7
Medical Radiation Studies
INTRODUCTION
Studies of patients irradiated for the treatment or diagnosis of diseases have provided considerable information for the understanding of radiation risks, particularly for specific cancer types, including thyroid and breast cancer (IARC 2000; UNSCEAR 2000b). Today, approximately 50% of cancer patients are treated using radiation (Ron 1998), and several million cancer survivors are alive in the United States, emphasizing the importance of investigating the long-term consequences of radiotherapy and examining the features of epidemiologic studies of medical radiation.
Large cohorts of radiation-treated patients who have been followed for long periods are available, allowing evaluation of cancer and other late effects. Population-based cancer registries in many countries have been used to identify these patients and to facilitate patient enrollment, thus allowing investigators to determine the risks of a second primary cancer after treatment with radiation for a primary cancer (Boice and others 1985). The characteristically detailed radiotherapy records for cancer patients and patients treated for nonmalignant conditions allow precise quantification of the doses to the organs of individuals, which in turn facilitates the evaluation of dose-response relationships. Frequently, patients with the same initial condition that receive treatments other than radiation are available for comparison, although the clinical indications for treatment may differ.
In most cases, patients received high doses of radiation on the order of 40–60 Gy to the targeted region, aimed at producing cell killing. These “high” doses would decrease with distance from the target tissue, and some tissues might receive doses that are referred to in this report as “low dose” (100 mGy or less). The use of such studies to estimate the effect of low-dose exposures raises a number of questions. The exposures were generally only partial-body exposures in persons who were ill, possibly resulting in a different risk than an equivalent whole-body uniform exposure. Because of their disease, patients may have a different sensitivity to radiation-induced disease than persons who do not have the disease. However, these studies are valuable and will likely become more important in the next decade, both for radiation protection of patients and for radiation protection in general because they provide a unique opportunity to address the following issues:
-
Effects of different radiation types
-
Risk of specific tumor types
-
Effects of potential risk modifiers, including sex, age, and exposure fractionation
-
Possible genetic susceptibility to radiation-induced cancer
In addition to studies of cancer survivors, long-term studies of patients who received radiation therapy for benign conditions such as enlarged tonsils and tinea capitis have also provided important information about radiation-induced cancer risk (UNSCEAR 2000b). These patients are particularly important in the evaluation of radiation risks in the absence of the possibly confounding effects of the malignant disease being treated and/or of concomitant therapy for cancer. Diagnostic radiation procedures, in contrast, generally result in small doses to target organs, and most studies of such exposure provide little information about radiation risks. A number of procedures, however, in particular repeated examinations of air collapse therapy for tuberculosis and of spine curvature for scoliosis, have resulted in sizable doses to specific tissues, and studies of patients who have undergone these examinations provide valuable information on radiation risks (UNSCEAR 2000b). It is noted that, although no informative studies are available, the recent use of computed tomography (CT) can deliver sizable doses, typically of the order of tens of millisieverts per examination (Brenner and Elliston 2004); UNSCEAR (2000b) reports cumulative doses of the order of 100 mSv for children.
As in the other review chapters in this report, studies were judged to be informative for the purpose of radiation risk
estimation if (1) the study design was adequate (see Chapter 5 concerning informative study designs and limitations); (2) individual quantitative estimates of radiation dose to the organ of interest were available for the study subjects; (3) if so, the details of the dose reconstruction approach were evaluated; and (4) a quantitative estimate of disease risk in relation to radiation dose—in the form of an estimated relative risk (ERR) or excess absolute risk (EAR) per gray—was provided.
Overall, more than 100 studies of patients receiving diagnostic or therapeutic radiation have evaluated the association between exposure to radiation and risk of cancer at multiple sites (IARC 2000; UNSCEAR 2000b). Studies that provide information about the size of radiation cancer risks are reviewed in detail in this chapter. Articles included in this chapter were identified principally from searching the PubMed database of published articles from 1990 through December 2004. Searches were restricted to human studies and were broadly defined: key words included radiation; neoplasms; cancers; radiation-induced; medical exposures; radiotherapy; diagnostic radiation; and iodine-131. Articles were also identified from UNSCEAR (2000b), from the references cited in papers reviewed, and from direct contacts with some of the main scientists who have been involved with studies of medical exposures in recent years. The data and confidence intervals are those given in the cited papers.
MEDICAL USES OF RADIATION
Medical use of radiation usually occurs under three circumstances: (1) treatment of benign disease, (2) diagnostic examination, and (3) treatment of malignant disease (Table 7-1). Diagnostic imaging using X-rays goes back to the time of Roentgen’s discovery in 1896. Diagnostic procedures, particularly the widespread use of X-rays, continue to be the most common application of radiation in medicine, even as non-ionizing radiation methods—ultrasound and magnetic resonance imaging—have become more generally accepted. Approximately 400 million diagnostic medical examinations and 150 million dental X-ray examinations are performed annually in the United States (Mettler and others 1996). On average, each person receives at least two examinations per year. The annual individual and collective effective doses from diagnostic medical X-rays have been estimated as 0.5 mSv and 130,000 person-Sv (UNSCEAR 2000b).
The range of X-ray techniques used includes radiography, fluoroscopy, CT, interventional radiology, and bone densitometry. These procedures are intended to provide diagnostic information and in principle are conducted with the lowest practicable levels of patient dose to meet clinical objectives. Ranges of typical doses from various medical diagnostic exposures are shown in Table 7-1.
TABLE 7-1 Estimated Range of Effective Doses from Diagnostic Radiation Exposures
Procedure |
Type of Examination |
Range of Doses |
Conventional simple X-rays |
Chest films X-rays of bones and skull X-ray of abdomen |
0.02–10 mGy |
Conventional complex X-rays |
GI series Barium enema Intravenous urogram |
3–10 mGy |
Computed tomography (CT) |
Head injuries Whole-body examinations |
5–15 mGy |
Spiral CT |
Head injuries Whole-body examinations |
10–20 mGy |
Angiography |
Coronary, aortic, peripheral, carotid, abdominal |
10–200 mGy |
Interventional procedures |
Angioplasties with stent placement Percutaneous dilatations, closures, biopsy procedures |
10–300 mGy |
Internal emitters |
Radioisotope studies |
3–14 mSv |
Although doses of single procedures are typically low, there is concern that populations of pediatric patients who may need repeated exams over time to evaluate their pulmonary, cardiac, urinary, or orthopedic conditions may receive relatively high cumulative doses. Similarly, adult patients may also require repeated examinations to evaluate fracture healing, or progression of pulmonary disease, or the regression or progression of neoplastic lesions.
In contrast, therapeutic exposures are less frequent, and the dose levels are higher in view of the different purpose. Currently, radiotherapy is used mainly for the treatment of cancer, where the intention is to deliver a lethal dose to malignant tissue within a well-defined target volume, while minimizing the irradiation of surrounding healthy tissue. In the past, high doses of radiation have also been used for the treatment of a number of benign conditions, such as enlarged thymus and ringworm of the scalp (tinea capitis). Doses from radiotherapy to the target organs are generally above 1 Gy (and typically in the range of 50–60 Gy for the treatment of malignant diseases). Radiotherapy involves mainly partial-body irradiation, however; hence very different doses are delivered to different organs or tissues of the body. Doses to distant organs are generally considerably lower (of the order of fractions of a gray), and studies of cancer risk in these organs are therefore potentially informative for the assessment of risks associated with low-level exposure. Further, many of the patients treated with radiotherapy received frac-
tionated doses, and studies of these patients provide the potential to study the effects of exposure fractionation and protraction.
