1
Our Local Cosmic Laboratory
Plasma is the fourth state of matter and is ubiquitous in the universe. Plasmas pervade intergalactic space, interstellar space, interplanetary space, and the space environments of the planets. With the help of magnetic fields, plasma organizes itself into galactic jets, radio filaments, supernova bubbles, accretion disks, galactic winds, stellar winds, stellar coronas, sunspots, heliospheres, magnetospheres, and radiation belts. Magnetic fields partition space into tubes and shells of all sizes from galactic to planetary scales. Plasmas generate cosmic rays, stellar flares, coronal mass ejections, interstellar and interplanetary shock waves, magnetospheric storms, and a cacophony of radio waves. Plasmas absorb energy flowing steadily from the nuclear reactions within stars and from angular momentum shed by spinning magnetized bodies and release it explosively as x-rays and energetic particles. Structured, dynamic, and permeating apparently “empty” space, cosmic plasmas moderate energy flow across an enormous range of space and time scales.
Our local space environment—the heliosphere with its central star (the Sun) and orbiting planets—provides examples of many of the structures and processes that cosmic plasmas exhibit. Because of its accessibility to space probes, it is a local laboratory for in situ astrophysical plasma research. Eugene Parker has noted: “The little piece of cosmic real estate that we call our own, or can probe with spacecraft, is the most important corner of the universe for astronomical research.”1 The discipline of solar and space physics concentrates on understanding the local space environment. This report examines some of the universal properties of cosmic plasmas that have been identified from the unique knowledge base provided by nearly a half century of solar and space physics research. This general scientific understanding of the complex dynamics of magnetized plasmas forms the basis for extrapolation to remote astrophysical plasma systems, inaccessible to direct study.
From the perspective of pure science, plasma astrophysics offers the deep intellectual challenge of understanding the universe as a collection of self-organized, multiscale, coupled systems of space plasma structures and processes. Phenomena unpredictable by analytical theory emerge from such complex systems. For example, Richard Feynman notes: “Our equations for the sun … as a ball of hydrogen gas, describe a sun without sunspots, without the rice-grain structure of the surface, without prominences, without coronas.”2 Eugene Parker could predict the solar wind and the spiral magnetic field, but after
decades of observations no one has predicted stellar flares or storms within magnetospheres. Without measurements within our local cosmic laboratory, we still would be oblivious of coronal mass ejections (CMEs), the most powerful local manifestations of cosmic storms. The CME epitomizes the dynamics of cosmic plasmas—a burst of energy on a global (heliospheric) scale drives convective (magnetospheric) motions on a macroscale. These motions, in turn, induce flow shears on a mesoscale (magnetotail) that stretch and stress magnetic fields that finally snap on a microscale (local reconnection) owing to instability. The snap initiates an explosion that triggers powerful energy release on every scale. Plasma processes throughout the universe are, by and large, variations on this theme.
During more than 40 years of progress marked by probes of geospace, visits to all our solar system planets but one and to six moons, three comets, and two asteroids, and spacecraft sailing to the edge of the heliosphere, the field of solar and space physics has observed and analyzed the many forms taken by magnetized plasma in the solar system. By documenting the particular attributes and behavior of solar system plasmas, the field of solar and space physics has been conducting fundamental plasma science within a unique natural laboratory—one in which plasma-physical phenomena can be studied in situ and without the limitations to which experiments in ground-based laboratories are subject. Sufficient knowledge has been amassed during the past four-plus decades that the study of fundamental plasma processes within our local cosmic laboratory is now considered an essential component of solar and space physics. By investigating these plasmas as they manifest themselves in the spacecraft-accessible regions of the solar system, we can explore and understand the structures and dynamics of magnetized plasmas throughout the more distant cosmos.
CONTRIBUTIONS TO UNDERSTANDING COSMIC PLASMAS
To illustrate the potential of solar and space physics to benefit other fields, this section recounts contributions that such studies have already made. The discovery in the second half of the 19th century of a phenomenon that we now call solar flares gave the first hint that cosmic plasmas have a propensity for explosive energy release. Since then, this tendency has revealed itself whenever instruments with new eyes have looked, making sudden energy release in the cosmos a central theme in space physics and plasma astrophysics. The deep mystery of how the Sun influences the geomagnetic field—an influence Lord Kelvin dismissed as “a mere coincidence” but Sir John Herschel lauded as presaging “a vast cosmical discovery such as nothing hitherto imagined can compare with”—led a century later to the prediction of the solar wind.3 Confirmation and generalization to stellar winds soon followed.
