2
Regional Water Resources: Physiographical, Historical, and Social Dimensions
As background to understanding the complex water quality problems of southwestern Pennsylvania, this chapter summarizes the region’s physical geography; its economic, demographic, and land use trends; and the history of its water supply and wastewater treatment practices. Since the 1970s, the Pittsburgh region has evolved from reliance on heavy industry to an economy based largely on technology, medical research, and higher education. This evolution has been accompanied by the decline of older industrial cities and towns and the spread of low-density development into outlying rural areas. As the region’s water quality problems are better understood (see Chapter 3), the need to update the management of the region’s surface and groundwater resources commensurately with its new economy and aspirations becomes manifest.
PHYSICAL SETTING
Pittsburgh and southwestern Pennsylvania are intrinsically identified with the “Three Rivers” that drain the region: the Allegheny, the Monongahela, and below their confluence at Pittsburgh, the Ohio River. The Allegheny and Monongahela basins drain more than 19,100 square miles in Pennsylvania, West Virginia, New York, and Maryland (altogether comprising 14 percent of the Ohio River Basin; see Figure 2-1). Historically, these three rivers served as major transportation arteries linking Appalachia with the Midwest. Based on 2001 data from the U.S. Army Corps of Engineers, Pittsburgh is the second busiest inland port in the nation and twelfth busiest U.S. port of any kind. Associated with the three rivers and their tributaries are many sites of historical, ecological, and recreational importance to the region and even the nation.
From its origin in Potter County, Pennsylvania, to its confluence with the Monongahela River in Pittsburgh, the Allegheny River drains 11,805 square miles and flows for 325 miles through 24 counties in Pennsylvania and New York (see Figure 2-2). Its major tributaries include some of the most biologically diverse, scenic, and historic streams in the country. Ecologically, the upper Allegheny River is particularly diverse, providing some of the best habitat for fish and freshwater mussels in northeastern United States. Indeed, about 85 miles of the main stem south of the Allegheny National Forest are part of the National Wild and Scenic
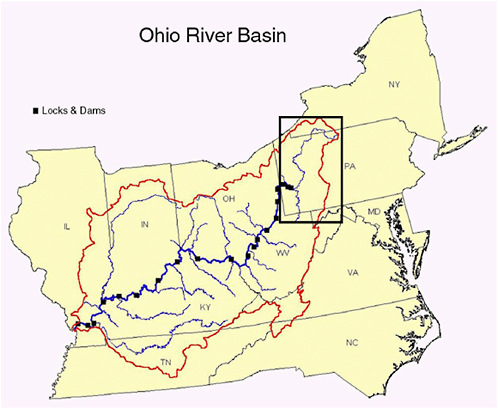
FIGURE 2-1 Ohio River basin showing locks and dams along the Ohio River; boxed area includes the Allegheny and Monongahela River basins and the headwaters of the Ohio River in Pennsylvania. SOURCE: Adapted from ORSANCO; available on-line at http://www.orsanco.org/rivinfo/basin/basin.htm.
Rivers System of the United States National Park Service.1 One of its tributaries, French Creek, is widely known for its diverse natural history, including aquatic species found in this region when European settlement arrived in the mid-eighteenth century.
The Monongahela River drains 7,340 square miles of Maryland, Pennsylvania, and West Virginia (see Figure 2-2). The river rises in the Allegheny Mountains and flows generally northward from Fairmont, West Virginia, through mountainous terrain, farming communities, urban and industrial areas, and coal fields to its confluence with the Allegheny River in Pittsburgh. Over its length of approximately 130 miles, the river is spanned by several locks and dams to facilitate barge traffic. Upstream, a network of dams constructed and operated by the Corps of Engineers since the late 1930s help enhance low flows.
1 |
See http://www.nps.gov/rivers/ and http://www.nps.gov/rivers/wsr-allegheny.html for further information about the program and the Allegheny River’s designation, respectively. |
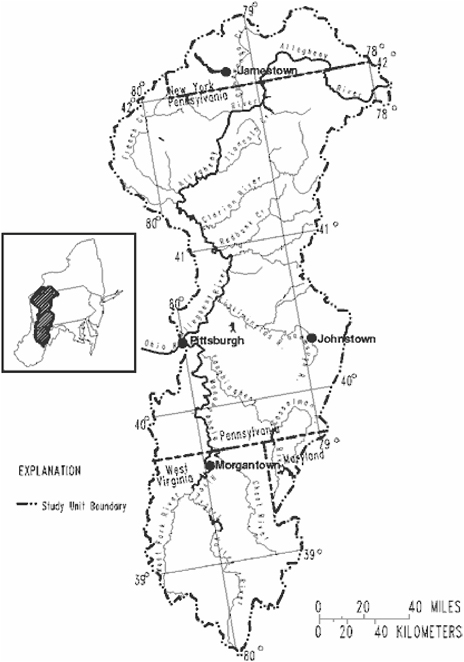
FIGURE 2-2 The Allegheny and Monongahela watershed. SOURCE: USGS, 1995.
Regional Geology, Soils, and Climate
The Pittsburgh region lies primarily in the Appalachian Plateau, which extends southward from New York to Alabama (see Figure 2-3) and is shaped by a geologic history that reflects the bituminous coal fields that have served as an important economic driver for the region for more than a century. However, historical and ongoing extraction of fossil fuels has left the region with a legacy of coal refuse piles, stripped landscapes, and acid mine drainage.
The physical landscape of the region was shaped in part by glaciation. As glaciers moved south during a series of ice ages, they reversed the course of the ancient Monongahela and Allegheny Rivers, which at one time flowed northward into the ancestral Lake Erie basin. As noted by John Harper (1997) of the Pennsylvania Bureau of Topographic and Geologic Survey:
As the earliest glacier moved into northwestern Pennsylvania about 770,000 years ago, the south-flowing ice blocked the northwest-flowing streams and caused lakes to form along the leading edge of the glacier. Eventually, the lakes became so deep that the water flowed over the divides (hilltops and ridges separating streams), reversing the ancient drainage of the Monongahela, Middle Allegheny, and the Upper Allegheny Rivers … The water had to go somewhere. Since it could not flow northward through the ice, it took a southerly course in all of these rivers, carving new water gaps through ridges, and taking over channels from established streams—Nature’s version of eminent domain.
The bedrock of the region consists predominantly of Pennsylvanian Age (290-330 million years ago; MYA) cyclic sequences of sandstone, red and grey shale, conglomerate, clay, coal, and limestone and of Permian Age (250-290 MYA) cyclic sequences of shale, sandstone, limestone, and coal. The bedrock is extensively fractured, providing potential avenues for contaminants to enter groundwater. With thin soil cover in many areas and low-permeability, clay-rich soils in some areas, throughout the region it is difficult to locate sites suitable for wastewater treatment and disposal by conventional septic systems.
The climate of the region is generally humid with temperatures that range widely throughout the year. In January, the mean minimum temperature ranges from approximately 12°F at the source of the Allegheny River in Potter County to 20°F near Pittsburgh, while July maximum mean temperatures range from 86°F just south of Pittsburgh to the mid-70s in Potter County. The mean annual precipitation for the region approximates 40 inches and is distributed rather uniformly throughout the year.2 Annual snowfall totals also average approximately 40 inches. Flooding has occurred as a result of intense precipitation in the region’s steep valleys, sometimes exacerbated by ice jams or inadequate urban drainage. Indeed, periodic floods plagued Pittsburgh for much of the twentieth century. The flood of record occurred on March 18, 1936—an historic event throughout the eastern United States—when the Ohio River crested at more than 25 feet above flood stage. In the past 50 years, owing to the presence of several upstream flood control dams constructed by the Corps of Engineers after the 1936 flood, streets in the downtown Pittsburgh business district have been flooded only a few times. The most recent flood in Pittsburgh occurred in January 1996 when the Point State Park and parking lots of the (now demolished and removed) Three Rivers Stadium were inundated.3
Southwestern Pennsylvania has a long history of flash floods that ravage farm communities and old industrial towns situated in narrow valleys along local streams and rivers. The infamous Johnstown Flood of 1888, about 100 miles east of Pittsburgh, resulted from collapse of a recreational dam during a heavy rainstorm, killing thousands in the industrial communities downstream.4
2 |
See http://www.erh.noaa.gov/er/pit/climate.htm#NORMALSN for further information. |
3 |
Prior to completion of this report, on September 17-18, 2004, heavy rains associated with the remnants of Hurricane Ivan caused widespread flooding throughout southwestern Pennsylvania. Whereas flooding on the Three Rivers at their confluence (i.e., Point State Park or the “Point”) may not have been as great as in 1996, the recent flooding and resultant damages on streams throughout the region were extensive. Thousands of buildings were damaged. Water supply systems were potentially vulnerable to sewer overflows into source waters, but they handled the flood threat well, with no microbial contamination having been detected in drinking water (http://www.pittsburghlive.com/x/search/s_255848.html). |
4 |
Historical (1889) account of the Johnstown flood is available on-line at http://prr.railfan.net/documents/JohnstownFlood/. |
Regional Biodiversity
The headwaters of the Ohio River in Pennsylvania are home to approximately 300 species of birds, 55 species of mammals, 35 species of reptiles, and 35 species of amphibians (WPC, 2004). Similarly, the diversity of aquatic organisms is exceptional, with 130 native species of fish and about 52 species of freshwater mussels (PABS, 1998). However, a U.S. Geological Survey (USGS) report on water quality in the Allegheny and Monongahela River basins (Anderson et al., 2000; see Chapter 3 and Appendix B) found that the water quality and aquatic life of much of the region had been affected significantly by land development and coal mining activities. That report further states that industrial activity in both large and small towns in the region has resulted in contaminated streambed sediments and contaminated fish tissue. The USGS report also highlights water quality successes, such as the treatment of drainage from active and abandoned mines that has generally resulted in improved water quality. “The general improvement in water quality … in sections of the Allegheny and Monongahela Rivers has been accompanied by an increase in the number and species diversity of fish … the recovery of rare species is a further indication of the degree of improvement” (Anderson et al., 2000). Of 52 fish species found in the Ohio River in 1818, only one today is no longer found in the river (Pearson and Pearson, 1989). In June 2004, Pittsburgh was chosen to host the 2005 Bassmaster Classic, a contest billed as the world championship of bass fishing (Belko, 2004). Improvements can be attributed to federal and state programs to improve water quality and the efforts of a growing number of grassroot and community watershed organizations.
JOBS, PEOPLE, LAND USE, AND GOVERNANCE
The Allegheny, Monongahela, and Ohio River system has been central to the development, history, and identity of southwestern Pennsylvania. The Three Rivers, as deepened for commercial navigation by locks and dams, connected Pittsburgh with the Ohio-Mississippi Valley waterway system, and the interior hinterlands of western Pennsylvania, West Virginia, and southwestern New York State (see Figures 2-1 and 2-2). Abundant coal and other natural resources, and the availability of convenient water and rail access within and beyond the immediate region, facilitated economic growth in Pittsburgh and surrounding communities from the mid-nineteenth century until the 1950s. The collapse of the steel industry around 1980 dealt the region a serious economic blow. Since the 1970s, the region’s economic base has shifted to other sectors, including technology, medical research, higher education, finance, tourism, and other services. However, southwestern Pennsylvania has continued to experience economic weakness, as reflected in population decline, unemployment rates, and other indicators such as poverty level and income.
