the surface at the time fallout ends can be expressed as SA = AA × V × T.11 Thus, if exposure is assumed to occur throughout the duration of descending fallout, Equation IV.C-1 becomes
D = I × (SA/I) × (1/V) × BR × DF, (IV.C-4)
where the duration of exposure, T, which corresponds to the duration of descending fallout at the location of concern, is eliminated.
The deposition velocity, V, in Equation IV.C-4 is based on an assumption that fallout particles descended from a height of around 10 km (104 m). Thus, V ~ (104 m)/t, where t again is the time (in hours) after a detonation when deposition of fallout is complete. The dose due to inhalation of descending fallout then is estimated as
D = I × (SA/I) × (10−4 m−1) × t × BR × DF. (IV.C-5)
Equation IV.C-5 is similar to Equation IV.C-3, which is used to estimate the dose from inhalation of resuspended fallout. The differences are that the term (10−4 m−1) is an “effective” resuspension factor, rather than an actual value, and t is the time (in hours) after a detonation when fallout deposition is complete and the maximum exposure rate occurs, rather than the duration of exposure. Again, the quantities I, SA/I, and DF are time-dependent, and SA/I and DF are calculated based on an assumed mixture of radionuclides in fallout.
Thus, activities of radionuclides per unit volume of air in descending fallout are estimated on the basis of estimated concentrations on the ground or other surface that resulted from fallout, given by I × (SA/I), and an effective resuspension factor given by the reciprocal of the assumed height of the atmospheric cloud. In essence, resuspension is deposition reversed in time, and if all deposited material is assumed to be resuspended, activity concentrations in air are given by the concentrations on the surface divided by the assumed height of the resuspended plume. This roundabout method is necessitated by the lack of data on concentrations of radionuclides in descending fallout.
In this scenario, concentrations of radionuclides deposited on the ground generally are based on measurements of external photon exposure rates at particular locations and times with field instruments rather than measurements of total external exposure with film badges worn by participants who were exposed to descending fallout (Barrett et al., 1986). The use of film-badge readings is inappropriate in this scenario because exposures generally continued for considerable periods of time after deposition of the fallout ceased, and the readings would lead to unreasonable overestimates of airborne concentrations of radionuclides in descending fallout. An important condition in applying the method is that exposure rates measured with field instruments must have been due primarily to deposited, rather than descending, fallout. Measurements of maximum exposure rates at times that descent of fallout is completed are the most appropriate.
In many dose reconstructions, it is assumed that intakes of fully inhalable fallout particles occurred only at times, t, later than 10 h after a detonation. At such times, calculations based on assumed mixtures of radionuclides in fallout indicate that the quantity SA/I is roughly constant, with a value of 0.16 Ci m−2 per R h−1. If the usual breathing rate of 1.2 m3 h−1 is assumed, the dose due to inhalation of descending fallout is calculated in these cases as
D = (2.0 × 10−5) × I × t × DF. (IV.C-6)
In contrast to the methods of estimating airborne concentrations of radionuclides in resuspended fallout or suspended activation products in soil described previously, the assumption of an effective resuspension factor of 10−4 m−1 in this scenario is not intended to result in overestimates of airborne concentrations of radionuclides in descending fallout. Rather, the NTPR program assumes that the presumption of exposure throughout the entire period of deposition and use of the maximum photon exposure rate at the time when fallout is complete will result in overestimates of airborne concentrations (Barrett et al., 1986).
IV.C.2.1.6 Scenario involving inhalation in an atmospheric cloud
The scenario involving inhalation of radionuclides in an atmospheric cloud is applied only for participants who flew through a cloud in an airplane or helicopter. In this scenario, the general expression for inhalation dose given in Equation IV.C-1 is written as
D = I × (AA/I) × BR × T × DF, (IV.C-7)
where AA/I is the reciprocal of the calculated exposure rate per unit activity concentration of a radionuclide in air. Values of AA/I generally are different from calculated values of SA/I used to estimate inhalation doses from resuspended or descending fallout, and they are calculated based on an assumption that the atmospheric cloud is uniformly contaminated and infinite in extent. An effective resuspension factor is not used in this scenario, because the quantity I is a measured photon exposure rate due to airborne radionuclides rather than radionuclides deposited on a surface. Again, the quantities I, AA/I, and DF are time-dependent, and AA/I and DF are based on an assumed mixture of radionuclides in air. The exposure rate, I, generally can be based on readings of film badges.
Calculations based on assumed mixtures of radionuclides in an atmospheric cloud indicate that the quantity AA/I is only weakly time-dependent and is inversely proportional to the average energy of emitted photons, E. Thus, in all dose reconstructions, AA/I is assumed to be given by (5 × 10−4)/E, where E is in MeV per disintegration. If a breathing rate of 1.2 m3 h−1 is again assumed, the dose due to inhalation in an atmospheric cloud is calculated in all cases as
D = I × (6 × 10−4) × T × DF/E. (IV.C-8)
When exposure of a person to an atmospheric cloud while the person was in an aircraft was inadvertent, inhalation doses generally are estimated based on concentrations of radionuclides in air obtained as described above and an assumption that no respiratory protection was used. However, in cases of planned exposure, such as occurred during cloud sampling, it generally is assumed that respiratory protection was used and furthermore that the equipment provided complete protection. Thus, the inhalation dose in such cases is assumed to be zero.
The method of estimating concentrations of radionuclides in an atmospheric cloud described above does not involve assumptions that intentionally result in overestimates of inhalation exposure. However, the NTPR program believes that use of film-badge readings in the method tends to result in some overestimation of inhalation exposure; the reason is that aircraft presumably would have avoided regions of highest activity concentrations in air, but these regions nonetheless contribute to external exposures measured in regions of lower activity concentrations where inhalation exposure occurred (Barrett et al., 1986).
IV.C.2.1.7 Summary of methods of estimating concentrations of radionuclides in air
In the various scenarios for inhalation exposure of atomic veterans, estimates of concentrations of radionuclides in air are based on the following data and assumptions:
-
Data on relative activities of different radionuclides produced in a particular nuclear detonation (all scenarios).
-
An assumption of no fractionation of radionuclides, except for removal of noble gases in fallout (all scenarios).
-
Data on external photon exposures or exposure rates due to radionuclides on the ground or other surfaces or in the air, as obtained from film-badge readings or field instruments, and calculations of exposure rates per unit concentration of radionuclides on a surface or in the air (all scenarios).
-
Assumptions about the amounts of radionuclides in fallout deposited on the ground or other surfaces or amounts of activation products in soil that were resuspended or suspended in the air, with resuspension also used to mimic deposition in the scenario involving inhalation of descending fallout (all scenarios except inhalation in an atmospheric cloud).
Thus, the data that underlie all calculations of inhalation dose are estimates of the amounts of different radionuclides in an atmospheric cloud or in soil that were produced in a detonation and measured external photon exposures or exposure rates due to radionuclides deposited on the ground or other surfaces (fallout), distributed over a depth of surface soil (neutron-activation products), or distributed in the cloud. Especially in scenarios involving inhalation of resuspended or descending fallout, a key assumption in the methods used to calculate inhalation dose is that there is no fractionation of radionuclides other than removal of noble gases; these two scenarios are the most important in inhalation exposures of many atomic veterans.
