7
Life, Fossils, and Reduced Carbon
PRESENT STATE OF KNOWLEDGE
The search for evidence of extant or fossil martian life will focus on life forms that are carbon-based, that require water, and that are microbial. The implied assumptions are well justified. All known forms of life are carbon-based and require water. Throughout Earth’s history, microbe-level life has been predominant—“simple” life forms can be expected to exist even if more advanced organisms have arisen subsequently. Moreover, it seems likely that life on Mars, if there is any, would take forms similar at some fundamental functional level to microorganisms on Earth, since the same laws of physics as they relate to biological energy transformations and to potentially habitable environments presumably apply on both planets. The subject of life detection in extraterrestrial samples is discussed in a recent study by the Space Studies Board.1
Microbes on Earth
Microbes are capable of surviving and growing under a broad range of environmental conditions (light intensity; total salinity; pH; temperature; oxygen abundance; carbon dioxide concentrations; water availability; and fluxes of ultraviolet, x-ray, gamma-ray, and highly ionizing radiation).2 Environments inhabited by microbial assemblages are equally diverse—searing sabhkas;3 antarctic lakes;4 rock crusts of frozen deserts;5 sea ice at -35°C (see Figure 7.1); solid salt crystals;6 seafloor hydrothermal fluids;7 and groundwaters and pore spaces of deep subsurface rocks.8,9,10 In recognition of this environmental flexibility, it has been proposed that particular assemblages of microorganisms having specific physiological capabilities could survive on Mars.11,12,13,14,15 Some of these proposals assume that the primary producers in such assemblages would be light-dependent (photosynthesizing) microbes for which survival at the martian surface may be precluded by harsh conditions. If there is no feasible photosynthetic zone on Mars, any extant life would have to obtain energy from inorganic sources. Such sources are used by terrestrial microbes in deep marine environments16 and in the pore spaces of deep subsurface rocks,17 but the detailed physiology of these microbes and the biological composition, spatial distribution, and ecology of the systems they live in have been incompletely investigated.
The present martian surface is oxidizing, desiccated, and bathed in intense ultraviolet radiation. It is possible, therefore, that the martian regolith (the pulverized rock debris that covers most of the surface) will prove to be uninhabitable by any living system and inimical to all but short-term survival of organic carbon.18 Conditions less
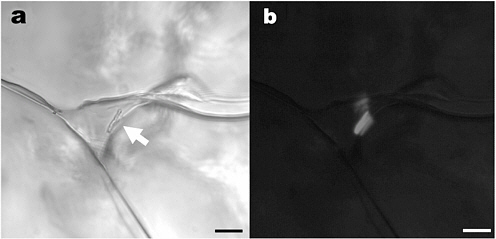
FIGURE 7.1 Inclusion of brine (triangular) at a triple junction between ice crystals in sea ice. Scale bars, 10 µm. (a) Transmitted light; arrow points to two rod-shaped bacteria along the wall of the inclusion. Higher magnification reveals apparent cell division. (b) Epifluorescent image of the same field; the bacteria are DAPI stained. Note the reflectance of the fluorescent emission from the organisms off the ice walls. SOURCE: K. Junge, C. Krembs, J. Deming, A. Stierle, and H. Eicken, “A Microscopic Approach to Investigate Bacteria Under In-Situ Conditions in Sea-Ice Samples,” in Selected Papers from the International Symposium on Sea Ice and Its Interactions with the Ocean, Atmosphere, and Biosphere, Fairbanks, Alaska, 18–23 June 2000, M.D. Jeffries and H. Eicken (eds.), Annals of Glaciology 33:304-310, 2001.
hostile to life may exist in sheltered habitats, and highly resistant spores or cysts dispersed by putative organisms occupying these or even more clement environments (such as the deep subsurface) might survive in the regolith. Moreover, as discussed below, the martian surface may have harbored life at an earlier time, when conditions were more favorable. If so, microscopic fossils of such organisms may be preserved in appropriately ancient sedimentary settings, and the evolutionary descendants of these microbes may inhabit the martian subsurface today. Indeed, if reports are valid of microbes surviving for 25 million to 40 million years encased in amber19 and up to 100 million years encased in halite crystals,20 it is conceivable that viable biological remnants might be harbored by sedimentary mineral precipitates on the martian surface. Mineral deposits of this sort also seem a promising site for the detection of inorganic microbial pseudomorphs (mineral structures precipitated as a result of microbial physiologic activity), just as they are on Earth in a number of settings.21,22,23,24 Examples of especially ancient (Precambrian) terrestrial microfossils are shown in Figure 7.2.