Indicators of Change
Demographic Trends
The Pittsburgh region has seen population declines in both the central city and the surrounding metropolitan area. Between 1970 and 2000, the population of the City of Pittsburgh
fell by 35.7 percent from about 520,000 to about 335,000 (see Table 2-1). Meanwhile, its metropolitan statistical area (MSA; see Box 1-2) population, which stood at 2.5 million in 1970, declined to 2.3 million by 2000, a loss of 7.7 percent (see Table 2-2). During the 1990s, the City of Pittsburgh lost 35,000 residents—a decline of 9.5 percent. By comparison, other northeastern central cities of more than 100,000 in 1990 lost an average of just 1 percent over the 1990s.5 Furthermore, the Pittsburgh MSA was one of only a handful of metropolitan areas nationally to experience a net population decline in the 1990s (amounting to a loss of 36,000 inhabitants, or 1.5 percent; see Table 2-2). Pittsburgh is not alone in losing population from its central city and inner suburbs. Most older industrial (“rustbelt”) cities in the Northeast have experienced loss of population although, unlike Pittsburgh, suburban population growth has generally exceeded inner city losses. This process is commonly referred to as the “hollowing out” of the older urban core, with a “spreading out” or “sprawl” at the metropolitan fringe. According to Sustainable Pittsburgh (2003; see also Appendix B), “seventy percent of [the Pittsburgh Region’s] municipalities have fewer residents today than they did 60 years ago.” Meanwhile, the Pittsburgh MSA reflected a statewide trend of urbanizing nearly 4 acres for every new resident between 1982 and 1997 (Brookings Institution, 2003).
Today, Pittsburgh’s residents are, so to speak, a smaller “family” inhabiting an aging but still elegant “mansion” constructed for a larger household in more prosperous times. The city retains the infrastructure and amenities built for a city of nearly twice its present population, including parks, schools, universities, health care, museums, theaters, water and sewer systems, and (of recent vintage) a light rail public transit system. It also retains a strikingly urbane downtown, excellent civic and commercial architecture, three major professional sports teams(two with relatively new sports stadiums), and a recently completed “green” convention center that is gaining national and international attention.
TABLE 2-1 City of Pittsburgh Population Change: 1970-2000
Year |
Pop. (000s) |
Decadal Change % |
Overall Change % |
1970 |
520 |
— |
— |
1980 |
424 |
−18.4 |
— |
1990 |
370 |
−12.7 |
— |
2000 |
334 |
−9.5 |
1970-2000: −35.7 |
SOURCE: Adapted from U.S. Census of Population, various tables. |
TABLE 2-2 Pittsburgh MSA Population Change: 1970-2000
Year |
Pop. (000s) |
Decadal Change % |
Overall Change % |
1970 |
2,556 |
— |
— |
1980 |
2,571 |
+0.5 |
— |
1990 |
2,395 |
−6.8 |
— |
2000 |
2,359 |
−1.5 |
1970-2000: −7.7 |
SOURCE: Adapted from U.S. Census of Population, various tables. |
5 |
For further information see the 2000 Census of Population, available on-line at http://quickfacts.census.gov/qfd/index.html. |
Despite its metropolitan population decline, the Pittsburgh MSA has been sprawling further onto rural land. According to a Brookings Institution study of urban sprawl from 1982 to 1997, the Pittsburgh MSA lost 8 percent in population but grew by 42.6 percent in urbanized area during that time (Fulton et al., 2001). This resulted in a loss of average density of 35.5 percent, the fourth greatest decline in density among northeastern MSAs during the study period (those exceeding Pittsburgh were Johnstown, Pennsylvania; Portland, Maine; and Utica, New York). Another recent study placed Pittsburgh among the top 20 “land consuming” metropolitan areas nationally, based on its estimated growth in developed land area of 201,000 acres, or 43 percent, between 1982 and 1997 (American Rivers-NRDC-Smart Growth America, 2002). The Pittsburgh MSA was the only metropolitan area of the top 20 to have lost population over the study period.
At the county scale, a picture of wide contrasts in population change and economic activity is presented, with some counties growing, some remaining relatively stable, and some declining (see Table 2-3). Of the total MSA population loss of 36,000 during the 1990s, 35,000 were lost to the City of Pittsburgh; the rest of the MSA outside Pittsburgh thus experienced only a net loss of 1,000 residents. Allegheny and Beaver Counties lost population (−4.1 percent and −2.6 percent, respectively). Butler and Fayette Counties gained population (+14 percent and +2.3 percent, respectively), and Westmoreland and Washington Counties were relatively stable. The five nonmetropolitan (non-MSA; see Box 1-2) counties in the study region were also divided between population losers (Lawrence −1.7 percent; Armstrong −1.5 percent); gainers (Greene +2.8 percent; Somerset +2.3 percent); and Indiana County, which, was stable. In the 11-county region, Allegheny County and 5 out of 6 counties adjoining it either lost population or were stable. Gainers in the region included most notably Butler County directly north of Allegheny (within commuting distance to Pittsburgh) and three rural counties south of Allegheny County, two of which (Greene and Fayette Counties) are within commuting distance of Morgantown, West Virginia.
Economic Trends
In addition to demographics, another important economic indicator is job growth. With the exception of a mild recession in 1990-1991, the last decade of the twentieth century was a period of exceptional job growth in the United States. Total employment in the United States increased by 20.6 percent from June 1990 to 2001 (Fuller et al., 2002). Employment growth in the Commonwealth of Pennsylvania, in comparison, lagged the nation with an overall growth rate of 10.3 percent. Notable exceptions to this trend in the Pittsburgh region were Butler County with employment growth of 32.5 percent and Fayette County with employment growth of 20.2 percent. Employment growth rates in Beaver, Greene, Fayette, Washington, and Westmoreland Counties lagged the nation but exceeded that of the state; employment growth in Allegheny, Armstrong, and Lawrence Counties lagged the state.
Unemployment rates for the region, state, and the United States are summarized in Table 2-4 for June 1990 and June 2001. With the exception of Allegheny County, southwestern Pennsylvania counties had higher unemployment rates than the state and nation in both periods. Particularly noteworthy are the very high unemployment rates in Armstrong, Fayette, Greene, and Indiana Counties. The high unemployment rates in these counties reflect a general tendency
TABLE 2-3 2000 Population Change in the Pittsburgh Region, By County
County |
2000 Population |
Change from 1990 % |
Allegheny |
1,281,666 |
−4.1 |
Armstrong |
72,392 |
−1.5 |
Beaver |
181,412 |
−2.5 |
Butler |
174,083 |
14.5 |
Fayette |
148,644 |
2.3 |
Greene |
40,672 |
2.8 |
Indiana |
89,605 |
−0.4 |
Lawrence |
94,643 |
−1.6 |
Somerset |
80,023 |
2.3 |
Washington |
202,897 |
−0.8 |
Westmoreland |
369,993 |
−0.1 |
Pennsylvania |
12,281,054 |
3.4 |
United States |
281,241,906 |
13.2 |
SOURCE: Adapted from U.S. Census Bureau and Pennsylvania State University’s Cooperative Extension as cited in Pennsylvania State University’s Center for Economic and Community Development Pennsylvania Census 2000, available on-line at http://cecd.aers.psu.edu/census2000/PAcountypop.PDF. |
TABLE 2-4 Unemployment Rates in the Pittsburgh Region (percent)
County |
June 1990 |
June 2001 |
Allegheny |
4.3 |
3.9 |
Armstrong |
7.1 |
8.0 |
Beaver |
5.9 |
5.0 |
Butler |
5.6 |
4.9 |
Fayette |
8.1 |
7.1 |
Greene |
9.3 |
6.8 |
Indiana |
7.6 |
6.4 |
Lawrence |
6.2 |
5.8 |
Somerset |
6.7 |
5.7 |
Washington |
5.6 |
4.9 |
Westmoreland |
5.7 |
5.4 |
Pennsylvania |
5.0 |
4.8 |
United States |
5.3 |
4.7 |
SOURCE: Fuller, et al., 2003. |
for rural Pennsylvania counties to have greater unemployment than metropolitan counties (Shields, 2002).
Three additional economic indicators are presented in Table 2-5: (1) median income; (2) median value of owner-occupied housing; and (3) poverty rate (i.e., percentage of households with incomes at or below the poverty level). With the exception of Butler County, median incomes in Pittsburgh region counties are below the Pennsylvania state average. Similarly, the median value of owner-occupied homes is below the state average in all counties except Butler. Seven of the eleven counties in the study region have poverty rates in excess of the state rate of 11 percent, and three exceed the U.S. rate of 12.4 percent.
TABLE 2-5 Median Income, Median Value of Owner-Occupied Housing, and Poverty Rate for the Pittsburgh Region
County |
Median Household Income (2000) |
Median Value of Owner Occupied Housing (2000) |
Poverty Rate (1999, %) |
Allegheny |
$38,329 |
$83,500 |
11.2 |
Armstrong |
$31,557 |
$63,800 |
11.7 |
Beaver |
$36,995 |
$83,200 |
9.4 |
Butler |
$42,308 |
$105,300 |
9.1 |
Fayette |
$27,451 |
$60,600 |
18.0 |
Greene |
$30,352 |
$55,800 |
15.9 |
Indiana |
$30,233 |
$68,300 |
17.3 |
Lawrence |
$33,152 |
$71,100 |
12.1 |
Somerset |
$30,911 |
$66,900 |
11.8 |
Washington |
$37,607 |
$85,400 |
9.8 |
Westmoreland |
$37,106 |
$87,600 |
8.6 |
Pennsylvania |
$40,106 |
$94,800 |
11.0 |
United States |
$41,994 |
$111,800 |
12.4 |
SOURCE: Adapted from U.S. Census Bureau Census 2000 Demographic Profile Highlights, available on-line at http://factfinder.census.gov/home/saff/main.html?_lang=en. |
Land Use Change and Water Quality
Development and demographic changes in the Pittsburgh region are fraught with significance for water and sewer investment decisions and water quality. There is a substantial literature demonstrating that water quality is impacted by land cover (e.g., Allan et al., 1997; Herlihy et al., 1998; Jones et al., 2001; Roth et al., 1996). In urbanizing watersheds, such as those in the Pittsburgh region, the impact of increasing impervious surface areas is becoming of increasing concern. Indeed, impervious surface is emerging as an important indicator of effects on water quality and biotic quality in streams. Numerous studies have attempted to identify thresholds in the relationship between impervious cover and various types of environmental impacts. A variety of studies indicate that 10 percent impervious cover represents an important threshold for many environmental impacts (e.g., CWP, 2003; Scheuler, 1994).
Impervious surfaces include roads, roofs, parking lots, sidewalks, and other constructed surfaces that are impenetrable to water. Increasing the amount of impervious surface within a watershed affects the hydrologic regime by altering the volume, pattern, and timing of hydrologic flows (CWP, 2003). With an increase in impervious surface area, less precipitation infiltrates the soil and more runs off directly into surface waters. Changes in water flows can lead to physical changes as increased stormwater runoff results in higher periodic stream flow, stream channel enlargement and incision, greater stream bank erosion, and increased sedimentation in the stream channel (CWP, 2003; EPA, 2001; Scheuler, 1994). The water quality impacts of increased stormwater runoff can also be significant. Reduced infiltration means that urban contaminants are carried more directly into surface waterbodies (EPA, 2001). Stream temperatures can be affected by the imperviousness of the watershed (Galli, 1990; Scheuler, 1994). These and other water quality impacts and their implications for humans and aquatic ecosystems are discussed in Chapters 3 and 4.
Apart from the physical impacts of development on water quality through the effects of increased impervious areas, development patterns and population shifts also affect the utilization of existing sewer and water infrastructure and the location of investment in new infrastructure.
As population declines in the central city and inner MSA counties, there is likely to be surplus water and sewer capacity in many of those communities, along with roads, schools, parks, and other infrastructure in place. Much of this infrastructure is old and in need of maintenance and upgrading which has led to sewer “tap-in” restrictions in a number of communities in the Pittsburgh region communities (described later). Meanwhile, new water and sewer infrastructure is under construction or under consideration to serve rapidly growing areas in the outer fringe counties and to remedy existing deficiencies in on-site water and septic facilities.