Radiotherapy for Malignant Disease
Studies of second cancer following radiotherapy have generally focused on patients treated for cervical cancer, breast cancer, Hodgkin’s disease (HD), and childhood cancers (i.e., patients that generally have a favorable long-term prognosis). Survivors of these cancers may live long enough to develop a second, treatment-related malignancy. It should be noted that chemotherapy and/or hormonal therapy used in the treatment of cancers is a potential confounding factor in investigations of the risk of a second primary cancer.
Cervical Cancer
The treatment of cervical cancer involves external beam radiotherapy or radium or cesium in applicators to deliver high local doses of X-rays and gamma rays to the cervix uteri and adjacent organs in the abdomen and pelvic area. Treatment is usually successful, and patients survive for years after radiotherapy. Although doses to the cervix are very high (typically 40–150 Gy), doses to distant organs are significantly lower: of the order of 0.1 Gy to the thyroid, 0.3 Gy to the breast and the lung, 2 Gy to the stomach, and 7 Gy to the active bone marrow (Kleinerman and others 1995).
Most of the information on second cancers following radiotherapy for cervical cancer comes from an international cohort study of approximately 200,000 women treated for cervical cancer. The study involved the follow-up, based on 15 cancer registries in eight countries (Canada, Denmark, Finland, Norway, Sweden, the United Kingdom, the United States, and Yugoslavia [Slovenia]), of a multinational cohort of nearly 200,000 women patients treated for cancer of the cervix after 1960. In 1985, Boice and colleagues reported on 5146 second cancers that were diagnosed in this cohort up to 1980 and showed an increased risk of cancer following radiotherapy at a number of sites (Boice and others 1985). Kleinerman and coworkers (1995) extended the follow-up of this cohort, adding an additional 10 years of incident cases. Several registries from the original study were retained, and other registries were added to increase the number of nonexposed comparison subjects. A total of 7543 cases were included. This study confirmed earlier findings of increased risk of malignancies following radiotherapy and the persistence of increased risk over time.
Case-control studies of specific cancer types, nested within this cohort, allowed the reconstruction of individual doses to specific organs and the estimation of site-specific cancer risks (Boice and others 1987, 1988, 1989). These studies are based on incidence data; the numbers of exposed and unexposed patients were large; there was long and complete follow-up (hundreds of cases and controls, with followup of 10–20 years or more); chemotherapy was rarely used; and the existence of radiotherapy records facilitated the development of a comprehensive dose reconstruction system to estimate individual doses.
In an expanded case-control study nested within this international cohort (Boice and others 1988), radiation doses for selected organs were reconstructed from original radiotherapy records. Very high doses, of the order of several hundred grays delivered to the cervix, significantly increased the risks for cancers of the bladder, rectum, and vagina and possibly bone, uterine corpus, cecum, and non-Hodgkin’s lymphoma (NHL). Doses of several grays increased the risks for stomach cancer and for leukemia. The ERR1 for stomach cancer was 0.54 Gy−1 (90% CI 0.05, 1.5), with an excess attributable risk of 3.16 per 104 person-years (PY) per gray (0.05, 10.4), based on 348 cases and 658 controls. A nonsignificant twofold increase in the risk of thyroid cancer was observed, with an average dose of 0.11 Gy (43 cases and 81 controls).
More detailed dose-response investigations were carried out for leukemia and breast cancer after treatment for cervical cancer. The case-control study of leukemia risk (Boice and others 1987) included 195 cases and 745 controls, of whom 181 and 672, respectively, had received radiotherapy. Radiation dose to the active bone marrow was estimated from detailed radiotherapy records of the subjects. Radiation exposure did not affect the risk of chronic lymphocytic leukemia (CLL; 52 cases). For other forms of leukemia taken together (143 cases), there was a significant twofold increase in risk associated with radiotherapy; the risk increased with increasing dose up to about 4 Gy and then decreased at higher doses and was modeled adequately by a linear-exponential function. The linear term of this model for leukemia other than CLL provides an estimate of the ERR per gray in the low-dose range, where cell killing is negligible; this estimate is 0.88 Gy−1 (standard error = 0.69).
The case-control study of breast cancer included 953 cases and 1806 controls (Boice and others 1989). Radiation doses to the breast (average 0.31 Gy) and ovaries (average 32 Gy) were reconstructed from original radiotherapy records. Overall, there was no association between radiotherapy and risk of breast cancer. Among women with intact ovaries (561 cases), radiotherapy was associated with a significant reduction of risk, probably attributable to cessation of ovarian function. Among women with no ovaries, there was a slight increase in breast cancer risk and a suggestion of a dose-response with a relative risk (RR)2 of 1.0, 0.7, 1.5, and 3.1, respectively, for the dose groups 0, 0.01–0.24, 0.25–0.49, and 0.5 + Gy. From these data, UNSCEAR (2000b)
estimated an ERR per gray of 0.33 (< −0.2, 5.8) for women with no ovaries and of −0.2 (< −0.2, 0.3) overall.
A cohort study of second cancer risk following radiation therapy for cancer of the uterine cervix was also carried out in Japan among 11,855 patients (Arai and others 1991). Significant excesses of leukemia and of cancers of the rectum, bladder, and lung were observed. No estimation of organ dose is available.
Hodgkin’s Disease
The large radiation therapy fields used to treat HD and the young age and long survival of patients provide an opportunity to study the risk of second cancer after exposure to ionizing radiation. Most patients, however, in the past 20 years, have been treated with a combination of radiotherapy and chemotherapy.
Following a first report by Arseneau and collaborators (1972), a number of authors have studied the risk of second cancer following treatment for HD (Boivin and others 1984). Initial reports focused mainly on the risk of leukemia following this treatment, but as longer follow-up periods were considered, an excess risk of a number of solid cancers (in particular breast and lung) became apparent.
The results of the first multinational study were published in 1987 by Kaldor and collaborators. The study involved the follow-up (based on 11 cancer registries in seven countries: Denmark, Finland, Norway, Sweden, Slovenia, Canada, and the United Kingdom) of a cohort of 28,462 patients treated for HD between 1950 (in the earliest countries) and 1984. Increases in the risk of NHL, leukemia, lung, bladder, and breast cancer were reported in this cohort. No treatment information was available in this study, and no information is provided on radiation risks. Nested case-control studies of leukemia and of lung cancer were carried out, allowing reconstruction of individual doses for the subjects and estimation of site-specific cancer risks (Kaldor and others 1990a, 1992).
The case-control study of leukemia included 163 cases and 455 controls. Radiation dose to the active bone marrow was estimated for subjects who had undergone radiotherapy, and doses were classified into three categories (<10, 10–20, and 20+ Gy). Among patients who did not receive chemotherapy, a significant increase in the risk of leukemia was seen at doses of more than 20 Gy (Kaldor and others 1990a).
Another case-control study from the same collaborative group involved 98 cases of lung cancer occurring between 1960 and 1987 and 259 matched controls (Kaldor and others 1992). Radiation dose to the lung as a whole was estimated for the 60 cases and 275 controls who had undergone radiotherapy, and doses were classified into three categories (<1, 1–2.5, and 2.5+ Gy). Among patients treated with radiotherapy alone, there was a nonsignificant increase in risk in relation to radiation dose level. It is noted that the follow-up was short in this study, with three-quarters of the lung cancer cases having been diagnosed within 10 years of their initial disease (Kaldor and others 1992).