The Search for Extant Life on Mars
Possible Abodes
The surface of Mars today is cold, dry, chemically oxidizing, and exposed to an intense flux of solar ultraviolet radiation. These four factors are likely to limit or even to prohibit life at or near the surface of the martian regolith.
Temperature is of interest not only because of its controlling influence on microbial metabolic rates, but also because of its influence on the stability of liquid water. Although the peak daytime surface temperature near the
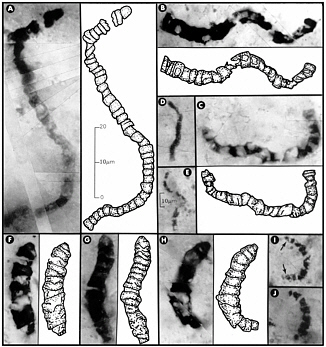
FIGURE 7.2 Carbonaceous prokaryotic fossil microorganisms, with interpretive drawings, in 3.46 billion-year-old siliceous rocks (chert) from Western Australia. All these fossils are completely encased in chert, which has been made into petrographic thin sections, rendering them visible under the microscope. The 10-µm scale bar in E is also applicable to D, I, and J; all other images are to the scale shown in A. SOURCE: Reprinted with permission from J.W. Schopf, “Microfossils of the Early Archean Apex Chert,” Science 260:640-646, 1993. Copyright 1993 by the American Association for the Advancement of Science. For additional information and an alternative interpretation see, for example, J.W. Schopf, A.B. Kudryavtsev, D.G. Agresti, T.J. Wdowiak, and A.D. Czaja, “Laser-Raman Imagery of Earth’s Earliest Fossils,” Nature 416:73-76, 2002; and M.D. Brasier, O.R. Green, A.P. Jephcoat, A.K. Kleppe, M.J. Van Kranendonk, J.F. Lindsay, A. Steele, and N.V. Grassineau, “Questioning the Evidence for Earth’s Oldest Fossils,” Nature 416:76-81, 2002.
martian equator can rise above the freezing point of water during much of the year, the average surface temperature is about -55° C, well below the freezing point of water.
All life on Earth is based on aqueous chemistry—liquid water is essential for life as we know it. Water is abundant on Mars (see Chapter 6 in this report), but not in liquid form.25 Water vapor and ice crystals are present in the atmosphere, and water ice is almost certainly present within the martian regolith at high latitudes and at the surface in polar regions. During the half-year-long, north-polar summer, the water ice present in the residual polar cap warms sufficiently to allow water to sublime into the atmosphere and be distributed globally. Because dissolution of salts can lower the freezing point, it is possible that liquid water exists transiently on or near the martian surface. Such occurrences are probably very rare, since such saline liquids would rapidly evaporate.26 Alternatively, ice crystals trapped in closed pores in rocks or regolith grains could melt under certain circumstances, and the resulting liquid water could be prevented from evaporating by virtue of being enclosed. At
increasing depth, as subsurface temperatures rise as a result of the planetary geothermal gradient, liquid water may be present in pore spaces.27,28
The evidence that the surface environment is highly oxidizing derives from analyses by instruments carried aboard the 1976 Viking landers. Although the exact nature of the oxidants has not been determined,29 the most likely dominant oxidant appears to be hydrogen peroxide, believed to form photochemically from atmospheric water vapor and to diffuse readily into the regolith. Such oxidants would react with organic molecules (including those that make up microbes) and may be responsible for the reported absence of organic molecules or their fragments in the martian samples analyzed by the Viking landers, despite the fact that organic material has been continually added to the regolith over planetary history by the impacts of carbonaceous meteorites.30
The martian atmosphere is thin, having an average pressure of ~6 mbar, and consists primarily of carbon dioxide. Because of the low concentration of atmospheric ozone, solar ultraviolet light reaches the surface of Mars with much less attenuation than on Earth, which is shielded by an ozone layer in its atmosphere. On Mars, winter-hemisphere atmospheric ozone can absorb some of the impinging ultraviolet radiation, but only during a brief period of the year and only over a small fraction of the planet. Thus, the entire martian surface is subject to an intense flux of ultraviolet radiation. Unless putative martian microbes were in some way shielded by ultraviolet-absorbing carapaces, composed of remarkably ultraviolet-stable organic compounds, and/or possessed intracellular molecular repair mechanisms decidedly more effective than those present in known terrestrial forms of life, any martian microbes would be expected to survive only in habitats protected from such radiation.