A balance is needed between the updating and maintenance of water and sewer services already in place versus recreating such capacity to serve outlying development at considerably lower density and therefore higher cost per household. According to a report by the Environmental Law Institute (ELI, 1999; see also Appendix B):
In the counties surrounding Allegheny, the perceived environmental problem is still the release and potential release of untreated or inadequately treated sanitary sewage—rather than the patterns of development which are producing these problems. Solutions are, in turn, driven by current financing realities and institutional preferences for new construction. These factors promote the extension of existing sewer collection systems to a larger ratebase by sewering larger areas of the region, and encourage the replacement of on-lot systems with sewers and wastewater treatment. While this promotes near term environmental improvement, the effect on development and future growth is significant … Common interests of the counties include the need to revitalize the older urban centers and not simply attract greenfields development at the margins of the respective counties.
Similarly, the 2003 report by Sustainable Pittsburgh, Inc.,6 states, “Fiscal expediency alone rationalizes steering development first to existing communities to simultaneously fix, upgrade, and use in-place surplus capacity as opposed to building new elsewhere.” In that report, Carnegie Mellon University President Jared Cohon maintains that, “Southwestern Pennsylvania’s waters are a priceless asset for residents, recreation, industry, and agriculture. To adequately protect that resource, we must make greater investments in infrastructure, and spend that money wisely.”
In a June 9, 2002, speech to a Pittsburgh Smart Growth Conference, Brookings Institution demographer Bruce Katz warned that Pittsburgh’s combination of declining population accompanied by increasing sprawl puts it and similar older industrial cities of the Northeast and Midwest at a competitive disadvantage in comparison with Sunbelt urban areas of the West and South:
I think from a fiscal perspective, from a social perspective, from an environmental perspective… from the perspective of how you compete over time, for new economy firms, for knowledge-based firms, that are seeking quality of life, seeking affordable housing, seeking the natural environment that has been preserved. You are undermining perhaps your competitiveness over time as a region.
6 |
See Appendix B for a summary of that report, available on-line at http://www.sustainablepittsburgh.org/citizensvision/CitizensVision.pdf. |
Among several factors that influence the rate of urban sprawl, Katz also listed infrastructure:
How do we spend the billions of dollars of state money on roads, on water, on sewer, on school facilities, on government facilities, on higher educational institutions? Do we spend this to reinforce, to support areas that we’ve already invested in—cities, older suburbs, townships—or do we continue to basically use them to facilitate more sprawl?
At the opposite end of the development spectrum, many of the same arguments can be made for providing appropriate infrastructure (centralized or decentralized) to certain outlying communities to assist them in preserving rural character, farmland, and open space vital to tourism and outdoor recreation. Because funds for rural water and sewage projects are limited, many of these areas do not obtain funding until commuter “bedroom community” growth (e.g., Burgettstown, Pennsylvania) or extension of roads and their accompanying development overwhelm or threaten to overwhelm the local capacity. Examination of Pennsylvania Act 537 Official Plan Aging database7 reveals that many of the more recent plan updates follow this pattern. Lack of comprehensive rural facilities planning to prioritize infrastructure development in areas of significant rural and recreational potential risks being a partner to abandonment of the urban center in promoting sprawl. The goal of making wise investments in water facilities and other infrastructure, as per President’s Cohon’s challenge above, is greatly hampered by the political fragmentation of metropolitan Pittsburgh and adjoining areas. In addition to the state and federal governments, the region is governed by 11 counties, 595 municipalities, and 492 other water and sewer providers (see Chapter 6 for further information). According to a recent op-ed column in the Pittsburgh Post-Gazette, the region “maintains an astonishing 17.7 general purpose governments per 100,000 residents. This overload of government is more than triple the nation’s metropolitan average of 6.1 governments per 100,000” (Katz, 2004) for the nation’s metropolitan areas.
Stormwater management under the Clean Water Act (CWA) is a critical water-related management function that is complicated by political fragmentation. In particular, efforts by certain jurisdictions to address water quality impairments through “best management practices” (BMPs) may be partially offset or limited by rising stormwater discharges from upstream sources in other communities within the same local watershed. For example, stormwater runoff to Turtle Creek from communities in Westmoreland County adversely affected Allegheny County’s ability to maintain water quality and prevent flooding downstream on that creek (McElfish and Casey-Lefkowitz, 2001). The prevalence of such externalities among jurisdictions sharing an urbanizing watershed highlights the need for intergovernmental cooperation through compacts, joint powers agreements, special districts, or other watershed-based institutional arrangements. Such cooperative efforts must address the type, design, and location of new development within watersheds: “Without strict land use planning, aggressive land acquisition programs, and integrated watershed management … erosion, sediment, and stormwater control programs cannot do the job” (Watershed Management Institute, 1997). The institutional aspects of regional management of water resources are discussed in detail in Chapter 6. In particular, the
7 |
For further information, see http://www.dep.state.pa.us/dep/deputate/watermgt/wqp/WQP_WM/537Map/537Plan.htm. |
concept of a “regional water forum” representing all sectors of stakeholders is recommended for consideration by southwestern Pennsylvania area leaders and citizens.
ECOLOGICAL RESTORATION IN THE REGION
Despite its economic and demographic challenges, key stakeholders in the Pittsburgh region are striving to improve the ecological condition and public enjoyment of its Three Rivers and their many local tributary watersheds. A recent paper by Collins et al. (2003) refers to
… recent struggles to reclaim the natural aspects of the rivers as part of a new post-industrial environmental infrastructure with natural amenities essential to the new economy. This evolving post-industrial reconceptualization frequently involves conflicting efforts of managing the rivers for their scenic and recreational as well as transport opportunities, and restoring and preserving the riverine ecological systems as a public commons.
With the support of area foundations, the Pittsburgh region’s main stem rivers have been rediscovered as amenities supporting water-based recreation and ecological habitat reaching virtually into the city center. Local and regional environmental organizations such as the Pennsylvania Environmental Council (PEC, 2003), 3 Rivers 2nd Nature (3R2N) at the Studio for Creative Inquiry at Carnegie Mellon University, and the Nine Mile Run Watershed Association are collaborating in the analysis and restoration of riparian habitat in selected sites (see Box 2-1). They also are promoting environmental education and public interest in the region’s abundant aquatic resources. As a result, the level of water quality in local streams, lakes, and rivers, as well as groundwater, is of rising public concern.
HISTORICAL SETTING
Pittsburgh Water Supply and Wastewater Treatment
Throughout its history, one of the most serious environmental problems facing the City of Pittsburgh and its outlying areas has been pollution of its streams and rivers. This pollution, from both domestic and industrial sources, accelerated during the nineteenth and twentieth centuries and severely impacted the quality of the water drawn from the rivers for domestic purposes and for various industries. It also sharply curtailed the use of the rivers for recreation.8 Current water pollution problems, especially from combined sewer overflows (CSOs), are the result of a series of decisions related to both water supply and wastewater collection and disposal made over the past century-and-a-half to:
-
draw Pittsburgh’s water supply from the neighboring rivers;
-
construct a water carriage system and build it following a combined rather than a separate sewer design;
-
use the rivers as the place of sewage disposal based on the concept that running water purified itself or provided adequate dilution to disperse the sewage;
-
filter and also chlorinate drinking water in order to deal with public health concerns rather than to use a protected upcountry source;
-
resist state orders to treat the city’s sewage and change the design of the city’s sewerage system (Tarr, 1996a); and
-
subsequent to World War II, treat the sewage of Pittsburgh and neighboring communities by creating an authority with a centralized wastewater treatment plant, but allow communities to own and maintain their own sewage collection systems.
Water Supply
For its first quarter century, Pittsburgh citizens drew their water supplies primarily from local ponds and wells. However, these sources became increasingly inadequate to meet domestic, industrial, fire protection, and public health needs. In 1826, after extensive debate over the issue of public or private ownership, the Pittsburgh Select and Common Councils approved the construction of a waterworks. The waterworks was subsequently completed in 1828 at an initial cost of $40,000 and utilized a steam pump to draw water from the Allegheny River, raising it to a million-gallon reservoir located on Grant Hill for gravity distribution throughout the city (Tarr, 1989).
Throughout the nineteenth century, the waterworks expanded in response to population and territorial growth. By 1915, the system had 743 miles of distribution pipes (Lanpher and Drake, 1930). Because of extensive waste and leaky pipes, the city had one of the highest rates of per capita water consumption of any city in the nation (Tarr and Yosie, 2004).
Waterworks funding was the single largest expenditure made by the city during its first 50 years, which is not surprising given the extent to which waterworks costs constituted a substantial part of the total municipal budget of nineteenth century American cities (Tarr, 1989). Pittsburgh’s willingness to make such a large expenditure for a public good can be explained by the coming together of a variety of interest groups—including merchants and industrialists, homeowners, fire insurance companies, and physicians and others concerned with the public health—to demand an adequate supply of water. Notably, these investments also occurred during a period of prosperity when many of the city’s great buildings and other facilities were being constructed.
Sewerage System
A supply of potable water was only one part of the city’s metabolic system; wastewaters from households and industries and stormwater had to be disposed of as well. During most of the nineteenth century, Pittsburghers placed household wastes and wastewater in nearby cesspools and privy vaults, not in sewers. The 1804 borough charter gave the city the right to regulate such receptacles, but the city councils did not enact an ordinance until 1816, when
BOX 2-1 Nine Mile Run Nine Mile Run is a stream whose watershed drains five Pittsburgh area municipalities, flowing through wooded Frick Park and an urban brownfield before it joins the Monongahela River. The brownfield features a mountain of steel mill slag (15-20 stories high), dumped by Duquesne Slag on the Nine Mile Run Floodplain between 1922 and 1972. The Nine Mile Run Greenway Project (NMR-GP) was established in response to a Pittsburgh housing redevelopment plan for the site that would have buried the stream. The NMR-GP team consisted of diverse academic and outside experts, under the leadership of the Studio for Creative Inquiry at Carnegie Mellon University (CMU). The primary purpose of the project was to explore the cultural and aesthetic issues of postindustrial public space and ecology. The team conducted studies that revealed the potential for aquatic and terrestrial approaches to ecological restoration on the urban brownfield and Nine Mile Run. In addition, the team conducted a series of “community dialogues” that helped develop a set of concept plans used as the framework for a subsequent U.S. Army Corps of Engineers restoration study and plan and a $7 million stream restoration project for the City of Pittsburgh. For further information, see McElwaine (2003) and http://slaggarden.cfa.cmu.edu. A partnership of the Pittsburgh Redevelopment Authority and four developers has recently undertaken a brownfields restoration of an unsightly, contaminated 238-acre slag mountain towering above Nine Mile Run. Following extensive environmental remediation and safety measures (Trout et al., 2001), the team is creating a multiphase “new traditional” urban development with walkable neighborhoods, greenspace commons, trail access to Frick Park and downtown, and housing styles and construction and lot layouts that recreate the look and feel of Pittsburgh’s close-knit, old-fashioned “streetcar neighborhoods.” Demand for the 65 residences in Phase I, completed in 2002, was so strong that a lottery was held to select buyers from a waiting list of 500 (McKay, 2002). Nine Mile Run and Stream Daylighting The aquatic restoration techniques used on Nine Mile Run emerged from water quality studies and water quantity studies based on fluvial geomorphology and wetland science. Ultimately, the |
citizen complaints about overflowing filth and smells from privy vaults caused the councils to approve fines in the case of nuisances. Privy vaults and garbage continued to foul the streets and pollute the rivers with waste, despite the cleaning efforts of private scavengers under city contract. In 1875, the Pittsburgh Board of Health observed that privy vault nuisances were the major health issue facing the city (Tarr, 1989).
