5
Water Quality Improvement: Decision-Making Strategies and Technical Solutions
INTRODUCTION
This chapter explores various wastewater management techniques and recommends actions to address water quality problems—especially wet weather-related problems—in southwestern Pennsylvania. As discussed in Chapters 3 and 4, the aquatic environment of southwestern Pennsylvania is impaired for a variety of designated beneficial uses including recreational use due to the likelihood of waterborne pathogens in surface waters and aquatic life use due to acid mine drainage (AMD). Although AMD is a significant cause of water quality impairment in the region, especially in the predominantly rural counties of southwestern Pennsylvania, this problem is most appropriately addressed at the state and federal levels. Continued funding for these ongoing efforts (see Chapter 4 for further information) is essential to improve water quality in southwestern Pennsylvania.
A fundamental prerequisite to the formulation of cost-effective plans for reducing water quality impairments in southwestern Pennsylvania is a systematic and extensive set of water quality data covering both sources of impairments and in-stream responses. The data should be sufficient to accurately assess the different sources of contamination and their impacts on receiving streams. Toward this end, Chapter 3 summarizes data available for the region. Increased monitoring by different groups and agencies has taken place over the past several years, and the available data are sufficient to conclude that serious water quality problems exist in southwestern Pennsylvania. However, there are not sufficient data to determine the relative seriousness of the related environmental and human health problems, the relative importance of the potential sources of contamination, and the improvements that are likely to result from alternative pollution control measures.
Despite these limitations, the Allegheny County Sanitary Authority (ALCOSAN) and its member communities are now facing enforcement action by the U.S. Environmental Protection Agency (EPA) through the Pennsylvania Department of Environmental Protection (PADEP) and the Allegheny County Health Department (ACHD) (see more below) for violations of the Clean Water Act related to combined sewer overflows (CSOs) and sanitary sewer overflows (SSOs). Thus, remedial actions are anticipated that will alter the relative contribution of different sources to the water quality problems in the region. It will be critical that evaluation of water quality improvements related to these activities be undertaken. Further, the implementation of solutions for identified sources of impairment does not preclude the need for additional information related to other sources and their contributions to water quality impairment. Monitoring and modeling efforts should proceed in conjunction with, and inform decisions with respect to, a variety of mandated water quality improvements currently being pursued (e.g., those listed in Table 5-7).
Several entities (ALCOSAN, 1999; TPRC, 2002; WSIP, 2002) have estimated recently that addressing the region’s CSO and SSO problems by conventional means, using a combination of storage, conveyance, and treatment improvements could cost several billion dollars. Although the problem of excessive discharge of untreated wastewater from CSOs and
SSOs is well documented, the data presented to the committee and those uncovered by its own research as summarized in Chapter 3 are inadequate to arrive at a definitive conclusion as to (1) the impact of these discharges on water quality in receiving streams and (2) what should be done to address the issue in the context of federal CSO policy.
Investing large sums of capital based only on currently available data may not ultimately solve the most important problems or provide appropriate solutions. Although it is true that no amount of additional data and analyses would remove all uncertainty about water quality investments, it is clear that currently available information is lacking in several critical areas, including the following:
-
the nature and magnitude of CSO effects on receiving streams during wet weather events;
-
whether effects are limited to indicator microorganisms (i.e., bacterial indicators of fecal contamination and, indirectly, the presence and quantity of fecal pathogens) and the extent to which they include floatable and settleable solids;
-
how much surface water runoff from separate stormwater conveyances affects water quality in receiving streams during wet weather events;
-
whether present discharges constitute a threat to the public as evidenced by health data; and
-
the extent of the effects of present and potential small community and on-site systems.
The causes and nature of water quality impairments, the parties responsible, and the individuals and waterways affected differ for each of the problem contaminants in the region. A comprehensive watershed-based approach is needed to address the spectrum of water quality problems; such a systematic approach should recognize interrelationships among problems and the need for the parties responsible for each water quality problem to share in its solution. Responsible groups may be the public at large, a segment of the population, individuals, or a particular industry or group of industries. Recognition of payment capacity of individuals and the region as a whole should also be considered in reaching equitable solutions (see Chapter 6 for further information).
EXISTING INFRASTRUCTURE AND INSTITUTIONS FOR WATER QUALITY MANAGEMENT
The Commonwealth of Pennsylvania and local governments in the Pittsburgh region have a long history of planning, regulations, capital investments, and development of managerial expertise to control water pollution. It is evident that more is needed, particularly in the management of CSOs, SSOs, separate storm sewers, and other sources of pollution. Future actions will build on or modify existing infrastructure and managerial institutions. Some of those facilities and arrangements are discussed in the sections that follow.
Sewer systems that convey wastewater or combined wastewater and stormwater to sewage treatment plants generally have multiple components and multiple owners. First, pipes within a residence collect wastewater and carry it to a house lateral pipe (see Figure 4-5). House lateral pipes are underground and owned by the homeowners. Laterals typically comprise 50 percent of the total length of pipe in a sewer system and are connected to a street sewer pipe; as a general rule, they may account for a substantial portion of the total infiltration and inflow into
the sewer system. Because a large portion of them are located on private property, and measuring flows in laterals is not common practice, identifying sources and fixing them requires special detective work and authority to order corrective measures. Sewer pipes are generally owned by the municipality that owns the street above it. This street sewer pipe joins with others and enters an interceptor. This larger pipe might be owned by a municipality, a sewer authority, or the same authority or organization that owns a wastewater treatment plant (WWTP). Finally, major interceptors carry the flow from all the small interceptors and pipes to the treatment plant. Major interceptors are generally owned by the same organization that owns and operates the treatment plant. These complexities are compounded in southwestern Pennsylvania because of fragmented ownership and responsibilities.
The Pennsylvania Sewage Facilities Act, commonly referred to as Act 537, was passed in 1966. It requires all municipalities to formulate and implement plans for management of current and future sewage. Individual municipalities may choose to administer their own plans, or they may choose to participate in a joint local agency (JLA) with other municipalities or county health department. These plans, subject to review and approval by PADEP, must be modified when new land development is proposed or other changes occur.
By far the largest JLA in the region is ALCOSAN (see Chapter 2 for further information). ALCOSAN owns and operates 83 miles of major interceptors and a wastewater treatment plant that provides primary and secondary treatment of up to 225 million gallons per day. Eighty-three communities are within the ALCOSAN service area (see Figure 1-1), and a total of 12 sewer authorities serve many of these communities. Although these “partner communities” own and operate their own sewer collection infrastructure (street sewer pipes and smaller interceptors), they do not operate WWTPs. All collected sewage (and stormwater flow where combined systems are used, unless released during a CSO event) is sent to the ALCOSAN interceptors and eventually to the central wastewater treatment plant. Thus, ownership and management of wastewater collection and treatment facilities for the most populous county in the region is extremely fragmented. The institutional complexities of the region are discussed more fully in Chapter 6.
This complexity is illustrated by the fact that as of March 2004, there were 591 Act 537 plans in the 11-county area. They are summarized by county in Table 5-1. Any development requiring the extension of sewer systems not included in an existing plan will trigger a plan revision. Twenty-eight percent of the plans have been revised in the past five years. Many of the plans cover rural areas in which no sewer systems are located or very little development is occurring. Indeed, more than half of the municipalities have had no reason to revise their plans for 20 years or more. A map of the sewered areas is provided in Figure 5-1. Act 537 also required municipalities to establish a permitting program for on-lot treatment and disposal systems (OLDS; referred to as on-site sewage treatment and disposal systems [OSTDS] in this report) for individual lots and community OSTDSs with design flows of up to 10,000 gallons per day. Like the sewage disposal plans, these programs are to be operated by individual municipalities or through JLAs. Each program is administered by a sewage enforcement officer (SEO) who is trained by PADEP. SEOs are responsible for determining the adequacy of sites to support OSTDSs and ensuring that system designs comply with Chapter 73 of PADEP’s regulations. The PADEP has developed a home buyers’ guide, a homeowners’ guide to maintenance, a manual to SEO’s decisions about repairs, and other educational material that is
TABLE 5-1 Act 537 Plans by County by Age of Plan
County |
Age of Plan (years) |
||||
<5 |
5-9 |
10-19 |
>20 |
All |
|
Allegheny |
75 |
9 |
5 |
41 |
130 |
Armstrong |
5 |
3 |
0 |
36 |
44 |
Beaver |
7 |
3 |
4 |
40 |
54 |
Butler |
8 |
7 |
4 |
28 |
47 |
Fayette |
13 |
2 |
4 |
24 |
43 |
Greene |
6 |
1 |
0 |
19 |
26 |
Indiana |
15 |
8 |
4 |
11 |
38 |
Lawrence |
7 |
2 |
2 |
16 |
27 |
Somerset |
5 |
1 |
6 |
38 |
50 |
Washington |
14 |
7 |
8 |
38 |
67 |
Westmoreland |
9 |
7 |
6 |
43 |
65 |
Total |
164 |
50 |
43 |
334 |
591 |
SOURCE: Data from www.dep.state.pa.us/dep/deputate/watermgt/Wqp/WQP_WM/537Map/. |
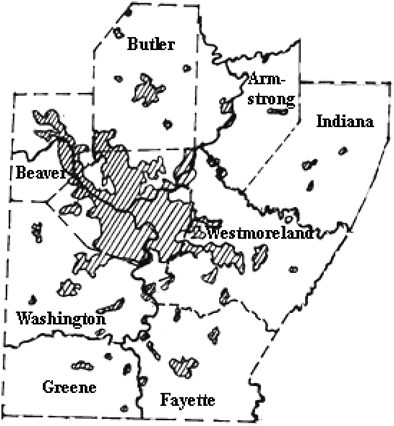
FIGURE 5-1 Approximate boundaries of sewered areas in Allegheny and surrounding counties.
NOTE: Lawrence and Somerset Counties of southwestern Pennsylvania (see also Box 1-2) are not included.
SOURCE: Adapted from SPC map, “Sewer Service Areas in the SPC Nine County Region.”
available on-line,1 but once a system is permitted, there is no program for regular inspection and maintenance. Pennsylvania’s stormwater program operates under authority of the Storm Water Management Act of 1978 (Act 167) and EPA regulations under the 1987 amendments to the Clean Water Act. Act 167 provides grants to counties to prepare stormwater regulations for designated watersheds, although initiation of planning is left to the counties. A county desiring to develop a plan then makes a proposal to PADEP for financial assistance. A requirement of the plan is preparation of a model stormwater ordinance, which must be adopted by any municipality within the watershed that does not have equivalent language in its building subdivision or land development codes. The ordinances address control of stormwater runoff from new development; they do not require retrofitting of existing development.
A recent map of Pennsylvania watersheds for which stormwater plans have been prepared2 shows that only six plans have been prepared for the approximately 60 watersheds within the 11-county area of southwestern Pennsylvania. Approved plans and dates of approval include the following:
-
Pine Creek, Girty Run, and Deer Creek in Allegheny County (1986);
-
Turtle Creek in Allegheny and Westmoreland Counties (1991 and 1992);
-
Montour Run in Allegheny County (1990); and
-
Monongahela River in Allegheny County (1990).
Two other plans are in preparation, one for Little Sewickley Creek in Allegheny County and one for Cokes Creek in Somerset County. The original Turtle Creek plan was prepared in 1986; its purpose was to reduce the impact of new development on peak discharges and related downstream effects on property and traffic. The plan established standards for post-development peak discharge rates from new development. Standards ranged from pre-development rates to 50 percent of post-development rates, depending on location of development within the watershed. The model ordinance affected about 30 municipalities in the watershed (John Maslanik, ATS-Chester Engineers, personal communication, 2004).
Amendments to the Clean Water Act in 1987 directed the EPA to expand the National Pollutant Discharge Elimination System (NPDES) permit system to include stormwater runoff. The EPA implemented that directive in two phases. The Phase I Rule was published on November 16, 1990 (55 Federal Register 47990) and required all operators of medium and large municipal separate storm sewer systems to obtain an NPDES permit and develop a management program to reduce the discharge of harmful pollutants. These regulations covered certain categories of stormwater associated with industrial activity and discharges of stormwater from urban areas with a population of 100,000 or more (EPA, 1996). Notably, Phase I included Allegheny County.
The EPA promulgated rules specifying who must apply for Phase II permits in August 1995; final Phase II rules were published in 1999 (EPA, 1999). That program automatically covers all municipal separate storm sewer systems located in an urbanized area as defined by the U.S. Bureau of the Census. A few special waivers apply, and small municipal separate storm
1 |
See http://www.dep.state.pa.us, “Subjects,” “Wastewater” for further information. |
2 |
Map available on-line at http://www.dep.state.pa.us/dep/deputate/watermgt/wc/subjects/stormwatermanagement/Stormwater_11_18_02_web.jpg. |
sewer systems outside urban areas may be waived by a permitting agency on a case-by-case basis.
In December 2002, PADEP updated its stormwater policy to include protection of water quality as mandated by the Phase I and Phase II rules (PADEP, 2002). The federal regulations for Phase II require six minimum measures:
-
public involvement in planning and decision processes;
-
detection and elimination of illicit discharges to storm sewers;
-
reduction of pollutants in stormwater runoff from construction sites;
-
management of post-construction runoff from new development and redevelopment;
-
pollution prevention and good housekeeping; and
-
public education and outreach.
The PADEP has developed several guidance documents to assist communities in achieving compliance with federal regulations, including a handbook of best management practices (BMPs) for developing areas3 and a model stormwater ordinance for municipalities (PADEP, 2002). The revised policy establishes post-construction management requirements that emphasize groundwater infiltration and BMPs to control volumes and rates of discharge. The policy sought to integrate those permits with its Act 167 authorities. Acceptable BMPs to promote groundwater infiltration, water quality, and rate and volume control are listed in Table 5-2.
The primary limitation of all of these stormwater programs is that they are focused mostly on controlling runoff from new development. They do cover illicit connections to storm sewers and redevelopment projects, but for the most part they have little effect on high density development in the urban core of Pittsburgh and surrounding communities that were developed prior to enactment of these programs.
DRIVERS FOR WATER QUALITY IMPROVEMENT UNDER WET WEATHER CONDITIONS
As discussed in Chapter 4, although the relative contribution of different sources of microbial loading to surface and groundwaters in southwestern Pennsylvania cannot be determined with available information, sufficient information is available to determine that improperly managed wastewaters resulting from human activities are degrading the microbiological water quality in the region. Furthermore, available water quality and human health data are insufficient to reach sound conclusions about the seriousness of this problem. Wet weather microbiological water quality in the main stem rivers is demonstrably worse than dry weather quality, suggesting that stormwater and sewer overflows may be important contributors. In this regard, bacterial (predominantly coliform) indicator levels greatly exceed acceptable standards for body contact recreation for a significant portion of the year in the three main stem rivers and many of their tributaries, especially during and immediately after precipitation events. This is a particular issue in and around the City of Pittsburgh that is probably exacerbated by upstream and downstream sources of microbial loading, including agricultural runoff. Microbiological water quality in many tributaries does not meet standards in
3 |
Available on-line at http://www.pacd.org/products/bmp/bmp_handbook.htm. |
TABLE 5-2 Best Management Practices Deemed Acceptable to PADEP for Stated Purposes
Groundwater Infiltration |
Water Quality |
Rate and Volume Control |
Permeable paving |
Permeable paving |
Permeable paving |
Grass swale |
Bioretention |
Stormwater infiltration |
Bioretention |
Grass swale |
Dry ponds |
|
Filter strip |
Stormwater wetlands |
|
Wet pond (extended detention pond) |
Wet ponds (extended detention pond) |
|
Rooftop runoff management |
Rooftop runoff management |
|
|
Bioretention |
SOURCE: Adapted from www.dep.state.pa.us (“Stormwater”). |
either wet or dry weather, suggesting that failing OSTDSs and sewers may be important contributors.
Notwithstanding the data limitations, the most pressing water quality problem in the region from a regulatory perspective is caused by CSOs, SSOs, and stormwater drainage resulting from wet weather conditions. In fact, the EPA views CSO and SSO problems as sufficiently serious that it has promulgated regulations requiring CSO, SSO, and stormwater controls. Because southwestern Pennsylvania has one of the nation’s most extensive CSO and SSO control problems, EPA, acting through PADEP and ACHD, has issued a series of administrative consent orders to many of the communities served by ALCOSAN (see also footnote 1, Chapter 1) to address this problem. It is important to state that the ALCOSAN draft consent decree with EPA remained attorney-client privileged4 as this report neared completion in December 2004 so the committee’s conclusions and recommendations may not be consistent with whatever actions result from the final legal agreements that may be reached. Thus, the following discussion concerning CSO and SSO problems should be viewed with this caution in mind. However, the consent orders for controlling CSOs and SSOs in the ALCOSAN partner communities have become publicly available during the study period, and many of the committee’s recommendations are aligned with these activities and are discussed later in this chapter.
EPA’s Regulatory Approach to CSO Remediation
EPA has produced a variety of guidance for municipalities to manage CSOs, including the following:
-
Combined Sewer Overflow Policy (EPA, 1994)
-
Combined Sewer Overflows Guidance for Long-Term Control Plan (EPA, 1995a)
-
Combined Sewer Overflows Guidance for Nine Minimum Control Measures (EPA, 1995b)
-
Combined Sewer Overflows Guidance for Funding Options (EPA, 1995c)
-
Combined Sewer Overflows Screening and Ranking Guidance (EPA, 1995d)
-
Combined Sewer Overflows Guidance for Financial Capability Assessment and Schedule Development (EPA, 1997)
-
Combined Sewer Overflows Guidance for Monitoring and Modeling (EPA, 1999)
-
Guidance: Coordinating Combined Sewer Overflows (CSO) Long-Term Planning with Water Quality Standards Reviews (EPA, 2001b)
Owners of CSOs are required to obtain discharge permits and establish programs that would implement nine minimum control measures (discussed below), and each is required to develop and implement a long-term control plan (LTCP) for controlling CSOs. The 1994 EPA CSO policy states:
EPA expects a permittee’s long-term CSO control plan to give the highest priority to controlling overflows to sensitive areas. Sensitive areas, as determined by the NPDES authority in coordination with state and federal agencies, as appropriate, include designated Outstanding National Resource Waters, National Marine Sanctuaries, waters with threatened or endangered species and their habitat, waters with primary contact recreation, public drinking water intakes or their designated protection areas, and shellfish beds.
In Pittsburgh’s case, the sensitive areas are those waters below CSOs with primary contact recreation as their designated use and those that serve as public drinking water intakes (see Figure 4-4).
There are two basic remedial approaches for controlling CSOs: (1) the demonstration approach and (2) the presumption approach. In brief, the “demonstration approach” relies on data collection and simulation to demonstrate that a proposed management strategy will result in meeting water quality standards and considers all factors that are likely to influence success; there is no reliance on criteria governing by how much CSOs may be reduced. The demonstration approach seems inherently advantageous because it relies on actual data collection and analysis and also has the benefit of lending itself to adaptive implementation (described later) by determining the progressive performance, in the watershed context, of each measure undertaken. Although the demonstration approach has the advantage of focusing investment on measures likely to achieve water quality standards, because it relies on time-consuming data collection and analysis, it could result in delaying the reduction of pollutants to receiving streams.
In contrast, the “presumption approach” presumes that meeting certain criteria, including a statistical reduction (85 percent) of the annual volume of wet weather overflows, is likely to result in meeting water quality standards as reasonably determined by the regulatory agency. Under this approach, if the owner of CSOs has satisfied given criteria for reducing CSOs and some uncertainty remains about satisfaction of water quality standards, the owner is given the presumption that CSOs are no longer contributing to noncompliance with water quality standards. Satisfaction of the criteria for reducing CSOs usually involves large capital investments. The presumption approach was included as an alternative by EPA in the 1994 CSO policy because data and modeling of wet weather events do not always provide a clear picture of the level of CSO controls that are necessary to meet water quality standards.
More specifically, EPA (1994) noted that “because data and modeling of wet weather events often do not give a clear understanding of the level of CSO controls necessary to protect water quality standards, one of three technology and performance standards could be used to
satisfy a presumption that water quality standards would be met.” The criteria include the following:
-
a limit on the number of overflow events per year;
-
elimination or the capture for treatment of no less than 85 percent by volume of the CSO discharge; or
-
elimination or removal of no less than the mass of the pollutants identified as causing water quality impairment through the sewer system.
In later guidance, EPA (1999) added some clarification to its CSO control policy:
Because CSOs are subject to the technology-based requirements of the Clean Water Act (CWA), permitting authorities must specifically determine best available technology economically achievable (BAT)/best conventional pollutant control technology (BCT) on a case-by-case basis using best professional judgment (BPJ) during the permitting process [emphasis added]…Therefore, evaluation of CSO controls beyond the nine minimum controls may appropriately focus primarily on water quality issues…State and Federal NPDES authorities must coordinate throughout the planning process to ensure that after implementation of the controls in the proposed LTCP, CSOs will not cause or contribute to nonattainment of WQS.