Status of the Search
The accepted interpretation of results from the Viking landers is that the surface materials tested were devoid of organic molecules and of any other evidence of life.31 However, even without consideration of alternative interpretations,32 the Viking results cannot be taken as indicating that life does not currently exist on Mars. Organisms at the Viking sites might have been missed because the experimental conditions (nutrients provided, processes followed) may not have been chosen correctly. Even more importantly, martian life might reside in aqueous oases, such as any recently active volcanic vents or fumaroles distant from the Viking landing sites, or at depths far beneath the surficial regolith sampled by the Viking experiments. Dormant life could exist for a time in dry settings, such as the interiors of rock fragments excavated from depth by cratering events.
The Search for Past Life on Mars
Possible Sources
The surface environment of Mars may not always have been as hostile to life as it is today. Early in the planet’s history, the average temperature may have been warmer and the atmosphere more dense, and liquid water may have existed at the surface.
Evidence for the presence of surface water early in martian history is preserved in the geomorphology of the planet’s surface (see Chapter 5 in this report), particularly in areas interpreted on the basis of the distribution and density of meteoritic impact craters to be older than about 3.5 billion years. Two aspects of this particularly ancient terrain suggest that the early climate differed substantially from that of the present.33 First, large, early-formed craters have been nearly obliterated or substantially degraded in a style regarded to be indicative of aqueous erosion. Later-formed craters have survived essentially intact, suggesting that rates of erosion early in martian history may have been as much as 1,000 times greater than those of more recent epochs. Second, the pre-3.5 billion-year-old surface exhibits networks of dendritic valleys that have geomorphologic patterns closely similar to those of terrestrial stream channels carved by water. Regardless of the exact mechanism of formation of these networks (whether by runoff of precipitation, seepage or “sapping” of subsurface water, or erosion by water-rich debris flows or catastrophic floods), the valleys provide strong evidence of the presence of liquid water at or very near the martian surface.34 Overall, the geomorphologic evidence indicates that the martian climate was wetter, warmer, and appreciably more hospitable to life prior to about 3.5 billion years ago than it is at present.
Hot-spring environments, another setting habitable by microbial forms of life, may also have been present, perhaps abundantly, on early Mars.35 The formation of hot springs and hydrothermal systems requires the co-occurrence of crustal water and substantial heat. Meteoritic impacts, particularly common early in martian history, would have provided a strong source of local heating. Moreover, isotopic evidence from martian (SNC) meteorites indicates that Mars, like Earth, underwent crustal differentiation and, probably, global melting, during and shortly after planetary accretion. Thus, volcanism, no doubt especially extensive in early martian history (and possibly continuing to the near-present36), would have served as a second major heat source. Given the extensive evidence both of crustal water and of strong heat sources, biologically habitable hot springs and hydrothermal systems are likely to have been common on ancient Mars (and perhaps to have persisted throughout martian history).
A third widespread zone that seems likely to have been habitable throughout martian history is the crustal subsurface, where water may exist in a liquid state. The geothermal gradient of Mars is probably such that liquid water is present at depths as shallow as 2 km near the equator.37 The discovery of terrestrial microbes living deep within the Columbia River basalts in the Pacific Northwest of the United States38,39 and elsewhere on Earth, at depths as great as 3 km,40 is consistent with the possible presence of microbes living in similar settings on Mars. On Earth, such microbes survive by metabolizing hydrogen produced by chemical reactions between pore water and the enclosing rocks. Although they are thought to be completely independent of the food chain and chemical gradients established by photosynthetic organisms at Earth’s surface, they presumably migrated into such deep subsurface settings from elsewhere rather than having originated in place. If life was present at or near the surface of Mars early in its history, when the surface environment must have been wetter, warmer, and biologically more benign than at present, a similar migration to the subsurface might have occurred as the martian surface became increasingly less hospitable.