The provision of running water to households and the adoption of water-using appliances such as sinks, showers, and water closets, exacerbated the problem of overflowing cesspools and privy vaults. On the one hand, improving the availability and volume of water supply was a benefit, but on the other hand it had the potential to deliver contaminated water with resulting impacts on public health.9 In many cases, in order to dispose of the wastewater, households connected these appliances to existing wastewater disposal sinks (i.e., cesspools and
restoration techniques included “stream daylighting,” the act of unburying, and renaturalizing or restoring a stream and its channel that flowed through Pittsburgh’s Frick Park. The project team also developed concept plans for the redesign and reconstruction of the main stream channel to address a century of erosion caused by stormwater from nearby sewers and roads. By 2005, Nine Mile Run is slated to follow a new streambed, its natural sinuosity restored and wetlands preserved and extended, reducing erosion and allowing for more storage of stormwater during a flood event. Terrestrial restoration was based on a botanical assessment of existing steep slope slag vegetation and greenhouse studies of soil nutrients and native seed sources that held the promise of an ecologically viable habitat (in slag soils) linking the interior forests of Frick Park to the Monongahela River corridor. The plan was for increased landscape diversity, with native plants and a stream that was redesigned for current flow conditions, restoring the potential for a warm water fishery. 3 Rivers 2nd Nature 3 Rivers 2nd Nature (3R2N) is a five-year project funded by the Heinz Endowments that addresses the meaning, form and function of the Monongahela, Allegheny, and Ohio Rivers and riverbanks and 53 tributary streams within Allegheny County. This project builds on the successful linking of ecosystem analysis and community dialogue developed by CMU’s Studio for Creative Inquiry in the Nine Mile Run watershed project discussed above. The project team works with scientists, engineers, planners, and policy experts from the Three Rivers Wet Weather Demonstration Program (3RWW), Allegheny County Sanitation Authority, Allegheny County Health Department, University of Pittsburgh, and private practice. The project has developed partnerships to conduct fieldwork and establish an ecological baseline for Allegheny County and undertakes terrestrial studies of riverbanks and forests and aquatic studies of stream biology and fisheries. It also conducts dry weather physical chemistry and bacterial indicators for pathogen studies in rivers and streams. Like the Nine Mile Run project, it has established a series of river dialogues with local citizens. The project works with communities to address the cultural understanding of living rivers and water quality, as well as the potential to preserve and restore natural ecosystems in a postindustrial urban setting. The 3R2N project will develop a waterfront plan and a series of waterfront node designs with artists, landscape architects, and ecological designers as the project comes to a close in late 2004. For more information, see http://3r2n.cfa.cmu.edu. |
privyvaults). In 1881, for instance, about 4,000 of the 6,500 water closets in Pittsburgh were connected to privy vaults and cesspools but only about 1,500 to street sewers (Yosie, 1981).
Such conditions raised the possibility of waterborne disease outbreaks and highlighted the need for improved sanitation and construction of a sewer system. The city first constructed underground sewers in the commercial district, mainly to prevent flooding by draining urban runoff. By 1866, the commercial district had a “fairly adequate” system of main sewers (Thompson, 1948). Other areas of the city, however, had much more uneven and haphazard service. By 1875, the city had about 25 miles of sewers (brick and pipe), mostly for stormwater drainage. These early sewers suffered from design faults and were often either undersized or oversized and subject to constant clogging. Sewers did not conform to topography (the city lacked topographical maps until the 1870s) or follow an overall engineering plan since the city commonly built sewers in response to council members’ attempts to meet specific constituent demands. In addition, many households constructed their own sewers, which were often unrecorded and not reliable (Tarr, 1989).
Debate raged between engineers and physicians about the design of a new centralized sewer system. Should it be a separate, small pipe system that carried only domestic and
industrial wastes (the technology advocated by the famous sanitarian George E. Waring, Jr.), or should it be a larger, combined system that could accommodate both wastewater and stormwater in one pipe (a design then favored by many sanitary engineers)? Physicians argued that the separate system was preferable because it would protect health by removing wastes from households before they had begun to decompose, thus generating disease-causing sewer gas. They considered stormwater a secondary matter, best handled by surface conduits. Engineers took a different position and maintained that sanitary wastes and stormwater were equally important; therefore, a large pipe system that would accommodate both was more economical. After years of debate, the superior stormwater removal virtues of the combined system convinced city officials, and by the late 1880s, Pittsburgh had begun to build a system of large combined sewers (Tarr, 1996b; Tarr and Yosie, 2004).
Between 1889 and 1912, civil engineers from the new Bureau of Engineering of the Public Works Department constructed more than 412 miles of sewers, almost all of the combined type. The combined sewer carried both stormwater and domestic sewage in one pipe, but because of the fluctuations in the size of rainstorms, the system included overflow valves at critical points throughout the system. The construction of the planned centralized sewerage system signified a movement away from the “piecemeal, decentralized approach to city-building characteristic of the 19th century” (Peterson, 1979). In constructing a large centralized combined sewer network, Pittsburgh was following the lead of other large American cities such as Boston, Chicago, and New York. Like other cities, Pittsburgh discharged its untreated sewage directly into neighboring waterways, with 47 public sewer outlets into the Monongahela River and another 98 into the Allegheny and Ohio Rivers (Tarr and Yosie, 2004).
Typhoid Fever and Water Filtration
While Pittsburgh was discharging its untreated sewage into its neighboring rivers, upstream communities were also building sewers and discharging their wastes into the same rivers. By 1900, more than 350,000 inhabitants in 75 upstream municipalities were discharging their untreated sewage into the Allegheny River—the river that provided drinking water for most of Pittsburgh’s population. Some of Pittsburgh’s own sewers discharged into the river at locations above the city’s water supply system intakes. The resulting pollution gave Pittsburgh the highest death rate from typhoid fever of the nation’s large cities, more than 100 deaths per 100,000 people from 1873 to 1907.10 In contrast, in 1905 the average death rate for northern cities was 35 per 100,000 persons (Koppes and Norris, 1985). At least one reason why the poorer, immigrant neighborhoods may have had higher death rates is that although even these neighborhoods were required to be sewered, the communal toilet facilities themselves were often not water-carriage and did not convey the waste into the sewers (Byington, 1910).
Concerned over the growing typhoid mortality and morbidity rates, in the early 1890s various professional groups formed a joint commission to study the question of water pollution
and water quality. The commission’s report, issued early in 1894 and based on chemical, bacteriological, and statistical methods, found Pittsburgh water supply “not only not up to a proper standard of potable water but … actually pernicious” (Tarr and Yosie, 2004). That commission recommended that the water of Pittsburgh be treated using a slow-sand filtration system. This recommendation was followed in 1896 by the mayor’s appointment of a Pittsburgh Filtration Commission. In 1899, after detailed investigation by outside consultants, the commission recommended that the city construct a slow-sand filtration plant drawing water from the Allegheny River. Thus, Pittsburgh joined cities such as Philadelphia, Cincinnati, and Cleveland that took their water from neighboring rivers and filtered it, whereas other cities, such as Boston, Newark, and New York, chose to rely on protected watersheds—an option rejected by the filtration commission because of high costs. In December 1907, the water department delivered the first filtered water and the city’s typhoid rates began to drop. In 1912, the city began chlorinating its water supply and Pittsburgh’s death rate from typhoid fever dropped to the national average for large cities (Lanpher and Drake, 1930; Tierno, 1977).
Sewage Disposal: Retaining the Rivers as Sewers
Water filtration provided one safety net with regard to sewage-contaminated water, but many sanitarians and public health physicians during the first decades of the twentieth century believed it also necessary to treat the city’s sewage prior to release in nearby streams and rivers. Many professional and business groups had begun to protest sewage disposal by dilution in streams. They demanded that municipalities treat their sewage and called for state laws against stream pollution (Tarr, 1996a). In the early twentieth century, states such as Connecticut, Massachusetts, New Jersey, New York, Ohio, and Pennsylvania, responding to a series of unusually severe typhoid epidemics, gave their health boards increased power to control sewage disposal in streams (Tarr, 1996a).
In 1905, a law “to preserve the purity of the waters of the state for the protection of the public health” (the Purity of Waters Act11) was passed by the Pennsylvania state legislature in response to a typhoid epidemic in 1903. The act forbade the discharge of any untreated sewage into state waterways by new municipal systems. Although it permitted cities already discharging their untreated sewage to continue the practice, it required them to secure a permit from the state health commission if they wished to extend their systems (Snow, 1907).
Although Pittsburgh was filtering its drinking water after 1907, the city continued to dispose of its untreated sewage in neighboring rivers, endangering the water supply of downstream communities. In 1910, the city requested that the Pennsylvania Department of Health (PADOH) grant it a permit allowing it to extend its sewerage system. The department, then headed by Dr. Samuel G. Dixon, responded by requesting a “comprehensive sewerage plan for the collection and disposal of all of the sewage of the municipality” before it would grant the permit (Tarr, 1996a). In addition, PADOH argued that in order to attain efficient treatment, the city should consider changing from a combined sewer system to a separate sewer system. The department’s chief engineer, F. Herbert Snow, maintained that the plan was needed to protect the
public health of communities that drew their water supplies from rivers downstream from Pittsburgh (Gregory, 1974; Tarr, 1996a).
The City of Pittsburgh responded to the PADOH order by hiring the engineering firm of Allen Hazen and George C. Whipple to act as consultants for the required study. The engineering firm argued in its report that a Pittsburgh sewage treatment plant would not free the downstream towns from threats to their water supplies from the need to filter them, since other upstream communities would continue to discharge raw sewage into the rivers. The method of disposal by dilution in rivers, they maintained, was sufficient to prevent nuisances, particularly if storage reservoirs were constructed upstream from Pittsburgh to augment flow during periods of low stream discharge. Hazen and Whipple argued that there was no case “where a great city has purified its sewage to protect public water supplies from the stream below” (Tarr, 1996a). “Rivers are the natural and logical drains and are formed for the purpose of carrying the wastes to the sea,” argued N.S. Sprague, superintendent of the Pittsburgh Bureau of Construction in forwarding the Hazen-Whipple report to the director of public works (Tarr and Yosie, 2004).
Hazen and Whipple’s most powerful argument addressed the lack of economic feasibility of converting Pittsburgh’s combined sewerage system to separate sewers and building a sewage treatment plant. There was no precedent, they claimed, for a city replacing the combined system with the separate system “for the purpose of protecting water supplies of other cities taken from the water course below” (Tarr, 1996a). The report calculated that financing such a project would cause the city to exceed its municipal indebtedness level and thus violate state law. Moreover, because the sewage treatment plant was intended for the protection of downstream communities, it would not give Pittsburgh any direct benefits, while downstream cities would still have to filter their water to protect against waterborne disease. Hence, they concluded that “no radical change in the method of sewerage or of sewage disposal as now practiced by the City of Pittsburgh is necessary or desirable.”
Although the engineering press received the Hazen-Whipple report with enthusiasm, PADOH health commissioner Dixon deemed it an insufficient response to his original request for a long-range plan for a comprehensive regional sewerage system. Dixon argued that water pollution had to be viewed from a health rather than a nuisance perspective and that the immediate costs of sewage treatment would be outweighed by the long-range health benefits. The time had come, Dixon stated, “to start a campaign in order that the streams shall not become stinking sewers and culture beds for pathogenic organisms …” (Tarr, 1996a). Given the political climate and financial limitations of the city, however, Dixon had no realistic means by which to enforce his order. In 1913 he capitulated and issued Pittsburgh a temporary discharge permit.
Pittsburgh Treats its Sewage
For Pittsburgh to be compelled to treat its sewage would require major policy and value changes on the part of both government officials and the public. In 1923, the Pennsylvania General Assembly enacted legislation proposed by then Governor Gifford Pinchot that created, within the Department of Health, a Sanitary Water Board whose function was to balance economic growth and improved water quality (Saville, 1931; Stevenson, 1923). The board created a stream classification system that designated streams into three categories for municipal and industrial users: (1) steams that were relatively clean and pure; (2) streams in which
pollution existed but could be controlled; and (3) streams that were so polluted they could not be used as public water supplies or for fishing and recreational purposes without treatment and, therefore, could continue to be used for the discharge of untreated wastes. Pittsburgh rivers fell in the third category; that is, they were to continue to be used as open sewers.