The CSO policy is clear—attainment of water quality standards is a requirement. However, the policy also recognizes that an unambiguous determination of what constitutes compliance with water quality standards may not be possible. The policy appears to indicate that when there is significant uncertainty about whether a plan will lead to compliance with water quality standards, the permittee is entitled to the presumption approach if the plan satisfies one of the three aforementioned criteria.
As discussed in the next section, ALCOSAN proposed to rely primarily on the 85 percent reduction criterion as described in its March 1999 report Draft Combined Sewer Overflow Program Phase I Activity Report: Regional Long Term Wet Weather Control Concept Plan (ALCOSAN, 1999, pp. 1-2, 3-1). An independent third party review (TPR) of ALCOSAN’s draft LTCP has cast serious doubts on whether the 85 percent reduction criterion would satisfy water quality standards. That analysis itself was based on several reasonable but unverifiable assumptions that are discussed in the TPR report (TPRC, 2002). Until the fundamental gaps in knowledge of regional water quality are filled (see Chapters 3 and 4 for further information), it remains unclear whether achieving an 85 percent reduction in CSO volume would satisfy water quality standards.
Use of the demonstration approach for controlling CSOs places a heavy burden of proof on the region to demonstrate that a particular control plan will satisfy water quality standards. As discussed later in this chapter, it is difficult with complex models to get an adequate estimate of uncertainty or to know precisely when a satisfactory “demonstration” has been achieved. Results of water quality modeling must be combined with substantial professional judgment in making a determination about compliance.
The demonstration approach for controlling CSOs can be used in southwestern Pennsylvania by incorporating a strategy of adaptive implementation, which is discussed in detail later in this chapter. In brief, it begins with monitoring actual CSO discharges and their water quality impacts. Field monitoring should be coupled with water quality models that enable
planners to estimate the extent to which reductions in discharges will be necessary to meet water quality standards. Because effects of CSOs and discharges from separate storm sewers are intermingled in the region’s primary receiving streams, monitoring and modeling of CSOs and their impacts on streams during wet weather events should occur simultaneously with monitoring and modeling of separate stormwater sewer systems during wet weather events. Conventional control strategies for reducing pollutant loading from CSOs should be conducted in parallel with experiments on innovative but unproven technology such as vortex separators (see more below). All of these investigations can be conducted over a relatively short period (e.g., three to five years). Upon completion, information available at that time should be used to help judge which CSO control strategies are cost-effective and subject to acceptable levels of uncertainty.
ALCOSAN’s Long Term Wet Weather Control Concept Plan
To address the EPA and Commonwealth of Pennsylvania wet weather regulatory requirements, in March 1999 ALCOSAN produced a draft LTCP; its fundamental goal “is to improve and preserve the water environment in the ALCOSAN service area and to fulfill ALCOSAN’s obligations under the Clean Water Act and the Pennsylvania Clean Streams Law.”
More specifically, the draft LTCP (ALCOSAN, 1999, p. 1-1) has three primary phases to attain wet weather water quality standards that are summarized below:
-
implement a program for nine minimum CSO controls;
-
plan, design, and implement a regional LTCP; and
-
participate in regional and interstate watershed-based planning and analyses.
Phase One—Nine Minimum Combined Sewer Overflow Controls
The EPA’s nine minimum controls5 for CSOs do not require significant engineering studies or major construction and can be implemented in a relatively short time frame (EPA, 1995b). These include (1) proper operation and maintenance of the sewer system, (2) maximum use of the collection system for storage, (3) modification of the pretreatment program, (4) maximization of wastewater flow to the treatment plant, (5) elimination of chronic dry weather overflows, (6) control of solids and floatables, (7) pollution prevention, (8) public notification of overflow occurrences and impacts, (9) and monitoring to characterize sewer overflow impacts.
Phase Two—LTCP
According to the draft LTCP, ALCOSAN (1999, p. 1-2, 3-1) proposed to use the presumption approach guidance as outlined by the EPA to address its CSO problem. This approach permits meeting regulatory requirements by the indirect method of reducing the amount of combined sewage overflow and presuming that this action will meet water quality standards. In brief, the presumption approach as outlined in the draft LTCP includes expanding
5 |
For further information on EPA’s nine minimum controls for CSOs, see http://cfpub.epa.gov/npdes/cso/ninecontrols.cfm?program_id=5. |
the existing ALCOSAN wastewater treatment plant over a 20-year period from the current 225 million gallons per day (mgd) to a total wet weather capacity of 875 mgd and also bringing about infrastructure changes to the sewerage system in the form of interceptors to significantly increase the proportion of wet weather flows arriving at the treatment plant instead of discharging untreated into streams. Of this increased flow, 310 mgd would receive full secondary treatment and the remaining 565 mgd of wet weather flow would receive primary treatment and disinfection only. ALCOSAN estimates that these proposed changes will permit capture of 85 percent of the wet weather combined sewage flow—the majority of which will be given primary treatment only.
A major portion of the ALCOSAN interceptor sewer system roughly parallels the three major rivers in the Pittsburgh area, the Allegheny, Monongahela, and Ohio Rivers. Flows within the sewer system in excess of those planned for conveyance to the main treatment plant would undergo high-rate flow regulation and primary treatment by five of the system’s swirl (vortex) separators (see more below). The other major portion of the ALCOSAN interceptor sewer system roughly parallels four major tributary streams: Chartiers Creek, Saw Mill Run, Turtle Creek, and Thompson Run (see Figure 1-2). These interceptors are installed in relatively shallow excavations, and under the draft LTCP (ALCOSAN, 1999, pp. 1-2, 3-5) the excess wet weather flow in these interceptors would be handled differently from that of the main river interceptors. For these interceptors, up to 85 percent of the wet weather flow would be handled by a combination of interceptor upgrades, peak flow storage, and two of the system’s vortex separators.
A portion of ALCOSAN’s existing interceptor sewer system, built for the most part at the upper extensions of the main interceptors, is designed for collection of sanitary sewage only. Unfortunately, at the lower ends of the interceptors this sanitary sewage becomes mixed with combined sewage such that much of the benefit of the separate sewage infrastructure is lost. In addition, the wet weather flow in the separate sewers averages 1,000 gallons per capita per day (gpcd), while the dry weather flow averages only 190 gpcd (ALCOSAN, 1999). This indicates that an excessive amount of runoff from precipitation enters the separate sewage system, leading to separate system overflows, which are illegal under the federal Clean Water Act. According to the draft LTCP, ALCOSAN expects that member municipalities will commit to a long-term (approximately 50-year) effort to reduce this inflow and infiltration.
The estimated costs of the interceptor system and associated regulator/grit treatment upgrades under the draft LTCP are provided in Table 5-3. The total construction cost in 1998 dollars is approximately $922 million, with an annual operating cost of $3.51 million. However, this does not include the cost of implementing the previous (1996) ALCOSAN plans for upgrades to expand ALCOSAN’s plant to 875 mgd wet weather flow in accordance with the requirements of Pennsylvania Act 537. Total 2002 costs for the LTCP, expansion of its treatment plant ($210 million), and upgrades to the non-ALCOSAN-owned collection system ($1.9 billion) are expected to exceed $3 billion (TPRC, 2002).
In order for the LTCP to be successful, an extensive rehabilitation and/or reconstruction of the overall ALCOSAN sewerage system must ultimately be accomplished to reduce infiltration and inflow. However, ALCOSAN controls only a portion of the sewers and a portion of the total watershed area of the rivers and streams flowing into its service area; thus, the success of much of this effort will depend on action by other entities in the region. The long-
TABLE 5-3 Estimated Capital and Operations and Maintenance Costs for Interceptor and Nonplant Treatment Modifications
Planned Modifications |
Capital Costs ($ million) |
Operation and Maintenance Costs ($ million/year) |
Three Rivers Interceptors |
|
|
Allegheny |
146.7 |
0.512 |
Monongahela |
105.4 |
0.333 |
Ohio |
38.1 |
0.170 |
Subtotal |
334.1 |
1.965 |
Tributary Interceptors |
|
|
Saw Mill Run |
37.7 |
0.283 |
Thomson Creek/Turtle Creek |
121.5 |
0.71 |
Chartiers Creek |
366.0 |
0.145 |
Subtotal |
525.2 |
1.136 |
Other |
62.4 |
0.410 |
Totals (1998 dollars) |
921.7 |
3.513 |
Totals (2002 dollars) |
1,030.5 |
3.928 |
SOURCE: ALCOSAN, 1999. |
range reconstruction and rehabilitation of the collection systems by member municipalities is estimated to cost between $1.2 billion and $4.2 billion (in 1999 dollars) over a 50-year period (ALCOSAN, 1999).
Phase Three—Regional and Interstate Participation
Although the third phase of ALCOSAN’s LTCP was not discussed in detail in the March 1999 draft plan, that report does state (p. 1-2) that “As a stakeholder in the watershed planning process, ALCOSAN is willing to participate in regional and interstate watershed management activities.” The ALCOSAN third party review (TPRC, 2002) briefly describes the role of regional and interstate participation in the LTCP in that a “regional approach” is referred to in summaries of activities to be undertaken in Phases I, II, and III. The “regional approach,” however, appears to be limited to ALCOSAN, the 83 member communities served by ALCOSAN, the Three Rivers Wet Weather Demonstration Program (3RWW), and the regulatory community—those entities identified as the principal stakeholders. That wording suggests that this regional approach encompasses only Allegheny County, rather than southwestern Pennsylvania as a whole. Chapter 6 of this report addresses the roles of local, regional, state, and federal organizations in addressing water quality improvement in southwestern Pennsylvania and, consistent with this chapter, specifically recommends that a much larger watershed area be considered. Furthermore, Chapter 7 includes a discussion of several related issues that have broader implications beyond southwestern Pennsylvania.
Summary of Third Party Review of ALCOSAN’s Draft LTCP
Following the establishment of a Review Committee in 1998 and an Engineering Peer Review Committee in 1999, ALCOSAN formed a third party review committee (TPRC) in 2001
to address several questions raised by affected member communities concerning ALCOSAN’s draft LTCP (see also Appendix B). Toward this end, the TPRC, consisting of eight prominent residents of southwestern Pennsylvania, directed an independent assessment of the LTCP by a team of environmental engineering consultants (Greeley and Hansen LLC, HydroQual, Inc., and McGuire Woods, LLP). The collective result of their effort was a June 2002 report (TPRC, 2002; see also Appendix B) entitled Third Party Review of the ALCOSAN Regional Long Term Wet Weather Control Concept Plan.6 That report addressed questions raised about the LTCP and suggested a way forward. Although this committee did not conduct a comprehensive review of the TPR report, it acknowledges the overall value of that report and its findings in helping the committee to prepare the present report.
As noted previously, the draft LTCP includes modification and expansion of the wet weather treatment capacity of the ALCOSAN wastewater treatment plant to 310 mgd peak secondary treatment capacity and 565 mgd wet weather treatment for a total of 875 mgd (ALCOSAN, 1999). The TPRC concluded that the portion of the LTCP to expand the treatment plant, an activity that has already advanced to the facilities-planning stage, is a cost-effective component of the plan and should move forward. However, many concerns with other aspects of the draft LTCP were raised in that report.
The draft LTCP calls for reducing infiltration and inflow into separate sewer systems connected to the ALCOSAN interceptors from peak flows of 1,000 gpcd to a Pennsylvania state design standard of 250 gpcd at an estimated cost of $2 billion (ALCOSAN, 1999). The TPR report questions, however, whether such an investment would be adequate to reduce flows to this level (TPRC, 2002). Furthermore, the TPRC raised the question as to why such a reduction would be needed since the LTCP facilities were planned as if no reduction in flow were achieved.
The draft LTCP does not address costs of specific facilities required for systems other than the ALCOSAN-owned system, except for the Municipal Collection System Rehabilitation and/or Reconstruction Program (ALCOSAN, 1999). In order to convey large amounts of sewage to the ALCOSAN wastewater treatment plant during wet weather events, additional trunk sewers in partner communities may be required, but costs for this are not included in the LTCP. If paid for separately by the partner communities, there are likely to be inequities in payment schedules for the different communities and the TPRC (2002) recommended that this be addressed.
As noted previously, several recent developments in law, policy, and guidance have changed the regulatory requirements for addressing CSOs and SSOs. Because of these changes, the TPR report suggests that detailed monitoring and modeling are necessary to assess water quality impacts of wet weather events. They are also necessary to determine what actions and controls will be most cost-effective and timely, essentially favoring activities similar to those required in the demonstration approach. The TPRC report notes that other cities have invested large funds in overflow controls but have nevertheless faced subsequent and stringent enforcement actions because of public dissatisfaction that priority problems were not addressed. Public participation in the selection of facilities is recommended in the TPR report, together with a wider range of alternatives to better help determine how pollution prevention is related to facilities costs. A full financial capability analysis, including consideration of financial impacts on disadvantaged communities, is needed.
The TPR report raises concerns about heavy reliance on the use of vortex separators, because their effectiveness for water quality improvement has been inconsistent across the
6 |
Available on-line at http://www.alcosan.org/directory/third_party.htm. |
country (TPRC, 2002). In the draft LTCP, vortex separators are proposed for use without disinfection, so the separators may be ineffective for reducing the concentration of microorganisms in combined sewage. Also, questions about the acceptability of the many proposed upstream treatment and storage facilities have been raised. Additionally, the draft LTCP does not address many other water quality problems in the region, such as urban stormwater, acid mine drainage, upstream sewer overflows from other communities, wildcat sewers, failing septic systems, and agricultural runoff (see Chapters 3 and 4).
Based on these criticisms, the TPRC (2002) questions the ability of the presumption approach proposed by the draft LTCP to meet water quality standards. In fact, a conceptual screening model approach provided in that report indicated that bacterial water quality standards were unlikely to be met by the draft LTCP. The TPRC recommended that a watershed approach be adopted in order to determine what watershed needs are most important to stakeholders and how they can best be addressed and funded. Such an approach may affect the relative significance of CSOs and SSOs. The TPR report also recommends that a phased approach be used. The first phase would involve implementing the portions of the LTCP that are clearly cost-effective and would be part of any final plan for the region. This includes inspecting priority areas of the collection system and correcting structural deficiencies, gathering information needed to complete comprehensive facilities plans, conducting a comprehensive financial capability analysis, and beginning a process to determine the ultimate wet weather water quality requirements. In the second phase, additional abatement projects that are identified as a result of system inspections would be implemented. In the third and final phase, the extended LTCP actions identified and prioritized through use of a watershed approach would be undertaken. Lastly, the TPRC recommended that to develop a workable approach, several social, institutional, and financial realities must be addressed.
Cost Comparisons of ALCOSAN’s Draft LTCP
Other regions of the United States have experience with addressing wet weather-related water quality problems, and the cost of these efforts has been documented. The ALCOSAN draft LTCP includes a cost estimate of $3 billion, which includes activities by ALCOSAN within its own systems at $1 billion and costs for rehabilitation in the municipal collection systems and of private homeowners’ laterals at $2 billion (in 1999 dollars). These costs lead to an estimate of a total cost for the LTCP of $9,000 per ALCOSAN customer. They do not include costs for the more than 500,000 sewered customers in the region who are served by organizations other than ALCOSAN or costs associated with rehabilitation of OSTDSs in southwestern Pennsylvania. However, these costs represent an important point for comparison between the region and other areas of the country with wet weather water quality issues.
The third party review of the ALCOSAN draft LTCP (TPRC, 2002) provides EPA data on CSO program costs for representative U.S. municipalities (see Table 5-4). Although not directly comparable since these programs did not include costs for lateral repair, the cost per household indicated is lower than anticipated in the ALCOSAN draft LTCP. Based on the proposed multibillion-dollar investment in reducing untreated sewage overflows, regional investments to improve water quality must be prioritized.
TABLE 5-4 EPA Data on CSO Program Costsa for Representative U.S. Municipalities
As noted previously, the estimated cost of the ALCOSAN draft LTCP is in excess of $3 billion over approximately 50 years. This translates to an investment of about $9,000+ per household in the ALCOSAN service area (see Figures 1-1 and 1-2) or approximately $3,400 per person (TPRC, 2002). Because of the different infiltration and inflow problems in different wastewater collection systems and the uneven distribution of needed lateral repairs in the region, these costs will not be divided evenly over all ALCOSAN customers. However, an average cost over the customer base (amortizing the $3 billion bill over 50 years at a 5 percent interest rate) would be $40 per month. Some customers would pay much less and some much more; however, since this value is already at the affordability level suggested by the EPA for the median income in the United States (EPA, 2001a), the estimated LTCP costs are considered high. It is likely that this additional cost, however it is distributed among the population, would result in wastewater and water bills for some that would be substantially higher than in most communities around the country. Furthermore, comparisons of quarterly water and wastewater bills for Pittsburgh, detailed in Chapter 6, indicate that even before customers pay for the challenge of solving CSO problems, Pittsburgh’s water and sewer bills are higher than those of many U.S. cities with comparable population sizes.
Past federal grants have been a substantial part of the solution for many communities nationwide, thus reducing the local burden, but such federal grants are no longer likely to be available. Grant funding, which existed at a national annual rate of $8 billion to $10 billion at today’s values, has been replaced with annual appropriations of $2 billion to $3 billion directed primarily to loans to small communities with severe water quality problems and limited affordability capability. Extensive 20-year efforts by various water utilities and communities to obtain significant funding have not been successful, but federal funds remain available for research, security, and development of specific projects that received congressional approval based on the national significance of the project. Although such opportunities exist in southwestern Pennsylvania, in the committee’s judgment the likelihood of obtaining major federal grants for wet weather projects is remote.
Current Status of ALCOSAN Wet Weather Plans
The Narrative Summary of Active Projects: 2004 ALCOSAN Capital Budget (ALCOSAN, 2004) summarizes many ongoing and planned activities and their relationship to the ongoing consent decree negotiations, the draft LTCP (ALCOSAN, 1999), and the TPR report (TPRC, 2002) that are of direct relevance to this report, including CSO and SSO abatement
Programs; interceptor system improvements; planned (Phase II) wet weather plant expansion; distributed control system upgrade; the Satellite Treatment Facilities Demonstration Program; and the Flow Monitoring/Modeling/Water Quality Sampling Program. These important projects will be of central importance in improving water quality in the region’s urban core areas and critical to the success of a broader water quality management plan for southwestern Pennsylvania.
Regulatory Compliance Strategy
Regardless of the regulatory approach used in ALCOSAN’s LTCP for controlling CSOs, the committee concludes that it is necessary to address watershed-wide problems and sources of contaminants other than CSOs and SSOs. CSO abatement elements must operate as part of a system of controls, meaning that the individual abatement systems outside ALCOSAN’s authority and the ALCOSAN system must be reasonably optimized. The institutional and management needs and options to administer and finance such a system are presented in Chapter 6. Any solution must be cost-effective, and previously estimated costs (e.g., TPRC, 2002) have resulted in a high cost per person. Even so, the risk of failure to achieve water quality standards in the receiving streams is high. The system-wide solution must also include considerations of affordability and be cost-effective. Finally, ALCOSAN’s draft LTCP does not adequately consider the potential for innovative technologies and approaches for controlling wet weather discharges such as those described later in this chapter.
Economic Benefits of Reducing Water Quality Impairment
As discussed in Chapter 2, abundant water for human populations, industrial production, and transportation has been a cornerstone of the historic development of southwestern Pennsylvania. Since the early 1970s, there have been significant improvements in water quality, but problems remain. Addressing these problems would benefit the region’s current and future residents, as well as people who visit for recreational or other purposes; however, there will also be significant costs. Accordingly, a key issue is striking a balance between benefits and costs of water quality improvement. Cost and financing issues are addressed in more detail in Chapters 6. In this section, the general economic benefits of water quality improvements are examined.
Economic Services of Water
The contributions of water resources to the economy and the quality of life of the region derive from the services that water resources provide to people directly (e.g., for drinking, recreation, as an amenity) and indirectly from the use of water in the production of goods and services that people consume or value and that provide income and employment. The services that water resources provide to society are incredibly diverse. For a comprehensive review of this rapidly growing field, see Valuing Ecosystem Services: Toward Better Environmental Decision-Making (NRC, 2004b). Historically, water resources have been economically valued and managed for conventional uses such as public drinking water supplies, power generation,
irrigation, transportation, recreation, fisheries, and waste disposal and assimilation. Until recently, decision makers have tended to take for granted (i.e., place no value on) many other services provided by water resources that can be difficult to value economically such as providing habitat for aquatic ecosystems and aesthetics. However, these types of services are increasingly being recognized, leading to new demands on water management.