Solar and space physics has given science the concept of magnetospheres and the first viable model of a magnetic dynamo that can generate planetary, stellar, and galactic magnetic fields. In less than 20 years, dedicated space physics missions and modeling brought the subject of collisionless shocks from an oxymoron to one of the most sophisticated examples of data-theory closure in science. Collisionless shock theory has been applied to the study of particle acceleration in both space and astrophysical plasma regimes, leading to a deep understanding of the way in which solar energetic particles and anomalous and galactic cosmic rays are accelerated.
The study of what happens when the solar wind encounters the local interstellar medium (LISM) has given rise to the concept of the heliosphere, the region of space dominated by the solar wind and the interplanetary magnetic field. Although spacecraft have yet to reach the boundaries of this region, remote sensing observations have detected radio emissions from just beyond the collisionless shock formed by the solar wind’s encounter with the LISM and have revealed the existence of a “wall” of interstellar hydrogen just upstream of the heliosphere. Loosely speaking, as the LISM flows around the heliosphere, interstellar neutral hydrogen piles up, forming a wall-like structure at the nose of the heliosphere. The concept of such
a wall of interstellar material now drives research programs to look for interstellar hydrogen walls around other stars, several of which have been reported.
Cosmic plasmas emit radio waves that furnish the means to detect these plasmas from Earth. Studies by space physicists of auroral kilometric radiation provide a terrestrial example of how the coupling of in situ observations and theory has led to a detailed understanding of the electron-cyclotron maser instability, a wonderfully efficient mechanism for moving energy from particle motions into radio waves. This theory is finding wide application in interpreting emissions from all magnetized outer planets (in particular, Jupiter), impulsive solar flares, binary stellar systems, and flare stars.
A last example of contributions by solar and space physics that have wide application is magnetic reconnection, perhaps the most universally invoked concept in studies of cosmic plasmas. The theory of magnetic reconnection has recently joined the ranks of long-standing, tough problems that are well on the way toward satisfactory solution. Cracking the problem entails identifying which mechanisms from a large field of candidates are important, and then understanding the coupling between disparate mechanisms that operate on widely separated spatial scales.
THE IMPORTANCE OF MAGNETIC FIELDS IN THE UNIVERSE
A key to understanding cosmic plasmas is the role that magnetic fields play in their dynamics and structure. Magnetic fields can act as a source of pressure and can interact with plasmas to cause expansion (e.g., stellar winds and jets). The presence of magnetic fields often causes the motion of the plasma to be turbulent (e.g., in the solar wind, galactic radio jets, and Earth’s magnetotail). In magnetized plasmas, magnetic energy is often explosively converted into particle kinetic energy (e.g., stellar flares and magnetospheric substorms). In many plasma regimes, the magnetic fields structure and organize the plasma. Magnetically structured matter tends to define shells, tubes, and sheets (e.g., radiation belts, flux ropes, and current sheets). The solar system serves as a local laboratory for the study of such universal properties of astrophysical plasmas.
LOCAL PLASMA ASTROPHYSICS
Astronomy and astrophysics are sciences that have mature aspects (e.g., many objects observed in the optical regime) as well as discovery-mode aspects (e.g., observations in new wavelength regimes that reveal fundamentally new phenomena). Plasma astrophysics, as practiced in the local solar system laboratory, that is, space plasma physics, is relatively mature. As a science, space plasma physics is moving beyond the initial discovery phase to one in which detailed understanding of the physics is being sought.
Much of what we have learned about the behavior of plasmas in space can be thematically organized in the following universal categories:
-
Creation and annihilation of magnetic fields,
-
Formation of structures and transients,
-
Plasma interactions,
-
Explosive energy conversion, and
-
Energetic particle acceleration.
These categories form the basis for the discussion in the chapters that follow. Figure 1.1 shows these topics and their contents as far as researchers have identified them.
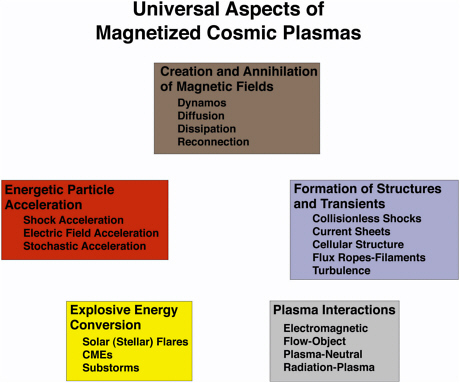
FIGURE 1.1 Five fundamental behaviors characteristic of magnetized cosmic plasmas.