Although some local advances in sewage treatment were made in the 1920s and 1930s, most of the sewage discharged by municipalities into streams in the Ohio basin remained untreated. The sewage and industrial wastes from Pittsburgh and surrounding municipalities formed the basin’s largest pollution load and created a notable oxygen depletion zone in the Ohio River below the city. By the late 1930s, offensive sights and smells from the rivers had increased, causing “unsightly and malodorous conditions along all water fronts,” as sewage from the city as well as from upstream communities overwhelmed stream oxidation capacity (USPHS, 1944).12 Many water supplies suffered from problems of taste and odor, and sewage contamination threatened to adversely impact public health. Although filtration and chlorination had sharply reduced typhoid death rates, death rates from diarrhea and enteritis remained elevated. In addition, ongoing leakage increased the costs of water filtration for Pittsburgh residents. These obvious water quality problems caused a gradual increase in citizen awareness that water pollution had adverse economic consequences, reduced environmental quality, and threatened the public health.
In 1937, in response to the demands of various stakeholders including conservation groups and public health authorities for improved stream quality, the Pennsylvania General Assembly passed the Clean Streams Act (Casner, 1999). This act gave the Sanitary Water Board the power to issue and enforce waste treatment orders to all municipalities and most industries, notably excluding acid drainage from coal mines.13
Faced by a state law that provided the state authority with enforcement powers, the city and other western Pennsylvania towns were forced to seriously consider means to reduce their river pollution. Movement toward reform began in the late 1930s, only to be interrupted by the outbreak of World War II. In 1944, however, with the war coming to an end, the Sanitary Water Board announced comprehensive plans to reduce pollution of Pennsylvania streams. In a major step, the board required that all municipalities treat their sewage “to a primary degree.” In June l945, it issued orders to the City of Pittsburgh and 101 other Pennsylvania municipalities, including more than 90 Allegheny County industries, to cease the discharge of untreated wastes into state waterways. State officials decreed that these communities comply with the treatment orders by May of 1947 (Yosie, 1981).
Municipal and county officials reacted with consternation to the 1945 state waste treatment orders as they confronted difficult administrative and technological issues. The previous argument that state spending limits prevented spending for sewage treatment plants could no longer be used, since new legislation and court decisions in 1934 and 1935 permitted local governments to create authorities and exercise user charge financing to provide public services such as sewage treatment (Reader, 1954). Among the most pressing issues were
questions as to whether sanitary policy should be determined by each municipality or by a regional agency, how sewage treatment should be financed, whether a central treatment facility or multiple disposal plants would be most efficient, and what form of treatment technology made the most sense given the existing system of sewage collection and drainage and local conditions of population density and topography (Yosie, 1981).
The county commissioners proceeded to survey affected Allegheny County municipalities to determine if they would support the incorporation of a special district governmental authority to plan and implement a waste disposal program. Commissioner John J. Kane argued that a countywide waste treatment agency financed by revenue bonds could service participating localities without increasing their level of bonded indebtedness. User charges collected from households and industries connected to the treatment system could provide a self-financing mechanism for efficient and professional waste disposal service. Finally, these officials pointed out that the construction of an authority-managed treatment system would benefit the Pittsburgh metropolitan economy because of the demands it would create for building materials, labor, and technicians.
As a result of a set of meetings sponsored by the county commissioners with Allegheny County municipalities, a pro-authority consensus developed. Seventy-four Allegheny County communities in receipt of state treatment orders resolved that their individual waste disposal problems could be best solved “on a county-wide basis.” This provided the support that the county commissioners needed, and on December 19, 1945, acting under the recently passed Pennsylvania Municipal Authorities Act, the commissioners adopted a resolution to create a sanitary authority. Three months later, in March, 1946, the Secretary of the Commonwealth of Pennsylvania officially approved the formation of the Allegheny County Sanitary Authority (ALCOSAN) (Laboon, 1973). The county commissioners appointed John P. Laboon, who had previously served as Allegheny County Public Works Director, as chief engineer. The ALCOSAN board subsequently elected Laboon as chairman.
The ALCOSAN research staff conducted a number of studies and made several recommendations in 1945 and 1946 regarding the creation of an integrated sewage treatment system utilizing activated sludge technology. An early investigation by ALCOSAN showed that county municipalities with a population of 678,000 and industries collectively discharged 65 million gallons of wastewater per day. The authority decided to remove 50 percent of the biochemical oxygen demand generated by wastes entering its proposed activated sludge treatment plant, thereby complying with or exceeding discharge standards established by the Sanitary Water Board (Laboon, 1973). The research staff recommended that a single plant (located on a site situated on the north bank of the Ohio River north of Pittsburgh) would be more cost-effective than multiple plants and lead to fewer siting and odor objections from local populations (Yosie, 1981).
The ALCOSAN staff was also responsible for construction of an extensive intercepting sewer system, connecting various outfalls throughout the service district, and transporting wastes to the treatment works. Because the building of the interceptors promised extensive disruptions of transportation and industrial activity, ALCOSAN officials recommended subaqueous sewer construction (Laboon, 1973). As noted previously, individual municipalities would be responsible for owning and maintaining their own collection systems.
Although the financial and construction details of ALCOSAN were essential, the authority would become feasible only through delicate political negotiations with the City of Pittsburgh and with Allegheny County municipalities protective of their independence. Intensive
negotiations brought about agreements between the city and ALCOSAN, the essence of which was to enlarge the number of communities permitted into the service district and to increase city control over the board (Yosie, 1981). Initially, 67 surrounding municipalities joined ALCOSAN, but the refusal of others (see Box 2-2) to join reflected the governmental fragmentation of Allegheny County and the region and the difficulties of securing consensus on governmental issues.
To secure financing (estimated at $82 million in 1948), the city and ALCOSAN decided to utilize non-debt revenue bonds financed by the imposition of waste treatment charges on those households and industrial plants located within the service district (ALCOSAN, 1948). To determine service charges, sanitary officials metered water consumption levels at the household tap or plant site. State legislation required water utilities to furnish water meter readings to ALCOSAN and empowered the agency to terminate water service to individual customers delinquent in their payment of sewage treatment bills (Laboon, 1973). On October 1, 1958, after a four-year loan of $100 million had been secured, official dedication of the plant occurred. Wastewater treatment in and around the City of Pittsburgh became a long awaited reality (Yosie, 1981; Tarr and Yosie, 2004). However, the basic combined sewer collection system for the City of Pittsburgh remained, as it did for other neighboring municipalities, and continued to discharge untreated raw sewage through overflow relief valves into streams and rivers under wet weather conditions.
Sewer Tap-in Restrictions
The presence of aging and inadequate sewer systems in some of the region’s municipalities has slowed development and caused serious problems for towns seeking to increase their tax bases. During the 1990s, the Pennsylvania Department of Environmental Protection (PADEP) imposed tap-in restrictions on a number of communities in the Pittsburgh region because of problems such as wet weather overflow, overflows at treatment plants, and leaking sewers. Among those affected, for instance, were Rostraver Township, Pleasant Hills, Plum Boro, Cranberry, and East Huntingdon Township. In 2002, PADEP imposed tap-in restrictions on 31 Allegheny County municipalities including Bethel Park, Ross, Shaler, and Upper St. Clair. In Upper St. Clair, construction of new homes was put on hold because of such restrictions. Retail business was affected also, and in Shaler Township a new shopping mall was delayed and one retailer left the town because it was unable to tap into Shaler’s sewers (Fitzpatrick, 2002).
ALCOSAN and Sanitary Sewer Overflow
Several communities that were members of ALCOSAN had sanitary sewers rather than combined sewers (see also Figure 1-2). Stormwaters were intended to be handled by storm sewers, surface channels, or surface runoff into neighboring streams. Sanitary sewer flows, however, often included runoff from connected roof drains. Such connections were, according to one authority, the “construction standard” in Allegheny County and were common in other cities as well. These connections resulted in the sanitary sewer flows often being diluted. Under the system design planned by the authority, all flows into the treatment plant were to be regulated,
BOX 2-2 The Borough of Penn Hills is a suburban community located directly east of the City of Pittsburgh on roughly 19 square miles of hilly land (see Figure 1-1). It occupies parts of three watersheds: the Allegheny River on the northwest; Turtle Creek on the south, which runs into the Monongahela River; and Sandy Creek, a minor watershed in the central west region that drains to the Allegheny River. It has a total of 13 drainage areas. Originally known as Penn Township, it had a population of 15,000 in 1940 but experienced explosive growth in the post-World War II period, reaching a population peak of 62,000 in 1970. The federal Works Projects Administration’s real property inventory estimated that approximately 40 percent of the homes in the township had no indoor plumbing in 1934, depending on wells for water and probably on-lot septic tanks or privy vaults for waste disposal. In the postwar growth period these conditions changed rapidly. Water was provided by two quasi-public utilities, and developers supplied most new housing developments with sanitary sewers since Penn Hills subsurface conditions did not lend themselves to the use of septic systems. Some septic systems remained, however, as in the oldest area of the township bordering Pittsburgh, where some homes had septic tanks while others were connected to a combined sewer. In addition, Penn Hills was an old mining area, and as in many such communities, some homes still had straight pipes run into the old mines for sewage disposal. Few provisions were made for stormwater, with the expectation that it would drain on the surface or be channeled via short storm sewers into local creeks. Penn Hills began treating part of its sewage before World War II, and by 1955 the township possessed six treatment plants of various sizes that provided primary and secondary treatment. The reliance on multiple plants rather than a single centralized plant was necessitated by the hilly topography. By 1988, there were five such treatment plants, four of which had been constructed in the previous 10 years or so. By this date, most homes utilizing septic tanks had been forced to connect to sewers, although some newer developments on the fringe of the municipality still utilized such subsurface disposal methods. Penn Hills began to experience problems with its wastewater collection and treatment system during the period of its most rapid growth in the 1970s and 1980s. These problems related largely to a failure to provide funding for proper system maintenance, inefficient administration, and poor land development practices. Penn Hills also possessed a political culture that strongly resisted raising taxes in order to pay for improved services or to maintain those that existed. Even when sewer fees were levied, they were frequently not collected. Inadequate finances meant that the sewerage system was poorly maintained, with extensive inflow and infiltration problems, frequent incidences of sewage backed up in basements, and a failure to effectively provide for stormwater flows. In their haste to build, developers had exacerbated the system difficulties by building on steep slopes and hooking up roof gutters and driveway drains into sanitary sewers, even though the township began prohibiting such procedures in the late 1950s. The diversion of stormwater to sanitary sewers resulted in a large volume of stormwater entering the sanitary system. On wet weather days the normal flow was increased 5 to 30 times in volume. This led to hydraulic overloading of the existing wastewater treatment plants and state |
and they required that sanitary sewer overflow relief be provided. Sanitary sewer overflows (SSOs) were prohibited under the 1987 amendments to the Clean Water Act but action was not taken against ALCOSAN until 1996.