To summarize, fossil evidence of past martian life, if there is any, may be preserved in surface water-laid deposits such as lake or streambed sediments, in evaporitic mineral pans,41 and in hydrothermally deposited mineral crusts. Subsurface settings are not as readily accessible, but materials from them might have been dislodged and brought to the surface by meteoritic impacts.
Status of the Search
Within the past few years, results of detailed studies have been interpreted as indicating that martian (SNC) meteorite ALH84001 contains possible evidence of biological activity thought to date from about 3.6 billion years ago.42 The possibly biological indicators detected include mineral-zoned carbonate disks and grains of magnetite similar to those interpreted in some terrestrial deposits as being of biological origin, polycyclic aromatic hydrocarbons interpreted to be possible remnants of decayed and geochemically altered biological organic matter, and globular or filamentous microscopic structures thought possibly to be microbial fossils. These claims concerning ALH8400143 have engendered much discussion, both pro and con, regarding each of the several intriguing indicators proposed.44 To date, none has been shown to be decisively of biological origin. But the widespread interest stirred by the report illustrates the importance attached to the question of the past or present existence of life on Mars, by both the scientific community and the public at large.
It is important to maintain as the focus of the search for life the question, Did life ever arise on Mars? This is a broad and complex question, and the evidence may be so deeply buried in the past that the question can be answered only by acquiring an extensive and deep knowledge of Mars. For example, on Earth, enzyme-driven metabolic processes can create characteristic biogenic isotopic signatures (affecting, in particular, the ranges of compositions of the stable isotopes of carbon, sulfur, nitrogen, hydrogen, and possibly iron). However, in order to use isotopic measurements to test for the past presence of life, one needs to know the scope of abiotic fractionating processes. The search for life should be based on this premise, and one should be as prepared for a negative answer as for a positive one. The importance of a positive answer is clear, but a negative answer would prompt inquiries into what the implications are for the planetary differences between Earth and Mars.
NEAR-TERM OPPORTUNITIES
The search for evidence of present or past life on Mars is a classic hunt for a “needle in a haystack.” Accordingly, the appropriate strategy is to first identify the “haystack”—promising sedimentary deposits and mineral accumulations, concentrations of reduced carbon—and only later to expect to find the “needle” that may be hidden therein: living microbes, fossil microbes, or diagnostic organic compounds and isotopic compositions. In any case, the context for the needle and the haystack must be well understood through a balanced exploration and science program. The first sample-return mission will be the start of this process. Further missions are likely to extend for a half century or more. Success must be measured not in terms of finding the needle but in terms of steadily increasing our understanding of Mars and whether it could ever have had its own biota.
Robotic Exploration
Analyses from orbit hold promise for identifying biologically or paleontologically favorable sites for sample collection—lake or streambed sediments, evaporitic mineral pans, and hydrothermal deposits, hot springs, and similar locales.
The Mars Exploration Rovers, scheduled for launch in 2003, and the Mars Science Laboratory (MSL),a scheduled for launch in 2007,b will contribute strongly to an overall understanding of the Mars system and thus provide vital information about the context for life and for prebiotic chemical processes. These are very significant points that will help to refine thinking about biological issues, but no decisive revelations about martian life can be expected from these missions. Especially if equipped with means for appropriate chemical analyses and imaging at the proposed 30-µm resolution, the rovers hold promise for useful in situ analyses of evaporitic mineral crusts and finely layered hydrothermal and other sedimentary deposits, as well as a search for concentrations of reduced carbon. The U.K.’s Beagle 2, scheduled for launch in 2003 aboard the European Space Agency’s Mars Express, is most promising in this regard. Uniquely among the landed missions and rovers, it has the potential to provide results that bear directly and dramatically on the life sciences at the same time that it contributes strongly to important planetological questions.