As emphasized at the beginning of Chapter IV, the goal of the NTPR program is to obtain estimates of dose to atomic veterans that are upper bounds (at least 95% confidence limits) of possible doses; that is, the estimated doses are intended to be “high-sided.” In attempting to meet that goal when estimating
inhalation doses, the NTPR program relies to a large extent on an assumption that resuspension factors used in some scenarios result in substantial overestimates of airborne concentrations of radionuclides to which most participants were exposed. That is, data on relative activities of radionuclides produced in a particular detonation, the assumption of no fractionation of radionuclides except for removal of noble gases, data on external exposures or exposure rates obtained with film badges or field instruments, calculations of exposure rates per unit concentration of radionuclides on a surface or in the air, and assumed breathing rates used in dose reconstructions usually are not intentionally biased in an effort to yield overestimates of inhalation exposure. But assumptions about resuspension often are intended to be “high-sided” to an extent sufficient to compensate for possible errors and uncertainties in all other factors that enter into a calculation of inhalation dose, including the inhalation dose coefficients discussed in the following section.
IV.C.2.2 Dose Coefficients for Inhalation of Radionuclides
Inhalation dose coefficients (equivalent doses to specific organs or tissues per unit activity of radionuclides inhaled) used in all dose reconstructions are 50-year committed doses. That is, they represent the total dose received over a period of 50 years after an acute intake. The use of committed doses takes into account that an acute intake of a radionuclide may result in a dose that is received over many years after intake, and the 50-year period for calculating committed doses represents an average life expectancy of a young adult. For some radionuclides, the entire 50-year committed dose is received within a short time after an intake. For example, the committed dose due to intakes of 131I, with a half-life of 8 days, is received within a few months. However, in cases of intakes of long-lived radionuclides that are tenaciously retained in the body, such as plutonium, the dose to an organ or tissue of concern can be protracted throughout the rest of life, with only a small fraction of the committed dose received in each year. In all dose reconstructions, 50-year committed equivalent doses are assigned to the year in which exposure occurred regardless of the dependence of the total dose received on time after an intake (the time dependence of the dose rate).
All inhalation dose coefficients used in dose reconstructions are calculated with two kinds of models:
-
Biokinetic models that describe the deposition, retention, translocation, and absorption of inhaled radionuclides in the respiratory tract or ingested radionuclides in the gastrointestinal (GI) tract and the transfer, deposition, and retention of absorbed radionuclides in different organs and tissues of the body.
-
Dosimetric models that are used to calculate equivalent doses to different organs or tissues (the target organs) per decay of a radionuclide in each source organ (site of deposition or transit through the body).
Inhalation dose coefficients used in all dose reconstructions were calculated based essentially on dosimetric and biokinetic models described in ICRP Publication 30 (ICRP, 1979a; 1979b; 1980a; 1981a; 1981b; 1982a; 1982b). In some dose reconstructions, inhalation dose coefficients are those given in the ICRP Publication 30 reports; these dose coefficients also are given by Eckerman et al. (1988). In many dose reconstructions, however, inhalation dose coefficients were obtained from Oak Ridge National Laboratory (ORNL) reports (Killough et al., 1978a; Dunning et al., 1979). The models used to calculate inhalation dose coefficients in the ORNL reports (Killough et al., 1978b) are in many respects the same as the models described in ICRP Publication 30. In particular, the model of the behavior of inhaled radionuclides in the respiratory tract is the same.
However, there are differences between the two models. For example, the behavior of radioactive decay products in the body is treated differently. In the ORNL models (Killough et al., 1978b), radioactive decay products that are produced in the body after intakes of a parent radionuclide are assumed to behave according to the biokinetic model for the decay product, whereas in the ICRP models (ICRP, 1979a), decay products that are produced in the body are assumed to behave according to the biokinetic model for the parent. Also, for some radionuclides, there are differences in parameter values used in the biokinetic models. For example, the fraction of ingested plutonium absorbed in the GI tract used in the ORNL calculations (Dunning et al., 1979) is a factor of 3 higher than the value assumed by ICRP (1979a). The same difference occurs in dose coefficients for organs or tissues other than those in the GI tract when plutonium is directly ingested or inhaled plutonium is swallowed.
The committee is not aware of any written policy in the NTPR program to assist an analyst in choosing between ICRP and ORNL dose coefficients for use in dose reconstructions for atomic veterans; both sets of data are included in the FIIDOS computer code (Egbert et al., 1985) and are readily available. Nor did the committee find explanations for choosing one data set over the other in dose reconstructions for individuals or participant groups.
In selecting inhalation dose coefficients from the ORNL or ICRP reports, several assumptions about conditions of exposure and other factors are required, including the size of inhaled particles, chemical form of inhaled radionuclides, and biological effectiveness of alpha particles relative to that of photons and electrons. Assumptions used in dose reconstructions for atomic veterans are described below.
IV.C.2.2.1 Size of inhaled particles
Inhalation dose coefficients used in dose reconstructions for atomic veterans assume that inhaled radionuclides are attached to particles. The size of inhaled particles is specified by a quantity called the activity median aerodynamic diameter (AMAD). In any inhaled materials, there is a distribution of particle sizes
(usually assumed to be lognormal), and AMAD is defined as the diameter in an aerodynamic particle-size distribution for which the total activities attached to particles above and below this size are equal (NCRP, 1997). The particle size (AMAD) used in calculating inhalation dose is taken to be the diameter of a sphere of unit density that has the same terminal settling velocity in air as that of the particle whose activity is the median for the distribution of particle sizes (ICRP, 1979a; NCRP, 1997).
In many dose reconstructions, a particle size (AMAD) of 1 μm is assumed, on the basis of a standard assumption recommended for use in radiation protection in ICRP Publication 30 (ICRP, 1979a). In some dose reconstructions, however, inhalation dose coefficients for large, essentially nonrespirable particles based on an assumed AMAD of 20 μm are used, especially when this choice results in higher doses to the organ or tissue in which a cancer in an atomic veteran occurred. Particle size affects inhalation dose coefficients in the following way.
The pattern of deposition of inhaled particles in different regions of the respiratory tract depends on particle size. For an AMAD of 1 μm, the lung model developed by ICRP (1979a) assumes that 30% of the inhaled activity is deposited in the nose and throat, 8% in the trachea and bronchial tree, and 25% in the pulmonary lung; the remainder is assumed to be exhaled without deposition in the respiratory tract. For an AMAD of 20 μm, however, nearly all the inhaled activity is assumed to be deposited in the nose and throat, with little activity deposited in other regions of the respiratory tract or exhaled (ICRP, 1979a). Furthermore, when radionuclides are deposited in the nose and throat, rates of absorption into blood or transfer to the GI tract by swallowing are assumed to be substantially higher than when deposition in the pulmonary region occurs (ICRP, 1979a). Thus, for example, the dose to the thyroid from inhalation of 131I attached to 20-μm particles is higher than when an AMAD of 1 μm is assumed because, first, a larger fraction of the inhaled material is assumed to be deposited in the respiratory tract and then absorbed into blood from the nose and throat or GI tract and, second, a substantial fraction of the smaller particles is deposited in the pulmonary lung, where the activity is reduced by decay before absorption into blood occurs; the effect of the larger deposition fraction is the more important. Similarly, the dose to organs of the GI tract is higher when an AMAD of 20 μm is assumed, because a larger fraction of the inhaled activity is assumed to be transferred to the GI tract by swallowing (ICRP, 1979a). When radionuclides attached to large particles are moderately or highly insoluble, for example, nearly all the activity is assumed to be transferred from the nose and throat to the GI tract, but the fraction of such transfers is substantially lower when an AMAD of 1 μm is assumed.