Mitchell and Carson (1986) provide a useful typology for considering the economic value of services from freshwater, and the sources of benefits or costs from changes in water quality (see Table 5-5). The values of water are broadly categorized as use values and nonuse (existence) values. The use class consists of current direct and indirect ways in which individuals or other entities make physical use of water. Examples include domestic uses (drinking, cleaning, preparing food, watering trees and lawns), recreational uses (swimming, fishing, boating, near-water recreation), and use for the production of goods and services (e.g., water used in industrial production, power generation, irrigation of crops, and hotels and restaurants). Use values can be subdivided into in-stream uses (“nonconsumptive” uses such as swimming, boating, fishing) and uses that require withdrawal (“consumptive” uses such as drinking, irrigation, cooling). The indirect category is subdivided into aesthetic and ecosystem values. Nonuse values (also called existence values or passive use values) are values that people hold for a resource without using or visiting that resource and do not require direct experience of use of a resource (Freeman, 2003; NRC, 2004b). Thus, people who make no direct or indirect use of particular water resources may still value their existence and condition.
Water quality degradation as found on various rivers and stream segments in the southwestern Pennsylvania region impairs water for various uses and possibly for existence values. Economic losses are associated with these impairments, and economic benefits are to be gained from their abatement and removal. Estimates of the benefits and costs of water quality protection projects could be very useful for helping to set water quality priorities and selection of projects that make economic sense (see Box 5-1). There are, however, no comprehensive estimates of the economic benefits of addressing the remaining water quality problems for southwestern Pennsylvania or from proposed projects to address the region’s water quality
TABLE 5-5 A Typology of Possible Benefits Resulting from Improvements in Freshwater Quality
Benefit Class |
Benefit Category |
Benefit Subcategory (Examples) |
Use |
In-stream |
Recreational (fishing, swimming, boating) Commercial (fishing, navigation) |
|
Withdrawal |
Municipal (drinking water) Agriculture (irrigation) Industrial/commercial (process treatment) |
Aesthetic |
Enhanced near-water recreation (hiking, picnicking) Enhanced routine viewing (office and home views) |
|
Ecosystem |
Enhanced recreation support (duck hunting) Enhanced general ecosystem support (food chain) |
|
Nonuse (Existence) |
Vicarious consumption |
Significant others (relatives, close friends) Diffuse others (general public) |
|
Stewardship |
Inherent (preserving remote wetlands) Bequest (family, future generations) |
SOURCE: Adapted from Mitchell and Carson, 1986. |
BOX 5-1 Concepts and methods for estimating the benefits and costs of environmental improvements are well-developed (see for example, EPA, 2000a; Freeman, 2003; NRC, 2004b). The fundamental theoretical concept for valuing the benefits of water quality improvement is willingness to pay (WTP). An individual’s WTP for a water quality improvement is the maximum amount of money the individual would voluntarily exchange for an improvement in water quality rather than have water remain in the existing condition. The underlying principal is that the economic value of goods and services, whether sold in a market or made available through other means, is defined by the economic trade-offs individuals are willing and able to make in order to consume the good or service. When the amount that an individual is willing to pay exceeds the cost of the good or service to the individual, the individual realizes an “economic surplus.” Benefit estimation essentially entails measurement of changes in economic surpluses. The total benefit of a water quality improvement is the sum of the individual benefits. For example, suppose a particular individual considers a good day of fishing to be worth $50. Presently, the individual must travel an hour to find a spot that will provide a good day of fishing, the cost of which, including time, is $40.00. Thus, the economic surplus per day of fishing is $10.00. Now suppose that a stream restoration project nearer to home reduces the travel time and cost for a good day of fishing in half. The economic surplus for a fishing day at the new site is $30.00, resulting in an economic surplus gain of $20.00 per fishing day. Suppose this hypothetical individual fished 10 days a year at the original site but would fish 15 days a year at the closer, less expensive site. The total benefit to the individual is then $350.00 per year. While the concept of WTP may not have immediate meaning or appeal to the noneconomist, some of the measurements to infer WTP may have utility. These measurements would include the following: increased value of residential properties adjacent to streams, rivers, or lakes that would benefit from water quality improvements that increase the amenities of living on or near such water resources; reduced expenditures on medications and medical care that would result from reduced incidence of waterborne disease transmitted in drinking water or by exposure through in-stream recreation; reduced expenditures on water treatment or procurement by households, municipalities, and industries to achieve mandated or desired water quality levels in drinking water or water used in industrial processes; and increased profits to businesses that realize productivity gains from water quality improvements. It is important to emphasize that environmental valuation for project and policy analysis generally is not concerned with the total economic value of an existing or prospective environmental condition, but rather in the benefit or cost that results from a change in condition, because this is the relevant information in assessing whether a change is economically beneficial. An assessment of the benefits of a water quality project or policy should in principle begin with a set of questions about current conditions without the policy and the conditions projected to exist with the policy. In the typical “effect-by-effect” approach to benefit assessment (EPA, 2000a; Ribaudo and Shortle, 2001), key data would include the following:
|
problems. Nevertheless, potentially large benefits from addressing remaining water quality problems in the region can reasonably be anticipated. To obtain a greater sense of the potential economic benefits of water quality protection and improvements, the benefits of drinking water protection and recreational benefits are discussed in the following sections.
Benefits of Reducing Drinking Water Contamination
Given the frequent discharges of untreated sewage upstream of drinking water intakes and frequent findings of pathogens and their microbial indicators in source waters discussed in Chapters 3 and 4, it can be expected that there would be considerable economic benefits from reducing microbial contamination of source waters, contingent on a positive probability of treatment system failures. Use of contaminated source waters for drinking imposes at least three types of economic costs. The first is the cost of water treatment to eliminate or reduce the presence of disease-causing agents in drinking water to acceptable levels. The second is cost of the risks of disease from potential exposure to contaminated drinking water in case of treatment system failure. Economic benefits would accrue from reduced discharges of untreated sewage if the reduction permits treatment costs to be diminished or waterborne disease risks to be reduced. Costs are also incurred in monitoring water quality and in treating water to meet federal and state drinking water standards.
Generally, the risks of endemic waterborne disease in a well-managed system are very low (see NRC, 2004a). However, in some instances, treatment system failures can result in episodes where finished water is grossly contaminated and poses a significant threat to the health of customers. In such instances, water system authorities must notify customers that the water is unfit for consumption and issue advisories to boil water or take other steps to protect their health (see also Box 3-4). Costs associated with measures to prevent disease are referred to as avoidance costs and can be very high depending on the number of people affected and the length of advisory (e.g., Abdalla, 1994; Harrington et al., 1991; Laughland et al., 1996). The costs associated with morbidity from waterborne diseases include those of medical treatment, days lost at work, and related pain and suffering. The costs of morbidity and mortality from waterborne disease outbreaks can be substantial depending on the number of people affected and the severity of illness.
The costs of a water contamination event can be illustrated by a giardiasis outbreak in Pittston, a town in northeastern Pennsylvania. The PADEP issued a boil water advisory for the community in December 1983. The advisory was lifted for some residents in March 1984 but remained in effect for nearly nine months. A study by Resources for the Future estimated the costs of the waterborne disease outbreak at between $23 million and $55 million (in 1984 dollars) (Harrington et al., 1991). Medical costs, lost work time, lost work productivity, and lost leisure time of those who became ill were estimated at $5.6 million. The costs of actions taken by community residents to avoid drinking contaminated water (e.g., boiling water, hauling or purchasing water) were estimated at $12.9 million. Costs to area businesses (e.g., restaurants) from measures to contend with contaminated water or from business losses were estimated at $3.6 million. It is apparent from this example that the economic costs of a widespread contamination event resulting in a boil water advisory and illness affecting one of the many community water supply systems in southwestern Pennsylvania could be quite substantial.
Recreational Benefits
In addition to the potential economic benefits resulting from drinking water protection, economic benefits may also be derived from improvements in water quality for other uses. As discussed in Chapter 3, approximately 26 miles of streams and rivers have been listed as impaired for contact recreational use in southwestern Pennsylvania. Research on water quality benefits has demonstrated that there can be substantial economic value in restoring water quality to levels that permit recreational uses. These benefits can accrue from reductions in the risks of waterborne disease and their related costs; from increases in the opportunities and levels of swimming, boating, and fishing; and from improvements that enhance the quality of recreational experiences (see, for example, EPA, 2000a; Mitchell and Carson, 1986).
The benefits of water quality improvements at recreational sites on the Monongahela River in the early 1980s were estimated by Smith et al. (1986). Depending on the specific valuation methodology, the study found the value per trip per person for an improvement in water quality from boatable to swimmable to be between $12.95 and $56.39 (updated to 1998 dollars as reported in Koteen et al., 2002). These findings in conjunction with the significant improvements in water quality in southwestern Pennsylvania over the past 30 years would suggest that the region has already realized possibly large economic benefits from water quality protection and improvement.
The essential issue now is the additional gains that may be obtained from further reductions in recreational impairments. Clearly lacking for such an analysis at this time are estimates of the increased recreational use of waters that would occur with possible water quality improvement projects. There is, however, ample evidence that current residents are willing to pay for recreational water quality improvements. For example, Heberling et al. (2004) estimate the economic benefits of remediating AMD in Clearfield Creek in Clearfield County, Pennsylvania, to the east of the study region. The study estimated that, on average, Clearfield County households would be willing to pay at the margin $54 per year to increase the quality of the stream from its currently highly degraded baseline level to fishable (holding all else constant). Farber and Griner (2000) estimate the benefits of improving the water quality of Loyalhanna Creek and the Conemaugh River, both subwatersheds of the Allegheny, that are degraded primarily by AMD. For one scenario, improving stream condition from severely degraded to unpolluted, they find valuations ranging from $75.63 to $112.44 per household per year. Both studies were conducted in the mid-1990s.
Less place specific, but still indicative of the economic values people place on recreational use of waters, are estimates of the economic value of various water-based recreational activities adapted from a literature review by Rosenberger and Loomis (2001). These are presented in Table 5-6. The values are per person per activity-day and can be used to provide a rough guide to possible gains resulting from increased recreational use along with reasonable projections of the increased recreational use of water resources associated with water quality improvements.
Summary
There are no comprehensive estimates of the economic benefits of addressing the remaining water quality problems of southwestern Pennsylvania or from proposed projects to
TABLE 5-6 Selected Average Consumer Surplus Values per Activity-Day per Person from Recreation Demand Studies (1967-1998)a
address the region’s water quality problems. Nevertheless, the region would be expected to benefit economically from measures that significantly reduce drinking water risks and enhance recreational opportunities. Whether benefits would exceed the costs of water quality projects cannot be known without analysis of specific options. Estimates of the benefits, in addition to the costs, of specific water quality projects could be very useful for helping to set water quality priorities and selecting projects that make economic sense considering both benefits and costs. Further, to the extent that it can be shown that benefits exceed costs, benefit estimates could be used as a tool for building public support for regional water quality initiatives.
COMPREHENSIVE WATERSHED ASSESSMENT AND RESPONSE PLAN
The committee believes that the evaluation of causes and sources of water quality impairment in southwestern Pennsylvania must be refined and integrated with the decision-making structure for water quality improvement actions. Further, the appropriate scale for this evaluation and for water quality improvement decisions is the watershed level. In this regard, the flow of water from the Allegheny and Monongahela Rivers to the Ohio River is a single hydrologic regime. Prudent management of water quality in this system should consider all factors that affect water quality and anticipate future uses of the water resources of the region. To achieve this, it is necessary to develop both a technical and an institutional-financial approach. The institutional and financial approach is discussed in Chapter 6, and the technical approach is embodied in what the committee calls the “Comprehensive Watershed Assessment and Response Plan,” or CWARP is discussed in this chapter. It is important to note that the “Three Rivers CWARP” described in this report is not a single document or program. It is an intentionally flexible umbrella concept identifying activities that can be carried out by the organizations that are most technically and institutionally capable of achieving the desired results. Therefore, activities will be conducted at various levels and by various organizations depending on existing and potential capabilities.
The CWARP relies on the acquisition of sufficient knowledge about the Three Rivers watershed’s response to various contaminant loads at different temporal and spatial scales to prioritize problem agents, develop specific action plans, predict water quality benefits, and estimate related costs and affordability. It is especially important that this plan be followed in the ALCOSAN service area and neighboring communities where very large capital outlays have been proposed to reduce or eliminate CSOs.
Planning and Implementation Framework
The framework recommended for planning and implementation of CWARP consists of five basic steps as follows:
-
Problem identification
-
Assessment of existing conditions, including quantification of loads and modeling their relationships to water quality
-
Projection of future loads, their timing, location, and impacts on streams
-
Formulation and evaluation of alternative management strategies, including assessment of the effects of alternatives on future conditions and ordering and scheduling of various elements of the preferred strategy
-
Adaptive implementation of elements of the strategy, relying on feedback from implementation of each element to inform reformulation and evaluation of subsequent elements.
Water quality problems in the region occur at several different temporal and spatial scales, arise from several different classes of sources, and will require very different planning and management strategies and different kinds of organizational arrangements. At one end of the spectrum are problems related to long-distance transport of contaminants and their impacts within river basins at the scale of the Allegheny, Monongahela, and Ohio Rivers over which state and interstate agencies have jurisdiction. At the other end of the spectrum are threats to health of individual families served and/or affected by nearby failing on-lot wastewater disposal systems, the regulatory responsibility for which has been delegated to local governments. In between are the problems of contamination from CSOs, SSOs, and stormwater runoff during and after rainstorms in high-density urban areas. In this report, planning and management for water resources is viewed as consisting of needs at four interrelated scales:
-
river basin;
-
multicounty/metropolitan scale;
-
high-density urban areas; and
-
rural areas.
The five-step process must be adapted to address each of these scales.
The committee recognizes that the region is not starting with a blank slate, but nonetheless it is useful to provide this framework. Step I has been largely completed for all four scales. The assessment of water quality and sources of contamination as discussed in Chapters 3 and 4 provides a reasonably complete picture of the problems. Substantial progress has been made on estimation of loads and development of predictive models as called for in Step II, but as noted earlier, significant gaps remain. Because the problems are largely associated with existing conditions and there is only modest growth in the region as a whole, Step III may be less important, but changes in land use that are occurring in suburban areas (see Chapter 2) cannot be ignored. Some of the alternatives called for in Step IV of CWARP have been formulated in ALCOSAN’s draft LTCP (ALCOSAN, 1999) and focus on the urban core areas of the region, but that set of options is limited in scope. Many steps toward water quality improvement in the region have already been identified and implemented, but it is clear that many more will be required under Step V for all scales of CWARP.
River Basin Planning and Management
Southwestern Pennsylvania lies primarily within the Allegheny, Monogahela, and Ohio River basins that transport contaminants into the region and export remnants of its waste and stormwater to downstream reaches of the Ohio. Several water quality problems in the area appear to transcend regional boundaries and are logically the jurisdiction of state and interstate agencies. The two most prominent large-scale water quality problems appear to be: (1) metals related to AMD, and (2) mercury and persistent organics, both of which may be legacy contaminants, resulting from past agricultural, industrial (especially mining), stormwater, and sewage practices. The microbiological contaminant linkages are uncertain, despite the current regulatory environment. Given the lack of established linkages at the river basin scale, CWARP should be focused on Steps I and II, which are discussed together below.
Steps I and II of the planning process at the basin scale should seek to enhance data collection and analysis by various agencies, ongoing or planned, to better define long-distance transport and fate of contaminants into and out of the region. One program that shows promise is EPA’s rejuvenated Environmental Monitoring and Assessment Program (EMAP).7 The EPA has recognized that comprehensive, large-scale monitoring information on large river ecosystems is vital but lacking. One component of that program, called Great Rivers,8 has as its objective to improve the scientific basis for water quality assessment at the large scale. It uses a probability sampling approach to generate statistically valid inferences about the status and trends of water quality in those rivers.
Preliminary designs for monitoring and analysis have been completed for 3 of 11 river systems nationwide, including the Ohio River from the City of Pittsburgh to its confluence with the Mississippi. The Three Rivers CWARP should take advantage of the Great Rivers component of EMAP to help design and fund the needed additional water quality monitoring. The Great Rivers effort presents an opportunity for EPA and Pittsburgh region water quality authorities to collaborate on water quality data collection, because there is a shared interest in generating water quality status and trends information for improved decision making, especially as it relates to provisions of the Clean Water Act. While the program is still in development, it would seem wise to extend it spatially to capture the lower reaches of the Allegheny and Monongahela Rivers. This could assist in developing a validated probabilistic approach to sampling as well as in eliciting valuable direction and critique from EPA and the Ohio River Valley Water Sanitation Commission (ORSANCO; see Chapter 6 for further information).
Several programs of the U.S. Geological Survey (USGS) should also provide valuable sources of water quality information for CWARP. The USGS is widely recognized for its national stream gauge and water quality programs, but it is a major contributor to regional water quality programs, as well. The USGS has provided valuable water quality information for more than a century in the Ohio River basin in Pennsylvania and in the greater Pittsburgh region. More specifically, USGS administers both historic and current data for more than 400 water quality sampling sites in southwestern Pennsylvania, where it tests for various and multiple parameters of water quality across the region. Although most of the historic USGS sampling efforts targeted chemical (e.g., nutrients, pH), physical (e.g., temperature), biological (e.g., dissolved oxygen), inorganic, radiologic, or sediment attributes of water quality, beginning in
7 |
Further information about EMAP is available on-line at http://www.epa.gov/emap/index.html. |
8 |
For more information on EPA’s Great Rivers program, see http://www.epa.gov/nheerl/arm/greatrivers.htm. |
2001, sampling in some areas now includes testing for fecal coliforms, Escherichia coli, enterococci bacteria, and turbidity (see Chapter 3 for further information).
Some of these multiple-test sites represent collaborative efforts in the region to address water quality issues from the USGS National Water Quality Assessment Program (NAWQA), the National Stream Quality Accounting Network, the U.S. Army Corps of Engineers and other federal agencies; ORSANCO; and Carnegie Mellon University. Southwestern Pennsylvania provides a good example of how a national-level program can generate water quality monitoring data at regional and local scales in a timely, responsive, and reliable manner that can be used in support of decision making. The NAWQA program, in particular, for several years sought to gain understanding of water quality conditions, trends, and stressors in the Allegheny and Monongahela basins (see Anderson et al., 2000 and Appendix B) but unfortunately, that focused effort has been terminated for budgetary reasons (see NRC, 2002). Nonetheless, data collected on water quality are maintained by the USGS, are available to the public, and provide a long-term, rich source to track changes and trends in water quality in the Allegheny, Monongahela, and Ohio Rivers.
Biological Water Quality Monitoring
Because regional information on the biological quality of receiving waters is scant (see Chapter 3), its collection during and in support of CWARP at the river basin scale is critical. To be comprehensive, it is necessary to assess and evaluate not only physical and chemical, but also biological water quality information to determine ecosystem health (see Box 5-2). Biological water quality indices, and their change over time, can yield important information about ecosystem change and help quantify the environmental benefits affected by pollution abatement. Thus, information collection for CWARP should include biological data to assist in ecosystem health assessment benchmarking and to help document changes to the ecosystem that occur as a result of changing stressors. At a minimum, CWARP should be designed to establish an Index of Biotic Integrity (IBI) benchmark so that changes in environmental health can be linked to changes in the IBI for the regions’ three main stem rivers.
Step III should focus on models to track the fate and transport of contaminants within the basins. It should be noted that EMAP’s Great Rivers program is limited to an assessment of status and trends of aquatic ecosystems. The preliminary design for the Ohio River component is focused only on effects; it does not include any tasks to track causes of degradation or to relate cause and effect through water quality or ecosystem models.