Rovers will come to have the capability of drilling, but it is questionable whether they can ever reach beneath the surface zone of Mars in which conditions are particularly hostile to life and apparently to the survival of organic materials. Moreover, although any cores collected can be studied robotically, it would be far more profitable to return them to Earth for study.
Returned Samples
The capabilities of robotic instruments that can be launched from Earth and flown to Mars are dwarfed by those of Earth-bound laboratories. Diverse physical and chemical characteristics of any returned sample of martian soil can be analyzed grain by grain. The results will provide information not just about a particular sample of soil, but about all of the rocks that had been weathered to provide that soil, about the fluids with which those grains had come in contact, and about the processes by which the grains had reached the site of collection. The resulting picture will provide information about the region from which the samples are collected and about the history of the whole martian environment. Robotic missions have much to offer but, in terms of the search for life or for evidence of past life, access to returned samples is incomparably superior to remote analyses.
RECOMMENDED SCIENTIFIC PRIORITIES
Earlier studies of Mars exploration strategy (see Appendix B: [1.7, 1.19, 3.3, 5, 6.2, 7, 9.1, 10.3, 11.3.1]), reinforced by the establishment and importance of the Astrobiology program in NASA, have given very high priority to the search for life, extant or fossil, on that planet. Among the earlier recommendations are these:
-
From the 1990 COMPLEX report 1990 Update to Strategy for Exploration of the Inner Planets:45 “Consistent with the SSB report The Search for Life’s Origins: Progress and Future Directions in Planetary Biology and Chemical Evolution (National Academy Press, Washington, D.C., 1990), the committee endorses the continued search for evidence of past life and biochemical evolution on Mars, as well as the continuing study of the history of water on Mars” (Appendix B: [3.3]).
-
From the 1998 COMPLEX letter report “Assessment of NASA’s Mars Exploration Architecture”:46 “An appropriate focus for NASA’s Mars program is the comprehensive goal of understanding Mars as a possible abode of past or present life” (Appendix B: [9.1]). The wording of this recommendation is highly significant. Understanding Mars as a possible abode of life will be a stepwise process. Mandatory and fundamental steps are the establishment of a geochronologic framework for martian surface deposits, reconstruction of the history of volatiles at the martian surface in as much detail as possible, and development of a good overview of the geologic processes affecting the martian surface. As that work proceeds, it will be possible to define new objectives more tightly focused on biological processes and products. Returning to the needle-in-a-haystack analogy, first one must find the right fields, then select the most promising haystacks, and then search for the needle.
-
From the 2000 MEPAG report “Mars Exploration Program: Scientific Goals, Objectives, Investigations, and Priorities”:47 “Explore high priority candidate sites (i.e., those that provide access to near-surface liquid water) for evidence of extant (active or dormant) life” (Appendix B: [11.3.1]).
ASSESSMENT OF PRIORITIES IN THE MARS EXPLORATION PROGRAM
NASA has made the search for life, or evidence of it, central to its program of Mars exploration. The definitive detection of life on Mars and its study will almost certainly require the return of samples to Earth (see Chapter 11 in this report). Unfortunately, in response to mission failures in 1999 and to the ensuing restructuring of its plans for Mars exploration, NASA has deemed it necessary to defer the launch of the first sample-return mission by at least 8 years, from 2003 when the first launch had originally been planned to 2011 under the Mars Exploration Program schedule.
The priorities recommended by advisory panels, including those quoted above, can be summarized by what has come to be an often-cited NASA slogan: “Seek, In Situ, Sample.” The first two elements of this slogan are being pursued, in the form of the robotic orbiter and lander missions described throughout this report, and COMPLEX judges that the planned effort is sufficient to set the stage for rational choice of a landing site in 2011 (see Chapter 12 in this report). Regarding the third element of the slogan, little has been done except to commit to it in principle. NASA has taken few concrete actions in support of sample-return missions. COMPLEX recommends that the following important steps be taken (see also Chapter 12).