On the basis of considerations of the dependence of inhalation doses on particle size, an AMAD of 20 μm is assumed in some dose reconstructions, especially when the organ or tissue of concern is the thyroid—in which case the
dose is due mainly to intakes of 131I—or one of the organs of the GI tract. That assumption may be used in estimating doses due to inhalation of descending or resuspended fallout, based on the consideration that fallout to which participants were exposed consisted mainly of large particles. However, inhalation dose coefficients for large particles are not used when the lung is the organ of concern, even when inhalation of large particles may be more important, because an assumed AMAD of 1 μm always results in a higher estimate of dose to the lung, due to the greater deposition of smaller particles in this part of the respiratory tract. In addition, an AMAD of 1 μm normally is used in estimating inhalation doses due to suspension of activation products in soil, regardless of the organ or tissue of concern, on the basis of an assumption that resuspended soil particles were relatively small. The lower particle size is sometimes used in cases of resuspension of deposited fallout, especially when fallout was deposited well before the time of exposure, on the basis of an assumption that large particles in fallout were reduced in size by weathering.
Thus, with the exceptions noted above, the standard practice in the NTPR program is to choose the particle size that gives the higher estimate of inhalation dose to the organ or tissue of concern (Schaeffer, 2002b), and dose coefficients for particle sizes (AMAD) of 1 and 20 μm are included in the FIIDOS code (Egbert et al., 1985) for this purpose.12
IV.C.2.2.2 Chemical form of inhaled radionuclides
Once inhaled radionuclides are deposited in different regions of the respiratory tract, rates of transfer to blood or to the GI tract by swallowing depend on the assumed chemical form of the radionuclides. Different chemical forms have different solubilities, and materials that have a higher solubility are transferred more rapidly than materials that have a lower solubility. In the lung model used in the NTPR program (ICRP, 1979a), three solubility (lung clearance) classes of inhaled radionuclides in particulate form are defined: highly soluble materials that are assumed to be cleared from the respiratory tract in a matter of days (Class D), moderately insoluble materials that are cleared in a matter of weeks (Class W), and insoluble materials that are cleared in a matter of years (Class Y).13
Inhalation of soluble Class D materials usually results in relatively low doses to tissues of the respiratory tract and relatively high doses to other organs and tissues. As the solubility decreases, doses to tissues of the respiratory tract increase and doses to other organs and tissues decrease, although there are some exceptions in organs of the GI tract.
Inhalation dose coefficients that apply to radionuclides in oxide form are used in all dose reconstructions (Egbert et al., 1985), on the basis of the expected insolubility of radioactive materials in fallout and the insolubility of oxide forms of many radionuclides compared with other chemical forms. That assumption usually results in the highest estimates of dose to the lung and organs in the GI tract, but in these cases, it often results in lower estimates of dose to other organs and tissues compared with doses based on an assumption of a more soluble chemical form.14 However, there are exceptions, including isotopes of strontium for which the oxide form is assumed to be Class D rather than Class Y (ICRP, 1979a). For some elements, including iodine, the same clearance class is assumed for all chemical forms.
IV.C.2.2.3 Biological effectiveness of alpha particles
On the basis of numerous studies in animals, alpha particles are known to be more effective than photons and electrons in inducing biological responses (NCRP, 1990). That is, if the same absorbed dose of alpha particles and of photons or electrons is delivered to an organ or tissue, the probability that a cancer or other stochastic radiation effect will result is substantially higher in the case of exposure to alpha particles. The biological effectiveness of alpha particles is an important concern, for example, in estimating equivalent doses due to inhalation of plutonium, which is an alpha-emitter.
Two sets of dose coefficients for inhalation (and ingestion) of alpha-emitting radionuclides were calculated by the ORNL group (Dunning et al., 1979); one set assumed a biological effectiveness of 10 for alpha particles compared with photons and electrons, and the other assumed a biological effectiveness of 20. However, only the dose coefficients calculated by the ORNL group that incorporate the higher biological effectiveness of alpha particles (20), thus giving higher equivalent doses, are included in the FIIDOS code (Egbert et al., 1985) and have been used in dose reconstructions since then. This assumption conforms to the current recommendation by ICRP (1991a), and is incorporated in all dose coefficients used in dose reconstructions that are obtained from ICRP Publication 30 (ICRP, 1979a).
IV.C.2.2.4 Summary of inhalation dose coefficients used in dose reconstructions
All dose coefficients for inhalation of radionuclides used in dose reconstructions for atomic veterans are based essentially on dosimetric and biokinetic models developed in ICRP Publication 30 (ICRP, 1979a) and are given in documentation of the FIIDOS computer code (Egbert et al., 1985). The dose coefficients assume that inhaled radionuclides are in oxide form and, in all cases of inhalation of alpha-emitting radionuclides, assume a biological effectiveness of alpha particles of 20 relative to photons and electrons.
In estimating inhalation doses to atomic veterans, an analyst must choose between dose coefficients calculated by the ORNL group (Killough et al., 1978a; Dunning et al., 1979) and values calculated by ICRP (1979a; 1979b; 1980a; 1981a; 1981b; 1982a; 1982b). There are some differences in inhalation dose coefficients in the two data sets, but the committee is not aware of any written policy in the NTPR program to guide the choice. An analyst also must choose inhalation dose coefficients based on an assumed particle size (AMAD) of 1 or 20 μm. With some exceptions that are based on reasoned arguments, the stated policy is that the particle size that gives the higher estimate of dose to the organ or tissue of concern will be used (Schaeffer, 2002b).
Inhalation dose coefficients used by the NTPR program are not intended to overestimate doses that would result from given intakes of radionuclides of known particle size and solubility. In using these dose coefficients, only the assumed particle size could result in substantial overestimates of inhalation dose to particular organs or tissues. Thus, as emphasized in Section IV.C.2.1.7, the NTPR program, in its effort to obtain “high-sided” estimates of inhalation dose, relies primarily on assumptions that are intended to result in overestimates of airborne concentrations of radionuclides to which participants were exposed, especially assumptions about resuspension factors that are applied to deposited fallout or neutron-activation products in surface soil.
IV.C.3 Methods of Estimating Ingestion Dose
When ingestion of radionuclides is assumed to occur, such as by consumption of food or water contaminated by fallout, equivalent dose to an organ or tissue of concern is calculated by using an estimated activity of each radionuclide ingested and the appropriate ingestion dose coefficient. Dose coefficients are obtained either from ORNL reports (Killough et al., 1978a; Dunning et al., 1979) or from ICRP Publication 30 (ICRP, 1979a; 1979b; 1980a; 1981a; 1981b; 1982a; 1982b). The latter set of dose coefficients also is given by Eckerman et al. (1988).