Sources and Linkages
Given existing evidence and that which may be forthcoming from the EMAP Great Rivers program and other monitoring, there is a need to identify probable sources of contaminants that places stress on the aquatic ecosystems in southwestern Pennsylvania. It would be desirable to use an aquatic ecosystem model such as EPA’s AQUATOX in such an effort. This tracks concentrations of nutrients and organic chemicals, temperature, and their
BOX 5-2 The use of biological indices as surrogates for in-stream environmental quality has become increasingly common across the United States. The modern water quality monitoring criteria were developed in order to provide a system for measuring the “biological integrity” sought by the Clean Water Act (Karr et al., 1986). Davis and Simon (1995) describe the development and application of modern indices of biological integrity. The most widely applied methods for assessing biological integrity involve collecting, counting, and enumerating species within a taxonomic group (typically fish or benthic macroinvertebrates such as aquatic insect larvae) and comparing the measured characteristics of the sampled community to a reference community using a scoring method. Standardized collection methods are codified for any local or regional area. Watershed areas are generally characterized within some defined biogeographic area (typically ecoregions). Habitat characterization is performed and usually compared to some concept of an expected habitat to support the assessed fish or macroinvertebrate community. Scores calculated by these methods are unit-less numbers that represent the relative health and performance of the chosen community in that location. The most common versions of these scores are generally termed Indices of Biotic Integrity for stream fishes in North America (Karr et al., 1986). The IBI is a “multimetric” index based on adding subscores for different characteristics of the distribution and abundance of species within a sample. The indices incorporate measures of pollution tolerance, pollution intolerance, distribution of different feeding groups, and other characteristics of resident fish that provide measures of community composition and performance. The original IBI has been modified and incorporated across a range of watersheds and has been developed for other groups such as macroinvertebrates and even some terrestrial communities such as birds (see O’Connell et al., 1998). The multimetric approach has been applied successfully in a regional and local context, such as the long term control plan for the City of Akron, Ohio.1 The methods are most successful where a good knowledge base exists about expected community composition within a regional or watershed area. Although some of the methods used to develop these indices can be standardized nationally, it is not possible to develop an index that can universally be applied across widely varying regions because of the different biota normally present in different habitats and climates. The National Research Council included multimetric indices in its review of biological and ecological indicators as a local or regional indicator (NRC, 2000). The Heinz Center has also included multi-metric indicators as a possible gauge of freshwater conditions nationally (by aggregation of regional scores) in its recent effort to provide a measure of ecosystem resources for the nation (Heinz Center, 2002). The Ohio River Valley Water Sanitation Commission Compact (1948)2 states that the Ohio River should support and maintain a balanced, diverse, and healthy ecosystem. To determine whether pollution control efforts have achieved this objective, ORSANCO routinely conducts biological assessments that include the following components: (1) macroinvertebrate population reviews, (2) fish population studies, and (3) a fish tissue contaminants program. Macroinvertebrate populations provide perspective on aquatic life conditions in the river because many species are highly sensitive to pollution and are relatively immobile. The ORSANCO conducts macroinvertebrate sampling each year including site-specific studies, pool-wide studies, and river-wide surveys. Fish population studies have been a major component of ORSANCO monitoring activities throughout the history of the organization. Studies in 11 of 20 pools on the Ohio River below Pittsburgh have resulted in the collection of data for the development of biological criteria, or biocriteria, for the Ohio River. Methods employed included sampling fish in the chambers of locks at dams on the Ohio River and electrofishing. The fish tissue contaminants program of ORSANCO characterizes the levels of certain contaminants, such as pesticides or other organic chemicals, in Ohio River fish and provides a basis for determining the need for human health or fish consumption advisories.
|
effects on selected species in the ecosystem, including fish, invertebrates, and aquatic plants.9 Outputs are the concentration or masses of species distributed over time and space. This approach has its limits. While it can track effects on individual species, mechanistic ecosystem models have not advanced to the point of being able to predict community structure or biotic integrity.
Even in the absence of formal models that relate cause and effect at the river basin scale, it is important that the most likely source of ecological stresses be identified through best available evidence. Processes are in place for Step IV of basin-scale planning. They include the establishment of assimilative capacities of streams consistent with water quality standards through the development of total maximum daily loads (TMDLs). Federal and state agencies have this authority under the Clean Water Act. They also have the authority to allocate these loads to sources. As discussed in Chapter 6, federal and state financial assistance can also be targeted to priority areas. Thus, planning at the river basin scale establishes priorities and performance standards that must be addressed by sources within the region, whether they be urban or rural in character.
Multicounty/Metropolitan Planning and Management
A second scale included in CWARP is the multicounty or metropolitan level. Regional planning for transportation and economic development at the multicounty scale is being conducted by the Southwestern Pennsylvania Commission (SPC; see Chapter 6 for further information). This organization is the officially designated metropolitan planning organization for transportation planning. It has developed an extensive database on the regional landscape, economic activities, land use, and transportation systems. However, water resources planning at that scale is not well-developed. Either SPC or an alternative organization should formulate regional water resource plans and integrate them with transportation and land use plans.
The CWARP planning process should focus on at least two aspects of water management at the multicounty level. First, large-scale transportation planning is occurring at that scale. Decisions about transportation infrastructure have significant effects on land use and watersheds. At the very least, water quality planning at this scale should be sufficient to inform regional interests of the potential effects (including constraints if any) of water quality conditions on future development; consequences of development on water quality where it occurs; and how these effects and consequences can and should be modified. Second, planning at this scale should also result in the identification of opportunities for economies of scale in the delivery of water and wastewater services through cooperative arrangements among local governments. Those opportunities are likely to exist primarily among clusters of local governments located in a common drainage area, but substantial benefits of cooperative arrangements also may accrue to sets of small communities and rural areas that do not and cannot afford to have the technical or administrative expertise to manage their water resources properly.
Step III should be an assessment of impacts of growth on the water resource, existing infrastructure for water supply and water quality, and opportunities to achieve economies of scale in the provision of water supply and wastewater management. A useful technique to support this assessment is development of alternative growth scenarios and their relationships to
9 |
For further information on AQUATOX and other ecosystem models, see http://www.epa.gov/waterscience/models/. |
regional transportation systems. In addition, water demands, wastewater generation, stormwater, nonpoint source pollution, and conversion of land uses should be estimated for each scenario.
Priority areas should be identified in Step IV based on results of Step III, and strategies should be formulated to address those priorities. Strategies should include both positive incentives to guide development away from critical areas and toward cost-effective infrastructure using a variety of techniques, including but not limited to public education, zoning, investments in infrastructure, transfer of development rights, conservation easements, and outright purchase of especially sensitive areas.
Finally, the multicounty process should include as Step V a continuing monitoring, assessment, and reporting process that keeps the public informed about the state of water resources and needed corrective actions as development occurs.
High-Density Urban Area Planning and Management
As noted earlier, the most pressing water quality problem in southwestern Pennsylvania from a regulatory perspective, especially in the region’s urban core areas, is degradation of the microbiological quality of streams due to CSOs, SSOs, and discharge from separate stormwater systems in wet weather conditions. Clearly the most significant of the high-density areas is the contiguous urban area centered on the City of Pittsburgh. An approximate boundary for that area is shown in Figure 5-2. It covers much of Allegheny County and portions of Beaver, Butler, Washington, and Westmoreland Counties (see also Box 1-2). More than 1.25 million people live in that area, and it is affected by both SSOs and CSOs. There are other high-density urban areas outside the contiguous urban core. Several smaller cities and boroughs not directly linked to the urban core have densities in excess of 3,300 persons per square mile—far above the criterion of 1,000 used by the U.S. Census Bureau for defining urban areas. Among them are Indiana, Greensburg, Uniontown, Washington, Butler, and New Castle. These urban areas may be experiencing SSOs, excessive infiltration and inflow, and other water quality problems, but they are not plagued by the problem of widespread CSOs. In the discussion that follows, all urban areas are treated alike, but steps in the process related to CSOs would not be applicable to the outlying urban areas. Some of the outlying urban areas also do not offer the same opportunities for cost-effective collaboration as in the contiguous urban core.
As discussed in Chapter 3, elevated levels of fecal indicator bacteria (e.g., coliforms) far in excess of water quality standards have been observed during and immediately after storm events that caused CSOs. What appears to be lacking is an adequate quantification of sources of fecal indicator bacteria and an effective strategy to reduce their levels to acceptable concentrations. Although Step I of the planning process is reasonably complete at the urban scale, Steps II-V are not and are discussed in the following sections.
Step II—Assessment of Existing Conditions
Step II of the CWARP process for high-density urban areas should include simultaneous monitoring of (1) wet weather discharges into the region’s streams and rivers and (2) impacts on these receiving streams. It should also include development of models that are sufficient to disentangle effects of multiple sources on water quality in receiving streams. For example,
models that track flows of stormwater and sanitary sewage from contributing watersheds into and through the array of collection systems to points of discharge are also included under Step II. The models and data should be available for public review, and data from these technical studies should be reduced and translated into needed corrective actions in a manner that is understandable to decision makers and the public in general.
Pollutants of primary concern in this context include the following:
-
pathogenic microorganisms such as enteric viruses, Cryptosporidium, Giardia, and their surrogates (indicators) including total coliforms, fecal coliforms, and Escherichia coli;
-
oxygen-demanding substances including carbonaceous and nitrogenous organics, suspended solids and sediments; and
-
“conservative” toxic substances such as metals and toxic organics, including pesticides and herbicides.
Monitoring of Discharges of CSOs and Separate Stormwater Systems. The primary sources of wet weather contamination in the high-density urban areas are CSOs, SSOs, and surface water runoff, including direct runoff to streams and runoff that is transported through separate stormwater sewer systems (i.e., stormwater drainage).
An initial task is to delineate the areas that contribute to these sources. This includes not only the high-density urban areas, but lesser-developed areas of watersheds from which stormwater flows are mingled with urban runoff. Delineation of these areas will require a detailed examination of watershed boundaries and urban development in the area. A preliminary delineation is shown in Figure 5-2 for illustrative purposes with an overlay of contiguous urban areas and watershed boundaries in and adjacent to Allegheny County.
Within these watersheds it is important that monitoring stations be established and operated so as to provide estimated temporal and spatial patterns of pollutant loads from CSOs and from separate stormwater sewer system discharges. As discussed in Chapter 4, urban stormwater discharges are associated with numerous pollutants (including fecal indicator bacteria) and effects on receiving waters, though the impact of stormwater on public health has largely been ignored.
Montoring and Modeling of Receiving Water. A basic task in Step II of implementing CWARP in high-density urban areas is to construct a credible relationship between the sources of stressors (discharges from CSOs and separate stormwater sewer systems) and quality of water in receiving streams. This requires simultaneous monitoring of discharges and instream responses during storm events. It also requires that concentrations of contaminants in streams flowing into the system from upstream sources be accounted for by monitoring at system boundaries. A detailed monitoring system should be designed using specific information about locations of streams and stormwater management within contributing watersheds.
However, a substantive discussion of such information is considerably beyond the scope of this report. Thus, only a tentative delineation of the area to be monitored and modeled is provided (see Figure 5-3). To isolate the greater Pittsburgh area from upstream sources of pollution to the Monongahela, Youghiogheny, and Allegheny Rivers, boundary monitors would
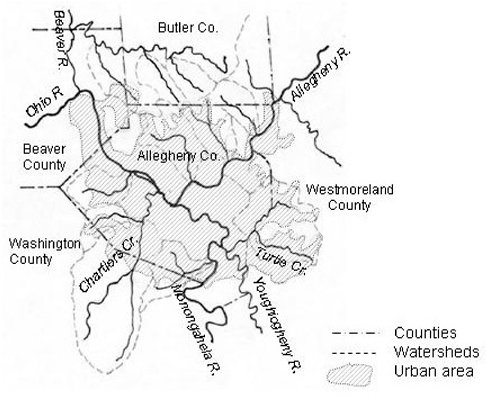
FIGURE 5-2 Watersheds and contiguous urban areas in and adjacent to Allegheny County. SOURCES: U.S. Census Bureau, http://ftp2.census.gov/geo/maps/urbanarea/uaoutline/UA2000/ua69697/ and http://tiger.census.gov/cgi-bin/mapbrowse-tbl.
have to be established somewhere in the vicinity of those shown in Figure 5-3. Downstream of those locations, there are approximately a dozen major watersheds that also contribute urban runoff and CSOs that affect water quality in the Monongahela, Allegheny, and Ohio Rivers. Each of these watersheds should be monitored to determine stresses placed on the major receiving streams. Instream monitoring should extend as far below the CSO and WWTP outfalls as necessary to determine the fate of contaminants entering the three major rivers. Wet weather monitoring should be focused on a short list of pollutants that are either known to cause or are suspected to cause significant water quality degradation during storm events. Fecal coliform bacteria or other indicators of waterborne pathogenic microorganisms would be high on that list.
Receiving water models should be able to simulate the fate of conservative (e.g., salt, metals), nonconservative (e.g., oxygen demand, nutrients), and microbiological (e.g., coliforms, Cryptosporidium) pollutants. The CWARP data collection program must be designed to quantify pollutants of concern discharged to the rivers as well as to calibrate the models with selected data on river concentrations of these same pollutants within the study area.
Wet weather events occur over relatively short periods of time (days), and there is considerable variation in both temporal and spatial patterns of loads and impacts within each event. Therefore, models covered by this discussion must be capable of representing both temporal and spatial variations, and they must include hydrologic and hydraulic behavior as well as water quality. Receiving water models exist for almost every type of waterbody such as wetlands, streams and rivers, lakes, reservoirs, and estuaries. Like collection system models, receiving water models have been available for some time and for almost every type of conceivable application. Some of the first, and still most frequently used, receiving water
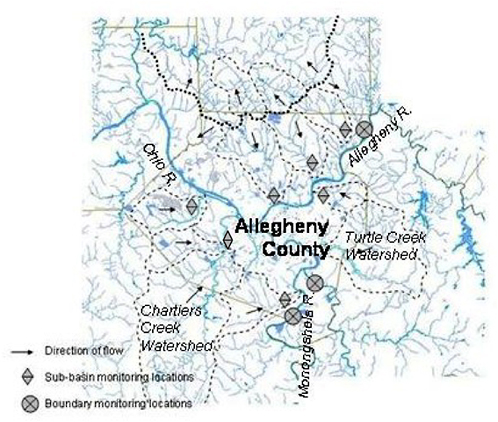
FIGURE 5-3 Preliminary representative sites for CWARP monitoring activities in the region’s urban core areas.
models were developed by the U.S. Army Corps of Engineers, EPA, and the U.S. Department of Agriculture Natural Resource Conservation Service. Water quality models of this type, including the EPA’s WASP6 and AQUATOX models, tend to be more complex and require much more input data than the steady-state models frequently used by regulatory agencies for waste load allocations. Despite their complexity, these models are continually being supported by federal agencies, and there is a body of experience with their use.
As with collection system models, the most recent improvement in the application of receiving water models is coordination with a geographic information system (GIS). This allows users to quickly generate input files, run modeling scenarios, and graphically and spatially view the modeling results through a GIS interface.
The type of water quality model necessary to simulate the Three Rivers watershed system for short-term CSO flow and load impacts is categorized as a multidimensional, time-variable water quality model of the finite difference or finite element mathematical process. Assuming the Three Rivers hydrodynamic regime is vertically well mixed (nonstratified), then a two-dimensional, advective (forward flow) hydrodynamic model coupled with a fully capable water quality model, including dissolved oxygen deficit and nutrients and sediment oxygen demand, would be necessary. The time increment used to advance the model must be sufficiently short to address the impact of short-term CSO wet weather flows and load.
Although receiving water quality modeling appears to be extremely limited in the region’s three main stem rivers, the committee recommends that it be used to estimate impacts of pollution loadings on the receiving streams and to help prioritize alternatives for pollution control. Programs that have used similar models include ORSANCO’s work in the Ohio River basin (see, for example, ORSANCO, 2002) and EPA’s Great Lakes Initiative.10
It is important to recognize that in one of the first activities in CWARP planning and implementation (i.e., data/information collection), expert consultation is necessary to more fully define the particular model, the exact hierarchy of pollutant investigation, and the monitoring program necessary to calibrate and validate the mathematical model simulations prior to using the predictive components of the model.
Sewer System Routing Models. The use of an additional set of models from that used in Step II will be necessary to support Steps III and IV of CWARP in the high-density areas of southwestern Pennsylvania. Driving forces on the most severe wet weather events in the Pittsburgh region that have adverse effects on water quality are rainstorms. Although it is likely that melting snow packs also cause problems, they do not occur during seasons of intense recreational use of the region’s streams and rivers. Both types of events could adversely affect any downstream water quality and supply, and aquatic ecosystems.
An understanding of the temporal and spatial patterns of the flow of stormwater and sanitary sewage into and through the extensive network of separate and combined sewer systems in the urban core of the Pittsburgh region is essential to the formulation and evaluation of management strategies. It is necessary to understand these flow patterns so as to identify key locations for which in-line or off-line storage and treatment options may be used to achieve cost-effective strategies. Flow patterns must be understood for a range of typical storm events to support design of collection systems. There is also a need to have monitoring and models of real time flows in the networks to support management of these flows during storm events that minimize adverse effects on receiving streams.
The suite of models for this task must include the following:
-
rainfall-runoff models for all tributary watersheds, including both urbanized and nonurban areas that generate both hydraulic and contaminant inputs to combined sewer systems and separate stormwater sewer systems;
-
dynamic stormwater routing models that transport stormwater inflows and their pollutant loads from points of entry to points of discharge; and
-
dynamic combined sewer models that perform similar functions.
Dynamic Sewer System Modeling. Dynamic sewer system modeling can be utilized to simulate hydraulic and pollutant loading characteristics of extensive sewage collection systems, including CSO regulators. Mathematical models such as XP-SWMM (a stormwater and wastewater modeling package) have been used extensively for such purposes. ALCOSAN and its consultants utilized dynamic modeling in the development of the draft LTCP (ALCOSAN, 1999). The committee recommends that this application be expanded to simulate watershed-
10 |
For more information on the EPA’s Great Lakes Initiative, see http://www.epa.gov/waterscience/GLI/. |
wide collection system behavior under CWARP. Hydraulic and pollutant load data developed from ALCOSAN’s partner communities should be evaluated for inclusion in such an expanded model, if necessary. It is important that sewer system hydraulic modeling include analysis of express sewer contributions to the collection system.
Dynamic Stormwater Modeling. Dynamic (time-variable) stormwater models can combine hydrological (storm runoff hydrographs) and flood routing mechanics to calculate flows associated with precipitation from storms. These simulations are important for estimating the rate of stormwater flow at various locations and times over land and in the collection system, especially if sanitary sewer separation is recommended as one option to reduce or eventually eliminate CSOs. Understanding the integrated relationships between CSOs and separate stormwater flow is necessary for dealing with the safe and effective transport and discharge of each.
Real-Time Sewer Flow Control Modeling for Analysis and Operation. A key feature of a well-structured, long term CSO control plan is effective operation of the wastewater system in order to maximize in-system storage as well as flow to and through the treatment plant. Not only are these concepts embodied in EPA’s CSO regulations (the nine minimum control provisions; see EPA, 1995b), but by effectively achieving these objectives, a municipality can substantially reduce the cost of compliance.
At the core of a successful operational control technology for both collection and treatment is the ability to monitor the system and then to respond to changes in the system in a manner that maximizes system capacity and minimizes adverse impacts on the environment resulting from the discharge of untreated sewage. Historically, most combined sewer systems have relied on static controls—such as fixed overflow weirs and off-line storage tanks—to regulate flows within the system. In most cases however, such methods will not meet EPA’s nine minimum control criteria (EPA, 1995b).
To satisfy control requirements of the federal CSO program, while maximizing treatment and minimizing costs, a municipality must rely on the benefits inherent in real-time control (RTC) of the collection and treatment system. RTC modeling is a recent addition to the mathematical modeling toolbox for managing wastewater treatment facilities and collection systems that carry stormwater, whether in a combined system or in a separate stormwater collection system. Real-time control expands the utility of software—for example, through the use of a GIS interface—to coordinate predictive software models with artificial intelligence to “learn” from previous events, predict future events, and control the wastewater system accordingly. Applications include coordination of collection system models with collection system controls, and integration of treatment facility models with instrumentation and control equipment at the plant. Three basic approaches may be employed:
-
Manual control. Conceptually, this is the simplest approach. It requires that an operator remotely control a regulating device (e.g., an influent gate at a WWTP), in response to changing conditions. However, due to the inability of operational personnel to apply this response mechanism in a consistent and timely manner, this approach generally is not feasible for all but the most limited applications.
-
Supervisory control. This approach applies reactive control logic, in which system conditions are monitored and controlled in response to observed conditions, generally without direct operator intervention. If the control logic responds to monitored conditions near the flow control device (directly upstream or downstream of the gate), it is called local reactive control (LRC). If the control logic responds to remote measurement, it is termed extended reactive control (ERC). These supervisory control approaches, LRC and ERC, are the most common types of RTC for sewer systems and offer far greater control than manual approaches.
-
Automatic control. This approach involves the most complex type of RTC and uses rule-based systems (e.g., heuristic, expert system, fuzzy set theory, learning production theory, neural networks) or model-based systems to control system operation. However, the entire approach is collectively termed “optimal global predictive” (OGP) RTC. Although more complex than supervisory control, automatic control via OGP can maximize the capture, storage, and conveyance of combined flow by the sewer system. It simultaneously minimizes the cost of infrastructure improvements necessary to achieve these objectives. For these reasons, OGP provides the most efficient and effective method to meet the nine minimum control requirements of EPA’s CSO program.
Using RTC, the network system has the means to be controlled more efficiently according to system feedback and responding to variations monitored during wet weather events. In this regard, RTC improves the operation of flow-regulating devices by way of automation and makes better use of available capacity, maximizing both the flow to the treatment plant and the use of available storage (in-line and off-line) in order to reduce the volume of CSOs.
It is beyond the scope of this report to provide more specific information for a given wastewater application without a control strategy study and modeling evaluation of network behavior. CSO control goals can also be a significant factor in the ultimate choice in technology. It is strongly recommended that RTC be thoroughly evaluated concurrently with the potential of storage/treatment of CSO in abandoned mines in the region described later in this chapter. Although the future of RTC modeling for managing wastewater appears very promising, the success of such modeling will rely heavily on the accuracy and quantity of input data and the ability to accurately link cause-and-effect relationships. The same type of pipe physical metrics (diameter, slope, roughness, etc.) and flow data are also needed to run these simulations.