In 1995, Boivin and collaborators published results of a joint Canada-U.S. study of second cancer risk among 10,472 patients treated for HD between 1940 and 1987. A total of 122 leukemia and 438 solid tumors were found, and nested case-control studies were carried out. Significant increases in the risk of cancers of the respiratory system, intrathoracic organs, and female genital system were observed among patients followed for 10 years or more after surgery. Estimates of organ doses were not available, and analyses by level of radiation dose are not shown.
Van Leeuwen and collaborators (1995) conducted a case-control study of lung cancer nested in a cohort of 1939 patients treated for HD between 1966 and 1986 in the Netherlands. Radiation dose to the parenchyma, bronchi, and trachea were estimated for patients who had received radiotherapy (30 cases and 82 controls). A statistically significant increase in the risk of lung cancer was observed, with an RR of 9.6 (95% CI 0.93, 98.0) for patients who had received 9 Gy or more compared to patients who had received less than 1 Gy. The increase was greater among those who either continued smoking or started smoking after diagnosis, and a multiplicative interaction was observed between radiation dose and tobacco smoking.
Swerdlow and collaborators (2001) carried out a nested case-control study of lung cancer in a cohort of 5519 patients with HD treated in Britain between 1963 and 1993. The study included 88 cases and 176 controls for whom treatment and other risk factor information was abstracted from medical records. An increased risk of lung cancer following radiotherapy was observed. No individual reconstruction of dose to the lung was carried out.
Travis and colleagues (2002) carried out a case-control study of lung cancer nested within a multinational cohort of 19,046 HD patients diagnosed between 1965 and 1994 and reported to population-based cancer registries in Connecticut, Iowa, Denmark, Finland, the Netherlands, Sweden, and Canada (Ontario). The study included 222 cases and 444 matched controls. Nineteen of the cases were included in the previous case-control study by Kaldor and coworkers (1992). Dose to the specific location of the lung where the tumor had developed (and to a comparable location for matched controls) was calculated from radiotherapy records. The mean dose was 27.2 Gy in cases and 21.8 Gy in controls. In subjects who had not undergone chemotherapy, a significantly increased risk of lung cancer was observed (odds ratio [OR]3 5.9; 95% CI 2.7, 13.5) for a dose of 5 Gy or more. A significant trend in risk was observed with increasing dose.
In a follow-up to this study, Gilbert and colleagues (2003) analyzed radiation effects among 227 lung cancer cases and
455 controls (the 199 cases and 393 controls from the Travis 2002 study who had adequate radiation dose information and 28 cases and 62 controls from the Dutch study of van Leeuwen and others 1995). Doses to the lung ranged from zero to more than 60 Gy; the distribution of doses was bimodal, with most subjects having received doses of less than 5 or more than 30 Gy. To account for a possible latent period between radiation exposure and lung cancer resulting from that radiation exposure, only doses received more than 5 years in the past were considered. Among the 146 cases and 271 controls who had received radiotherapy more than 5 years in the past, a significant association was seen between radiation dose and risk of lung cancer, with an ERR per gray of 0.15 (95% CI 0.06, 0.39). There was little evidence for nonlinearity of the dose-response, despite the fact that the majority of patients received doses to the lung in excess of 30 Gy. Information about smoking and radiotherapy was available for the study subjects. A multiplicative interaction was seen between radiation dose and tobacco smoking and an additive interaction with chemotherapy. The ERRs for men and women were respectively 0.18 (0.063, 0.52) and 0.044 (−0.009, 0.53); the difference between the sexes was not statistically significant.
Breast cancer following treatment for Hodgkin’s disease has also been studied in a number of cohorts. Travis and collegues (2003) carried out a nested case-control study of breast cancer in a cohort of 3817 one-year survivors of HD diagnosed at age 30 years or younger between 1965 and 1994 and included in cancer registries in Iowa, Denmark, Finland, Sweden, the Netherlands, and Ontario, Canada. Individual doses to the area of the breast from which the tumor arose were reconstructed using detailed radiotherapy records and results of experiments with phantoms. Mean dose delivered to the location of the breast where cancer developed was 25.1 Gy (range: 12.0–61.3 Gy) in cases and 21.1 Gy (range 0–56.0 Gy) in controls. The study included 105 cases and 266 controls. A significant increase in the risk of breast cancer was seen following doses of 4 Gy or more (OR 3.2; 95% CI 1.4, 8.2); the increase remained significant even following very high doses (OR 8, 95%; CI 1.6, 26.4, of 40 Gy or more). No significant association between age at exposure or reproductive history was seen in this study, but the risk was lowered among women who received 5 Gy or more to the ovaries or who were also treated with alkylating agents. The estimated ERR per gray for women who did not receive alkylating agent chemotherapy or high radiation doses to their ovaries was 0.15 (95% CI 0.04, 0.74).
Van Leeuwen and colleagues (2003) also studied the risk of breast cancer among female survivors of HD treated in the Netherlands. The study included 48 cases who developed breast cancer 5 years or more after HD diagnosis and 175 matched controls. It should be noted that 40 of the 48 cases in the study of van Leeuwen and colleagues were also included in the study by Travis and coworkers (2003). The object of the study was to evaluate the joint roles of radiation dose, chemotherapy, and hormonal factors in breast cancer following HD. As in the Travis study, the risk of breast cancer increased with radiation dose up to at least 40 Gy. A substantial risk reduction was associated with chemotherapy, which affects menopausal age, suggesting that ovarian hormones promote tumorigenesis after radiation-induced initiation. No estimate of ERR or EAR4 per gray is given. Little if any increased risk was seen for patients treated after age 30.
Most recently, Dores and colleagues (2002) studied the risk of second cancers in general among 32,581 HD patients (including 1111 25-year survivors of HD) registered in 16 population-based cancer registries in North America and Europe. A total of 2153 second cancers were observed between 1935 and 1994. As before, significant increases in the risk of a number of second malignancies were observed. Although the elevated risks of cancers of the stomach, breast, and uterine cervix appeared to persist for 25 years or more, an apparent decrease in the risk of other solid tumors is suggested. These cohort studies, although they provide important information concerning treatment-related second neoplasms and their patterns of risk over time, do not provide quantitative information on the risk of radiation-induced cancer because of the absence of individual dose estimates.
The risks of breast, thyroid, and gastrointestinal cancers were also investigated in patients treated for HD at Stanford University Medical Center (Hancock and others 1991, 1993a; Birdwell and others 1997). Increases in these diseases were observed, but no dose estimates were available. Hancock and colleagues (1993b) also investigated mortality from heart disease following treatment for HD in a cohort of 2232 patients treated from 1960 to 1991 with an average follow-up of 9.5 years. The RR for mortality due to heart diseases was 3.5 (95% CI 2.7, 4.3) among those who received mediastinal radiation doses of more than 30 Gy. The increased risk was highest for exposures that occurred before the age of 20 and increased with time since exposure. No increased risk was observed among subjects who received doses lower than 30 Gy. In a separate study, Heidenreich and coworkers (2003) found a high prevalence of asymptomatic heart disease—specifically aortic valvular disease—following mediastinal irradiation.
Breast Cancer
Leukemia, lung cancer, soft tissue sarcoma, and contralateral breast cancer have been studied in patients receiving radiotherapy for breast cancer.