Recommendations
-
The Mars Quarantine Facility in which martian samples will be processed, stored, and released for scien-tific study, and in which a very limited range of studies will be carried out, must be designed, built, and certified.48
-
Research must be initiated on several outstanding questions that will affect the design of the Mars Quaran-tine Facility (e.g., combining biological isolation with clean-room conditions; establishing the efficacy and detrimental effects of sterilization techniques).49
-
The study of life in extreme environments on Earth, which can aid in the design of life-detection tests, should be supported, as is already being done. In general, research areas that improve sensitivities of life-
detection techniques must be supported, and a life-detection protocol to be implemented and tested in the Mars Quarantine Facility must be developed.
-
Techniques must be developed for the collection, packaging, and return of samples.
-
The research programs of Mars orbiter and lander missions must be designed to support the collection of those samples with the greatest potential for life detection (this process is underway).
NASA should focus its Mars program, and sample-return missions in particular, on the comprehensive goal of understanding Mars as a possible abode of life. That is, the interpretation of biologically relevant in situ and laboratory observations can be maximized only if data are gathered in the context of a broad framework of research aimed at understanding the origin and evolution of the martian environment.50
REFERENCES
1. Space Studies Board, National Research Council, Signs of Life: A Report Based on the April 2000 Workshop on LifeDetection Techniques, National Academy Press, Washington, D.C., 2002.
2. See, for examples, references 33 through 61 in J.W. Schopf, “Disparate Rates, Differing Fates: Tempo and Mode of Evolution Have Changed from the Precambrian to the Phanerozoic,”Proceedings of the National Academy of Sci-ences91: 6735-6742, 1994.
3. B.K. Pierson, “Modern Mat-Building Microbial Communities: Introduction,”pp. 247-251 in The Proterozoic Bio-sphere—A Multidisciplinary Study, J.W. Schopf and C. Klein (eds.), Cambridge University Press, New York, 1992.
4. B.C. Parker, G.M. Simmons, Jr., G. Love, R.W. Wharton, and K.G. Seaburg, “Modern Stromatolites in Antarctic Dry Valley Lakes,”Bioscience31: 656-661, 1981.
5. E.I. Freidman and R. Ocampo-Freidman, “Endolithic Microorganisms in Extreme Dry Environments: Analysis of a Lithobiotic Microbial Habitat,”pp. 177-185 in Current Perspectives in Microbiology, M.J. Klug and C.A. Reddy (eds.), American Society of Microbiology, Washington, D.C., 1984.
6. C.F. Norton, T.J. McGenity, and W.D. Grant,“Archeal Halophiles (Halobacteria) from Two British Salt Mines,”Journal of General Microbiology139:1077-1081, 1993.
7. H.W. Jannasch, “Microbial Interactions with Hydrothermal Fluids,”pp. 273-296 in Seafloor Hydrothermal Systems:Physical, Chemical, Biological, and Geological Interactions, Geophysical Monograph 91, American Geophysical Union, Washington, D.C., 1995.
8. T.O. Stevens and J.P. McKinley, “Lithoautotrophic Microbial Ecosystems in Deep Basalt Aquifers,”Science270: 450-454, 1995.
9. T.O. Stevens, “Lithoautotrophy in the Subsurface,” in Proceedings of the Third International Symposium of Subsur-face Microbiology, Sept. 15-21, 1996, Davos, Switzerland, Swiss Society of Microbiology, Zurich, 1996.
10. S. Kostelnikova and K. Pederson, “Ecology of Methanogenic Archea in Granitic Groundwater from Hard Rock Laboratory, Sweden,” in Proceedings of the Third International Symposium of Subsurface Microbiology, Sept. 15-21, 1996, Davos, Switzerland, Swiss Society of Microbiology, Zurich, 1996.
11. E.I. Freidman and R. Ocampo-Freidman, “Endolithic Microorganisms in Extreme Dry Environments: Analysis of a Lithobiotic Microbial Habitat,”pp. 177-185 in Current Perspectives in Microbiology, M.J. Klug and C.A. Reddy (eds.), American Society of Microbiology, Washington, D.C., 1984.
12. C.P. McKay, E.I. Freidman, R.A. Wharton, Jr., and W.L. Davies, “History of Water on Mars: A Biological Perspec-tive,”Advances in Space Research12: 231-238, 1992.