In the years following its founding, ALCOSAN built additional facilities, improved its treatment processes to meet higher levels set first by the Pennsylvania Sanitary Water Board and later by the U.S. Environmental Protection Agency (EPA) under the CWA. In fall 2003, 26 and
prohibition of any new tap-ins, slowing development in the borough. All the Penn Hills wastewater treatment plants utilized secondary treatment with activated sludge as the secondary process. Waste biosolids (sludge) from the activated sludge process were sometimes allowed to discharge into streams via a bypass mechanism during increases in the incoming flows to the plant. In addition, untreated wastewater was permitted to bypass the treatment plant during periods of wet weather because of the existence of sanitary sewer overflows. When the City of Pittsburgh and other Allegheny County municipalities created ALCOSAN in the 1950s, Penn Hills refused to join because it already had its own treatment plants and was unwilling to forgo its capital investments or the fees and patronage that went with their operation. In addition, it disliked the fee system that ALCOSAN had established. Two peripheral parts of the township, however, one on the northwest and one on the southwest, were connected with sewer systems in the neighboring township of Verona and with that of the City of Pittsburgh because of watershed boundaries. Both of these systems became part of ALCOSAN and Penn Hills was required to pay monthly fees for using their facilities. This fee situation caused further political discontent within the municipality. Throughout the 1980s and early 1990s, as state and federal water quality programs and contaminant standards were strengthened, Penn Hills experienced increasing problems with its sewerage system. These were compounded because of an inadequate funding base to upgrade the collection system and the treatment plants. One of the major difficulties was created by the decision of Penn Hills to cease dewatering its sludge and end its contracts for landfill disposal, a decision based on a financial shortfall. Sludge was permitted to build up at the treatment plants and then flow into the river on wet weather days along with untreated sewage. The result was a rise in coliform counts in the Monongahela River and a clear violation of the Clean Water Act. In 1989 and 1990, the Pennsylvania Department of Environmental Resources (PADER) ordered Penn Hills to improve conditions at its sewage treatment plants but the municipality failed to act and failed to provide legally required sewage discharge reports to the U.S. Environmental Protection Agency (EPA). In August 1991, the U.S. Attorney’s Office, acting on behalf of the EPA and PADER, asked for a preliminary injunction to force Penn Hills to stop dumping raw sewage into the Monongahela. A court granted the injunction and, in September 1993, followed with a permanent injunction obliging Penn Hills to comply with the Clean Water Act by January 1996. The joint EPA-Pennsylvania lawsuit accused Penn Hills of discharging pollutants in excess of its National Pollutant Discharge Elimination System (NPDES) permit limits; discharging raw sewage through unlawful bypasses within its sewerage system; failing to properly dispose of sludge; failing to properly maintain and operate its facilities; and failing to accurately monitor and report as required under its NPDES permits. In addition, charges were brought against two sewage plant employees for falsifying discharge records, with both being found guilty and one going to prison. During the next five years, Penn Hills spent approximately $50 million to upgrade its sewage system in order to prevent new violations of the Clean Water Act. A settlement of the lawsuit was reached in July 1998 in which Penn Hills agreed to pay a $525,000 penalty, improve its wastewater treatment operations, eliminate unauthorized overflows, and improve monitoring and reporting. In addition, it agreed to become a member of ALCOSAN (EPA, 1998). |
55 Allegheny County communities served by ALCOSAN had negotiated consent orders with the EPA, PADEP, and the Allegheny County Health Department for CSOs and SSOs, respectively (see also Chapter 1, footnote 1).
Water Supply and Wastewater Disposal Practices in Rural Southwestern Pennsylvania
Sanitary conditions in rural southwestern Pennsylvania and rural areas throughout the nation were generally considered primitive before the post-World War II period. As late as 1943, a text Municipal and Rural Sanitation noted that “At the present time, and in all probability for many years to come, excreta will be disposed of without water carriage at the vast majority of farmhouses, at residences in the smaller towns and villages …” (Ehlers and Steel, 1943). Studies by PADOH confirmed that rural farm areas and small towns often were poorly served by sanitary facilities and often relied on wells for drinking water located close to privies (PADOH, 1915).
The most common means for disposal of human waste was the privy vault. Privies differed by type, including surface privies where excreta accumulated on top of the ground and liquids were allowed to leach away; pit privies in which excreta fell into a pit in the ground; and drop privies that overhung brooks or rivers where the excreta dropped into the water to be carried away by the stream (Rosenau, 1927). Pit privies were considered threats to neighboring wells, while drop privies threatened downstream water supplies. Cesspools, usually of the percolating type, were also frequently used. In the smaller towns, some public sewers existed, although private sewers (straight pipes) from water closets or privies frequently drained into neighboring waterbodies. All of these types of privies and conditions existed in southwestern Pennsylvania during the early decades of the twentieth century (PADOH, 1915).
Some small towns had especially bad conditions. A 1915 survey by PADOH of the Allegheny River basin, for instance, noted that in the small town of Derry in Westmoreland County (about 2,000 population), about 200 small private sewers discharged into tributaries of the Allegheny River. In addition, many Derry residents used privies “of a shallow type, almost universally overflowing.” According to that report, all of the streams in the community were “badly polluted” (PADOH, 1915).14
An unusual feature of southwestern Pennsylvania was the number of small towns or “patch towns” established by coal mining companies. Coal companies constructed these towns in order to provide housing for miners near the mines. Homes in these patch towns were built according to a rather common model, with four “holer” privy vaults located in the back. Water was often provided through a spigot on the side of the house or, in more primitive communities, by wells. Many of these patch communities still exist today, and the company housing has long since been sold to private individuals. Although a substantial number of these homes have been modernized in recent years, many did not receive modern indoor plumbing until after the 1950s and some still rely on “straight pipes” to dispose of their untreated sewage into neighboring streams.15
In the 11-county Pittsburgh region today (see Box 1-2), Allegheny County is the only county with a health department. Throughout most of the twentieth century, PADOH had responsibility for all public health activities outside of incorporated municipalities and provided health services for the rural areas of the state. The state appointed and paid for a part time
14 |
In response to an order of the PADOH, Derry constructed a system of sanitary sewers and sedimentation tanks circa 1915. |
15 |
See the Virtual Museum of Coal Mining in Western Pennsylvania at http://patheoldminer.rootsweb.com/ for further information. |
medical director for each county, providing public health nurses, school medical inspectors, and sanitary inspectors (Smillie, 1939). With regard to water supply and sewerage, the state conducted sanitary surveys of various rivers basins, while after 1923 the Sanitary Water Board had the authority to grant permits for sewage treatment and construction of new sewers.
New residential development in rural areas increasingly distant from Pittsburgh, as well as older rural housing (both individual and community) and older coal mining patch towns, often did not have practical access to large municipal central sewerage systems. Thus, these communities and developments relied on a literal patchwork of community and on-lot sewage disposal methods. The lack of coordination, standardization, and oversight of system siting, design, construction, and maintenance resulted in inadequate and malfunctioning sewer systems in these areas. Substandard wastewater systems include straight piping of sewage from homes to streams or ditches, septic tanks discharging directly into streams or ditches, drywells and cesspits, homes connected to neighborhood or community straight pipes (“wildcat sewers”), sewage discharges into abandoned underground coal mines, and failed or malfunctioning community “package plant” treatment systems. In addition, because many of these substandard systems predate modern sewage regulations and ordinances, no records exist for a majority of them.
According to the Pennsylvania Association of Township Supervisors, the post-World War II housing boom in Pennsylvania resulted in an overloading of urban area central treatment systems, a proliferation of poorly operated small treatment systems, and between 500,000 and 1,000,000 malfunctioning on-lot systems throughout the Commonwealth. Concerns about surface water and groundwater deterioration, and the accompanying public health risks, led to the passage in 1966 of Act 537, the Pennsylvania Sewage Facilities Act.16 This act was subsequently amended to expand options for local-level cooperation and enforcement and to expand on-site wastewater treatment options (ELI, 1999).
Although Act 537 has addressed many sewage problems, southwestern Pennsylvania’s rural areas still face significant sewage disposal challenges. Many sewage problems accompany substandard, deteriorating housing where residents rely on fixed retirement income, low income jobs, or unemployment compensation. Local tax bases are often inadequate to support the required staffing and resources for data collection to adequately define local problems, to devise the sometimes unconventional solutions needed to address the problems, and to provide necessary management and record keeping. In some of these areas there may be added pressures from adjacent new home development or from economically struggling neighboring farms likely to be sold for new or second-home development.
In 1997, PADEP issued a guidance report Policy Establishing New Program Direction Policy for Act 537 Comprehensive Planning recognizing the special needs of rural municipalities (e.g., low development density, lack of available funding) and describing the department’s role in assisting these municipalities in finding both technical and financial solutions to sewage
problems. That report states that some low-income rural areas may require up to 90 percent grants to afford sewerage projects using conventional methods. It acknowledges that phased implementation and long-term goal setting may be needed to address rural sewage treatment issues, and it encourages the development of local management programs and decentralized (“noncentralized”) sewage treatment alternatives (PADEP, 1997a).
Also in 1997, PADEP issued the policies and procedures document Impact of Use of Subsurface Disposal Systems on Groundwater Nitrate Nitrogen Levels (PADEP, 1997b). That document details the requirements for technical studies, siting, and system design and technologies to avoid nitrate contamination of groundwater in vulnerable areas.
Acid Mine Drainage
The sulfuric acid discharge from coal mines has been the most pervasive and widespread water pollution problem in southwestern Pennsylvania’s industrial history (see also Chapters 3 and 4). The region’s bituminous coal has a high sulfur content and produces enormous acid loads. Drainage from extensive networks of abandoned underground mines; thousands of small, abandoned “country bank” mines (used for local domestic purposes); and large numbers of active commercial mining operations contributes to stream degradation throughout the region. Historically, acid mine drainage (AMD) destroyed fish communities and altered the flora along both small streams and major rivers, caused millions of dollars of damages to domestic and industrial water users, and increased the costs of water and sewage treatment (Casner, 1994).
Acid mine drainage also affects human quality of life and public health. AMD diminishes the quality of drinking water sources and impairs water delivery. Its corrosive action has destroyed pipes and pumps, forcing water authorities to build neutralizing plants as a component of their overall water treatment systems.
The coal industry also has incurred damages from AMD. The most immediate problems occurred at the mines themselves, where the corrosive action of the acidic water damaged and destroyed the pipes and pumps installed to remove water. Damaged machinery increased expenses and also hindered timely coal extraction, while leaks caused by corrosion increased the cost of energy production and damaged equipment. In highly acidic environments, bronze, lead, and wooden linings and covers were used to protect mining equipment (Casner, 1994).
As acid mine discharges moved into neighboring streams and rivers, other industries encountered degraded water, making treatment a necessity. The effect on the steel industry was severe, requiring all mills to have water treatment facilities. Railroads, which until the 1950s largely used coal-burning locomotives that depended on frequent water stops, were affected adversely as well. Their costs included larger coal bills, boiler repairs, and cleaning (Bardwell, 1953). American railroads were forced to build water treatment plants in an effort to avoid or reduce such costs. By 1934, rail lines had built 1,200 water treatment plants and were treating 90 billion gallons per year. Regional railroads developed water supply systems by building reservoirs above mining districts and running pipelines along rail networks (Crichton, 1927).
By the 1920s, municipalities, recreational users of the rivers, and industry in southwestern Pennsylvania had begun pushing government for solutions to counter the growing burden of AMD. For government policy makers, acidic water offered a significant engineering and policy dilemma because it hindered effective water treatment and presented an indirect public health threat. However, the economic importance of coal production in southwestern
Pennsylvania inhibited coercive action. Indeed, the coal industry argued emphatically that no “suitable” method existed for the treatment of acid mine water. Even if authorities contemplated action, the industry had achieved legal protection in a precedent set by the seminal 1886 case of Pennsylvania Coal Company v. Sanderson and Wife, which concerned destruction of the water supplies for a family farm near Scranton, Pennsylvania. In this case the Pennsylvania Supreme Court maintained that “the right to mine coal is not a nuisance in itself” and that the acidic substances entered the stream via natural forces that were beyond company control. The justices also considered the economic importance of the coal industry and its provision of jobs, arguing that “the trifling inconvenience to particular persons must sometimes give way to the necessities of a great community” (Casner, 2004). In 1905, when the Pennsylvania legislature passed the Purity of Waters Act, it specifically exempted “waters pumped or flowing from coal mines.” In 1923, at then Governor Gifford Pinchot’s initiative, and as noted previously, the Pennsylvania legislature created the Sanitary Water Board with investigatory and advisory powers. Again, however, state legislators specifically exempted AMD from possible proposed restrictions, upholding its protection under state law (Broughton et al., 1973; Wolman, 1947).