A case-control study of leukemia (excluding CLL) was carried out nested within a cohort of 82,700 women with breast cancer in the United States. A total of 90 cases and 264 controls were included with individual estimates of dose
to the active bone marrow. A significant dose-response was seen for acute nonlymphocytic leukemia after adjustment for the amount of chemotherapy, with an RR of 2.4 among those who received radiotherapy alone (Curtis and others 1992). No information was provided on the magnitude of the risk per gray or on the risk of other forms of leukemia.
A case-control study of contralateral breast cancer was carried out nested within a cohort of 41,109 women in Connecticut diagnosed with breast cancer between 1935 and 1982. A total of 655 cases and 1189 controls were included. The average dose to the contralateral breast was 2.8 Gy. A significant increased risk was seen only among women who received radiotherapy before age 45 (RR 1.59; 95% CI 1.07, 2.36, based on 78 exposed cases); a significant dose-response was observed in this group (Boice and others 1992).
No excess risk of contralateral breast cancer was seen in a cohort of 14,000 women treated between 1946 and 1982 in Denmark (Basco and others 1985). The study included 194 cases with individual dose estimates (mean doses ranging from 1.4 to 3.3 depending on the type of radiotherapy and the field considered). The RR per 100 cGy was 0.99 (95% CI 0.76, 1.30); little difference was seen for those diagnosed 5–10 years or more after their first tumor.
A case-control study of contralateral breast cancer was conducted among women with primary breast cancer entered in the Danish Cancer Registry from 1943 to 1978 (Storm and others 1992). A total of 529 cases and 529 controls were included, and individual doses to the contralateral breast were estimated from detailed radiotherapy records for all subjects who had received radiotherapy. The mean dose to the contralateral breast was estimated to be 2.5 Gy. There was no significantly increased risk of contralateral breast cancer in this study (RR = 1.04; 95% CI 0.74, 1.46).
A case-control study of lung cancer was conducted based on the Connecticut Tumor Registry (Inskip and others 1994). The study included 61 cases of lung cancer and 120 controls. Cases were diagnosed between 1945 and 1981 among women who had been treated for invasive breast cancer between 1935 and 1971 and survived at least 10 years. Individual radiation dose to different segments of the lung was estimated from detailed radiotherapy records. Average dose to the lung was 15.2 Gy to the ipsilateral lung and 4.6 Gy to the contralateral lung. Patients who received radiotherapy had a 1.8 times higher risk of developing lung cancer than those who did not (95% CI 0.8, 3.8). The risk increased with time since exposure and appeared to be higher among women exposed under age of 45, although this was not significant. The risk was highest for the ipsilateral lung. The ERR was estimated to be 0.2 Gy−1 to the affected lung (95% CI −0.62, 1.03), based on 15 exposed cases.
A nested case-control study of second malignant neoplasms was carried out for a cohort of 7771 women initially treated for breast cancer between 1954 and 1983 at the Institut Gustave Roussy near Paris, France (Rubino and others 2003). Individual doses to the location of the second tumor were estimated from detailed radiotherapy records. More than 40% of the irradiated patients received a local dose of less than 1 Gy. A significant quadratic dose-response was found in this study, with an excess risk of all second malignant neoplasms combined of 0.2% (95% CI 0.05, 0.5%) at 1 Gy.
Darby and coworkers (2003) studied cardiovascular mortality in a cohort of 89,407 Swedish women identified from the Swedish cancer registry as having had unilateral breast cancer at the ages of 18 to 79 years between 1970 and 1996. Mortality from cardiovascular disease was higher in women who had left-sided tumors (OR 1.10; 95% CI 1.03, 1.18) 10 years or more after the diagnosis of breast cancer; for ischemic heart disease, the OR was 1.13 (95% CI 1.03, 1.25). No dose estimates were available in this record linkage study, but the fact that the increase was restricted to women with tumors in the left breast and that no increase in mortality from other causes (except breast cancer) was seen in this population lends plausibility to the hypothesis of a radiation effect on the risk of heart disease.
Ovarian Cancer
A case-control study of leukemia within an international cohort of 99,113 survivors of ovarian cancer showed no significant excess risk for leukemia associated with radiotherapy alone (Kaldor and others 1990b). A more recent case-control study was carried out, nested within an international cohort of 28,971 patients in whom ovarian cancer was diagnosed between 1980 and 1993 (Travis and others 1999). The study included 96 leukemia cases and 272 controls. Individual dose to the active bone marrow was estimated for the 26 cases and 79 controls who had received radiotherapy. The median dose to the bone marrow was 18.4 Gy. Radiotherapy increased the risk of leukemia following platinum-based chemotherapy. No increased risk of leukemia was observed in subjects who had radiotherapy alone; the data are sparse: one exposed case and 36 exposed controls.
Testicular Cancer
Travis and colleages (1997) studied second cancer incidence in a multinational cohort of 28,843 men who had been diagnosed with testicular cancer between 1935 and 1993 in the United States, Denmark, Finland, the Netherlands, Sweden, and Canada (Ontario). Cases of second cancers occurring between 1965 and 1994 in this cohort were identified from population-based cancer registries in these countries. Significantly increased risks of second cancers in general, as well as of leukemia (64 cases) and stomach cancer (93 cases), were observed among patients who had received radiotherapy 5 years or more in the past. No individual doses were available.
Travis and colleagues (2000) conducted a case-control study of leukemia nested within a multinational cohort of 18,567 patients diagnosed with testicular cancer between
1970 and 1993 and registered in cancer registries in Iowa, Connecticut, New Jersey, Canada (Ontario), Denmark, Finland, the Netherlands, and Sweden. The study included 36 cases and 106 matched controls. Individual radiation dose to the active bone marrow was estimated from detailed radiotherapy records. In men who did not receive chemotherapy (mean radiation dose to 12.6 Gy), a 3.1-fold elevation of leukemia risk was observed (95% CI 0.7, 22). The risk increased with radiation dose to the active bone marrow, with an OR of 19.7 (95% CI 1.5, 59) for doses of 20 Gy or more (based on four exposed cases). No estimate of ERR or EAR per gray is given.
Thyroid Cancer
A cohort of 834 thyroid cancer patients treated with iodine-131 and of 1121 thyroid cancer patients treated by other means in Sweden between 1950 and 1975 was followed for cancer occurrence (Hall and others 1991). The average 131I cumulative activity administered was 4.55 GBq. The average duration of follow-up was 14 years. A total of 99 second cancers were found 2 years or more after 131I therapy among those treated with this modality and 122 among those treated by other means. The incidence of second malignancy was higher among those treated with 131I. Among women, the overall standardized incidence ratio (SIR)5 was 1.45 (95% CI 1.14, 1.83), and significantly elevated SIRs were found for tumors of the salivary glands, genital organs, kidney, and adrenal gland. A significant trend was seen with increasing 131I activity, with a SIR of 1.80 (95% CI 1.20, 2.58) for administered activities of 3.66 GBq and above.
A cohort of 1771 patients treated with 131I for thyroid cancer was followed up for incidence of second cancers (de Vathaire and others 1997). The average 131I cumulative activity administered was 7.2 GBq, resulting in an estimated average dose of 0.34 Sv to the bone marrow and 0.80 Sv to the whole body. After a mean follow-up of 10 years, no case of leukemia was seen. Eighty patients developed a secondary solid cancer, including 13 colorectal cancers. The risk of colorectal cancer was related to the total activity administered (ERR = 0.47 GBq−1; 95% CI 0.1, 1.6). The overall ERR for solid tumors in this study was 0.38 per estimated effective sievert (95% CI −0.22, 1.2); when tumors of the digestive track were excluded, the ERR was reduced to −0.15 Sv−1 (95% CI −0.35, 0.22).