Ingestion of radioactive materials apparently is considered only rarely in dose reconstructions for atomic veterans. The one case encountered by the committee concerned a small group of participants on Rongerik Atoll in the Marshall Islands who were exposed to very high levels of fallout after Operation CASTLE,
Shot BRAVO (Goetz et al., 1987). In the dose reconstruction for that group, ingestion of radionuclides that had deposited on food eaten during the period of fallout was considered to be the most important intake pathway.15
It also should be noted that ingestion dose coefficients are used in estimating doses due to inhalation of large particles (AMAD, 20 μm). When inhalation of large particles is assumed, ingestion dose coefficients are applied to the large fraction of inhaled activity that is assumed to be transferred from the nose and throat to the GI tract by swallowing.
IV.C.4 Absorption Through the Skin or Open Wound
Intakes of radionuclides due to absorption through the skin or an open wound are rarely considered in any dose assessment. The one exception is in cases of exposure to an atmospheric cloud of 3H in the form of tritiated water vapor, when intakes by skin absorption usually are assumed to be half the estimated intakes by inhalation (ICRP, 1979a).
Documentation of the FIIDOS computer code (Egbert et al., 1985) does not include consideration of intakes of radionuclides by absorption through the skin or an open wound. Therefore, the NTPR program presumably has not considered those intake pathways in dose reconstructions for atomic veterans. Absorption through intact skin is unlikely to be important, but absorption through an open wound or cut could have occurred when a veteran had such a condition and radioactive materials were deposited on the affected part of the body surface. That situation could occur, for example, while a person was digging a trench or crawling in a contaminated area.16
IV.D DOSE RECONSTRUCTIONS FOR OCCUPATION FORCES IN JAPAN
After Japan surrendered on September 2, 1945, US military forces occupied Hiroshima and Nagasaki and therefore may have been exposed to residual radioactive contamination from the August 6 and 9 atomic bombings. In addition, some prisoners of war (POWs) were exposed before arrival of occupation forces. This section describes methods used in the NTPR program to estimate doses to service personnel in Japan.
The Manhattan Engineering District conducted radiological surveys in Nagasaki from September 20 to October 6 and in Hiroshima from October 3 to 7. The Naval Medical Research Institute conducted surveys in Nagasaki from October 15
to 27 and in Hiroshima on November 1 and 2. Their data and later measurements made by the Japanese constitute the basis of dose reconstructions for participants who were in either city after the war ended (McRaney and McGahan, 1980).
The radiation surveys identified two areas of contamination in each city. One was at and near ground zero, and the other was some distance downwind. Measured radiation levels were low; the exposure rate was usually less than 1 mR h−1. The residual radiation near ground zero was most likely due to neutron-induced activity. According to laboratory studies of neutron activation in soil and building materials, the only radionuclides of significance at the time of the surveys were 46Sc and 60Co. The measured exposure rates at ground zero were less than 0.075 mR h−1 at Nagasaki and 0.1 mR h−1 at Hiroshima.
The second area of contamination was due to the reported downwind “black rain” resulting from deposition of fission products. The Nagasaki surveys showed a maximum exposure rate of 2 mR h−1 at the Nishiyama Reservoir. In the downwind area at Hiroshima, the maximum exposure rate in the Kita-Kogo area (west Hiroshima) was 0.045 mR h−1 and so was much less than at Nagasaki’s Nishiyama Reservoir.
Before occupation of Hiroshima and Nagasaki, a POW recovery team arrived in Nagasaki on September 11. During the period September 11-23, about 10,000 US and allied POWs who were on Kyushu Island were processed through Nagasaki for evacuation to hospital ships. Dates of arrival and departure of major units of the occupation forces and their assigned strength and basic assignments are known. Locations of command posts and billets were believed to be outside the radiologically contaminated areas.
“Worst-case” scenarios were developed for the servicemen (McRaney and McGahan, 1980). Those scenarios assume that a participant was with his unit for the entire duration of the unit’s occupation period and that he spent 8 h d−1 within the small area defined by the highest radiation intensity. External doses at Hiroshima and Nagasaki were estimated by integrating measured exposure rates over time. Because fallout in Hiroshima was insignificant compared with that in Nagasaki, inhalation and ingestion doses were calculated only in Nagasaki. To estimate inhalation doses, the surface activity was estimated from the measured photon exposure above ground (see Section IV.C.2.1). A “high-sided” resuspension factor of 10−4 m−1 was used in the vicinity of ground zero to account for the relatively high resuspension caused by mechanical activities, and a resuspension factor of 10−5 m−1 was used at locations in fallout fields away from ground zero. In the Nagasaki fallout field, the worst-case calculation of ingestion dose assumed direct ingestion of water from the Nishiyama Reservoir. Concentrations of fallout radionuclides in water were calculated by assuming complete mixing in the reservoir. This assumption should overestimate concentrations in water because fallout particles presumably were highly insoluble and tended to deposit in sediment.
A summary of upper-bound estimates of external, inhalation, and ingestion doses to the occupiers of Japan is given in Table IV.D.1 (McRaney and McGahan,
TABLE IV.D.1 Upper-Bound Estimates of Dose to Occupiers of Hiroshima and Nagasakia
|
Hiroshima |
Nagasaki |
|||||
Ground Zero |
Fallout Area |
Ground Zero |
Nishiyama Area |
||||
41st Division |
24th Division |
41st Division |
24th Division |
2nd Division |
2nd Division (RCT-2) |
2nd Division (Artillery Gp) |
|
External dose |
0.030 rem |
0.030 rem |
0.019 rem |
0.014 rem |
0.081 rem |
0.47 rem |
0.63 rem |
Inhalation doseb |
|
||||||
Bone |
0.004 rem |
0.004 rem |
|
0.018 rem |
0.14 rem |
0.58 rem |
|
Red bone marrow |
0.033 rem |
0.13 rem |
|||||
Whole body |
0.003 rem |
0.003 rem |
0.014 rem |
0.017 rem |
0.068 rem |
||
Ingestion doseb |
|
||||||
Bone |
|
0.09 rem |
0.02 rem |
0.07 rem |
|||
Red bone marrow |
0.05 rem |
0.01 rem |
0.04 rem |
||||
Whole body |
0.03 rem |
0.01 rem |
0.02 rem |
||||
aSee Table 4 of McRaney and McGahan (1980). b50-year committed equivalent dose. cDose coefficient for red bone marrow for 46Sc was not available; dose to red bone marrow should be less than dose to bone but greater than dose to whole body. |
1980). The belief that the estimated doses are upper bounds is based on consideration of two assumptions used in the analysis. First, the assumption that a person spent 8 h d−1 in the most contaminated area for the entire period of occupation by the person’s unit should overestimate actual exposures because the area of maximum contamination was only about 1% of the entire area occupied; this assumption probably results in an overestimate of dose by at least a factor of 2. Second, it is highly unlikely that units were stationed near the Nishiyama Reservoir over the entire period; this assumption probably results in an overestimate of dose by a factor of about 10.
The results in Table IV.D.1 indicate that the maximum estimated dose was received by the 2nd Division Artillery Group in Nagasaki; the estimated external dose is 0.63 rem, and the estimated 50-year committed internal dose to bone is 0.65 rem, with lower internal doses to other organs. Again, those estimates are expected to be upper bounds of actual doses. At Hiroshima, the maximum dose occurred near ground zero. At that location, the estimated upper-bound external dose is 0.03 rem, and estimated internal doses are less than 0.005 rem. Thus, occupation forces in Hiroshima experienced little radiation exposure.