The top box in Figure 1.1 is “Creation and Annihilation of Magnetic Fields.” Cosmic magnetic fields result from an ever-evolving competition between creation by magnetic dynamos and destruction involving one or more of the following processes: diffusion, dissipation, and magnetic reconnection. Dynamos are evident on the Sun and within most planets (Mercury, Earth, evidently early Mars, and the giant planets) and within at least one moon (Ganymede). With respect to annihilation, magnetic reconnection deserves special mention because it is universal in two senses. First, it likely occurs wherever dynamos create magnetic fields—almost everywhere in the universe. Second, magnetic reconnection plays a central role in solar flares, coronal mass ejections, and the dynamics of magnetospheres.
Next in Figure 1.1 (moving clockwise) is the category “Formation of Structures and Transients.” Collisionless shocks are ubiquitous in cosmic plasmas (e.g., planetary bow shocks, CME-driven interplan-
etary shocks, interstellar shocks associated with supernova remnants) and are important sites of particle acceleration. Shocks are created when the relative velocity between plasma regimes creates sharp transitions. Magnetism in plasmas spontaneously generates current sheets (e.g., the heliospheric current sheet and the magnetotail current sheet), cellular structures (e.g., coronal arcades and magnetospheres), flux ropes or filaments (e.g., plasmoids and sunspots), and turbulence (e.g., solar wind fluctuations and bursty bulk flows). The generation of filaments and flux ropes results from differential flows that stretch magnetic fields, which then, through instability or reconnection, segregate into coherent tubes of fixed flux. Current sheets spontaneously form whenever and wherever magnetized plasmas of different origins meet. They also spontaneously form when random velocity fields shuffle and twist field lines (such as in the Sun’s photosphere).
Next in the circuit of Figure 1.1 is the category “Plasma Interactions.” Plasmas interact with other plasmas and also with matter not in the plasma state. The solar wind interacts with planetary magnetospheres as well as with the ionospheres and neutral atmospheres of unmagnetized bodies such as Venus and comets. Planetary ionospheres and magnetospheres interact, with important consequences for both plasma regimes, as a result of their coupling by magnetic-field-aligned currents. Ionospheric plasmas interact collisionally with the neutral gases of planetary upper atmospheres, resulting in a mutual exchange of energy and momentum. Plasma interactions thus take a variety of forms and involve a number of different physical processes.
The next box in Figure 1.1 is “Explosive Energy Conversion,” with examples of solar flares, CMEs, and substorms. The entry “solar flares” covers a hierarchy of phenomena from nanoflares, unresolvable by telescope, to importance-4, X-class bursts, visible to shielded but otherwise unaided eyes. The process called substorms at Earth appears to have analogues at Mercury and Jupiter. Explosive energy conversion occurs when magnetic energy builds slowly through stretching by differential flows and is released suddenly by one or more modes of instability. A key element is the role of magnetic reconnection in these processes—the merging of magnetic field lines is an efficient mechanism for generation of plasma flows and energy release. An important issue is whether differential flows that build magnetic energy or modes of instability that suddenly release it have properties in common. Is there a unified framework from which to understand explosive energy conversion as a manifestation of one or a few processes in different contexts? Or is each instance a case unto itself? This issue can be restated for nearly each example in Figure 1.1.
The remaining box in Figure 1.1 lists “Energetic Particle Acceleration” as a universal characteristic of magnetized plasmas. Solar system examples of energetic particle acceleration include anomalous cosmic rays, solar energetic particles, and radiation belts at Earth, Jupiter, Saturn, Uranus, and Neptune. The standing shocks of planetary magnetospheres, shocks associated with corotating interaction regions, and interplanetary shocks driven by CMEs all accelerate particles. The primary acceleration mechanism associated with shocks is known as Fermi acceleration, which results from the repeated passage of charged particles back and forth across the shock as they are reflected between the upstream and downstream plasma. Electric fields play a central role in the acceleration of charged particles in magnetized plasmas. These electric fields can be produced by time-varying magnetic fields (Faraday’s law of magnetic induction), by charge-separation, and by the dissipation of Alfvén waves in planetary ionospheres. Coherent electric field acceleration is responsible, for example, for the acceleration of particles in solar flares, in Earth’s magnetotail during magnetospheric disturbances, and in the auroral magnetosphere. Particle acceleration can also result from the action of plasma waves or turbulence (stochastic acceleration).
The intersection between space physics and plasma astrophysics provides fertile ground for the transfer of knowledge and generalization of specific, local cases to a much broader range of physical understanding
of plasma processes in the universe.4 As the chapters that follow demonstrate, there is a wide range of work that can now be used for continuing the evolution toward a closer relationship between space plasma physics and plasma astrophysics.