Childhood Cancers
The treatment for childhood cancers, often a combination of both radiotherapy and chemotherapy, has prolonged the life expectancy of children with cancer and increased the chance of development of second cancers. Since childhood cancer is rare, national and international groups such as the Late Effects Study Group (Tucker and others 1987a, 1987b, 1991) and several groups in the United Kingdom (Hawkins and others 1987) and France (de Vathaire and others 1989, 1999) have combined their data to evaluate risks. Results from these cohort studies have indicated that the risk for developing a second cancer in the 25 years after the diagnosis of the first cancer was as high as 12% (Tucker and others 1991). Further, genetic predisposition appears to have a substantial impact on risk of subsequent cancers. Among patients treated for hereditary retinoblastoma, the risk of developing a second cancer in the 50 years after the initial diagnosis was as high as 51% (Wong and others 1997b).
Three nested case-control studies including 64 cases of bone cancer and 209 controls (Tucker and others 1987a), 23 cases of thyroid cancer and 89 controls (Tucker and others 1991), and 25 cases of leukemia and 90 controls (Tucker and others 1987b) were conducted from the Late Effects Study Group cohort of 9170 children who developed a second malignant tumor at least 2 years after diagnosis of the first tumor. A significant increased risk of bone cancer was found among patients who received radiation therapy (RR 2.7; 95% CI 1.0, 7.7), with a sharp dose-response gradient reaching a fortyfold risk following doses to the bone of more than 60 Gy. A significant increased risk of thyroid cancer was also found among patients who had received radiation therapy; most of the increase was among those who had received doses of 2 Gy or more. There was no evidence of a dose-response relationship for leukemia.
In a U.K. cohort of 10,106 3-year survivors of childhood cancer, Hawkins and colleages (1987; Hawkins 1990) reported an excess of second tumors among subjects who had received radiotherapy in comparison with the general population. In addition, two nested case-control studies of 59 cases of second bone cancer and 220 controls (Hawkins and others 1996) and 26 cases of second leukemia and 96 controls (Hawkins and others 1992) were conducted within this cohort, with individual dose reconstruction to the organs of interest. The risk of bone cancer increased substantially with increased cumulative radiation dose to the bone (p < .001), although a decline in risk was seen at doses equal to or greater than 50 Gy. A nonsignificant increased risk of leukemia was observed among those who had received radiotherapy (RR 8.4; 95% CI 0.9, 81.0 based on seven exposed cases). A significant dose-response relationship was observed.
In a cohort study of 634 children treated for childhood cancer from 1942 and 1969 in the Institut Gustave Roussy in Paris, a twofold increase in the risk of second malignancy was seen after doses from radiotherapy of more than 25 Gy, based on two exposed cases (de Vathaire and others 1989). A nonsignificant dose-response was seen based on 13 cases who had received radiotherapy alone.
In a French-British cohort study (de Vathaire and others 1999) that overlapped partially with the Late Effects Study, the French study, and British studies, described above, an excess of second cancers was seen among 1045 children who received radiotherapy alone (based on 31 second malignant neoplasms, including 8 brain cancers). Fourteen cases of thyroid carcinoma were identified in the entire cohort of 4096 3-year survivors of childhood cancers. All 14 had received radiotherapy. The average dose to the thyroid in this study was 7 Gy. A significant dose-response was observed for thyroid cancer in this study—RRs were 4.0 (90% CI 0.7, 44), 11.0 (90% CI 2.3, 123), 13.0 (90% CI 2.2, 141), and 26.0 (90% CI 3.4, 308) for doses within the ranges of 0.25 to <1 Gy (3 cases), 1.0 to <10 Gy (5 cases), 10 to <30 Gy (3 cases), and 30+ Gy (2 cases), respectively.
In a joint analysis of data from childhood cancer survivor cohorts from France, Britain, and Nordic countries, a nested case-control study of melanoma was carried out. Radiotherapy appeared to increase the risk of melanoma for local doses greater than 15 Gy (OR 13.0; 95% CI 0.94, 174.0), based on three exposed cases; the ORs for doses less than 1 Gy and of 1–15 Gy were 1.4 (95% CI 0.28, 7.0) and 3.2 (95% CI 0.37, 27) based on very small numbers of exposed cases—five and two, respectively (Guerin and others 2003).
A partially nested case-control study of soft tissue sarcoma (STS) was carried within the French-U.K. cohort of 4400 3-year survivors of childhood cancer survivors; 25 cases and 121 controls were included. Individual dose to the site of STS development was calculated. A significant increase in the risk of STS was seen among those who received radiotherapy (OR 19.0; 95% CI 3.0, 60.0). The risk increased with the square of the radiation dose and was independent of chemotherapy (Menu-Branthomme and others 2004).
Other Cancers
The health effects of radiotherapy for a number of other cancer types have also been considered in single studies. Travis and colleagues (1991) studied the risk of second cancers among 29,153 patients diagnosed with NHL between 1973 and 1987 in nine areas of the United States. Radiation therapy appeared to increase the risk of acute nonlymphocytic leukemia and possibly of cancers of the lung, bladder, and bone. No estimate of radiation dose was available.
Curtis and coworkers (1994) studied the risk of leukemia following cancer of the uterine corpus in a cohort of 110,000 women assembled from nine population-based cancer registries in the United States, Canada, Denmark, Finland, and Norway. Radiation doses were computed to 17 sections of the active bone marrow for 218 women who developed leukemia and 775 matched controls. There was no association between radiation dose and risk of CLL (RR 0.90; 95% CI 0.4, 1.9). For all leukemia excluding CLL, however, the RR was 1.92 (95% CI 1.3, 2.9). There appeared to be no association with age at exposure in this study. A complex dose-response was observed, with a relative risk of 1.8 (95% CI 1.1, 2.8) following continuous exposures from brachytherapy6 at comparatively low doses and low dose rates (mean total dose 1.7 Gy). The risk was of the same order (RR 2.3; 95% CI 1.4, 3.7) after fractionated exposures at much higher doses and dose rates from external beam treatment (mean total dose 9.9 Gy).
Summary
Studies of second cancer following radiotherapy have generally focused on patients treated for malignant diseases with a favorable long-term prognosis, such as cervical cancer, breast cancer, HD, and childhood cancers. Because many survivors of these cancers live long enough to develop a second, treatment-related malignancy, these studies have provided valuable information on the magnitude of risk following radiation exposure. The cohort studies generally do not provide quantitative information on the risk of radiation-induced cancer because of the absence of individual dose estimates.
Case-control studies of specific cancer types have been carried out, nested within cohorts of cancer survivors. In allowing the reconstruction of individual doses to specific organs for the subjects, they have provided important information for the estimation of site-specific cancer, even if the average doses to the target organs have generally been high. Studies of patients treated for HD have provided quantitative estimates of the risk of cancers of the lung and breast—organs that generally received fairly high doses (of the order of 20 Gy on average) from the radiotherapy. Studies of patients treated for cancer of the cervix have provided estimates of the risk of breast cancer, leukemia, and stomach cancer (at average doses of 0.2, 7, and 2 Gy, respectively). Studies of women treated for a first breast cancer have provided quantitative estimates of the risk of lung cancer, at average doses of the order of 5–15 Gy. These estimates are reviewed in detail, and compared with risk estimates derived from other medical exposure studies, in the section “Evaluation of Risk for Specific Cancer Sites.”