13. C.P. McKay, R.L. Mancinelli, C.R. Stoker, and R.A. Wharton, Jr., “The Possibility of Life on Mars During a Water-Rich Past,”pp. 1234-1245 in Mars, H.H. Kieffer, B.M. Jakosky, C.W. Snyder, and M.S. Matthews (eds.), University of Arizona Press, Tucson, 1992.
14. P.J. Boston, M.V. Ivanov, and C.P. McKay, “On the Possibility of Chemosynthetic Ecosystems in Subsurface Habi-tats on Mars,”Icarus95: 300-330, 1992.
15. T.O. Stevens and J.P. McKinley, “Lithoautotrophic Microbial Ecosystems in Deep Basalt Aquifers,”Science270: 450-454, 1995.
16. See, for example, H.W. Jannasch, “Microbial Interactions with Hydrothermal Fluids,”pp. 273-296 in Seafloor Hy-drothermal Systems: Physical, Chemical, Biological, and Geological Interactions, Geophysical Monograph 91, Ameri-can Geophysical Union, Washington, D.C., 1995.
17. See, for example, T.O. Stevens and J.P. McKinley, “Lithoautotrophic Microbial Ecosystems in Deep Basalt Aqui-fers,”Science270: 450-454, 1995.
18. J. Oró and G. Holzer, “The Photolytic Degradation and Oxidation of Organic Compounds Under Simulated Martian Conditions,”Journal of Molecular Evolution14:153-160, 1979.
19. R.J. Cano and M.K. Boruncki, “Revival and Identification of Bacterial Spores in 25- to 40-Million-Year-Old Domini-can Amber,”Science268: 1060-1064, 1995.
20. C.F. Norton, T.J. McGenity, and W.D. Grant, “Archeal Halophiles (Halobacteria) from Two British Salt Mines,”Journal of General Microbiology139:1077-1081, 1993.
21. T.J. Beveridge, J.D. Meloche, W.S. Fyfe, and R.G.E. Murray, “Diagenesis of Metals Chemically Complexed to Bacteria: Laboratory Formation of Metal Phosphates, Sulfides, and Organic Condensates in Artificial Sediments,” Applied Environmental Microbiology45: 1094-1108, 1983.
22. F.G. Ferris, R.G. Wiese, and W.S. Fyfe, “Precipitation of Carbonate Minerals by Microorganisms: Implications for Silicate Weathering and the Global Carbon Dioxide Budget,”Geomicrobiology Journal12: 1-13, 1994.
23. G. Southam and T.J. Beveridge, “The In-Vitro Formation of Placer Gold by Bacteria,”Geochimica et CosmochimicaActa58: 4527-4530, 1994.
24. G. Southam, F.G. Ferris, and T.J. Beveridge, “Mineralized Bacterial Biofilms in Sulphide Tailings and in Acid Mine Drainage Systems,”pp. 148-170 in Microbial Biofilms, H.M. Lappinscott and J.W. Costerston (eds.), Cambridge University Press, Cambridge, U.K.
25. See, for example, B.M. Jakosky and R.M. Haberle,“The Seasonal Behavior of Water on Mars,”pp. 969-1016 in Mars, H.H. Kieffer, B.M. Jakosky, C.W. Snyder, and M.S. Matthews (eds.), University of Arizona Press, Tucson, 1992.
26. B.C. Clark and D.C. Van Hart, “The Salts of Mars,”Icarus45: 370-378, 1981.
27. M.H. Carr, Water on Mars, Oxford University Press, New York, 1996.
28. See also the committee’s reservations about the distribution of groundwater, in Chapter 6 of this report.
29. D.M. Hunten, “Possible Oxidant Sources in the Atmosphere and Surface of Mars,”Journal of Molecular Evolution14: 71-78, 1979.
30. K. Biemann, J. Oró, P. Toulmin III, L.E. Orgel, A.O. Nier, D.M. Anderson, P.G. Simmonds, D. Flory, A.V. Diaz, D.R. Rushneck, J.E. Biller, and A.L. Lafleur,“The Search for Organic Substances and Inorganic Volatile Compounds in the Surface of Mars,”Journal of Geophysical Research82: 4641-4658, 1977.