Step III—Projection of Future Loadings
Southwestern Pennsylvania is not a rapidly growing or shifting urban area, but neither is it static; some areas of the region are growing while others have declining populations (see Chapter 2). Projections of changes in the regional landscape are important in the planning and implementation of Step III of CWARP. Planning studies at the multicounty/metropolitan scale should be sufficient for this purpose and should include the following:
-
projected transportation infrastructure at appropriate time intervals, perhaps every 5 years, over the 25-year planning horizon for the regional transportation plan;
-
spatial patterns of land use related to the regional transportation plan; and
-
probable patterns of water supply and wastewater disposal for future developments and the effects of alternative urban growth scenarios.
Step IV—Formulation and Evaluation of Alternatives
Steps II and III of the Three Rivers CWARP for high-density urban areas in the Pittsburgh region are preparatory to the formulation, analysis, and evaluation of alternative management strategies for addressing the problems of degraded water quality in receiving streams associated with wet weather discharges. Hydraulic, organic, and biological stresses imposed on receiving waters during wet weather events originate from CSOs, separate stormwater sewer system discharges, and SSOs transported by stormwater runoff. Management strategies should recognize the potential of each of those sources for significant impacts.
Elements of the Strategy. At least six components of a strategy to implement Step IV of CWARP for high-density urban areas should be considered, some of which are mandated under provisions of the Clean Water Act. These include the following:
-
Rehabilitation of separate and combined sanitary sewers to reduce inflow and infiltration
-
On-site measures to reduce rates of stormwater runoff before it enters either a combined sewer, a separate storm sewer, or direct discharge to a receiving stream
-
Segregation of flows in combined sewers into separate sewers in areas where it is cost-effective to do so
-
Real-time control of combined and separate sewers to control rates at which discharges occur and control of inflow rates to treatment systems
-
Storage and treatment of combined sewage and separate stormwater
-
Price incentives and enforcement actions to encourage implementation of the strategy.
1. Rehabilitation of Wastewater Collection Systems: Capacity, Management, Operations, and Maintenance (CMOM) Programs. Monitoring and modeling activities in Step II should be used to support an initial phase of the corrective action plan for urban areas served by sanitary and combined sewer systems. All municipalities and special districts that operate separate sanitary and combined sewers should review and, as necessary, upgrade their operation and maintenance programs to current standards. Much of the existing infrastructure described in this report was built early in the last century (see Chapter 2 for further information). Concern about deterioration of these facilities has led to a renewed focus on their maintenance and orderly rehabilitation or replacement where necessary. CMOM has come to represent a careful plan to maximize the use of existing infrastructure and maintain its utility for as long as possible. CMOM infrastructure assessments are generally undertaken as part of utility plans for control of wet weather overflows. Currently, the federal CMOM program is not codified in federal regulations for controlling SSOs, but a memorandum11 dated April 27, 2002, from the EPA assistant administrator, Office of Enforcement and Compliance Assurance, encouraged the agency to incorporate elements of a CMOM program in actions to address CSOs and SSOs. Elements of a CMOM program include the following:
-
General standards. Properly manage, operate, and maintain all parts of the wastewater collection system; provide adequate capacity to convey base and peak flows; stop and mitigate
11 |
See www.epa.gov/Compliance/resources/policies/civil/cwa.strat312.pdf for further information. |
-
the impact of SSOs; provide notification to parties with a potential exposure to pollutants; and develop a written summary of the CMOM program.
-
Management program. Develop a CMOM program to comply with general standards.
-
Overflow response plan. Develop and implement an overflow response plan.
-
System evaluation and capacity assurance plan. Prepare and implement a plan for system evaluation and capacity assurance if peak flow conditions are contributing to an SSO discharge.
-
CMOM program audits. Conduct an audit, and submit a report of such an audit, evaluating the facility’s CMOM program and its compliance.
-
Communications. Communicate regularly with various interested parties on the implementation and performance of the facility’s CMOM program.
All future CMOM programs will be linked with the enforcement provisions of the Clean Water Act and EPA’s SSO policy,12 which remains under development. This differs significantly from the post-1972 cost-effectiveness analysis procedure established as part of the federal construction grants program because they were linked only tangentially with the enforcement provisions of the Clean Water Act.
As a result of recently signed consent orders (see more below), communities that are served by ALCOSAN will be implementing programs (see Table 5-7) that closely resemble a CMOM program for improving the operation and maintenance of their collection systems (see below). The committee recommends that the first priority for all wastewater collection systems located in the watershed, particularly in the urban core areas of southwestern Pennsylvania, is to be fully compliant with EPA’s CMOM policy or an equivalent program.
As noted in Chapter 1, on October 14, 2003, PADEP sent consent orders to 26 municipalities in Allegheny County to address problems related to CSOs. Furthermore, both the City of Pittsburgh and its Water and Sewer Authority signed a consent order and agreement to address CSOs on February 24, 2004.13 These consent orders set forth two phases for implementing corrective measures. In the first, affected municipalities will have to inventory and evaluate the condition of all sewers in their ownership and repair major defects. In the second phase, the municipalities must install flow monitors and implement operation and maintenance plans. They must also continue to implement the nine minimum controls required under EPA’s CSO policy. The committee recommends that similar actions be undertaken by all other municipalities that contribute sanitary sewer flows to the ALCOSAN system. These actions should be integrated with and supported by the dynamic sewer system modeling discussed earlier.
2. On-site Measures to Reduce Rates of Stormwater Runoff. Implementation of measures required under Phase I and Phase II of the federal stormwater regulations and more complete coverage of authorities under Act 167 should be utilized to reduce rates and volumes of stormwater runoff. Consideration should also be given to identification of high-priority sites where existing runoff could be reduced in a cost-effective manner.
12 |
For further information about EPA’s developing SSO policy, see http://cfpub.epa.gov/npdes/home.cfm?program_id=4. |
13 |
See http://www.dep.state.pa.us/NewsReleases/?ID=2778 for further information. |
TABLE 5-7 Comparison of Consent Order Versus CMOM Requirements for 83 Communities Serviced by ALCOSAN
Basic CMOM Requirements |
Consent Order Requirements |
1. More prescriptive characterization of collection system requiring detailed maps and extensive record keeping |
1. Hire an engineer to prepare a sewer investigation plan |
2. Televising of collection system includes 180 specific questions |
2. Inspect the collection system through physical surveys and TV cameras; conduct dye testing of roof leaders, driveway drains, springs, and catch basins |
3. Asset management program ties value of aging infrastructures and long-term repair costs |
3. Write ordinances and develop enforcement program to eliminate illegal laterals; develop a plan to determine capacity and remediate the problems in the collection system |
4. Requirements of program tied to NPDES permits |
4. Implement the plan and coordinate with ALCOSAN and the 83 communities |
5. Completion dates more long-term and comprehensive |
5. Completion dates not less than 5 years |
As noted in the previous section, the second part of consent orders directed local governments to continue to implement the nine minimum controls required under EPA’s CSO policy. Several of these measures are directed toward reducing stormwater flows entering collection systems. As for CMOM, the committee recommends that similar actions be undertaken by all other municipalities that contribute sanitary sewer flows to the ALCOSAN system.
These measures might also include a wide range of alternative technologies, including urban retrofitting, low-impact developments, and recently considered ecological techniques that may offer potential stormwater flow reductions and are being tested in other communities.
3. Segregation of Combined Sewer Flows into Separate Sewers. In some portions of the combined sewer systems, it may be cost-effective to separate stormwater from sanitary sewage by constructing parallel collection systems. In some instances, construction of separate sanitary sewers may be appropriate. Segregated stormwater could then be treated and discharged through decentralized treatment systems, including wet and dry detention basins and other technologies discussed in later sections of this chapter. In some cases, the quality of stormwater runoff may be sufficient to allow rerouting of segregated stormwater through open channels with direct discharge to streams.
4. Real-Time Control of Combined and Separate Sewers. The use of RTC of flows in separate sanitary and combined sewers and stormwater conveyance systems should be investigated and evaluated as a means to reduce peak loading on centralized and decentralized treatment systems. The October 2003 consent orders required affected municipalities to install
flow monitors. Those monitors should be integrated into a system-wide, real-time monitoring and control system.
5. Decentralized and Centralized Storage and Treatment. After initiatives have been taken to reduce peak rates and volumes of flow and after investigating the potential for real-time controls to maximize storage in existing collection systems, consideration should be given to decentralized and centralized options for storing and treating flows in combined sewer and separate stormwater sewer systems. Decentralized storage could be used in conjunction with real-time flow control to reduce peak flow, resulting in fewer CSO discharges and reducing the loading on ALCOSAN’s treatment plant. Reduced loads on that plant could result in reduction in the size of planned expansions needed at the central WWTP. In some instances it may be possible to treat and discharge stormwater to nearby streams before it enters combined systems.
During the course of the committee’s deliberations, various technologies and approaches (including some discussed in ALCOSAN’s draft LTCP and the TPRC report) were discussed to determine what, if any, role they might play as remedial actions for high-density urban areas of the watershed. All of these technologies could be characterized as innovative because they are still in the developmental stage at the national scale; others may have proven to be effective in other locations but cannot prudently be designed for effective operation without site-specific performance data. In this regard, the committee recommends at least the following technologies and approaches for consideration in the planning and implementation of Step IV of CWARP for the region’s high-density urban core:
-
CSO storage and treatment in local underground, abandoned mines;
-
vortex separators;
-
ballasted flocculation; and
-
in-river CSO storage using the flow balancing method.
There is a need to develop further information on the feasibility, performance, and costs of these technologies via prototype or full-scale studies. For example, uncertainties in the hydraulic performance of vortex separators could be investigated through the construction of large-scale physical hydraulic models or full-scale field (demonstration) units located in critical CSO control locations. Each of these innovative technologies or approaches is discussed later in this chapter, including the potential adoption of wet weather water quality standards, to help improve water quality in southwestern Pennsylvania.
6. Pricing and Enforcement Incentives. In addition to the technical strategies suggested, it is strongly recommended that pricing and enforcement incentives be adopted. Some of the municipalities contributing flow to the ALCOSAN system have contracts that prescribe pricing of services in proportion to flow; others have contracts that allow them to discharge to ALCOSAN for a fixed fee regardless of flow. The latter practice should be phased out, and consideration should be given to peak load pricing as well as volumetric pricing as recommended and discussed in Chapter 6. Any new pricing policies should be developed using concepts of marginal costs of providing the services. In addition to pricing incentives, enforcement of stormwater Phase I, Phase II, CMOM, and CSO regulations should be an active part of the
strategy. Without effective periodic inspections and enforcement, the regulations can be easily ignored. Alternative organizational arrangements for the establishment and enforcement of these regulations are also discussed in Chapter 6.
Step V—Adaptive Implementation of Strategy
Successive steps for implementing CWARP in high-density urban areas should be implemented in an adaptive manner. Before each step is implemented, the best available monitoring, modeling, predicted effectiveness, and cost information should be used to guide its design and implementation. After implementation of each step, monitoring and modeling should be updated to incorporate information obtained from implementing that action, and revised predictions of performance and cost should be used to modify designs for the next step. A graphical representation of such a process is provided in Figure 5-4.
Rural Area Planning and Management
Step I—Problem Identification.
Testimony before the committee from several persons throughout the course of the study cited numerous problems of inadequate water supply and waste disposal in rural areas. The most frequently cited issue was failing OSTDSs and straight-pipe discharges from individual homes, and even entire communities, to nearby streams. Although no systematic survey data were available, these anecdotal accounts were compelling. Given the large number of OSTDSs in southwestern Pennsylvania and the nature of soils in the region (see Chapter 2), a conclusive finding that widespread failures were occurring would not be surprising.
Nonpoint sources of pollution that contribute fecal bacteria to surface waters can be difficult to identify. In evaluating potential pathogen and indicator microorganism sources in watersheds with multiple nonpoint sources, investigators and watershed managers must be careful to avoid biases toward implicating any single source without sufficient data to support such conclusions. To target the most critical public health and environmental needs, to most effectively utilize the funding available for water quality improvements, and to avoid unwarranted financial burdens on private individuals, farms, and businesses, it is crucial to determine the major sources of microbiological contamination in a watershed and to prioritize efforts, funding, and/or user costs accordingly. Many rural watersheds may have several potential nonpoint sources of pathogens and indicator microorganisms, including single-home domestic wastewater, livestock, pets, wildlife, and biosolids (manure and treated wastewater sludge). All of these sources can contribute to microbial loading, although in some watersheds a particular source or sources may dominate.
The following sections describe how the CWARP process can be used to improve water quality in the predominantly rural areas of southwestern Pennsylvania.
Step II—Assessment of Existing Conditions
It is advantageous to use the approaches practiced in wellhead and source water protection, identifying the nature and spatial locations of microbial contaminant sources. As indicated in the Mud River case study (Box 5-3), it is not sufficient to sample only the main stem or downstream reaches of a stream; water quality samples and land use analysis in tributaries and headwaters are also needed for thorough watershed analysis. In some instances, more detailed, targeted monitoring and analysis of specific stream reaches may be needed.
In recent years, watershed scientists also have used a variety of techniques to attempt to track specific sources of bacterial contamination; some of these methods are based on microorganisms themselves, others rely on distinct chemical “identifiers” of specific sources. Box 5-4 discusses two cases of the successful application of microbial source tracking techniques in Virginia. Some of the techniques are costly, some require refinement and additional validation, and some are still considered experimental (see NRC, 2004a). It is important to note that none of them should be considered stand-alone methods; rather, they should be regarded as tools to be used in conjunction with other careful watershed analyses of use impairment. These two examples of the use of microbial source tracking in Virginia watersheds illustrate the importance of determining the dominant source of fecal indicator organisms.
Although there is no reason to doubt anecdotal data of the extent of OSTDS failure in parts of southwestern Pennsylvania, the extent of the problem and the location of concentrations of failures that pose the most serious problems cannot be determined without systematic assessments. Pennsylvania Act 537 requires registration of all septic systems since that law was passed, but it does not require registration of previously installed systems.
The committee recommends that best management practices for OSTDSs should be implemented throughout southwestern Pennsylvania within the CWARP framework, along with the following related actions:
-
within each county, register all individual on-site and cluster OSTDSs with the appropriate SEO; registration should include residences, houses of worship, schools, business establishments, and other locations;
-
institute a program of periodic mandatory inspection and certification (or decertification), either by a public entity or a qualified/licensed private contractor;
-
conduct statistically valid surveys of septic tank and absorption field conditions, residence by residence, to identify communities that should be given high priority for funding by PENNVEST (Pennsylvania Infrastructure Investment Authority) or the Rural Utilities Service (RUS) of the U.S. Department of Agriculture for remediation of failed and failing OSTDS throughout the region.
Step III—Projection of Future Loadings
Data gathered from Step III of CWARP for rural areas should be used to identify areas of high concentrations of OSTDS failures and their potential impacts on public health and the water quality (especially microbiological) of nearby receiving streams. Failure rates alone may provide an adequate indicator of potential public health effects. Modeling and monitoring may be necessary to determine impacts on receiving streams into which OSTDS drainage may be discharged.
Step IV—Formulation and Evaluation of Alternative Management Strategies
Although individual OSTDSs are permitted locally and current technical standards are available to ensure proper performance, they may be ignored. Furthermore, prevention of the discharge of untreated sewage into local waterways or ditches is difficult to enforce. The region needs a coordinated, well-funded program for oversight and routine maintenance of OSTDSs. Such a program can be self-sustaining through user charges, provided they are applied on a cooperative regional or county basis (see also Chapter 6). Such a registration and inspection program should also be used to identify and order elimination of illegal direct discharges of human waste into streams and to identify areas where cluster OSTDSs may be feasible. Several examples of approaches to these kinds of rural water quality problems could be reviewed and cited; one successful program is summarized in Box 5-5.
These recommendations are consistent with a detailed guidance published by the EPA in 2003 that provides voluntary national guidelines and a draft of a handbook for managing on-site or clustered wastewater treatment systems in rural areas (EPA, 2003a). Basic elements of a management program are listed in Box 5-6. EPA guidance suggests alternative models of the “who and how” of wastewater management, depending on the complexity of appropriate wastewater treatment systems and the nature of the risk they pose to human health and the environment (EPA, 2003b). Those models are summarized briefly in Box 5-7.
Step V—Adaptive Implementation
Wastewater treatment systems (e.g., cluster OSTDSs) for sparsely populated rural areas of southwestern Pennsylvania should be designed and implemented over a time horizon that allows for evaluation of outcomes of initial remediation projects before subsequent projects are undertaken. Lessons learned from initial projects should be used to modify later projects in the schedule.
Economic Evaluation of Strategies and Projects
The CWARP process can yield a list of alternative management strategies and projects that are technically feasible and capable of addressing the region’s water quality problems at a variety of scales, but the question remains: Which is the better option? Comprehensive evaluation of options under CWARP (especially Step IV for high-density urban areas) requires
BOX 5-3 In Lincoln County, West Virginia, the Mud River’s Left Fork—a small headwaters watershed in the Guyandotte River watershed—illustrates the critical information gaps that may exist when bacterial water quality sampling is confined to locations on a main stem river. In such cases, the nature, distribution, and sources of microbial contamination throughout the watershed may be poorly understood, leading programs for water quality improvement to target the wrong areas or sources or to achieve only partial reduction of contamination. Because the Left Fork’s land uses and terrain resemble those of many isolated rural watersheds throughout southwestern Pennsylvania, its watershed may serve as an example of the severity and complexity of localized bacterial contamination that can affect smaller, upstream tributaries in that region. The watershed of the Left Fork of the Mud River includes headwaters streams that are part of the much larger watershed (1,680 square miles) of the Guyandotte River, a tributary to the Ohio River at Huntington, West Virginia. The Mud River joins the Guyandotte in its lower reaches (approximately 20 miles east of Huntington), and the lowermost Mud River lies within this urban-suburban-industrial corridor. Similar to southwestern Pennsylvania, the geology of the Left Fork watershed comprises flat-lying or gently tilted layers of Pennsylvanian age sandstone, shale, clay, coal, and limestone. Terrain consists of steeply sloping hills and narrow valleys. The area is sparsely settled and dominantly forested, with scattered homes, small farms, and several small, unincorporated communities. In the late 1990s the U.S. Army Corps of Engineers constructed a dam at the confluence of the Left Fork and the main stem of the Mud River; the resulting Upper Mud River Lake is managed by the West Virginia Department of Natural Resources (WVDNR) as a fishing and recreational lake. Lincoln County maintains a youth camp and multipurpose recreational area on the lake’s shoreline near the mouth of the Left Fork watershed. West Virginia’s 1996, 1998, and 2002 Section 303(d) lists (see Chapter 3 for further information) included 123 impaired waterbodies within the Guyandotte watershed. Impairments included pH, iron, manganese, selenium, fecal coliforms, and biological impairment. TMDLs were completed in March 2004 for the Upper and Lower Guyandotte hydrologic unit codes (HUCs). Of the 123 waterbodies in the Guyandotte watershed, only the main stem Guyandotte is listed as impaired due to excessive fecal coliforms and sources are listed as unknown. Although individual grab samples for coliform were collected throughout the watershed during May 1998, only the main stem had a sufficient number and seasonal range of samples to support the 303(d) listing. Examination of WVDEP’s grab sample values throughout the watershed indicates that most of the extremely high fecal coliform counts (in the thousands per 100 mL) were obtained in more densely developed urban-suburban areas with WWTPs, CSOs, and urban runoff, but several areas of extremely high fecal coliform values are located in very rural subwatersheds tributary to the Mud River. One of these subwatersheds is the Left Fork headwaters, where three grab samples collected in May 1998 had fecal coliform counts of 5,000, 5,200, and 2,900 per 100 ml (EPA, 2004). During 1998, water quality in the Left Fork and Upper Mud River Lake raised concerns locally. High fecal coliform counts caused numerous closings of WVDNR’s public beach on the lake, and |
additional considerations, including costs, benefits, and fairness. It continues throughout the process of selecting a preferred long-term management strategy for a particular water quality problem at given scale. It is essential in the formulation of alternatives to provide feedback as to how initial designs have to be modified or discarded in the search for a cost-effective strategy. It is also essential to the process of establishing priorities among the several elements that may comprise the management strategy. Finally, it must be a continuing process during implementation to evaluate how well each element has performed. A variety of evaluation frameworks are available; some of the more prominent are discussed below.