Radiotherapy for Benign Disease Among Adults
In the past, radiotherapy has been used in different countries for the treatment of a number of benign conditions in children (skin hemangioma, tinea capitis, enlarged thymus) and adults (e.g., benign breast and gynecological disease, ankylosing spondylitis, peptic ulcer). Studies of patients treated with radiation (X-rays and gamma rays) for benign disease provide valuable information about the carcinogenicity of low-LET (linear energy transfer) radiation. Doses
used in the treatment of benign conditions were generally not as high as those used to treat malignant disease so that cell-killing effects do not predominate, survival after treatment is good because the conditions treated were generally not life-threatening, and there is minimal confounding from concomitant treatment.
Benign Breast Disease
A U.S. cohort of 601 women treated with radiotherapy for acute postpartum mastitis and 1239 women treated by other means between 1940 and 1957 was followed for 29 years. The average dose to the breasts was 3.8 Gy. A significant increase in the risk of breast cancer was seen among women who had received radiotherapy, based on 51 exposed breasts with cancer. Using a linear multiplicative model the risk increased by 0.4% per rad (ERR per Gy 0.4; 90% CI 0.2, 0.7). A dose-response curve that appeared to be essentially linear up to about 7 Gy was demonstrated, and an increased risk for breast cancer was observed based on 56 cases (Shore and others 1986).
A Swedish cohort of 1216 women treated for benign breast disease with radiotherapy and 1874 women treated by other means from 1925 to 1954 was followed for an average of 27 years for development of a subsequent cancer. Mean absorbed doses to the breast were determined from detailed radiotherapy records and experiments with phantoms. The average dose to the breast was 5.8 Gy (range 0–50): 278 cases of breast cancer were diagnosed; 183 of these cases had received radiotherapy. A significant linear dose-response relationship was seen, with a downturn at approximately 10 Gy and higher. The estimated ERR for breast cancer was 1.63 Gy−1 (95% CI 0.77, 2.89, based on 47 exposed cases) among subjects with less than 3 Gy and 1.31 Gy−1 (95% CI 0.79, 2.04, based on 75 exposed cases) among subjects with less than 5 Gy (Mattsson and others 1995).
Mattsson and colleagues (1997) also studied the risk of malignancies other than breast cancer. Average doses were estimated to 14 organs. A significant increase in the risk of all cancers combined (excluding breast) was observed. A significant linear dose-response was seen for stomach cancer: ERR per Gy 1.3 (95% CI 0.0, 4.4), based on 14 exposed cases and a mean dose to the stomach of 0.66 Gy (range 0–5.4). No significantly increased risk was seen for any other cancer site, including leukemia, based on a small number of exposed cases (Mattsson and others 1997). The estimated ERR for lung cancer was 0.38 (95% CI <0, 0.6), based on 10 exposed cases and a mean lung dose of 0.75 (range 0–9.0).
Peptic Ulcer
Cancer mortality up to 1985 was studied in a U.S. cohort consisting of 1831 patients irradiated between 1937 and 1965 for the treatment of peptic ulcer and 1778 who were not (Griem and others 1994). An elevated risk of circulatory disease mortality was observed among those who received radiotherapy compared to those who did not. Overall, a 50% increase in the risk of all cancers combined was observed. Significant increases were seen for cancers of the stomach, pancreas, and lung; the average doses to the organs were estimated to be 15, 13, and 1.7 Gy, respectively. For stomach cancer, a threefold increase in risk was observed in this study; the RR at 1 Gy was estimated to be 1.15, and the absolute risk was 4.19 per 104 PY per gray. The estimated RR of lung cancer was 1.66 at 1 Gy.
In an updated follow-up of this cohort up until 1997 (average follow-up 25 years), Carr and colleagues (2002) also reported significant exposure-related increases in the risk of cancers of the stomach, pancreas, and lung among 1859 patients treated with radiotherapy. For stomach cancer, the ERR was estimated to be 0.20 Gy−1 (95% CI 0, 0.73), based on analyses restricted to subjects who had received doses to the stomach of 10 Gy or less (mean dose to the stomach 8.9 Gy; number of exposed cases 11). The corresponding estimate for cancer of the pancreas was 0.34 Gy−1 (95% CI 0.09, 0.89), with a mean dose of 8.2 Gy and 14 exposed cancer cases. For lung cancer, the ERR was estimated to be 0.43 Gy−1 (95% CI 0.12, 1.35) among subjects in the lowest-dose quartile (<1.4 Gy—mean dose 1.1 Gy), based on 21 deaths from lung cancer. Although the risk of pancreatic cancer decreased with increasing age at exposure, no association with age at exposure was observed for stomach and lung cancer.
Benign Gynecological Diseases
A U.S. cohort of 4153 women treated with intrauterine 226Ra between 1925 and 1965 for uterine bleeding disorders was followed for an average of 27 years up to 1983 (Inskip and others 1990b). Individual organ doses were estimated based on detailed radiotherapy records and simulation of pelvic irradiation treatments on phantoms. A significantly increased standardized mortality ratio (SMR)7 for death from all cancers was seen in this population compared to the general population. In addition, significant increases were observed for deaths from colon and uterine cancer, cancers of the female genital organs, and leukemia. Estimated ERR per Gy were 0.006 (90% CI −0.01, 0.05) for cancer of the uterus, 0.41 (90% CI −0.69, 1.51) for other genital organs, 0.51 (90% CI −0.08, 5.61) for colon cancer, and 0.20 (90% CI 0.08, 0.35) for bladder cancer.
Inskip and colleagues (1990a) studied the risk of leukemia in relation to radiation dose among 4483 of these women. Individual doses to various sections of the red bone marrow were calculated from detailed radiotherapy records. The median dose to red bone marrow was 0.53 Gy. A significant excess of leukemia was observed; the risk was highest 2–5 years after treatment (SMR 8.1; 95% CI 2.6, 18.8,
compared to the general population) and among women over 55 years at irradiation (SMR 5.8; 95% CI 2.5, 11.3). The average ERR in this study was 0.19 Gy−1 (95% CI 0.08, 0.32) for intrauterine 226Ra exposure, and the average absolute excess mortality from leukemia was 2.6 per 104 PY per gray.
The risk of leukemia, lymphoma, and multiple myeloma was studied in an expanded cohort of 12,955 women treated for benign gynecological disorders at one of 17 hospitals in Massachusetts, Connecticut, Rhode Island, or New York State between 1925 and 1965 (Inskip and others 1993). Of these women, 9770 were treated with radiation (either intracavitary 226Ra or external beam X-rays), while the rest were treated by other methods. The average age at treatment was 46.5 years, and the average dose to active bone marrow in exposed women was 1.2 Gy. The RR for all cancers of hematopoietic and lymphatic tissue was 1.3 (95% CI 1.2, 1.5) for irradiated women, compared to nonirradiated. The risk of lymphomas, multiple myeloma, and nonacute lymphocytic leukemia was similar between irradiated and nonirradiated women. The RRs for acute lymphocytic leukemia and for myeloid leukemia were elevated, however: RR 3.7 (95% CI 1.3, 16) and 3.7 (95% CI 0.9, 36), respectively. For acute lymphocytic and nonlymphocytic leukemia, the SMRs were similar for women treated with radium only and with both modalities, and were smallest for X-ray patients (difference not statistically significant). The ERR per Gy was 0.29 overall; 0.37 Gy−1 (95% CI <0, 1.5) for treatment with radium only; 0.05 per Gy (95% CI −0.06, 0.33) for X-rays only; and ERR 0.21 per Gy (95% CI 0.05, 0.83) for the combination of both modalities. Average doses for the different treatment types were 0.6, 2.3, and 2.0 Gy, respectively, indicating a complex dose-response relationship.