The results of the generic assessment by McRaney and McGahan (1980) described above and summarized in Table IV.D.1 represent upper-bound estimates of dose to participants who were exposed in Japan. However, when a participant files a claim for compensation or requests a dose reconstruction, an individualized dose reconstruction is often performed, based on knowledge of the participant’s times of exposure, locations, and activities. Doses assigned in individual dose reconstructions often are substantially lower than upper bounds obtained in the generic assessment.
IV.E METHODS OF ESTIMATING OR ACCOUNTING FOR UNCERTAINTY
The following sections consider the methods used in the NTPR program to estimate or otherwise take into account uncertainty in exposure scenarios and in estimates of external and internal dose. Consideration of uncertainty in all aspects of a dose reconstruction is important when the veteran is to be given the benefit of the doubt and the policy of the NTPR program is to obtain credible upper-bound estimates of dose.
IV.E.1 Uncertainty in Exposure Scenarios
Uncertainty in exposure scenarios is inherently difficult to quantify, especially uncertainty in a veteran’s activities and locations of the activities during periods of potential radiation exposure. When uncertainty in a veteran’s activities involves two or more specific choices, such as two well-characterized ships that the veteran might have been on during a given interval of time, the veteran
could be given the benefit of the doubt by assuming that he was on the more heavily contaminated ship. Sometimes, however, the uncertainty is harder to represent so specifically and has to be resolved in favor of assumptions regarding what, in the judgment of the analyst, the veteran was most likely to have done. In those cases, the attending uncertainties in dose are not quantified, and sometimes there is a comment that the assumptions being applied regarding the scenario are inherently “high-sided.” Uncertainty regarding off-duty behavior—for example, souvenir hunting, using small boats to visit contaminated islands, and engaging in dusty sports activities—is not part of any official records and is typically ignored.
Uncertainties in the assumed radiation environment in which a veteran was exposed are assessed separately. Estimated uncertainties in external exposure rates measured with field instruments are imputed to the veteran for his specific path through space and time at the location of a particular test. Such uncertainties are based on what is known about the quality of instruments used at the time to monitor radiation combined with uncertainties in the interpolation methods that are used to smooth the spatial distribution of exposure rates and model their changing patterns over time, reflecting dispersion, fallout, and known rates of radioactive decay.
IV.E.2 Uncertainty in Estimates of External Gamma and Neutron Doses
The current policy of the NTPR program is to report an upper-bound estimate of external dose with the central (“best”) estimate. The upper bound is intended to be a 95th percentile, meaning that if one calculates the distribution of possible doses for the participant, the true dose is expected to be lower than the assigned upper bound in 95% of cases with an identical exposure scenario. Although some uncertainties were estimated in early unit reports, particularly if the dose was based primarily on a unit dose reconstruction, the NTPR program did not generally report upper bounds of total external dose to the veteran or the VA before 1992. However, upper bounds were estimated for some reconstructed doses on the basis of unit dose reconstructions beginning as early as 1979. From 1989 to 1992, after the National Research Council (NRC, 1989) film badge report was issued, upper bounds were reported on the individual components of the total dose, that is, film-badge results and reconstructed doses. That approach was taken because until the 1989 film-badge report, there was no established method of reporting uncertainty in film-badge data, and until 1992 no established protocol for combining film-badge and reconstructed uncertainties (Flor, 1992; Schaeffer, 2001a). Beginning in 1992, a combined central estimate of external dose and an upper bound has been reported (Schaeffer, 2002b). According to the NTPR program, by 1996 all external gamma and neutron dose estimates reported to VA have included an upper-bound estimate.
IV.E.2.1 Film-Badge Upper-Bound Estimates
Film-badge uncertainty estimates used by the NTPR program are based on a slight modification of the National Research Council recommendations (NRC, 1989). The uncertainty for a single film-badge reading is calculated as recommended by the NRC (1989). The film-badge reading is divided by the recommended bias factor for that test series but modified by assuming that the bias factor relating exposure (in roentgen) to deep equivalent dose (in rem) is 1.0 rather than 1.3. The upper bound for an individual film-badge reading is then calculated by multiplying the bias-corrected reading by the recommended 97.5th percentile uncertainty factor K = GSD1.96 (NRC, 1989), where GSD is the geometric standard deviation. The result is then converted to a 95th percentile upper bound to achieve correspondence with the measure of an upper bound adopted by the NTPR program for use in dose reconstructions. Because of the lower net bias applied, the upper bound for a single film-badge reading is about 30% higher than had the NRC (1989) recommendations been followed exactly.
The National Research Council report (NRC, 1989) suggested that a method of estimating the upper bound of a combination of lognormally distributed film-badge data that would overestimate a desired confidence limit (be generous to the veteran) would be to sum the upper bounds of each reading. Alternatively, the report suggested that the variances of the individual bias-corrected readings could be summed, assuming that the data were independent and uncorrelated lognormal variants. The result of summing multiple badge variances generally is much lower than the sum of upper bounds. The NTPR program combines the estimated variances of the individual readings to obtain an upper bound. However, it is assumed that some of the uncertainty estimates are correlated (the uncertainty due to how the badge is worn) or partially correlated for badges from the same test series (“environmental” uncertainty and uncertainty in the “R to rem conversion”). That modification is not documented to explain the rationale for choosing the degree of correlation assumed but was conveyed to the committee via a copy of the computer program used since 1992 to calculate the upper bound for a sum of badge data (Schaeffer, 2002d). The assumption that some of the uncertainty is correlated results in a higher upper bound than assuming no correlations but gives a lower result than summing the upper bounds of the individual readings.
IV.E.2.2 Upper-Bound Estimates in Unit Dose Reconstructions
Unit dose reconstructions usually estimate the uncertainty in the average or “best-estimate” dose to a unit or subunit on the basis of estimated uncertainties in measured exposure rates, estimated decay rates, estimated positions of troops versus time, shielding, the fraction of the day spent indoors versus outdoors, and so on. The estimated 95th percentile upper bound is assumed to appropriately reflect the upper-bound dose to each individual in the unit (Goetz et al., 1981).
Most of the estimated uncertainty is generally due to uncertainty in the assumed average exposure rate on a ship or an island or to uncertainty in field measurements of exposure rates for maneuver troops or observers. However, those averages are often based on sparse data.
IV.E.2.2.1 Upper bounds in unit dose reconstructions at the NTS
The generic unit dose reconstructions discussed in Section IV.B.2 generally also included estimates of uncertainty. As stated in Barrett et al. (1987), “where errors are determined, they are estimates of uncertainty in mean dose associated with the group activities. No attempt is made to predict the distribution of dose within a unit [and] departures by individuals from the average activity scenario are not considered.”