31. H.P. Klein, “The Viking Mission and the Search for Life on Mars,”Reviews of Geophysics and Space Physics17: 1655-1662, 1979.
32. See, for example, G.V. Levin, and P.A. Straat. “Viking Labeled Release Biology Experiment: Interim Results,”Science194: 1322-1329, 1976.
33. S.W. Squyres and J.F. Kasting, “Early Mars: How Warm and How Wet?”Science265: 774-779, 1994.
34. M.H. Carr, Water on Mars, Oxford University Press, New York, 1996.
35. G.R. Brakenridge, H.E. Newsom, and V.R. Baker, “Ancient Hot Springs on Mars: Origin and Paleoenvironmental Significance of Small Martian Valleys,”Geology13: 859-862, 1985.
36. R. Greeley and B.D. Schneid, “Magma Generation on Mars: Amounts, Rates, and Comparisons with Earth, Moon, and Venus,”Science254: 996-998, 1991.
37. M.H. Carr, Water on Mars, Oxford University Press, New York, 1996.
38. T.O. Stevens and J.P. McKinley, “Lithoautotrophic Microbial Ecosystems in Deep Basalt Aquifers,”Science270: 450-454, 1995.
39. T.O. Stevens, “Lithoautotrophy in the Subsurface,” in Proceedings of the Third International Symposium of Subsur-face Microbiology, Sept. 15-21, 1996, Davos, Switzerland, Swiss Society of Microbiology, Zurich, 1996.
40. See, for example, S. Kostelnikova and K. Pederson,“Ecology of Methanogenic Archea in Granitic Groundwater from Hard Rock Laboratory, Sweden,” in Proceedings of the Third International Symposium of Subsurface Microbiology, Sept. 15-21, 1996, Davos, Switzerland, Swiss Society of Microbiology, Zurich, 1996.
41. J.L. Gooding, “Soil Mineralogy and Chemistry on Mars: Possible Clues from Salts and Clays in SNC Meteorites, Icarus99: 28-41, 1992.
42. D.S. McKay, E.K. Gibson, Jr., K.L. Thomas-Keprta, H. Vali, C.S. Romanck, S.J. Clemett, X.D.F. Chillier, C.R. Macchling, and R.N. Zare, “Search for Past Life on Mars: Possible Relic Biogenic Activity in Martian Meteorite ALH84001,”Science273:924-930, 1996.
43. D.S. McKay, E.K. Gibson Jr., K.L. Thomas-Keprta, H. Vali, C.S. Romanck, S.J. Clemett, X.D.F. Chillier, C.R. Macchling, and R.N. Zare, “Search for Past Life on Mars: Possible Relic Biogenic Activity in Martian Meteorite ALH84001,”Science273:924-930, 1996.
44. A. Treiman, “On the Question of the Martian Meteorite: Recent Scientific Papers on ALH 84001 Explained, with Insightful and Totally Objective Commentaries,” available online at <http://cass.jsc.nasa.gov/lpi/meteorites/ alhnpap.html>, 2000.
45. Space Studies Board, National Research Council, 1990 Update to Strategy for Exploration of the Inner Planets, National Academy Press, Washington, D.C., 1990.
46. Space Studies Board, National Research Council, “Assessment of NASA’s Mars Exploration Architecture,”letter report to Carl Pilcher, NASA, November 11, 1998.
47. NASA, Mars Exploration Payload Assessment Group (MEPAG), “Mars Exploration Program: Scientific Goals, Objectives, Investigations, and Priorities,” December 2000, in Science Planning for Exploring Mars, JPL Publication 01-7, Jet Propulsion Laboratory, Pasadena, Calif., 2001.
48. Space Studies Board, National Research Council, Quarantine and Certification of Martian Samples, National Acad-emy Press, Washington, D.C., 2002.
49. Space Studies Board, National Research Council, Quarantine and Certification of Martian Samples, National Acad-emy Press, Washington, D.C., 2002.
50. Space Studies Board, National Research Council, “Assessment of NASA’s Mars Exploration Architecture,”letter report to Carl Pilcher, NASA, November 11, 1998.