A cohort of 2067 women who received radiotherapy for metropathia hemorrhagica (uterine bleeding disorders) in Scotland between 1940 and 1960 was followed until the end of 1990 (Darby and others 1994). The average follow-up was 28 years. Absorbed doses to the active bone marrow and to 20 solid organs or anatomical sites were estimated from treatment records. Overall, 331 deaths from cancer were observed, and significantly elevated SMRs were observed for cancers at heavily irradiated sites (average local dose >1 Gy): cancer of pelvic sites, particularly urinary bladder cancer (mean dose 5.2 Gy); colon cancer (mean dose 3.2 Gy); leukemia, and multiple myeloma (mean total active bone marrow dose 1.3 Gy). A deficit of breast cancer mortality was also observed in this cohort, due mainly to a large deficit in women who had received doses to the ovary of 5 Gy or more. No estimate of risk per unit dose was presented.
A Swedish cohort study included 2007 women treated for metropathia hemorrhagica between 1912 and 1977. Of these, 788 received radiotherapy for this condition. The population was followed up for cancer mortality and incidence from 1958 to 1982, with a mean follow-up period of 28 years (Ryberg and others 1990). A total of 107 cancers were observed among irradiated women. The SIR for cancer was 1.22 among irradiated women and 1.09 among nonirradiated. A significant increase in the SIR for cancers at heavily irradiated sites in the pelvic area was only observed 30 years or more after irradiation. A decreased risk for breast cancer was also observed in this cohort, except for women treated at the age of 50 or more. No estimate of risk per unit dose was presented.
Hormonal Infertility
A U.S. cohort of 816 women who received X-ray therapy to the ovaries and/or pituitary gland for refractory hormonal infertility and amenorrhea between 1925 and 1961 was followed up until the end of 1990 (Ron and others 1994). The average duration of follow-up was 35 years. Individual organ doses were estimated from radiotherapy records. Average doses were 0.01 Gy to the breast, 0.9 Gy to the ovary, and 1.0 Gy to the sigmoid colon. Seventy-eight deaths from cancer occurred in this cohort. No increase in mortality rates was found for leukemia or sites directly exposed to radiation, such as the ovary or brain, based on a very small number of deaths (two leukemia, three ovary, and one brain cancer death). The SMRs were significantly elevated, however, for cancer of the colon (15 deaths) and for NHL (6 deaths). No estimate of risk per unit dose was presented.
Ankylosing Spondylitis
A U.K. cohort consisting of 15,577 patients diagnosed with ankylosing spondylitis between 1935 and 1957 was followed for mortality up to the end of 1991 (Weiss and others 1994). The average duration of follow-up was 25 years. Of these subjects, 14,566 had received X-ray treatment for their disease. Radiation doses to various organs were calculated for a sample of patients, and average estimated doses from all treatment courses occurring within 5 years of the initial treatment courses were attributed to all patients. The mean total body dose was estimated to be 2.6 Gy. Irradiated patients had a significantly greater mortality rate from cancer than expected from the national rates for England and Wales, and significant increases were seen for leukemia, NHL, multiple myeloma, and cancers of the esophagus, colon, pancreas, lung, bones, connective and soft tissue, prostate, bladder, and kidney. A linear dose-response model for all cancers except leukemia gave an ERR of 0.18 Gy−1 (95% CI 0.10, 0.27) 5–24 years after treatment (based on 741 deaths), decreasing significantly to 0.11 Gy−1 25 years or more (based on 845 deaths) after treatment. For lung cancer, the ERR was 0.09 Gy−1 (95% CI 0.03, 0.15) 5–24 years after treatment, based on 282 deaths and an average dose to the bronchi of 8.88 Gy. For stomach cancer, the ERR was −0.004 Gy−1 (95% CI −0.05, 0.05) 5–24 years after treatment, based on 127 deaths and an average stomach dose of 3.21 Gy. There was no increased risk in breast cancer in
this population, based on 84 deaths (ERR 0.08 Gy−1; 95% CI − 0.30, 0.65); this may result from the fact that the average dose the ovaries was high—5.5 Gy).
The risk of leukemia mortality in this cohort was studied further by Weiss and colleagues (1995), using a case-subcohort approach. A total of 60 leukemia deaths were observed during the follow-up period. Radiotherapy records were obtained for all but six of the deaths from leukemia excluding CLL, and individual doses to the red bone marrow were estimated as in the previous study; estimated doses were also available for the subcohort, as described in Weiss and colleagues (1994). The average dose to the total red bone marrow was estimated to be 4.44 Gy, but doses were nonuniform, with the heaviest dose to the lower spine. A linear-exponential model (in which the exponential term allows for cell sterilization in heavily exposed parts of the bone marrow), varying with time since exposure, provided a good description of the risk for non-CLL. The estimated ERR per Gy was 12.4 (95% CI 2.3, 52.1) 10 years after exposure; 1–25 years after exposure, the average ERR per Gy was estimated to be 7.0, based on 35 cases.
A Swedish cohort of 20,024 patients who received X-ray therapy between 1950 and 1964 for painful benign conditions of the locomotor system (including arthrosis and spondylosis) was followed for cancer incidence and mortality until the end of 1988 (Damber and others 1995). The average length of follow-up was 25 years. Average conversion factors between surface dose and mean absorbed dose in the red bone marrow were estimated by treatment site (for six sites), based on the treatment records of random samples of 30 subjects drawn from the cohort (Damber and others 1995). The conversion factors were applied to the entire cohort and used for stratification of subjects in different levels of exposure. The average absorbed dose to the red bone marrow was estimated to be 0.39 Gy. A total of 116 leukemia cases (115 deaths) were observed during the study period. The SIR and SMR for subjects with mean absorbed doses of 0.5 Gy or more were 1.40 (95% CI 1.00, 1.92) and 1.50 (95% CI 1.05, 2.04), respectively. No estimate of risk per unit dose was presented.
Thyroid Diseases
Iodine-131 is currently the treatment of choice for hyperthyroidism, largely because no serious side effects are known. Concerns remain, however, about the subsequent risk of cancer. Several studies of patients treated with 131I for hyperthyroidism have been carried out in the United States, Sweden, and the United Kingdom.
The occurrence of leukemia and of thyroid neoplasms (both benign and malignant) was studied among 36,050 patients treated for hyperthyroidism between 1946 and 1968 and included in the Cooperative Thyrotoxicosis Therapy Follow-up Study (Saenger and others 1968; Dobyns and others 1974). Approximately 20,000 subjects had been treated with 131I. The follow-up was active, with an average duration of 8 years. No excess of leukemia or thyroid cancer was observed among patients treated with 131I.