Table IV.B.1 in Section IV.B.2.1 lists the total uncertainty (actually, the doses to be added or subtracted from the indicated dose to obtain the 5th and 95th percentile doses) estimated in a unit dose reconstruction for maneuver troops at Operation UPSHOT-KNOTHOLE at the NTS (Edwards et al., 1985). Maneuver troops observed the tests from trenches several thousand meters from ground zero. Shortly after the blast, they left the trenches and marched toward ground zero to predetermined objectives. After reaching their objectives, the troops proceeded to display areas to observe the effects of the test on various types of equipment. The calculated uncertainty range arises from two basic sources: the uncertainty in the gamma-radiation field and the uncertainty in the space-time scenario of troop movements. Errors in position, time, and gamma intensity are not independent, owing to the radiation-safety (rad-safe) constraint that limited troops to areas with exposure rates less than 2.5 R h−1 (except at Shot GRABLE). It was assumed that there was no violation of rad-safe limits, except for Battalion Combat Team (BCT)-B at Shot NANCY, so the assumption that troops kept close to the limits when detouring is expected to result in “high-sided” estimates of dose. Thus, the exposure rate of 2.5 R h−1 is assumed to apply without error under these conditions. The uncertainty is all assumed to be in the duration and path length of any detour.
In the display areas, limits of advance were not always reported. An assumption was then made that all the displays within rad-safe limits were inspected; this assumption should tend to overestimate exposures. The timing of the troops’ march was based on the reported time of attack, time of arrival at the objective, and arrival at the pickup point. Reasonable march speeds (usually about 70 ± 20 m min−1) and display-area stay times (usually 5 min with a range of 2.5−10 min) were assumed to construct a scenario consistent with the known times. The most important influence of timing on the uncertainty in dose was assumed to be the time spent at the positions of highest exposure.
The various sources of uncertainty were combined approximately because it was asserted that they could not be combined rigorously, owing to the disparity of
their associated distributions. For each source of uncertainty, the limits on dose are interpreted in terms of error factors on the best-estimate doses given in Table IV.B.1. For example, for BCT-B (forward unit) at Shot NANCY, the dose to add to or subtract from the total dose of 2.4 rem (−0.7 rem and +1.5 rem) was determined by combining the error factors for the components described below:17
-
The contribution due to uncertainty in stay times at halt points and displays: −0.5 to +1.0 rem.
-
The contribution due to uncertainty in march speed: −0.3 to +0.5 rem.
-
The contribution due to uncertainty in gamma exposure rate: −0.0 to +0.4 rem.
For both BCTs at Shot SIMON, the total uncertainty was assumed to be dominated by the time spent at the 2.5-R h−1 rad-safe limit. The total uncertainty in this case thus is assumed to be due entirely to the uncertainty in march speed and arrival and stay times. The error factors due to march-time uncertainty were estimated to be −0.06 and +0.12 rem, and the estimated error factors due to stay time were −0.13 and +0.27 rem, with a combined uncertainty range in total dose of 3.1 − 0.2 (= 2.9) to 3.1 + 0.3 (= 3.4) rem.
The uncertainty analyses for other NTS unit dose reconstructions are similar to that described above, and the central (“best”) estimates generally are asserted to be “high-sided.” However, the estimated uncertainty ranges for various parameters vary from shot to shot and among units, depending on the available information and specific exposure scenario.
IV.E.2.2.2 Upper bounds in unit dose reconstructions at Pacific test sites
The Pacific-test-site unit dose uncertainties are usually based on the estimated coefficient of variation (CV) in the measured post-shot exposure rate at various locations. In some of the dose reconstructions for the later test series, where most participants were badged, the upper bound for reconstructed doses is based on a comparison with the standard deviation of the available film-badge measurements (for example, see Weitz, 1995b; 1997). The upper bounds based on the measured post-shot monitoring data generally assume that multiple exposures to fallout from the same shot are independent, that is, that a participant is exposed at random locations each time he is outdoors or topside on a ship. The upper bound is calculated by assuming that the uncertainty in the dose incurred during each interval of exposure on deck or outdoors to the same fallout (assuming three intervals per day totaling 40% of the day topside for ships and 60% outdoors for islands) is completely independent of the previous exposure, that is, that the participant’s location with respect to the distribution of fallout exposure-rate
measurements is completely random. The uncertainty in the sum of all doses outdoors or on deck is calculated by summing the variances in each individual dose (for example, see Thomas et al., 1982; 1984). The dose incurred below decks is assumed to be very low because of an assumed 90% shielding factor. The consequence of those assumptions is that the more time the participant is assumed to be exposed to the same fallout, the smaller the estimated fractional uncertainty in the total dose received.
A default CV of 50% is usually applied to measured average exposure rates on the basis of survey data from ships on which about 30 or more measurements were made (for example, see Thomas et al., 1984). However, the CV based on the distribution of measurements on some ships or islands for some fallout events often was much greater. For many ships, only an average exposure rate was reported. If data are not available for a particular ship, data from a nearby ship or island are used because it is assumed that the amount of fallout would have been similar. Although an additional systematic uncertainty of a factor of 1.2 is asserted for the effective shielding factor18 (Thomas et al., 1984), it appears that this was not usually applied in practice. No uncertainty is apparently assumed in the decay rate or in the default CV in calculating these upper bounds.
Upper-bound factors (ratios of upper bounds to central estimates) for participants exposed for an entire test series on a particular ship or island based on the above assumptions are tabulated in the relevant unit dose-reconstruction reports. The values are usually applied and referenced in the individual dose-reconstruction reports. Although the upper-bound factor should be greater than the tabulated values for participants exposed for only a part of the test series, it appears that the same upper-bound factor was used regardless of the time exposed. If it is assumed that all exposures outdoors (and indoors on islands) are random, an estimated average upper bound for participants exposed over an entire test series will be only about 10–20% greater than the central estimate (Thomas et al., 1982; 1984).
IV.E.2.3 Upper-Bound Estimates of Neutron Dose
An upper bound of the dose from external exposure to neutrons is also estimated in the relevant unit dose reports and is based on the estimated uncertainty in the transport-calculated exposure and the uncertainty in the shielding correction. Uncertainty in the quality factor for neutrons (an uncertainty in the relative biological effectiveness of fission neutrons versus gamma rays) has not been taken into account in estimating the upper-bound neutron dose.
IV.E.3 Uncertainty in Estimates of External Beta Dose
The NTPR program does not perform uncertainty analyses for beta-particle dosimetry, relying instead on an assumption that estimates of dose are upper bounds (“high-sided”). Beta doses from contaminated ground or other surfaces, for example, are calculated by multiplying a presumably overestimated beta-to-gamma dose ratio by an upper-bound gamma dose. As noted in Section IV.B.4, the current methodology for assessment of beta-particle dose from sources external to the body is described in Barss (2000). The method has remained substantially the same since routine assessment of skin dose began in 1998, although numerical values of the beta-to-gamma dose ratios have evolved.
IV.E.3.1 Exposure to Contaminated Ground
As noted in Section IV.B.4.1, beta dose to the skin or lens of the eye from external sources is accrued simultaneously with gamma dose from radioactive fallout, contamination, or neutron-induced radionuclides. As a result, the beta dose is proportional to the gamma dose, and its magnitude can be mathematically expressed by a beta-to-gamma dose ratio.
Uncertainty in the beta-to-gamma dose ratios is discussed by Barss (2000), and sources of uncertainty are identified, with emphasis on how simplification of the assessment process leads to estimates that are higher than the likely actual doses. It is stated that the uncertainty most difficult to quantify is that in the reduction in beta-particle fluence between the source and receptor locations, which is much more dependent than gamma fluence on shielding material, chemical and physical properties of the radionuclide and the surface, and distance from the source deposited on a surface.