water in the lake near the youth camp on the Left Fork suddenly acquired a reddish color for a short period of time. As a result of these concerns, a water quality investigation was undertaken with funding from the Service Learning Program at West Virginia University (WVU). In collaboration with the Lincoln County WVU Extension Office and science students from two local high schools, professors and students from WVU sampled 53 locations in the Left Fork watershed during April 1999 to April 2000. At 42 of the locations (79 percent), samples exceeded the West Virginia State Board of Health’s one time sample total coliform limit of 2,400 and at 16 locations (30 percent) samples exceeded the one time sample fecal coliform limit of 400 for primary contact recreation. During April 2000, the fecal coliform standard was exceeded in most of the watershed. Counts were exceptionally high (many in the tens of thousands) during a high-flow sampling in that month. Very high values were also obtained at clusters of houses. In one small tributary with no houses, several samples exceeded standards, but no reason for the high counts was determined. In a subsequent investigation of possible links between watershed bacterial contamination and septic systems (presence, condition, and maintenance), the investigating team examined county health department and National Small Flows Clearinghouse survey information. Notably, of 8,000 homes in the county, 7,000 have on-site septic systems. Failure rate is estimated to be 50 percent, and repairs are common due to system age, inadequate size, impermeable or saturated soils, damage to the system, inadequate removal of surface water, and improper maintenance. The team conducted a survey of 77 of the 250 households in the watershed. While 90 percent of residents reported that a septic system handled all (76 percent) or part (14 percent) of their wastewater, about 50 percent did not know the size or condition of their system, and only 30 percent had performed any maintenance on their systems. However, 85 percent of the respondents perceived that few problems exist with their systems, and 80 percent perceived no effects on their water wells, neighbors’ water wells, groundwater, or surface water. Local health department records indicate that in the 12 years prior to the study, only 2 permits for septic systems and 6 permits for home aerobic units had been issued, and the team surmised that many households either had systems constructed without permits or had little actual knowledge of the wastewater system (if any) serving their homes (Collins et al., 2000). Using that study as a basis for a proposal, in 2003, Lincoln County and the WVU Extension Service were awarded a grant from EPA for a collaborative demonstration project involving a detailed, comprehensive study of bacterial contamination in the Left Fork and installation of alternative on-site wastewater treatment technologies. Although the earlier study of the watershed suggested strong links between inadequate household wastewater treatment and bacterial contamination in the watershed, the investigators did not specifically examine the possible role of other bacterial sources. As part of the EPA demonstration project, preliminary examination of land uses in the watershed, using aerial photographs and field surveys, indicates several areas where cattle are confined immediately adjacent to a tributary stream or where a small tributary flows through a farm’s feedlot. In addition, noticeable concentrations of Canada geese waste were observed at several locations on the shore of the lake. These observations, as well as the previous study’s “anomalous” tributary, suggest the need for thorough investigation of sources and careful documentations of household wastewater-related contributions, to effectively prioritize and correct watershed contamination. |
Cost-Effectiveness
Cost-effectiveness analysis ranks projects based on their relative costs in achieving a specified outcome. Essential to this analysis is consideration of long-term costs of alternatives that may have varying useful lives and the actual improvements expected to be achieved. The term of the analysis should be 20 or more years, and appropriate criteria should be used to consider inflation and the time value of money. Economic efficiency (cost containment) is a
BOX 5-4 Water quality monitoring in the Page Brook watershed in Clarke County, Virginia, exhibited use impairment due to excessive concentrations of fecal coliforms. Land uses in the watershed suggested two likely nonpoint sources of fecal contamination: (1) relatively widespread livestock (cattle) farming and (2) widespread use of single home septic systems. Routine water quality surveys in this watershed were unable to determine the relative contributions of these two sources. To field test a microbial source tracking method using patterns of antibiotic resistance in fecal streptococci, Hagedorn et al. (1999) initiated a watershed improvement project in 1996. Application of the antibiotic resistance method at three highly contaminated sites within the watershed identified the dominant source (79 percent of the isolates) as cattle, with small proportions of waterfowl, deer, and unidentified sources. Based on these results, cattle access to the stream was restricted through BMPs (installation of fencing and in-pasture watering stations). Fecal coliforms were subsequently reduced at the three sites by an average of 94 percent, from pre-BMP average populations of 15,900 per 100 mL to post-BMP average populations of 960 per 100 mL. After fencing, less than 45 percent of fecal streptococcus isolates were classified as being from cattle, indicating that the dominant source of contamination had been successfully remediated Septic systems were suspected as a major source of fecal coliform impairment contamination of shellfish beds at a tidal inlet on Virginia’s Eastern Shore. Using shoreline survey techniques and discrete sampling over small areas, Simmons et al. (1995) tracked nonpoint sources of fecal coliform at tidal inlets on Virginia’s Eastern Shore and at an uninhabited island. Based on DNA fingerprinting methods, they characterized Escherichia coli from raccoon, goose, otter, and muskrat. These results, along with examination of land use and wildlife management patterns in the area, led them to conclude that fecal contamination of tidal inlets, bays and estuaries on Virginia's Eastern Shore could be largely attributed to fur-bearing animals. The populations of these animals have increased over the past several decades due to lack of predation and land development patterns that concentrate their populations in undeveloped shoreline areas. Simmons and colleagues also determined that septic systems, when sufficiently elevated above the water table and not mechanically damaged or overloaded, are effective at removing E. coli, thus avoiding the need for costly replacement systems that would likely have had minimal impacts in reducing fecal contamination. |
serious issue in the region, given that resources for water quality protection and other public objectives are scarce. In colloquial terms, cost-effectiveness analysis is about getting the “biggest bang for the buck.” Simply stated, if two projects yield the same outcome, the preferred project is the less expensive one. Cost-effectiveness is particularly useful for optimizing theachievement of a well-defined policy target. In this context it is used to identify a single project or set of projects that minimize the costs of achieving the target. The use of cost-effectiveness analysis for ranking projects becomes limited when the outcomes are not comparable.
Benefit-Cost Analysis
Benefit-cost analysis ranks projects based on the relationship between benefits and costs. The economic benefits of measures to reduce or eliminate discharges of untreated sewage into the region’s source waters would include the reduction in the likelihood of such events and the associated costs of averting activities and disease. Other things being equal, desirable projects yield benefits in excess of costs, with projects being ranked according to their relative net benefits. Benefit-cost analysis is more powerful than cost effectiveness analysis for project evaluation because it allows for comparison of projects with otherwise noncomparable outcomes.
BOX 5-5 Water quality improvements in southwestern Virginia’s Guest River watershed provide an excellent example of a successful rural watershed partnership between grassroots community groups and government agencies. Draining approximately 100 square miles of Appalachian Plateau in Wise County, Virginia, the Guest River watershed is a tributary of the Clinch River, which is in turn part of the larger Tennessee River basin. Environmental, economic, and social issues in the watershed are typical of those affecting many rural, coal-mining-impacted communities in the central Appalachians. Although the watershed’s rugged terrain, coalfield history, and rural Appalachian folk life offer potential for tourism and recreation development, contamination of the watershed’s streams, as well as inadequate water and sewage service, creates barriers to attracting new economic development. In 1995 several groups of watershed residents and more than 15 local, state, and federal government agencies formed the Guest River Group, an informal coalition dedicated to the protection and restoration of the Guest River watershed. Efforts of this group have led to the development of the Guest River Restoration Project, an integrated program of multiple projects to address a variety of pollution sources for the entire watershed. Projects have included septic system pumpouts and inspections, repairs, and replacements; illegal solid waste dump cleanups; stream bank restoration and slope revegetation; porous paving projects; abandoned mine cleanups; and outdoor classrooms for the school system. Financial support for the Guest River Restoration Project has come from Clean Water Act Section 319 grants; Virginia Water Quality Improvement Fund grants; EPA’s National Onsite Demonstration Project (NODP); and other local, state, and federal sources. Elimination of sewage problems is a major part of the Guest River Restoration Project. Typical of many small communities in the central Appalachian coalfields, the former coal camp of Imboden had community sewers leading to two “community straight pipes” on a small tributary stream and limited land available for conventional septic systems. Because of its rugged topography and relative remoteness from other communities, Imboden had no realistic possibility of connecting to any existing municipal sewage systems. With a combination of NODP funds, local funds, and in-kind contributions, the community has completed a “cluster system” consisting of new septic tanks (two households per 1,500 gallon tank), small-diameter sewers, and a central treatment system consisting of a recirculating textile filter and a community drainfield. Approximate cost (including in-kind worth) per household was $7,000. The municipal sewer utility in the town of Appalachia will provide system management for operation and maintenance. For further information, see Clean Water Action Plan Partners (2000). |
BOX 5-6
SOURCE: EPA, 2003a. |
BOX 5-7 Management Model 1 “Homeowner Awareness”
Management Model 2 “Maintenance Contracts”
Management Model 3 “Operating Permits”
Management Model 4 “Responsible Management Entity (RME) Operation and Maintenance”
Management Model 5 “RME Ownership”
SOURCE: EPA, 2003b. |
In the context of the Pittsburgh region, projects with comparable outcomes would be those that produce essentially the same impacts on water quality for all water quality criteria. However, many feasible projects may fare better by some water quality criteria than by others. If multiple water quality criteria are important, projects with differing water quality outcomes may not be comparable for the purposes of cost-effectiveness analysis. Benefit-cost analysis would allow comparisons between such projects. Further, benefit cost-analysis would allow inclusion of ancillary benefits if any. Importantly, to be useful in setting priorities for projects and policies, estimates of benefits and costs must be tied to the water quality gains from specific initiatives.
Although different techniques of varying data intensiveness exist to estimate the benefits of reducing the risks of waterborne disease, essential information is lacking for estimating the relationships between CSO control and the likelihood and severity of contamination events. For example, a key question that cannot be answered with current data on sources of pathogens and the effectiveness of prospective control measures (see also Chapter 4) is the extent to which the likelihood of contamination events in various systems will be diminished. Recent work by Casman et al. (2000) illustrates an approach to modeling drinking water risks that would offer a good starting point for systematic analysis of risk management in the region. Further, for the
purposes of drinking water protection, consideration of the relative cost-effectiveness of different strategies merits attention. For example, to what extent should limited financial resources be allocated between water system upgrades and source water protection in the region?
The use of cost-effectiveness as the primary method of evaluating options for achieving water quality objectives in the region is recommended. It should include an analysis of incremental costs to achieve elimination of low-probability contamination events. The committee also recommends the use of benefit-cost analysis in the evaluation of water quality improvement projects in the region, and in helping to set priorities. Although “state-of-the art” studies can be very expensive, it may be possible to gain useful information using “lower cost” methods. For example, “benefits transfers” is a method for estimating benefits of environmental quality improvements using results from other regions. The EPA’s (2000b) Guidelines for Preparing Economic Analyses describes methods and procedures for cost-effectiveness and benefit-cost studies.
Multicriteria Methods
Decision makers in the region may want to apply criteria beyond long-term cost-effectiveness and benefit-cost analysis to project evaluation. They may also have interest in metrics of performance beyond costs, benefits, or water quality indicators, such as equity, social justice, and other social objectives. To the extent that this is the case, multicriteria methods offer tools for systematic evaluation of water quality improvement projects and related decisions when there are multiple competing objectives. A brief introduction to the array of methods is presented in Janssen and Munda (1999).
TMDL PROCESS FOR SIGNIFICANT CONTAMINANTS
As the CWARP process is being implemented, it is essential that it be integrated with the ongoing process of establishing total maximum daily loads (TMDLs) for impaired streams being conducted by PADEP under requirements of the Clean Water Act. There are many parallels between CWARP and the process for establishing TMDLs. A TMDL defines the pollutant load that a waterbody can assimilate without causing violations of its water quality standards. The term TMDL also refers to a plan for those waters in violation of their water quality standards, in which the excess pollutant loading is allocated between contributing point sources and nonpoint sources and subsequent actions are taken to control and eliminate these excesses.
Although the TMDL program originated from the Clean Water Act (Section 303d), it was largely overlooked during the 1970s and 1980s as states focused on controlling point sources of pollution through compliance with NPDES permits (NRC, 2001). Beginning in the 1980s, citizen lawsuits forced EPA to develop guidance for the TMDL program, which is now considered pivotal in achieving the nation’s water quality goals.
The NRC (2001) review of the scientific basis of the TMDL program recommended that the program meet certain objectives, some of which are also an integral part of the CWARP approach recommended in this report. These objectives include the following:
-
improve the condition of waterbodies as measured by attainment of designated uses;
-
encompass all stressors that determine the water quality conditions of the waterbodies;
-
develop appropriate use designations for waterbodies prior to TMDL development;
-
apply adaptive implementation consisting of monitoring, modeling, design, and construction of facilities followed by further monitoring to assess whether expected improvements were attained;
-
utilize biological criteria, in conjunction with physical and chemical criteria, to determine whether a waterbody is meeting its designated use, and define all chemical and some biological criteria in terms of magnitude, frequency, and duration of exceedences of criteria; and
-
utilize reasonably obtainable monitoring data to assess attainment of water quality standards.
As shown in Figure 5-5, “adaptive implementation” is a cyclical process in which TMDL plans are assessed periodically for their achievement of water quality standards, including designated uses. If the implementation of a TMDL plan is not achieving attainment of the designated use, scientific data and information should be used to revise the plan. Adaptive implementation is needed to ensure that the TMDL program is not halted because of a lack of data and information, but rather progresses while more and better data are collected and analyzed with the intent of improving upon initial TMDL plans. Adaptive implementation is an important concept in CWARP at all scales of its planning and implementation.
The TMDL process recommended in the 2001 NRC report—supplemented by additional analyses of constituents that may not be readily subject to the rigorous TMDL approach, including biological, environmental, and other measurable factors—should be combined with watershed, regional, and subregional analysis of beneficial uses to provide the basis for selection of remedial actions in the study area. Table 5-8 summarizes the current schedule of TMDLs to be completed in Pennsylvania, divided into AMD and non-AMD categories.
IMPLEMENTING CWARP
The CWARP effort should be completed quickly to provide timely support for those water quality improvements that are required and others deemed in the public interest. It is difficult to estimate the cost of implementing CWARP, but in the committee’s judgment it should be low compared to the cost of improvements and more than offset by potential savings. It is important to reemphasize that CWARP is an adaptive implementation process. Large tasks and responsibilities to be started first in the multiyear program include the following:
-
advance plan and design approach for the construction of pilot and/or demonstration vortex separators in the ALCOSAN system;
-
implement CMOM requirements for all wastewater collection systems in the watershed, especially in the high-density urban areas of southwestern Pennsylvania;
-
plan and implement the monitoring and modeling components of CWARP; and
-
begin feasibility studies for innovative technologies and approaches.
In addition, the major effort to better define and implement the institutional regionalization needed to manage CWARP at all scales of implementation as described in Chapter 6 should proceed immediately.
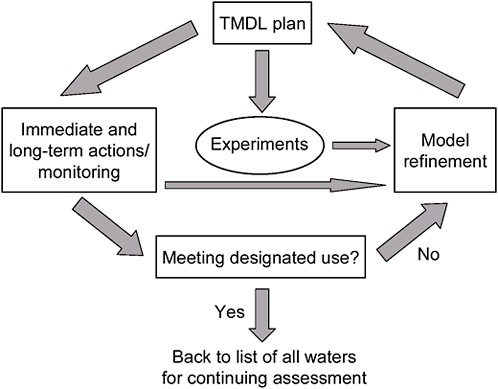
FIGURE 5-5 Adaptive implementation flow chart for TMDL plans in southwestern Pennsylvania under CWARP. SOURCE: Adapted from NRC, 2001.
TABLE 5-8 Schedule of TMDLS to Be Completed in Pennsylvania Through 2009
Year |
Non-AMD |
AMD |
2005 |
30 |
85 |
2007 |
30 |
85 |
2009 |
— |
169 |
SOURCE: Adapted from PADEP, 2004. |
Lastly, ALCOSAN’s draft LTCP for controlling CSOs should be reevaluated in the context of the overall CWARP approach to reflect ongoing consent order negotiations, CMOM, and information from CWARP as it is developed in the future. The CWARP approach is recommended as a framework for development of the LTCP and similar documents because of the circumstances (especially data limitations discussed throughout this report) that exist in southwestern Pennsylvania and, in principle, would apply to other regions of the United States with similar water quality problems and circumstances. The implementation of CWARP, the utilization of real-time control of CSOs, the possibility of CSO storage and treatment in nearby abandoned mines, and other innovative technologies and approaches discussed in the following section, could potentially result in reducing the need to expand existing wastewater treatment plants. As a result, the costs and time factors associated with these activities should be evaluated to ensure that potentially unneeded investments are not made.
INNOVATIVE TECHNOLOGIES AND APPROACHES
As noted previously, there are at least five innovative technologies and approaches to water quality improvement in southwestern Pennsylvania, especially for addressing wet weather water quality problems in the region’s urban core areas. Although not specifically recommended, the committee believes these should be explored, and each is discussed below.
CSO Storage and Treatment in Underground, Abandoned Mines
One possibility for reducing the cost of CSO collection and treatment is to take advantage of existing, abandoned caverns from past coal mining activity to store very large volumes of CSO for subsequent treatment at a remote location where inexpensive land is plentiful. This innovative approach to CSO abatement could potentially allow for minimum disruption of surface infrastructures, high reliability and availability, reduced maintenance and operational costs, and large and easily expanded capacity. Potential advantages of this concept include minimizing concerns with existing inflow and infiltration, no need for conveyance to the ALCOSAN plant, and expansion of decentralized treatment facilities to handle short-term high flow rates.
It is important to note that a feasibility study for short-term CSO storage during wet weather in abandoned coal mines in the Pittsburgh region is currently being conducted under the sponsorship of the Township of Upper St. Clair (Gateway Engineers, Inc., and GAI Consultants, Inc., 2003). The evaluation is focusing on the Lower Chartiers Creek watershed, with specific emphasis on the possibility of temporary mine storage of overflows from the McLaughlin Run interceptor. The stored combined sewage, together with the acid mine drainage with which it would become mixed, would be pumped to the surface and sent by interceptor to the ALCOSAN treatment plant for subsequent processing and discharge to the Ohio River. The project goals include determination of the feasibility of the proposed technology with respect to performance criteria and regulatory requirements, specifically the following:
-
mining and geotechnical issues, including mine subsidence, mine sealing, mine storage capacity, and mine ownership;
-
gas generation and health and safety issues, including an evaluation of the potential for gas generation, migration, and odor control;
-
water quality impacts, including the potential effects of sewer overflow storage on surface and groundwater quality and interaction with mine water;
-
treatment system requirements and impacts, including long-term generation of solids within the mines and removal requirements, and impacts and requirements for the existing collection and treatment system for handling the wastewater after the end of the wet weather event;
-
hydrologic and hydraulic analysis, including evaluating inflow to the mine during wet weather and overflows and groundwater seepage;
-
economic analysis including life-cycle costs; and
-
legal and institutional analysis addressing requirements for construction, operation, and administration.
An even closer look into the potential for acid mine storage of CSOs is warranted. As discussed in Chapter 2, the Pittsburgh Coal Seam has been extensively mined in southwestern Pennsylvania for decades and offers a potential set of appropriate “reservoirs.” It is a vast, 1,600 square mile elliptical basin with the northern end of its long axis ending in Pittsburgh and the southern end in Charleston, West Virginia. The lowest point of the basin is near sea level and is reached in northern West Virginia. The Pittsburgh Seam outcrops along the hills immediately south of the Monongahela and Ohio Rivers. The seam dips below the elevation of surface streams around Cannonsburg, Pennsylvania.
For illustrative purposes, acid mine storage and treatment of CSOs could be attempted in the Montour #4 coal mine located about 10 miles south of Pittsburgh, where mining ceased in the 1980s. Since that time, the mine has been allowed to flood to within 30 feet of the base of Chartiers Creek near Cannonsburg, Pennsylvania. Its water level is maintained by Consol Energy’s pump and treatment plant along Chartiers Creek, which is pumping the mine at the rate of 5.5 million gallons per day. Peak CSO discharges in the region are estimated to be about 400 mgd. The flooded area of Montour #4 is about 15 square miles, providing about 8 billion gallons of void space; it is surrounded by other, similarly situated mines (see Figure 5-6). Montour #4 produces net alkaline water with about 70 mg/L of ferrous iron that is treated with aeration and iron flocculate handling.
To store CSO flows in abandoned mine voids, CSOs would discharge into a series of room-and-pillar main headings developed in the underlying Freeport Coal Seam along the main rivers, with laterals collecting additional CSOs as needed. The headings could be constructed similarly to main haul ways in coal mines with heavy unmined coal pillars designed to prevent subsidence. There is an existing Freeport Coal Seam tunnel under the Allegheny River at Springdale, Pennsylvania, indicating good roof conditions and precedence for tunneling under the main rivers in the Freeport. The mains could be constructed using conventional mining equipment and would be three to four entries wide to provide access, ventilation, and conveyance of the mined coal. The extracted coal could be marketed, to help offset development costs.
The preceding strategy might meet design criteria at costs likely to be significantly lower than surface storage and treatment because the excavation is already done and the need for multiple pump stations is greatly reduced due to the availability of gravity flow. It is recommended that an expert panel be established to carefully evaluate and further assess the feasibility of this type of approach to CSO abatement. The scenario outlined above would have to be subject to intensive review and, if feasible, designed by mining and civil engineers as well as tested in a pilot or demonstration project before application. Further details of coal seam CSO storage are provided in Box 5-8.