The first significant challenge to the protected status of coal mining came in 1923, in the case of the Pennsylvania Railroad v. Sagamore Coal, et al. when the Pennsylvania Supreme Court rendered a decision that undermined the 1886 Sanderson doctrine. In this case, the Pennsylvania Railroad successfully sued several coal mining companies for polluting its reservoir in the Indian Creek watershed (located 65 miles southeast of Pittsburgh) on the grounds that the acid pollution created a public health nuisance since it polluted the water supplied to several regional water companies. The court ruled that coal companies possessed “no right of any kind” to discharge acidic water into streams when the public made use of the water (Casner, 2004).
In the 1920s, pressures to resolve problems resulting from AMD emerged from different stakeholders including domestic and individual water users, industrial users, and sportsmen’s groups. Various strategies were suggested, but the one that gained the most favor involved sealing abandoned coal mines. Sealing of mines causes flooding of the mine voids, which substantially reduces the amount of oxygen, and thus acid production, in those voids. In 1924, the Pennsylvania Supreme Court ordered the mines above Indian Creek sealed in order to prevent further contamination of the Pennsylvania Railroad’s reservoir. In the 1920s, the U.S. Bureau of Mines studied mine sealing as a pollution abatement method. The technique initially seemed an inexpensive and simple remedy to an expensive and complex problem, but it failed to take into account the number and size of abandoned mines, natural geologic factors, and industry’s strong preference for the government to pay for sealing (Casner, 2004).
In the 1930s, mine sealing projects were undertaken under the auspices of the federal Civil Works Administration and the Work Projects Administration (WPA). In the first two years of the WPA program (1935-1937), sealing crews covered more than 47,000 openings at 1,527 sites in four states including Pennsylvania, Ohio, West Virginia, and Kentucky. Pennsylvania secured the most openings, with a reported 30,000 at 317 mines in 22 counties. The sealing temporarily produced the desired effect, and in 1940 the U.S. Public Health Service estimated that the average residual load of acid on the main Ohio River measured 48 percent of what it was prior to the sealing project (USPHS, 1944). On the Monongahela River, sealing reduced acid loads by 51 percent. On the Kiskiminetas River, a tributary of the Allegheny River and at that time the primary source of acidic water affecting the Pittsburgh water supply, reduction efficiency at abandoned mines achieved one of the highest levels with a decline of 78 percent
(Casner, 2004). Most public authorities through the 1950s considered the project an unqualified success. The program had reduced acid concentrations in the waters of the Ohio River basin and served as an excellent example of the benefits of federal cooperation with state efforts in water pollution control. Mine sealing, however, produced only temporary relief from acid mine drainage because seals frequently broke down and allowed air and water to enter or water to escape the mines. By the 1960s, it was clear that AMD pollution of Pennsylvania streams remained a major state environmental problem.
Beginning in the late 1960s, Pennsylvania, West Virginia, and the federal government enacted legislation requiring active mining operations to treat polluted water prior to discharge. The Clean Water Act instituted the National Pollutant Discharge Elimination System (NPDES), which required all “point sources” of pollution to apply for an NPDES permit and meet discharge water quality standards. Both Pennsylvania and West Virginia were granted primacy such that their respective agencies received authority under the Federal Water Pollution Control Act of 1972 to issue and enforce NPDES permits. Permitted discharge limits for mining operations are typically governed by “technology-based limits.” The act (which in 1977 became known as the Clean Water Act; see also Box 1-1) was amended by Congress in 1987 to establish the Section 319 Nonpoint Source Management Program, recognizing that regulated point sources in many regions of the country, accounted for only a minor share of the pollutant loadings.
While the CWA initiated federal oversight of pollution, creation of the federal Office of Surface Mining, Reclamation and Enforcement (OSM) and its Surface Mining Control and Reclamation Act (SMCRA) of 1977 brought federal oversight to the permitting of new coal mines. Title IV of SMCRA identified abandoned mine lands (AMLs) as mines that were abandoned or left in an inadequate state of reclamation prior to August 3, 1977. Title IV also created the Abandoned Mine Land Reclamation Fund supported by a tax on coal production. A portion of these funds has been distributed to eligible states and used to reclaim abandoned mines, reduce hazards, and make water quality improvements. As of 2003, the Abandoned Mine Land Reclamation Fund had a balance of about $1.5 billion (OSM, 2003; see Chapter 4 for further information). Section 403 of SMCRA assigns the following priorities to the expenditure of AML funds:
-
protection of public health, safety, general welfare, and property from extreme danger of adverse effects of coal mining practices;
-
protection of public health, safety, and general welfare from adverse effects of coal mining practices;
-
restoration of land and water resources and the environment previously degraded by adverse effects of coal mining practices, including measures for the conservation and development of soil, water (excluding channelization), woodland, fish and wildlife, recreation resources, and agricultural productivity;
-
protection, repair, replacement, construction, or enhancement of pubic facilities such as utilities, roads, recreation, and conservation facilities adversely affected by coal mining practices; and
-
development of publicly owned land adversely affected by coal mining practices including land acquired as provided in this title for recreation and historic purposes, conservation and reclamation purposes, and open space benefits.
The magnitude of the abandoned mine land problem dictated that much of the historic funding was devoted to priorities 1 and 2, while significant efforts to use the fund to remediate water (AMD) issues only began around 1995. Significant programs for AMD cleanup have been developed and are administered by the Commonwealth of Pennsylvania, EPA, OSM, and various watershed organizations.
SMCRA requires that any new mining permit be accompanied by a bond to cover the cost of reclamation in the event that the permittee is financially unable to do so. These bonds have not been assessed in amounts adequate to treat AMD and are based on surface disturbance. Typically, bond rates are less than $5,000 per acre. Bond forfeiture results from a finding by the state regulatory agency that the company is unable to fulfill its environmental requirements under its mining permit. The state then uses the bond amount to reclaim the surface disturbances.
Prior to enactment of the SMCRA, Pennsylvania established Operation Scarlift in the late 1950s specifically to deal with abandoned mines. Operation Scarlift constructed a series of lime neutralization treatment stations to neutralize some AMD discharges in severely affected watersheds. However, it was funded by a revenue bond, which when exhausted caused the program to become inactive in the 1970s. In recent years, Pennsylvania has instituted statewide programs to deal specifically with AMD discharges from bond forfeiture sites.
As noted previously, mine drainage is classified as a point source if it originates from an active, post-August 1977 mine. Discharges from these mines are governed by their respective NPDES permits. Discharges from mines that were abandoned prior to August 3, 1977, are considered nonpoint sources. They are unregulated and in most coal field watersheds are responsible for the overwhelming majority of metal ion and acidity loadings to surface waters. Policies regarding the states’ responsibilities in maintaining NPDES permit conditions on bond-forfeited AMD sites are an emerging issue in both Pennsylvania and West Virginia. Recently, in Pennsylvania, the liquidation of LTV Corporation’s coal assets placed five large underground coal mines along the Monongahela River under state responsibility. As a result, PADEP is evaluating ways to either operate LTV’s AMD treatment plants or find more efficient methods for treating AMD (Hopey, 2003).
Current and Anticipated AMD Loadings in the Pittsburgh Basin
Coal mines in the Pittsburgh basin,17 which generate about 5,500 tons of dissolved iron annually, also contribute to an acid loading of about 16,000 tons contained in 19 billion gallons of water (see Chapter 4 for a detailed discussion of the characteristics and effects of AMD in southwestern Pennsylvania). About one-third of the basin’s discharge from mines is treated by the mining industry. Abandoned mines generate the remainder and currently pollute many of the
major tributaries to the Monongahela and Ohio Rivers. If all of this mine water were untreated however, it would be sufficient to add substantial metal loadings and acidity to already impaired tributaries with possible localized, severe effects on the Monongahela River. The Monongahela River’s alkalinity at the West Virginia-Pennsylvania state line is about 36 mg/L and its low flow is about 6,000 cubic feet per second. This rate of mine drainage would supply about 200,000 tons of alkalinity per year. The majority of flooded mines are currently discharging net alkaline water, with soluble Fe 2+ concentrations in the range of 25 to 100 mg/L. Given the high volumes of these mine discharges, iron staining and oxygen depletion in the Monongahela River are more likely to be problematic than acidity. Thus, under all but low-flow periods (late summer, early fall); dilution will likely ensure that effects of additional mine pool discharges would be localized, with affected plumes extending along the banks of the river for miles. During low-flow periods, water movement between navigation pools is extremely slow and oxygen deficits in the rivers would be exacerbated by mine drainage. Probably the worst-case scenario would entail a neutral, net alkaline Monongahela River at the Pittsburgh Point (confluence of Allegheny and Monongahela Rivers) with enough suspended ferric hydroxide to color the river orange. Oxidation of ferrous to ferric ion would contribute to the river’s oxygen deficit, but a discussion of the effect on fish populations is beyond the scope of this report.
SUMMARY
The Allegheny, Monongahela, and Ohio River system has been central to the development, history, and identity of southwestern Pennsylvania. Abundant coal and other natural resources and the availability of convenient water and rail access within and beyond the immediate region facilitated economic growth and, at the same time, extensive air and water pollution in the City of Pittsburgh and surrounding communities from the mid-nineteenth century through the 1950s. With the decline of the steel industry in the late twentieth century, the region’s economic base shifted to other sectors, including medical research, technology, and higher education. While there has been a remarkable transformation and recovery of the region’s economy in the last two decades, many communities in southwestern Pennsylvania continue to experience significant economic problems resulting from the decades-long decline in mining and traditional manufacturing sectors. As a result, the population of the City of Pittsburgh declined steadily from about 520,000 in the 1970s to its present level of about 335,000. Despite this net loss of population, the Pittsburgh metropolitan area has been sprawling further onto rural land at rates that exceed other cities in the northeastern United States.
Although the environmental quality of the 11 counties of southwestern Pennsylvania and the City of Pittsburgh has improved dramatically in recent decades, pervasive water quality problems remain a legacy that transcends municipal, county, and even state lines. In this regard, acid mine drainage, effluent from on-lot septic systems, and raw sewage continue to enter local streams, the region’s three major rivers, and underlying groundwater in both urban and rural areas. These problems threaten the region’s public health, environment, economy, and image.
Many of the region’s current urban water quality problems can be traced to historical water supply and wastewater infrastructure decisions. The City of Pittsburgh, ALCOSAN, and its 83 serviced communities are facing extensive and costly regulatory action under the federal Clean Water Act for both combined and sanitary sewer overflows. Furthermore, some sewage-related water quality problems persist even in dry weather because the presence of aging and
deteriorating septic systems and sewer pipes that are a major source of sewage contamination to groundwater supplies. This problem is exacerbated by the fact that southwestern Pennsylvania is dominated by poor shallow soils, a high water table, and sloped terrain, making the region one of the most challenging in the country for use of on-site sewage treatment and disposal systems such as septic tanks and leach fields.
REFERENCES
ALCOSAN (Allegheny County Sanitation Authority). 1948. Report on the Proposed Collection and Treatment of Municipal Sewage and Industrial Wastes by the Allegheny County Sanitary Authority. Pittsburgh, PA.
Allan, J., D. Erickson, and J. Fay. 1997. The influence of catchment land use on stream integrity across multiple spatial scales. Freshwater Biology 37:149-161.
American Rivers, NRDC (National Resources Defense Council), Smart Growth America. 2002. Paving Our Way to Water Shortages: How Sprawl Aggravates the Effects of Drought. Washington, DC.
Anderson, R., K. Beer, T. Buckwalter, M. Clark, S. McAuley, J. Sams, and D. Williams. 2000. Water Quality in the Allegheny and Monongahela River Basins: Pennsylvania, West Virginia, New York, and Maryland (1996-98). Denver, CO: U.S. Geological Survey.
Bardwell, R. 1953. Water treatment for railway systems. Water Works and Sewage 80:9.