An example offered by Barss (2000) concerns deposition locally on the ground, for which large particles associated with tower shots provided substantial self-shielding. As a result of weathering, environmental transport, and dispersion, fallout particles may also penetrate to such a depth in soil as to substantially reduce beta doses compared with such material being on the surface. The inability to model the magnitude of each of these reduction effects adequately, and their degree of interdependence, are said to limit the usefulness of any attempt to quantitatively model their associated uncertainties.
Another example offered by Barss (2000) concerns initial decontamination techniques used on ships and aircraft surfaces (washdown systems and fire hoses), which tended to remove loosely bound or attached particles but would “fix” residual material to the surface to an extent proportional to the surface porosity or accessible surface area. The implication is that after washdown the remaining contamination would be largely fixed in surficial pores, causing more attenuation of beta particles than of gamma rays and resulting in a lower beta-to-gamma dose ratio than if the material were truly on top of a surface.
The Barss (2000) report indicates that calculated beta-to-gamma dose ratios 1 and 2 years after detonation are substantial overestimates because they ignore the effects mentioned in the two foregoing examples. The report further indicates that an enormous expenditure of resources would be needed to adequately describe and quantify the uncertainties in model parameters, given the high degree of variability in the environmental interaction with residual radionuclides. Additional resources would be required to further propagate the uncertainty associated with each model parameter to obtain an estimate of the overall uncertainty in a calculated beta dose.
The report notes that although there are environmental models that attempt to achieve the objectives (quantify parameters and propagate uncertainty), their usefulness remains inversely proportional to their degree of scientific debate and interpretation. The discussion concludes that the best resolution of the dilemma (quantification of uncertainty), in the absence of a rigorous and scientifically appropriate approach to quantify and apply modification factors for environmental and particle effects, is consideration of field measurements. It is stated that, in some comparisons, the current beta-to-gamma dose ratios are in reasonably good agreement with previous calculations and available measurements and, at worst, overestimate the measurements by a factor of 2-3.
There is no discussion of factors that might cause underestimation of beta doses, such as errors in estimating time since detonation and underestimates of distances from contaminated surfaces or exposure times. It is clear from Barss (2000) that application of the method is expected to result in a “high-sided” dose.
IV.E.3.2 Immersion in Contaminated Air or Water
As noted in Section IV.B.4.2, beta doses from immersion in contaminated air or water are calculated by using dose coefficients, durations of exposure, and composite beta-spectrum radiation energies associated with a reconstructed gamma exposure or film-badge reading. The calculated beta dose is added to the upper-bound gamma dose for the corresponding period.
There is no discussion of uncertainty by Barss (2000) related to beta-particle doses from immersion, although it seems clear from examination of Figure IV.B.8 (see Section IV.B.4.2) that a small uncertainty in the time of onset of exposure could lead to a large uncertainty in a composite dose coefficient, particularly during the period shortly after detonation.
IV.E.3.3 Skin Contamination
As noted in Section IV.B.4.3, dose coefficients from Kocher and Eckerman (1987) can be used to calculate beta dose from skin contamination, with adjustments for backscatter and for the case in which a glove is contaminated. The
VARSKIN code (Durham, 1992) can be used to calculate skin dose for specific source geometries. Skin-contamination measurements are recommended as the best source of contamination data from which to calculate dose, but methods are also suggested for using dose or exposure-rate measurements to estimate contamination.
Barss (2000) does not discuss uncertainty related to beta-particle doses from skin contamination.
IV.E.4 Uncertainty in Estimates of Internal Dose
Estimates of uncertainty in calculated internal doses are not presented in dose reconstructions for individual atomic veterans or in unit dose reconstructions for participant groups. In all dose reconstructions that include an estimate of internal dose, the calculated dose is presented as a single value without uncertainty. Uncertainties in internal doses are also not evaluated or discussed in any detail in reports documenting the calculation methods (Egbert et al., 1985; Barrett et al., 1986).
Thus, the treatment of uncertainty in estimated internal doses differs from the approach to addressing uncertainty in estimated doses from external exposure to photons. As discussed in Section IV.E.2, dose reconstructions for individual veterans often provide an estimated upper bound of the external photon dose, especially if the veteran filed a claim for compensation. Many generic dose reconstructions for participant groups also include a quantitative analysis of uncertainty in external photon dose. An upper-bound estimate of external photon dose is intended to represent a 95% confidence limit, and the difference between the upper bound and the central estimate indicates the magnitude of uncertainty. Upper-bound estimates of dose are important because, in accordance with the policy that the veteran will be given the benefit of the doubt (see Section I.C.3.2), the NTPR program intends that upper bounds will be used in evaluating claims for compensation.
In the absence of a quantitative analysis of uncertainty in estimated internal doses, this uncertainty is addressed in the NTPR program by using an alternative approach mentioned in Section I.C.2.4. An argument is made that methods used to estimate internal doses incorporate assumptions that should result in overestimates of internal doses to participants. For example, the method of estimating dose from inhalation of resuspended fallout that was previously deposited on the ground or other surfaces (see Section IV.C.2.1) relies mainly on an assumption that resuspension factors that are applied in various exposure scenarios greatly overestimate the actual extent of resuspension of deposited fallout. On the basis of that type of argument, estimates of internal dose obtained in dose reconstructions are assumed to represent suitable upper bounds for use in evaluating claims for compensation; that is, the estimated doses are assumed to be “high-sided.” As discussed in Section IV.E.3, essentially the
same approach to accounting for uncertainty is used in estimating external beta dose to the skin or lens of the eye.
The committee reiterates that an approach of relying on “high-sided” assumptions to estimate credible upper bounds of possible doses, rather than an approach involving a quantitative analysis of uncertainty in a central estimate, is a reasonable way to address uncertainty. Furthermore, an upper bound so obtained is appropriate for use in evaluating claims for compensation. However, it is a valid approach to addressing uncertainty only if estimated doses are indeed “high-sided.” That is, on the basis of available information and scientific judgment, there must be a high degree of confidence that calculated internal doses do not underestimate actual doses to participants.
Thus, an evaluation of methods used in the NTPR program to estimate internal doses to atomic veterans essentially involves an assessment of the extent to which the methods are likely to overestimate doses. The committee’s evaluation of the methods of estimating internal dose is presented in Section V.C.
IV.F ESTIMATES OF TOTAL DOSE AND UNCERTAINTY FOR INDIVIDUAL PARTICIPANTS
Although many participants have received a dose assessment from the NTPR program based on film-badge data in their medical records or their unit’s generic dose reconstruction, VA may request a formal dose reconstruction from DTRA to evaluate a claim for compensation (see Section III.B). A veteran may also request a detailed dose reconstruction by directly contacting DTRA. An individual dose reconstruction attempts to determine all possible pathways and sources of exposure for the participant on the basis of his military records and personal statement. The analyst reviews the assumed exposure scenario for the participant and modifies or recalculates the unit dose reconstruction according to the time exposed (which may have differed from the time assumed in the generic reconstruction), special duties or missions, available film-badge data, and so on. The analysis and dose estimate are reported in a detailed memorandum that specifies the assumed exposure scenario, exposure rates and decay rates, references to the applicable unit dose reports, and any other analysis methods applied (see Section IV.G.1). If the participant was exposed at various times and places, the memorandum reports the estimated dose from each exposure and sums the individual doses to determine the total for all exposures from all test series that the veteran participated in.19 The neutron dose in rem is added to the estimated whole-body gamma dose, and the total is reported to VA or the veteran when an external dose is reported.