Vortex Separators
One remedial option for treating mixed rainwater and sewage is to use vortex separators, which target the settleable portions of the fecal matter. Vortex separators are designed to screen and remove all settleable solids from any flows that exceed WWTP capacity during precipitation events, including bacterial solids. They are cylindrical tanks that allow water to enter tangentially and move in a centrifugal fashion before exiting. The centrifugal motion forces suspended particles to the center of the device, where they settle to the bottom. In addition to concentrating settleable solids, vortex separators also provide flow regulation.
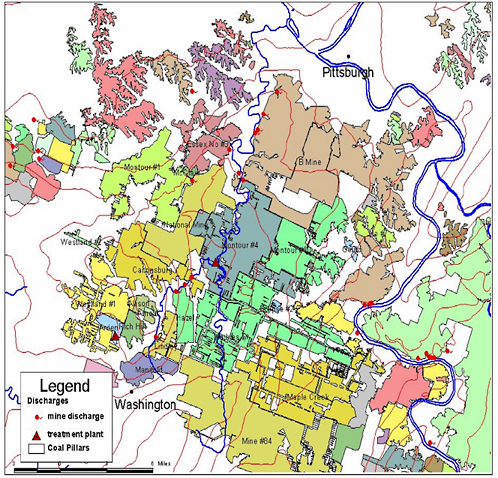
FIGURE 5-6 Pittsburgh seam coal mines south of the City of Pittsburgh.
NOTE: Montour #4 is in the center of the map; only Maple Creek and Mine #84 are still active.
There is currently no consensus opinion regarding the effectiveness of vortex separators. One advantage is that they do not typically require a lot of regular operator attention. In addition, although preliminary expenses are high, operation and maintenance costs are low. Vortex separators can be coupled with chemical disinfection (e.g., chlorine, sodium hypochlorite), which increases the separators’ overall effectiveness at meeting water quality standards for microbial indicators. Finally, they have no moving parts except for pumps to remove settled solids.
Unfortunately, removal efficiencies of the settleable solids are not as high with vortex separators as with other available technologies. Vortex separators are good at removing grits and heavier particles, but not as effective at removing lighter particles. For example, they provide 5-15 percent net BOD (biological oxygen demand) and TSS (total suspended solids) removal, as opposed to enhanced high-rate clarifiers, which remove 80-90 percent of BOD and TSS.
BOX 5-8 A typical, large underground coal mine in the Pittsburgh Basin is between 10 and 30 square miles in area. On average, the Pittsburgh Coal Seam is 6 feet thick. In southwestern Pennsylvania, between the Monongahela and Ohio Rivers, about 50 percent of the surface area is underlain by worked-out underground coal mines. After mining, much of that area will consist of collapsed roof rock that will form a “caved zone” up to 20 feet above the mine floor. Although exhibiting significant porosity, the caved zone will consist of large, broken fragments of shale from several inches to many feet long. Parts of the mine, such as main transportation corridors and ventilation entries, will be protected by large, intact coal pillars. In these areas, there may be little or no roof collapse decades after mining ceases. The estimated void volume of this mined area is 8 billion gallons. Groundwater entering the mine during operations is currently pumped to the surface and treated for release to a stream. Mines that are below the deepest point of the surface landscape will completely fill with groundwater, a process that is generally complete 5 to 10 years after pumping stops. Initially, the mines fill with strongly acidic water (pH ~2.7, acidity up to 2,500 mg/L), with high concentrations of Fe+2 (up to 1,000 mg/L) and SO42- (up to 3,000 mg/L). After flooding, this water is gradually replaced with net alkaline water (pH~6.3, alkalinity up to 600 mg/L). Fe+2 declines to between 20 and 100 mg/L, while SO42- will remain greater than 1,000 mg/L. The shift in acid-base balance is accompanied by a transition from oxidizing to reducing conditions in the mine water. Flooded mines will have a very large though finite capacity to store the water and leakage of groundwater into the mine will force an equal amount out at a rate that correlates with depth of the mine. Any additional water will accelerate the rate of discharge. The current excess stormwater flow for the Pittsburgh region is estimated to be a high of 400 mgd. The estimated total overflow volume is 11.5 million gallons per year, which is equivalent to an average flow rate of 32 mgd. If all of the excess stormwater was diverted into this abandoned mine, the storage time would be about 0.7 years. Using abandoned, underground mine voids for storage and treatment of sewage will require resolution of several complex issues—regulatory, financial, and technical. The chemistry itself will be complex and largely biologically controlled. In its simplest form, the addition of small community sewage to a flooded, anoxic mine pool will involve the following chemical reactions: sulfate reduction to iron and hydrogen sulfides, ferrous precipitation as iron sulfide, reduction of nitrates, and formation of ammonium compounds. All of these reactions will generate alkalinity, and all are probably operating in existing flooded coal mines. Addition of sewage will likely stimulate the existing microbial populations and accelerate most of these reactions. However, the fate of waterborne pathogens in these environments is poorly understood—especially for protracted periods of storage time. Thus, with this approach, many difficult issues would have to be addressed. |
However, vortex separators may not effectively handle widely varying flows, such as the transient flows of CSOs. They have a large footprint: the land area required for this process equipment is large, compared to other available treatment technologies. Finally, because vortex separators attain solids separation by physical means, they do not remove colloidal-sized particles. Therefore, indicator bacteria and pathogens contained in very small fecal particles or unattached to fecal particles are not removed and are passed on to receiving waters unless effective disinfection is applied. As might be expected from the preceding discussion, the effectiveness of vortex separator technology has been inconsistent across the United States. Although this technology has been an operational success in some places, demonstration projects in other locations have concluded that the technology does not meet local needs.
The ALCOSAN draft LTCP relies heavily on the use of vortex separators for treatment of CSOs, with the proposed use of 10 of these separators (TPRC, 2002). The total treatment capacity of the 10 vortex separators recommended in the ALCOSAN draft LTCP is 481 mgd
(ALCOSAN, 1999). While the LTCP specifies vortex separators as “treatment facilities” that treat and discharge all flow to adjacent waterways during periods of peak wet weather flow, they actually only split flow into two effluent streams—a “clarified discharge” stream (approximately 85 to 95 percent of the inflow at design flow conditions) and a “foul underflow” stream (approximately 5 to 15 percent of the inflow at design flow conditions). Although the clarified stream is in fact discharged to the water body, the foul underflow must still be conveyed to the WWTP for subsequent treatment.
The topographical and physical features of Allegheny County seem to be compatible with vortex separators because the steep slopes provide adequate energy to drive wet weather flows through the vortex devices. Moreover, the location of the deep interceptors in certain sections will also allow construction of vortex facilities that do not require effluent or foul flows to be pumped. However, the disadvantages discussed above justify a review of the ability of vortex separators to effectively improve water quality before a significant investment is made. If a desktop review is favorable, evaluation of the technology on a pilot or demonstration scale is an appropriate follow-up action.
Reservations about vortex separators were also expressed in the TPR report (TPRC, 2002). It noted that the number of remote treatment plant facilities should be reduced. Where possible, outfalls to centralized facilities should be consolidated. Siting of these facilities is likely to be a problem in Allegheny County as it has been in other densely populated areas. That is, a “not-in-my-backyard” attitude may develop in the community once ALCOSAN begins to attempt to acquire sites for the 10 vortex facilities currently in the draft LTCP. In addition, the operation of multiple facilities at remote locations will not be easy. The TPRC also noted that where vortex units are to be used, they should be designed so that typical hydraulic overflow rates remain below 10 gallons per minute per square foot (gpm/sf) and average 5 gpm/sf so that they act as primary treatment devices. The foul underflow will have to be conveyed from the vortex facilities in transmission conduits, some of which appear to have no additional capacity. This capacity limitation may be another reason to consider retrofitting some (if not many) of the regulators with automated gates and computer controls (RTC) so that room can be made in the flow-limited sections for vortex foul flows. Finally, the TPRC advised that during facility planning, chemical storage should be included in the facility layout, so some form of disinfection may be added in the future, if needed.
The committee shares the concerns about the performance of large-scale vortex separators described in the TPR report (TPRC, 2002). As this report was nearing completion, ALCOSAN advertised a request for proposals to plan and design the first two vortex separators. It is recommended that at a minimum, these projects be implemented prior to widespread application in the ALCOSAN system.
The draft LTCP also calls for the construction of 5 previously planned and 10 new storage basins away from the main ALCOSAN treatment plant, which (with existing basins) represent a total capacity of 110 million gallons (not including an additional 43 million gallons of new storage facilities in the local systems) (ALCOSAN, 1999). The operation and maintenance of such remote facilities can be burdensome and sometimes impractical. Although the remote facilities approach has been successful and economical in some communities (e.g., Columbus, Georgia), the committee also recommends that this aspect of the ALCOSAN draft LTCP be considered carefully before proceeding.
Ballasted Flocculation
Ballasted flocculation is a process in which small particles in water or wastewater are mixed with bridging agents and tiny, high-density sand particles to increase their original size and weight. These heavier, “bulked-up” particles settle much faster and, therefore, can be removed faster and with much smaller surface area of process vessels. This process train—consisting of two stages of mixing for flocculating particles with coagulant and then attaching them to sand grains with polymer, an inclined-plate settling tank, and centrifugal recovery of the sand ballasting agent—is marketed by vendors for treatment of drinking water, SSOs, and to a lesser extent CSOs (see more below). Numerous facilities of this type have been installed in Europe, where the technology was developed, and they are now being used throughout the United States. Ballasted flocculation is an option for treating excess flow from CSOs because the process is very compact, operates at high rates, and can be started on short notice when wet weather occurs and flow increases.
Use of the ballasted flocculation concept for at-source treatment of CSO is relatively new and is not practiced extensively in the United States. However, the small unit footprint and the “instant-on” nature of the process seem to be well matched with the intermittent, high-volume nature of CSOs. This suggests that some hydraulic stability and dependable, consistent solids removal relative to vortex separators might be achieved in this type of application.
In 2003, a ballasted flocculation treatment facility was installed in Lawrence, Kansas, to handle up to 40 mgd of excess sanitary sewer flow associated with heavy rainstorms (Wagner et al., 2003). Studies projected that by 2020, the peak flow to the city’s 25 mgd WWTP could reach 65 mgd during 10-year storm events. Localized storms can cause high sanitary sewer flows even though the receiving water, the Kansas River, may be in a low-flow condition due to low rainfall in the upstream watershed.
In the facility at Lawrence, excess flow is diverted at an excess flow splitter at the WWTP, where ferric chloride coagulant is added prior to the wastewater going to ballasted flocculation for treatment. Coagulated wastewater enters a mixing tank where a powder-fine sand (microsand) and polymer are added. Polymer addition results in attachment of coagulated wastewater to the microsand during a short period of flocculation that is employed before the settling process. The microsand causes the floc that forms to be much denser than ordinary floc, so the settling tank can be operated at overflow rates up to 60 gpm/sf—40 to 60 times higher than conventional overflow rates. This very high settling rate permits the use of a much smaller settling basin, enabling the size of the treatment facility to be a small fraction of the size of a conventional facility. The clarified ballasted flocculation effluent is chlorinated and then dechlorinated before discharge to the receiving water, while the settled sludge is separated from the microsand in a cyclone separator and then treated in the existing wastewater treatment plant.
A ballasted flocculation facility can be started up and treatment optimized in about 15 minutes. To aid operators in anticipating the need to operate the facility, a monitoring system was installed in collection sewers and at pumping stations, so the Lawrence treatment plant personnel will know when high flows are coming and when to turn on the auxiliary facility.
In June 2003, performance testing was conducted in each of the two 20 mgd ballasted flocculation treatment trains, using primary wastewater plant effluent. Total suspended solids removal rates ranged between 65 and 80 percent (Wagner et al., 2003). Shortly after the SSO treatment facility was tested in the summer of 2003, it was operated during a major storm event in which Kansas City and the surrounding area received about 10 to 13 inches of rain in a two-
day period. The ballasted flocculation facility was operated for 32 hours, with flows ranging from 5 to 28 mgd (Cindy Wallis-Lage, Black & Veatch Corporation, personal communication, 2003). During this period of operation, total suspended solids removal ranged from 80 to 90 percent, and after the first three hours of operation, effluent total suspended solids ranged from 28 mg/L to 11 mg/L. For the entire project, construction costs for all of the facilities to monitor and treat excess flow were about $9 million, or $0.22 per gallon of installed capacity (Wagner et al., 2003).
The applicability and feasibility of ballasted flocculation should be evaluated as an alternative to vortex separators for CSO source treatment in the ALCOSAN system, and laboratory or pilot-scale studies should be conducted.
In-River CSO Storage Using Flow Balancing
In-river CSO storage using the flow balancing method (FBM) may be feasible for certain point sources. The basic concept is that a volume of CSO can be contained in a tank, consisting of flexible plastic curtains placed in a receiving stream for the temporary storage of CSOs. Combined sewer overflow that results during and immediately after wet weather enters the tank and displaces river water contained in the tank. After the storm event, the stored CSO is pumped back into the sewer to be transported to the treatment plant, and river water flows back into the tank. The plastic curtains forming the tank are suspended by pontoons and anchored to the riverbed (thus forming the base of the tank) by concrete weights.
A pilot-scale FBM, following a concept developed in the late 1970s in Sweden, was constructed and evaluated by the EPA in conjunction with the New York City Department of Environmental Protection in the 1990s at Fresh Creek in New York City (Field et al., 1994, 1995; Fordran et al., 1991). The Fresh Creek FBM was somewhat different from the original Swedish concept, which was originally designed for installation in a lake. For that purpose, a series of tanks or bays was used, with the first one receiving the CSO and the last one discharging to the lake in a “plug-flow” manner. The series of tanks helped reduce mixing between the CSO and the lake water. In the Fresh Creek study, the receiving water consisted of seawater, which had a higher density than the low-salinity CSO and only a single tank was used. The CSO that entered the tank floated on top, displacing the seawater, which then passed out into Fresh Creek through openings in the tank bottom.
The initial capacity of the tank used in the Fresh Creek study was 0.41 million gallons, and this was later expanded to a final capacity of 2 million gallons (Field et al., 1994, 1995). Because the CSO volumes at this location were generally much larger, in the 5 million to 10 million gallon range, the volume of the pilot system was insufficient to contain much of the CSO. Nevertheless, the pilot study was sufficient to demonstrate the principles of operation and the ability of the system to withstand marine environmental conditions. No damage resulted due to stresses caused by saltwater, tidal exchanges, CSO events, or coastal storms. A phase-one study was conducted using the smaller-capacity system to determine the efficiency with which the CSO was captured by the FBM. Notably, 77 percent of CSO was captured for one wet weather event in which the CSO volume was less than the volume of the tank. However, an operational difficulty with the Fresh Creek FBM was that a portion of the suspended solids within the CSO tank settled to the creek bed. For this reason a system of sediment pumps was needed to capture the settled solids.
An FBM design to help control periodic CSOs in southwestern Pennsylvania—and, more specifically, to help equalize flows to ALCOSAN’s collection system and wastewater treatment plant—would need to be different because of the differences between flow in a creek in New York City and the main stem rivers in the Pittsburgh region. A design more similar to that of the original Swedish design would likely be required, though a number of questions would have to be addressed. These include how the flexible curtains would be anchored in the river bottom; how to provide for differences in river stage between low flow and flood stage, if pontoons are used to suspend the flexible curtains; the extent to which the cross-sectional area of the river would remain available for unimpeded river flow with the curtain walls in place; and the effects of river current on the flexible curtain during flood stage. Although widespread use of an FBM system would not be adequate for the majority of CSO discharges in southwestern Pennsylvania because of the typical volumes involved, there may be locations in which CSO volumes are sufficiently low, and the cost of a conveyance system to a WWTP or more conventional CSO control approaches (e.g., basin or tunnel construction) so high, that an FBM system could be a good alternative to explore. Therefore, the committee recommends that in-river CSO storage using FBM technology be explored and, if feasible, piloted at a particularly suitable location for such a system.
Wet Weather Water Quality Standards
As part of Step IV of the CWARP planning and implantation process for high-density urban areas, the Pittsburgh region, in cooperation with PADEP and EPA, may find it necessary to revisit Pennsylvania's water quality standards. Existing policy recognizes that absolute limits on water quality parameters may not be economically achievable under all hydrological and climatological events. Water quality criteria for a variety of parameters, including chemical contaminants, microbiological indicators of fecal contamination, and physical characteristics such as color and temperature are usually set and enforced to protect ambient waters during very low flows in streams. For example, in Pennsylvania, effluent limits for temperature and pH are to be established using a design flow of the lowest 30-day average that is expected to occur every year with a probability of 10 percent (25 PA Code §93, Water Quality Standards). If flow drops below that level, exceedances of numerical standards for temperature and pH are not considered to be violations of water quality standards.
In southwestern Pennsylvania, wet weather, high-flow events are some of the leading contributors to water pollution. Thus, under very high flow conditions, numerical standards for some contaminants may be exceeded but designated uses of a stream may not be impaired. For example, during flood events, numerical turbidity standards in steams are frequently exceeded, but recreational uses of the stream may be foreclosed for safety reasons, not because of water quality conditions. The stream may then return to normal uses when flood flows recede. Achievement of numerical standards during all high-flow events could be prohibitively expensive. Determining an acceptable frequency and duration for such high flow events when exceedances of numerical standards are allowed is a difficult and controversial decision.
The EPA recognized this possibility when it promulgated the national CSO policy in 1994. That policy permits modification of state water quality standards and related uses when the standards cannot be achieved because of CSOs. For example, EPA’s CSO policy allows for
the possibility of revisions to wet weather water quality standards as evidenced by the following excerpt from its 1999 memorandum:
Data developed during LTCP development can inform decisions about the attainability of designated uses and the appropriateness of any WQS [water quality standard] revisions. State and federal WQS authorities need to be involved throughout the planning process to ensure that, if the LTCP is based in part on anticipated changes to WQS, those changes are appropriate and satisfy federal regulatory requirements.
Leo (1999) reviewed the history of EPA's CSO policy through 1999 and stated that the intent of the policy was to control CSOs up to the point at which maximum benefits could be achieved. Water quality standards would then be modified to allow exceedances for wet weather events that were more extreme. Leo reported that EPA had approved water quality standards in Ohio, Maine, and Massachusetts that did account for wet weather flows. In some cases, use attainability analysis would be required before the standards could be applied to particular streams.
Adoption of wet weather water quality standards is likely to be a highly controversial process. Woodworth (2000) recounts the process in Washington, D.C. All streams in Washington, D.C., were classified for primary contact recreation, and the related standard prior to preparation of a regional LTCP had both a narrative and a numerical standard. The narrative standard stated that the waters shall be free of untreated sewage, a condition that could not be attained under all high-flow events. Efforts to change the standard to account for wet weather flows were criticized as rolling back environmental standards. Woodworth was very critical of the process by which the standard was modified.
This committee recommends that changing water quality standards be considered as a last resort and concurs with Woodworth’s (2000) admonition that “Water quality standards should be reevaluated only after a comprehensive long-term control plan has been designed, approved, and implemented. Provisions should be made to monitor and upgrade the plan as necessary.” In addition, the committee also recommends that (1) a detailed estimate of incremental costs and an assessment of the impact on existing designated uses be included in any reevaluation of water quality standards, and (2) any reevaluation be conducted in close cooperation with PADEP and with broad public participation.
SUMMARY: CONCLUSIONS AND RECOMMENDATIONS
A fundamental prerequisite to the formulation of cost-effective plans for reducing water quality impairments in southwestern Pennsylvania is a systematic and extensive set of water quality data covering both sources of impairments and instream responses. As discussed in Chapters 3 and 4, serious water quality problems exist in southwestern Pennsylvania, but there are not sufficient data to determine the relative seriousness of the environmental and human health problems, the relative importance of potential sources of contamination, and the improvements that are likely to result from alternative pollution control measures.
The most important water quality problem in the region from a regulatory perspective and the potential for adverse human health effects is controlling microbial contamination of streams that derives from the effect of wet weather conditions on sewer systems (CSOs, SSOs, and stormwater), failing OSTDSs, and agricultural and urban runoff. Remedial actions are
planned and anticipated by ALCOSAN and many of its partner communities in response to a series of consent orders that will alter the relative contribution of different sources to the water quality problems in the region. The evaluation of water quality improvements related to such activities will be critical. However, the implementation of solutions for identified impairment sources does not preclude the need for additional information related to other sources and their contributions to water quality impairment in the region. To develop better understanding of sources of contamination in southwestern Pennsylvania, water quality monitoring and modeling efforts should take place concurrently with mandated remedial activities.