Belko, M. 2004. Pittsburgh lands Bassmaster Classic for 2005. Pittsburgh Post-Gazette, June 16. Available on-line at http://www.post-gazette.com/pg/04168/332700.stm. Accessed June 16, 2004.
Brookings Institution. 2003. Back to Prosperity: A Competitive Agenda for Renewing Pennsylvania. Washington, DC: Brookings Institution.
Broughton, R., T. Koza, and F. Selway. 1973. Acid mine drainage and the Pennsylvania courts. Duquesne Law Review 11:499-555.
Byington, M. 1910. A Homestead Court in Homestead: The Households of a Mill Town, 1907-1908. New York: Charities Publishing Committee.
Casner, N. 1994. Acid water: A history of coal mine pollution in western Pennsylvania, 1880-1950. Ph.D. dissertation, Carnegie Mellon University.
Casner, N. 1999. Polluter versus polluter: The Pennsylvania railroad and the manufacturing of pollution policies in the 1920s. Journal of Policy History 11:179-200.
Casner, N. 2004. Acid mine drainage and Pittsburgh’s water supply. In Devastation and Renewal: An Environmental History of Pittsburgh and Its Region, J. Tarr (ed.). Pittsburgh, PA: University of Pittsburgh Press.
Center for Watershed Protection (CWP). 2003. Impacts of impervious cover on aquatic systems. Watershed Protection Research Monograph No. 1. Ellicott City, MD: CWP.
Collins, T., E. Muller, and J. Tarr. 2003. Pittsburgh rivers: From urban industrial infrastructure to environmental infrastructure. Paper delivered at Conference on Rivers in History: Designing and Conceiving Waterways in Europe and North America. Pittsburgh, PA: Carnegie Mellon University.
Crichton, A. 1927. Disposal of drainage from coal mines. Proceedings of American Society of Civil Engineers 53:1656-1666.
Ehlers, V., and W. Steele. 1943. Municipal and Rural Sanitation, 3rd Ed. New York: McGraw-Hill.
ELI (Environmental Law Institute). 1999. Plumbing the Future: Sewerage and Sustainability in Western Pennsylvania. Washington, DC: ELI.
EPA (Environmental Protection Agency). 1998. U.S. and Pennsylvania settle clean water lawsuit against Penn Hills. EPA Environmental News.
EPA. 2001. Our Built and Natural Environments: A Technical Review of the Interactions Between Land Use, Transportation, and Environmental Quality. EPA 231-R-01-002. Washington, DC: EPA.
Fitzpatrick, D. 2002. Pipe dreams: Lack of sewer, water hookups may keep region out of running for projects. Pittsburgh Post-Gazette. Available on-line at http://www.post-gazette.com/businessnews/20020721sewer0721p3.asp. Accessed June 18, 2004.
Fuller, T., M. Shields, and S. Smith. 2002. Road to 2003: Update on Pennsylvania: The Economy, Jobs, Forecasts and Telecommunications. University Park, PA: Pennsylvania State University Center for Economic and Community Development.
Fuller, T., M. Shields, and S. Smith. 2003. Road to 2004: Update on Pennsylvania: The Economy, Jobs, Forecasts and Telecommunications. University Park, PA: Pennsylvania State University Center for Economic and Community Development.
Fulton, W., R. Pendall, M. Nguyen, ad A. Harrison. 2001. Who Sprawls Most? How Growth Patterns Differ Across the U.S. Washington, DC: Brookings Institution.
Galli, J. 1990. Thermal impacts associated with urbanization and stormwater management best management practices. Washington, DC: Metropolitan Washington Council of Governments, Maryland Department of the Environment.
Gregory, G. 1974. A study in local decision making: Pittsburgh and sewage treatment. Western Pennsylvania Historical Magazine 57:25-42.
Harper, J. 1997. The formation of Pittsburgh’s Three Rivers. Pennsylvania Geology 28(3/4):4.
Herlihy, A., J. Stoddard, and C. Johnson. 1998. The relationship between stream chemistry and watershed land cover in the mid-Atlantic region of the U.S. Water, Air, and Soil Pollution 105:377-386.
Hopey, D. 2003. LTV OKs plan to treat mine drainage: Trust fund set up to pay for cleanup, reclamation. Pittsburgh Post-Gazette. Available on-line at http://www.post-gazette.com/pg/03247/217887.stm. Accessed April 19, 2004.
Jones, K., A. Neale, M. Nash, R. van Remortal, J. Wickham, K. Riitters, and R. O'Neill. 2001. Predicting nutrient and sediment loading to streams from landscape metrics: A multiple watershed study from the United States mid-Atlantic Region. Landscape Ecology 16:301-312.
Katz, B. 2002. The new metropolitan agenda: Speech to Southwestern Pennsylvania Smart Growth Conference, June 9.
Katz, B. 2004. Pittsburgh: The road to reform. Op-ed column. Pittsburgh Post-Gazette, January 18. Available on-line at www.brookings.edu/views/op-ed/katz/2004118.
Koppes, C., and W. Norris. 1985. Ethnicity, class, and mortality in the industrial city. Journal of Urban History 11:259-279.
Laboon, J. 1973. Chronological highlights of the history of the Allegheny County Sanitary Authority. Manuscript: 1-2. Pittsburgh, PA.
Lanpher, E., and C. Drake. 1930. City of Pittsburgh: Its Water Works and Typhoid Fever Statistics. Pittsburgh, PA: City of Pittsburgh.
McElfish, J., Jr., and S. Casey-Lefkowitz. 2001. Smart Growth and the Clean Water Act. Washington, DC: Northeast-Midwest Institute.
McElwaine, A. 2003. Slag in the park. In Devastation and Renewal: An Environmental History of Pittsburgh and Its Region, J. Tarr (ed.). Pittsburgh, PA: University of Pittsburgh Press.
McKay, G. 2002. Homes in Summerset at Frick Park blend old-time style, new urban amenities. Pittsburgh Post-Gazette, February 16.
Melosi, M. 2000. The Sanitary City: Urban Infrastructure in America from Colonial Times to the Present. Baltimore, MD: Johns Hopkins University Press.
Ogle, M. 1996. All the Necessary Conveniences: American Household Plumbing, 1840-1890. Baltimore, MD: Johns Hopkins University Press.
OSM (Office of Surface Mining). 2003. Abandoned Mine Land Reclamation: Reclamation of Abandoned Mine Land That Took Place Before the Surface Mining Law Was Passed n 1977. Available on-line at http://www.osmre.gov/annualreports/03aml.pdf.
PABS (Pennsylvania Biological Survey). 1998. Inventory and Monitoring of Biotic Resources in Pennsylvania: Current Ecological and Landscape Topics, Volume 1. Harrisburg, PA: Department of Conservation and Natural Resources.
PADEP (Pennsylvania Department of Environmental Protection). 1997a. Policy Establishing New Program Direction Policy for Act 537 Comprehensive Planning. 362-2206-007. Harrisburg, PA: Bureau of Water Quality Protection.
PADEP. 1997b. Impact of Use of Subsurface Disposal Systems on Groundwater Nitrate Nitrogen Levels. 362-2207-004. Harrisburg, PA: Bureau of Water Supply and Wastewater Management.
PADOH (Pennsylvania Department of Health). 1911. Fourth Annual Report of the State Department of Health. Harrisburg, PA: PADOH.
PADOH. 1915. Report on the Sanitary Survey of the Allegheny River Basin. Harrisburg, PA: PADOH.
Pearson, W., and B. Pearson. 1989. Fishes of the Ohio River. Ohio Journal of Science 89(5):181-187.
PEC (Pennsylvania Environmental Council). 2003. Three Rivers Conservation Plan: Draft. Pittsburgh, PA: PEC.
Peterson, J. 1979. The impact of sanitary reform upon American urban planning. Journal of Social History 13:84-89.
Reader, F. 1954. Financing municipal sewage treatment facilities in Pennsylvania by use of municipal authorities. Dickinson Law Review 58:335-336.
Rosenau, M. 1927. Preventive Medicine and Hygiene, 5th Ed. New York: D. Appleton and Co.
Roth, N., J. Allan, and D. Erickson. 1996. Landscape influences on stream biotic integrity assessed at multiple scales. Landscape Ecology. 11:141-156.
Saville, T. 1931. Administrative control of water pollution. Transactions, American Institute of Chemical Engineers 27:74-77.
Scheuler, T. 1994. The importance of imperviousness. Watershed Protection Techniques 1:100-111.
Shields, M. 2002. Pennsylvania’s Rural Economy: An Analysis of Recent Trends. Pennsylvania State University’s College of Agricultural Sciences Agricultural Research and Cooperattive Extension. Available on-line at http://cecd.aers.psu.edu/pubs/PA_rural_economy_trends.pdf.
Smillie, W. 1939. Public Health Law, 2nd. Ed. New York: The Commonwealth Fund.
Snow, F. 1907. Administration of Pennsylvania laws respecting stream pollution. Proceedings of the Engineers’ Society of Western Pennsylvania 23:266-283.
Stevenson, W. 1923. Pennsylvania sanitary water board. Engineering News Record 91:684-85.
Sustainable Pittsburgh. 2003. Southwestern Pennsylvania Citizens’ Vision for Smart Growth: Strengthening Communities and Regional Economy. Pittsburgh, PA: Sustainable Pittsburgh.
Tarr, J. 1989. Infrastructure and city-building in the nineteenth and twentieth centuries. In City at the Point: Essays on the Social History of Pittsburgh, S. Hays (ed.). Pittsburgh, PA: University of Pittsburgh Press.
Tarr, J. 1996a. Disputes over water-quality policy: Professional cultures in conflict, 1900-1917. In The Search for the Ultimate Sink: Urban Pollution in Historical Perspective, J. Tarr (ed.). Akron, OH: University of Akron Press.
Tarr, J. 1996b. The separate vs. combined sewer problem: A case study in urban technology design choice. In The Search for the Ultimate Sink: Urban Pollution in Historical Perspective, J. Tarr (ed.). Akron, OH: University of Akron Press.
Tarr, J., and T. Yosie. 2004. Critical decisions in Pittsburgh water and wastewater treatment. In Devastation and Renewal: An Environmental History of Pittsburgh and Its Region, J. Tarr (ed.). Pittsburgh, PA: University of Pittsburgh Press.
Tarr, J., S. Mershon, et al. 2002. Sewerage problems in Penn Hills, PA, 1930-1997, unpublished. Pittsburgh, PA: Carnegie Mellon University.
Thompson, J. 1948. A financial history of the City of Pittsburgh, 1816-1910. Ph.D. dissertation, University of Pittsburgh.
Tierno, M. 1977. The search for pure water in Pittsburgh: The urban response to water pollution, 1893-1914. Western Pennsylvania Historical Magazine 60:23-36.
Trout, H., S. Pfaff, and J. Matviya. 2001. An Overview of the Summerset at Frick Park Project. In proceedings Brownfields 2001, Chicago.
USGS (United States Geological Survey). 1995. National Water Quality Assessment Program- The Allegheny-Monongahela River Basin. Available on-line at http://pa.water.usgs.govreports/fs_137-95/report.html. Accessed April 4, 2004.
USPHS (United States Public Health Service). 1944. Ohio River Pollution Control: Report of the Ohio River Committee. 78th Cong., 1st sess. H. Doc. 266.
Watershed Management Institute. 1997. Institutional Aspects of Runoff Management: A Guide for Program Development and Implementation. Crawfordville, FL: Watershed Management Institute.
Wolman, A. 1947. State responsibility in stream pollution abatement. Industrial and Engineering Chemistry 39:561-565.
WPC (Western Pennsylvania Conservancy). 2004. Conservation Reserve Enhancement Program for Ohio River Basin in Western Pennsylvania. Pittsburgh, PA: WPC.
Yosie, T. 1981. Retrospective analysis of water supply and wastewater policies in Pittsburgh, 1800-1959. Doctor of Arts dissertation, Carnegie Mellon University.