Reconstructions of external dose that were done in recent years (1998 and later) also often include an assessment of dose to the skin or lens of the eye, particularly for participants claiming compensation for skin cancer or cataract, and these assessments include the contributions from beta exposure. Appendix A contains two examples of dose-reconstruction memoranda from sample cases reviewed by the committee.
Some individual dose reconstructions are unique—that is, not covered by a generic unit dose-reconstruction method—and require a fairly complex dose assessment. For these cases, the dose-reconstruction memorandum details the assumptions made in estimating the dose.
The total dose reported to VA or the veteran consists of the best estimate of the gamma-plus-neutron equivalent dose from all sources of external exposure and an upper-bound (95th percentile) estimate that combines the estimated upper bounds from each source of exposure and from estimates based on film-badge data and reconstructions. The neutron and gamma upper-bound estimates also are combined to estimate an upper bound for the sum. As discussed in Section IV.B, total upper bounds for external gamma-plus-neutron dose have been consistently reported in a formal dose reconstruction since 1996. The upper-bound calculations typically assume that exposures to different shots, or at different locations, are not correlated and can be combined in quadrature by summing the variances (Flor, 1992). In addition, DTRA is often asked by VA to provide upper-bound estimates for generic or film-badge doses that were previously provided to the veteran or VA for which upper bounds had not been estimated. Those upper-bound requests often result in the reporting of a revised central estimate based on a new method or new exposure scenario information.
If a skin or eye dose from beta exposure is calculated, it is reported separately. Estimates of beta dose are already considered to be “high-sided,” so no additional upper bound is reported. If a claim involves a disease of a specific organ, and an internal (inhalation) dose has been calculated for that organ, the calculated organ dose is also reported separately. As discussed in Sections IV.C.2.1.7 and IV.E.4, this estimate is also intended to be “high-sided,” so no additional upper bound is reported. (Often, even though an actual “high-sided” inhalation dose estimate is provided, the inhalation dose is also reported as less than the screening criterion of 0.15 rem; see discussion of the low-level internal dose screen in Section VI.C). When no specific organ dose is calculated, a committed effective dose equivalent from inhalation is sometimes calculated (see Section IV.C.2). Again, it considered to be a “high-sided” estimate, and no additional upper bound is reported.
Although the NTPR program does not combine external and inhalation dose estimates to estimate the total and upper-bound doses to a specific organ, the VA practice is to sum the reported external-dose upper bound (if an upper bound is provided) with the reported “high-sided” inhalation (internal) dose estimate to
obtain an estimate of the upper bound of the organ dose. As discussed in Section III.E, the sum is used in the process of evaluating whether it was at least as likely as not that a veteran’s disease was caused by the radiation exposure.
IV.G DOCUMENTATION AND QUALITY ASSURANCE
IV.G.1 Documentation of Dose Reconstructions
The documentation of dose reconstructions for individuals required by the NTPR program is specified in the standard operating procedures (SOPs) (DTRA, 1997). However, the discussion in the SOPs appears to be limited to the documentation that is to be sent to the veteran or his representative, rather than a complete documentation requirement. The SOPs state that: “In order to consistently serve the veteran, the veteran (or his representative) needs disclosure of the information that leads to his dose.” The documentation requirements are summarized as follows:
-
Documentation pertaining to relevant generic (unit) dose reconstructions.
-
All scenario and radiological information pertinent to the dose determination (explicitly or by reference).
-
Detailed information or analysis not fully covered in previous documents, which is to be communicated in an individual dose memorandum attached to the case correspondence or in the body of the correspondence.
-
Information that is too complex or generic or that otherwise detracts from the presentation of the individual dose memorandum or correspondence, which is to be covered by fact sheets (or other written material) distributed to the correspondent.
-
Information on availability of cited formal reports and unpublished documents (subject to Privacy Act-related redactions), which is to be included in the case correspondence.
-
Appropriate disclosure of other information, including representations from the time of the operation, such as operational summary data or data entered into individual records, even if such information is not corroborated; explanation of when this information, if it is not the most credible, is not retained in the final analysis; and other types of information that do not necessarily furnish the dose of record, such as dose entries in medical records and information contradicted elsewhere in records.
Beyond the referenced information, an individual dose reconstruction or synopsis should include an explanation of what is specific to the veteran’s case, for example:
-
The adaptation from a published report of the dose for the veteran’s period of participation.
-
The principal source of uncertainty that affects the upper-bound dose.
-
That the potential for an internal dose has been considered, even if the finding is of no internal dose.
-
That a finding obtained from internal dose screening (see Section VI.C) applies to the veteran’s target organ.
-
That internal dose assessments do not apply to assessments of dose to skin or lens of the eye.
-
The reason for a change in estimated dose from previous correspondence if the change is based on new data (a change that results from a procedural change is addressed in the correspondence but not in a dose-reconstruction memorandum).
-
Reporting of total doses in a dose summary, which is in tabular form if there are multiple contributions to external gamma or neutron dose.
The specifications for documentation discussed above are related to what should be provided to the veteran. Also of concern to the committee is the detailed internal documentation of the dose reconstructions themselves, which is necessary to make detailed, independent reviews possible. This is discussed in Section VI.A.
IV.G.2 Quality Assurance
The committee did not see details of a formal quality assurance (QA) program in the SOPs (DTRA, 1997), and the files of individual dose assessments reviewed by the committee did not contain the expected indications of a systematic QA process. The committee was informed (Schaeffer, 2001a) that: “There are no additional quality assurance written procedures other than those provided to the committee. The SOP indicates what constitutes a quality dose reconstruction and directs review for conformity with the SOP’s procedures, and appropriateness and responsiveness to the correspondence or request received by the NTPR program. The DTRA Program Manager conducts the final review/approval.”
The committee notes that the SOPs (DTRA, 1997) constitute more of a program overview than a detailed document that could guide specific day-to-day work, and they have little to say about QA. They do, however, specify the documentation discussed in the previous section that should accompany a dose assessment, to serve the veteran consistently, and that the assessment is to be reviewed (but not by whom).
On further inquiry by the committee, additional information on QA for the dose reconstruction program was given in a letter from DTRA (Schaeffer, 2002e), which is provided in Appendix D. The letter indicates that QA had always been a key element in management and direction of the NTPR program and that the DTRA solicitation for NTPR program support contained a program-management
requirement for QA monitoring, which was one of the contract-evaluation factors for award. In response to the solicitation, the contractor submitted a technical proposal that specified QA measures.
The statement of work included in the DTRA solicitation for NTPR program support indeed contains the following requirement for quality assurance: “The contractor shall provide quality assurance monitoring for the NTPR Program in the areas of database management, dose assessment, and veteran assistance.” As stated by DTRA (Schaeffer, 2002e): “In response to the solicitation, JAYCOR/SAIC submitted a technical proposal that specified quality assurance measures in the program task areas of database management, radiation exposure assessment, and veteran assistance.” The committee did not have the opportunity to review the technical proposal submitted by JAYCOR and SAIC and consequently did not see the specified QA measures.