It is clear that the causes and nature of water quality impairments, the parties responsible, and the individuals and waterways affected differ for each of the problem contaminants in the region. A comprehensive watershed-based approach is needed to address the spectrum of water quality problems, including wet weather problems; such a systematic approach should recognize interrelationships among problems and the need for parties responsible for each water quality problem to share in its solution. To achieve this, it is necessary to develop both a technical and an institutional-financial approach. The institutional and financial approach is discussed in Chapter 6, and the technical approach is embodied in what the committee calls the Comprehensive Watershed Assessment and Response Plan or CWARP. The Three Rivers CWARP described in this report is not a single document or program; it is a flexible umbrella concept identifying the activities that can be carried out by the organizations that are most technically and institutionally capable of achieving the desired results depending on existing and potential capabilities.
The framework recommended for planning and implementation of CWARP consists of the following five basic steps: (I) problem identification; (II) assessment of existing conditions; (III) projection of future loads; (IV) formulation and evaluation of alternative management strategies; and (V) adaptive implementation of elements of the strategy. This five-step CWARP process must be adapted to address each of the following interrelated scales: river basin, multicounty/metropolitan scale, high-density urban areas, and rural areas. The committee recognizes that the region is not starting with a blank slate, and Step I has been largely completed for each of these scales. Substantial progress has been made on Step II, but as noted in this chapter, significant gaps remain. Because the problems are largely associated with existing conditions and there is only modest growth in the region as a whole, Step III may be less important, but changes in land use that are occurring in suburban (formerly rural) areas cannot be ignored. Lastly, Steps IV and V do not appear to have been well developed at any of the scales, and these steps deserve much greater attention.
Because regional information on the biological quality of receiving waters is scant, its collection during and in support of CWARP at the river basin scale is critical. Biological water quality indices and their change over time can yield important information about ecosystem change and help quantify the environmental benefits affected by pollution abatement. Thus, information collection for CWARP should include biological data to both assist in ecosystem health assessment benchmarking and to help document changes to the ecosystem that occur as a result of changing stressors. At a minimum, the CWARP should be designed to establish an Index of Biotic Integrity for the main stem rivers. To this end, an effort should be made to expand the Ohio River component of EPA’s rejuvenated Great Rivers EMAP program, with an emphasis on the biological water quality of the main stem rivers.
At least two aspects of water management are of concern at the multicounty/metropolitan scale of CWARP. First, and at the very least, water quality planning at this scale should be
sufficient to inform regional interests of the potential effects (including constraints if any) of water quality conditions on future transportation and land development, the consequences of development on water quality where it occurs, and how these effects and consequences can and should be modified. Second, planning at this scale should also result in the identification of opportunities for economies of scale in the delivery of water and wastewater services through cooperative arrangements among local governments. Either SPC or an alternative organization should formulate regional water resource plans and integrate them with transportation and land use plans.
Several entities have recently estimated that solving wet weather problems in the urban core of the region by conventional means, using a combination of storage, conveyance, and treatment improvements, could cost several billion dollars. Investing large sums of capital based only on currently available data may not ultimately solve the most important problems or provide appropriate solutions. Although it is true that no amount of additional data and analyses would remove all uncertainty about water quality investments, it is clear that currently available information is lacking in several critical areas (e.g., how much surface water runoff from separate stormwater sewers affects water quality in receiving streams during wet weather events). Until these facts are known better, planning and implementation of cost-effective remedial measures will be impeded. Regardless of the regulatory approach (i.e., presumption or demonstration) used in ALCOSAN’s LTCP for controlling wet weather problems, the committee concludes that it is necessary to address watershed-wide problems and sources of contaminants other than CSOs and SSOs.
Step II of CWARP at the urban scale should include simultaneous monitoring of (1) wet weather discharges into the region’s streams and rivers and (2) the impacts on these receiving streams. Pollutants of primary concern in this context include pathogenic microorganisms such as Cryptosporidium and their surrogates (indicator microorganisms); oxygen-demanding substances including suspended solids and sediments; and “conservative” toxic substances such as metals and toxic organic chemicals. Step II should also include the development of a variety of models that are sufficient to disentangle the effects of multiple sources on water quality in receiving streams. The models and data should be available for public review, and data from these technical studies should be reduced and translated to needed corrective actions in a manner that is understandable to decision makers and the public in general.
Although receiving water quality modeling activities appear to be extremely limited currently in the region’s three main stem rivers, the committee recommends that it be used to estimate impacts of pollution loadings on the receiving streams and to help prioritize alternatives for pollution control. Other modeling activities needed to implement Step II of CWARP in the region’s urban core include sewer system routing models, dynamic sewer system modeling, dynamic stormwater modeling, and real-time sewer flow control modeling for analysis and operation. Projections of changes in the regional landscape are important in the planning and implementation of Step III of CWARP in the region’s urban core. Planning studies conducted at the multicounty/metropolitan scale should be sufficient for this purpose and include projections for several land use, transportation, water supply, and wastewater parameters discussed in this chapter.
At least six components of a strategy to implement Step IV of CWARP for high-density urban areas should be considered and are discussed in this chapter, some of which are mandated under provisions of the Clean Water Act. The first route to successful improvement of water quality in the region is to optimize utilization of existing infrastructure. To this end, the
committee strongly recommends that all wastewater collection systems located in the watershed, particularly in the urban core areas of southwestern Pennsylvania, be fully compliant with EPA’s CMOM policy or an equivalent program. Thereafter, related information, approaches, and technologies recommended in this chapter and report would be available to help guide major long-term investments in improving the region’s water quality.
Furthermore, ALCOSAN’s draft LTCP should be reevaluated in the context of the overall CWARP approach to reflect ongoing consent order negotiations, CMOM, and information from CWARP as it is developed in the future. The CWARP approach is recommended as a framework for development of the LTCP and similar documents because of the circumstances (especially data limitations) that exist in southwestern Pennsylvania and, in principle, would apply to other regions of the United States with similar water quality problems and circumstances. In addition, in the development of a final LTCP, ALCOSAN and other wastewater treatment providers in southwestern Pennsylvania should evaluate the utilization of real-time control of CSOs. Storage and treatment of CSO in abandoned mine voids, which is currently being evaluated for the Township of Upper St. Clair, Pennsylvania, should also be evaluated. The committee also recommends consideration of the following innovative technologies and approaches for improving water quality in southwestern Pennsylvania, especially in the region’s urban core: (1) at a minimum, implementation of pilot or demonstration projects prior to widespread application of vortex separators for CSO source treatment in the ALCOSAN system; (2) the feasibility of ballasted flocculation facilities and in-river CSO storage using FBM technology for controlling CSOs; and (3) the adoption of wet weather quality standards—although this is likely to be a highly controversial process and should be considered as a last resort.
Best management practices for OSTDSs should be implemented throughout the region using the CWARP framework. Although individual OSTDSs are permitted locally and current technical standards are available to ensure proper performance, they may be ignored. Furthermore, prevention of the discharge of untreated sewage into local waterways or ditches is difficult to enforce. The region needs a coordinated, well-funded program for oversight and routine maintenance of OSTDSs. Such a program can be self-sustaining through user charges provided they are applied on a cooperative regional or county basis. The committee recommends the following actions to help improve water quality in the predominantly rural areas of the region: (1) within each county, register all individual on-site and cluster disposal systems with the appropriate SEO; (2) institute a program of periodic mandatory inspection and certification (or decertification), either by a public entity or by a qualified/licensed private contractor; (3) conduct statistically valid surveys of septic tank and absorption field conditions, residence by residence, to identify communities that should be given high priority for funding by PENNVEST or the federal RUS for remediation of failed and failing OSTDSs throughout the region; and (4) use the registration and inspection program to identify and order elimination of illegal direct discharges of human waste to streams and identify where cluster OSTDSs may be feasible.
There are no comprehensive estimates of the economic benefits of addressing the remaining water quality problems for southwestern Pennsylvania or of projects proposed to address the region’s water quality problems. Nevertheless, the region would be expected to benefit economically from measures that significantly reduce drinking water risks and enhance recreational opportunities. The CWARP process can identify a list of alternative management strategies and projects that are technically feasible and capable of addressing the region’s water
quality problems at a variety of scales, but the question remains: Which is the better option? Comprehensive evaluation of options under CWARP (especially Step IV for high-density urban areas) requires additional considerations, including costs, benefits, and fairness. It continues throughout the process of selecting a preferred long-term management strategy for a particular water quality problem at given scale. It is essential in the formulation of alternatives to provide feedback as to how initial designs should be modified or discarded in the search for a cost-effective strategy. It is also essential to the process of establishing priorities among the several elements that may comprise the management strategy. Finally, it must be a continuing process during implementation to evaluate how well each element has performed. A variety of evaluation frameworks are available; some of the more prominent are discussed in this chapter, including cost-effectiveness analysis, benefit-cost analysis, and multicriteria methods. The use of cost-effectiveness as the primary method for evaluating options for achieving water quality objectives in the region is recommended and should include an analysis of incremental costs to achieve elimination of low-probability contamination events. The committee recommends the use of benefit-cost analysis in evaluating water quality improvement projects in the region and for helping to set priorities.
As the CWARP process is being planned and implemented, it is essential that it be integrated with the ongoing process of establishing TMDLs for impaired streams being conducted by PADEP under requirements of the Clean Water Act. There are many parallels between CWARP and the process for establishing TMDLs—especially in the application of adaptive implementation. The TMDL process—supplemented by additional analyses of constituents that may not be readily subject to the rigorous TMDL approach, including biological, environmental, and other measurable factors—should be combined with watershed, regional, and subregional analysis of beneficial uses to provide the basis for selection of remedial actions in the study area.
The CWARP effort should be completed quickly to provide timely support for those water quality improvements that are required and others that are in the public interest. It is difficult to estimate the cost of implementing CWARP, but in the committee’s judgment it should be low compared to the cost of improvements and more than offset by potential savings.
REFERENCES
Abdalla, C. 1994. Groundwater values from avoidance cost studies: Implications for policy and future research. American Journal of Agricultural Economics 76(5):1062-1067.
ALCOSAN (Allegheny County Sanitary Authority). 1999. Draft Combined Sewer Overflow Program Phase I Activity Report: Regional Long Term Wet Weather Control Concept Plan. Pittsburgh, PA: ALCOSAN.
Anderson, R., K. Beer, T. Buckwalter, M. Clark, S. McAuley, J. Sams, and D. Williams. 2000. Water Quality in the Allegheny and Monongahela River Basins: Pennsylvania, West Virginia, New York, and Maryland (1996-98). Denver, CO: United States Geologic Survey.
Casman, E., B. Fischhoff, C. Palmgren, M. Small, and F. Wu. 2000. An integrated risk model of a drinking water borne cryptosporidiosis outbreak. Risk Analysis 20:495-511.
Clean Water Action Plan Partners. 2000. The Guest River Watershed: River restoration in an Appalachian watershed. In Watershed Success Stories: Applying the Principles and Spirit of the Clean Water Action Plan. Washington, DC: Clean Water Action Plan.
Collins, A., R. MacDowell, M. Brooks, A. Kennedy, and J. Murphy. 2000. Water quality on the Left Fork of the Mud River: A watershed survey. Unpublished final report to West Virginia University Lincoln County Extension Office and Kellogg Community Partnership, College of Agriculture, Forestry, and Consumer Sciences. Morgantown, WV.
Davis and Simon, eds. 1995. Biological Assessment and Criteria Tools for Water Resource Planning and Decision Making. Boca Raton, FL: Lewis Publishers, Inc.
EPA (U.S. Environmental Protection Agency). 1994. Combined Sewer Overflow (CSO) Control Policy. FRL-4732-7. Federal Register (59)75. Available on-line at http://www.epa.gov/npdes/pubs/owm0111.pdf. Accessed March 29, 2004.
EPA. 1995a. Combined Sewer Overflows: Guidance for Long-Term Control Plan. EPA 832-B-95-002. Washington, DC: Office of Water.
EPA. 1995b. Combined Sewer Overflows: Guidance for Nine Minimum Controls. EPA 832-B-95-003. Available on-line at http://www.epa.gov/npdes/pubs/owm0030.pdf. Accessed March 29, 2004.
EPA. 1995c. Combined Sewer Overflows Guidance for Funding Options. EPA 832-B-95-007. Washington, DC: Office of Water.
EPA. 1995d. Combined sewer Overflows Screening and Ranking Guidance. EPA 832-B-95-004. Washington, DC: Office of Water.
EPA, 1996. EPA Overview of the Storm Water Program. EPA 833-R-96-008. Washington, DC: Office of Water.
EPA. 1997. Combined Sewer Overflows Guidance for Financial Capability Assessment and Schedule Development. EPA 832-B-97-004. Washington, DC: Office of Water.
EPA. 1999. Economic Analysis of the Final Phase II Storm Water Rule. EPA 833-R-99-002. Washington, DC: Office of Water.
EPA. 2000a. A Benefits Assessment of Water Pollution Programs Since 1972: Part 1: The Benefits of Point Source Controls for Conventional Pollutants in Rivers and Streams. Washington, DC: Office of Water and Office of Policy, Economics, and Innovation.
EPA. 2000b. Guidelines for Preparing Economic Analysis. EPA-240-R-00-003. Available on-line at http://yosemite.epa.gov/ee/epa/eed.nsf/webpages/Guidelines.html/$file/Guidelines.pdf. Accessed August 16, 2004.
EPA, 2001a. Clean Watersheds Needs Survey 2000: Report to Congress. EPA-832-R-03-001. Washington, DC: Office of Wastewater Management.
EPA. 2001b. Guidance: Coordinating Combined Sewer Overflow (CSO) Long-Term Planning with Water Quality Standards. EPA-833-R-01-002. Washington, DC: Office of Water.
EPA. 2003a. Handbook for Management of Onsite and Clustered Wastewater Treatment Systems (Draft). EPA 832-D-03-001. Washington, DC: Office of Water.
EPA. 2003b. Voluntary National Guidelines for Management of Onsite and Clustered Wastewater Treatment Systems. EPA 832-B-03-001. Washington, DC: Office of Water.
EPA. 2004. Metals, pH, and Fecal Coliform TMDLs for the Guyandotte River Watershed, West Virginia. Available on-line at http://www.epa.gov/reg3wapd/tmdl/pdf/Guyandotte/Sections%201-8,%20Ref/Sec-1_Guy_WV_TMDL.pdf. Accessed June 24, 2004.
Field, R., R. Pitt, D. Jager, and M. Brown. 1994. Combined sewer overflow control through in-river water storage: An efficiency evaluation. Water Resources Bulletin 30(5):921-928.
Field, R., R. Pitt, M. Brown, and T. O’Connor. 1995. Combined sewer overflow control using storage in seawater. Water Research 29(6):1505-1514.
Forndran, A., R. Field, K. Dunkers, and D. Moran. 1991. Balancing flow for CSO abatement. Water Environment & Technology:54-58.
Freeman, M. 2003. The Measurement of Natural Resource Values: Theory and Methods, 2nd Edition. Washington, DC: Resources for the Future.
Gateway Engineers, Inc., and GAI Consultants, Inc. 2003. Progress Report: Storage of Wet Weather Overflows in Abandoned Coal Mines. Pittsburgh, PA: Gateway Engineers, Inc.
Hagedorn, C., S. Robinson, J. Filtz, S. Grubbs, T. Angier, and R. Reneau Jr. 1999. Determining sources of fecal pollution in a rural Virginia watershed with antibiotic resistance patterns in fecal Streptococci. Applied and Environmental Microbiology 65(12):5522-5531.
Harrington, W., A. Krupnick, and W. Spofford, Jr. 1991. Economics and Episodic Disease: The Benefits of Preventing a Giardiasis Outbreak. Washington, DC: Resources for the Future, Inc.
Heberling, M., W. Delavan, J. Shortle, and R. Brooks. 2004. Prioritizing Stream Restoration Using the Stated Choice Method. Working paper from the Department of Agricultural Economics and Rural Sociology. University Park, PA: Pennsylvania State University.
Heinz Center (The H. John Heinz III Center for Science, Economics, and the Environment). 2002. The State of the Nation’s Ecosystems: Measuring the Lands, Waters, and Living Resources of the United States. Cambridge, UK: Cambridge University Press.
Karr, R., K. Fausch, P. Angermeier, P. Yant, and I. Schlosser. 1986. Assessment of Biological Integrity in Running Waters: A Method and its Rationale. Illinois Natural History Survey Special Publication No. 5. Champaign, IL: Illinois Natural History Survey.
Kocagil, P., N. Demarteau, A. Fisher, and J. Shortle. 1998. The value of preventing Cryptosporidium contamination. Risk: Health, Safety and Environment 9:175-196.
Koteen, J., S. Alexander, and J. Loomis. 2002. Evaluating benefits and costs of changes in water quality. PNW-GTR-548. Available on-line at http://www.fs.fed.us/pnw/pubs/gtr548.pdf. Accessed August 16, 2004.
Laughland, D., W. Musser, J. Shortle, and L. Musser. 1996. Construct validity of averting cost measures of environmental benefits. Land Economics 72:100-112.
Leo, William M. 1999. Wet Weather Water Quality Standards, HydroQual, Inc. Available on-line at www.hydroqual.com/Conf/c_wml_001.htm.
Metcalf & Eddy, Inc. 1991. Wastewater Engineering: Treatment, Disposal, Reuse, 3rd Edition. G. Tchobanoglous and F. Burton (eds.). New York: McGraw Hill Education.
Mitchell , C and R. Carson. 1986. Using Surveys to Value Public Goods: The Contingent Valuation Method. Washington, DC: Resources for the Future.
NRC (National Research Council). 2000. Ecological Indicators for the Nation. Washington, DC: National Academy Press.
NRC. 2001. Assessing the TMDL Approach to Water Quality Management. Washington, DC: National Academy Press.
NRC. 2002. Opportunities to Improve the U.S. Geological Survey National Water Quality Assessment Program. Washington, DC: National Academy Press.
NRC. 2004a. Indicators for Waterborne Pathogens. Washington, DC: National Academies Press.
NRC. 2004b. Valuing Ecosystem Services: Toward Better Environmental Decision-Making. Washington, DC: National Academies Press.
O’Connell, T., L. Jackson, and R. Brooks. 1998. The Bird Community Index: A Tool for Assessing Biotic Integrity in the Mid Atlantic Highlands. Report No. 98-4. University Park, PA: Pennsylvania State University Cooperative Wetlands Center, Forest Resource Laboratory.
ORSANCO (Ohio River Valley Water Sanitation Commission). 2002. A Study of Impacts and Control of Wet Weather Sources of Pollution on Large Rivers. Cincinnati, OH.
PADEP (Pennsylvania Department of Environmental Protection). 2002. Model Stormwater Ordinance, Draft. Available on-line at http://www.dep.state.pa.us/dep/subject/Proposed_regulations/SW_MS4_Model_Ordinance.pdf. Accessed May 29, 2004.
PADEP. 2004. Pennsylvania DEP’s Six-Year Plan for TMDL Development. Available on-line at http://www.dep.state.pa.us/dep/deputate/watermgt/wqp/wqstandards/TMDL/TMDL_6yearplan.pdf.
Ribaudo, M. and J. Shortle. 2001. Estimating the benefits and costs of pollution control policies. In Environmental Policies for Agricultural Pollution Control. J. Shortle and D. Abler (eds.). Oxon, UK: CAB International Publishing.
Rosenberger, R., and J. Loomis. 2001. Benefits Transfer of Outdoor Recreational Use Values: A Technical Document Supporting the Forest Service Strategic Plan (2000 Revision). Fort Collins, CO: U.S. Department of Agriculture, Forest Service.
Simmons, G., Jr., S. Herbein, and C. James. 1995. Managing nonpoint fecal coliform sources to tidal inlets. Journal of Contemporary Water Research and Education 100:64-74.
Smith, V., W. Desvousges, and A. Fisher. 1986. A comparison of direct and indirect methods for estimating environmental benefits. American Journal of Agricultural Economics 68:280-290.
TPRC (Third Party Review Committee). 2002. Third Party Review of the ALCOSAN Regional Long Term Wet Weather Control Concept Plan. Pittsburgh, PA: ALCOSAN.
U.S. Census Bureau. 1996. Table 3: Land Area, Population, and Density for Places in Pennsylvania: 1990. Available on-line at http://www.census.gov/population/censusdata/places/42pa.txt.
Wagner, D., M Schultze, and J. Keller. 2003. Kansas plant clarifies new overflow solution. Public Works 134(11):59-62.
Woodward, L. 1961. Ground water contamination in the Minneapolis and St. Paul suburbs. In Ground Water Contamination: Proceedings of the 1961 Symposium. Technical Report W61-5. Cincinnati, OH: U. S. Department of Health, Education, and Welfare, Public Health Service, Robert A. Taft Sanitary Engineering Center.
Woodworth, J. 2000. Balancing bathers and bacteria: Managing recreation, wet-weather flows and the legacy of a combined sewer. Abstract presented to the National Symposium on Designating Attainability Uses of the Nation’s Waters, sponsored by the EPA. Washington, DC, June 3-4. Available on-line at www.epa.gov/waterscience/standards/symposium/abstracts/.