In a follow-up to this study, Hoffman (1984) studied cancer risk up to 1979 in the subgroup of 3696 women who had been treated at the Mayo Clinic, one of the original participating centers. Among these, 1005 had received 131I therapy alone and 2141 had been treated with surgery alone. A total of 527 cancer cases were identified in these two study groups; 175 were excluded because they occurred within a year of treatment. The mean observation period was 15 years for patients treated with 131I. The average whole-body dose is estimated to be of the order of 0.06–0.4 Gy in this cohort. There was no increased cancer risk among those treated with 131I and no indication of a relation with 131I activity delivered. Nonsignificant increased risks were seen for cancers in the two most exposed organs (thyroid and salivary glands, based on three and two cases, respectively).
Goldman and colleagues (1988) reported on an extended follow-up of 1762 women, included in the Cooperative Thyrotoxicosis Therapy Follow-up Study, who were treated at the Massachusetts General Hospital between 1946 and 1964. A total of 1406 had been treated with 131I. No dose estimation was conducted. The average follow-up duration was 17.2 years. An elevated SMR was noted in this cohort (SMR 1.3; 95% CI 1.2, 1.4) for all causes of death but not for all cancers (SMR 0.9; 95% CI 0.7, 1.1). A nonsignificantly increased SMR was noted for breast cancer (SMR 1.2; 95% CI 0.9, 1.5); no association with 131I activity was found.
Ron and colleagues (1998a) reported on mortality to the end of 1990 in the Cooperative Thyrotoxicosis Therapy Follow-up Study. The cohort included 35,593 hyperthyroid patients, 91% of whom had been diagnosed with Grave’s disease. Fewer than 500 subjects were less than 15 years of age at the time of treatment. The mean length of follow-up was 21 years, and 51% of the subjects had died during the study period. Doses from 131I to 17 organs (other than the thyroid) were estimated for each study subject by multiplying the amount of administered activity by the age-specific dose factor and 24-h thyroid uptake provided for each organ by the International Commission on Radiological Protection (ICRP 1988). Treatment with 131I was not related to all cancer mortality (SMR 1.02; 95% CI 0.98, 1.07) or to mortality from any specific cancer, with the exception of thyroid cancer (SMR 3.94; 95% CI 2.52, 5.86, based on 27 cases). A nonsignificant increase in mortality from thyroid cancer was seen with increasing 131I administered activity—when deaths occurring in the first 5 years after treatment were excluded, there was no evidence of a relationship with total activity; it is therefore likely that the underlying thyroid disease played a role in the observed cancer increase.
Cancer incidence was also studied in 4557 patients who received 131I therapy for hyperthyroidism in Sweden between 1950 and 1975 at Radiumhemmet, Sweden (Holm 1984). Information on thyroid disease and treatment was abstracted
from medical records. Cancer diagnoses in these patients were identified from the Swedish Cancer Registry for 1958 to 1976. The average length of follow-up was 9.5 years and 398 cases were identified. There was no increased risk of cancer as a whole or of leukemia in this population. Only for central nervous system (CNS) tumors among women was an increased risk seen (RR 1.89, based on 17 cases); the RR was higher among those who received total 131I activities of 370 MBq or more (RR 2.30, based on 9 cases).
The risk of cancer was studied in 10,552 patients (including the 4557 in the previous study) treated for hyperthyroidism with 131I in seven hospitals in Sweden between 1950 and 1975 (Holm and others 1991). The mean age at treatment was 57 years. Information on thyroid disease and treatment was abstracted from medical records. The mean total activity administered was 506 MBq (360 MBq to patients with Grave’s disease and 700 MBq to those with toxic nodular goiter). The dose to various organs was estimated using conversion factors from ICRP (1988) tables and other sources. Dose to the thyroid was in the range of 60–100 Gy. Doses to other organs were lower: 0.25 Gy to the stomach, 0.07 Gy to the lung, and 0.06 Gy to the red bone marrow. A total of 1543 cancer cases were identified during 1958–1985. The mean follow-up time of subjects who survived more than a year after treatment was 15 years, with a maximum of 28 years. The SIR for all cancers in this population was 1.06 (95% CI 1.01, 1.11) compared to the Swedish population. Significant increases were seen for cancers of the lung and kidney and, among 10-year survivors, for cancers of the stomach, kidney, and brain. Only the risk for stomach cancer increased with the level of administered 131I dose and this increase was not statistically significant; the estimated RR at 1 Gy for stomach cancer was 2.32 and the absolute risk was 9.6 per 104 PY per gray.
A population-based study of cancer incidence in a cohort of 7417 patients treated with 131I in the West Midlands region of the United Kingdom between 1950 and 1991 was carried out (Franklyn and others 1999). The subjects were followed up for cancer incidence and mortality from 1971 to 1991. No estimation of dose from 131I is presented. Significant decreases in all cancer incidence (634 cases: SIR 0.83; 95% CI 0.77, 0.90) and mortality (448 cases: SMR 0.90; 95% CI 0.82, 0.98) were observed in this cohort. Significant increases in incidence were seen for cancers of the small bowel (six cases: SIR 4.8; 95% CI 2.2, 10.7) and thyroid (nine cases: SIR 3.3; 95% CI 1.7, 6.3).
Summary
Studies of patients treated with radiation (X-rays and gamma rays) for benign disease provide valuable information about the carcinogenicity of low-LET radiation. Doses used in the treatment of benign conditions were generally not as high as those used to treat malignant disease, so that cell-killing effects do not predominate, survival after treatment is good since the conditions treated were generally not life-threatening, and there is minimal confounding from concomitant treatment.
Studies of patients treated for ankylosing spondylitis, benign breast disease, benign gynecological disease, and peptic ulcer have provided valuable information for the quantification of radiation risk estimates for cancers of the lung, breast, and stomach and for leukemia. These estimates are reviewed in detail, and compared with risk estimates derived from other medical exposure studies, in section “Evaluation of Risk for Specific Cancer Sites.”
Studies of patients treated with 131I for thyroid diseases provide little quantitative information about radiation risks.
Radiotherapy for Benign Disease Among Children
Tinea Capitis
Between 1948 and 1960 nearly 20,000 children, primarily immigrants to Israel or children of immigrants from North Africa and the Middle East, were treated with radiation for tinea capitis (ringworm of the scalp) in Israel (Ron and others 1988b). This treatment modality was used in other countries as well, and a study also was carried out in New York (Shore and others 1984).
In Israel, mortality in a cohort of 10,834 irradiated children, 10,834 matched comparison subjects, and 5392 sibling controls was studied by Ron and colleagues (1989). Crude dose estimates were derived from treatment information (dosage, area), age of the child, and the use of filtration. Between 1950 and 1982, 609 subjects died. Radiotherapy in childhood was associated with an increased risk of mortality from tumors of the head and neck (particularly brain and thyroid tumors) and leukemia. For the latter (leukemia), the EAR was estimated to be 0.9 per 104 PY per gray (the mean average dose to the bone marrow was 0.3 Gy in this cohort).
The relation between radiation dose and risk of tumors of the brain and CNS in this cohort was examined further by Ron and colleagues (1988a). The dose reconstruction method used was improved compared to the above paper, relying heavily on dosimetric studies and measurements in a simulated phantom model of a 6-year-old child. The average dose to the brain in irradiated patients was 1.5 Gy (range 1–6 Gy), and the average minimal and maximal doses to specific areas of the brain were 0.8 and 1.8 Gy, respectively. Sixty neural tumors developed in irradiated subjects. The RR was 6.9 (95% CI 4.1, 11.6) overall, compared to the nonirradiated control groups; for neural tumors of the head and neck it was 8.4 (95% CI 4.8, 14.8). Increased risks were apparent for meningioma (RR 9.5, 19 deaths), gliomas (RR 2.6, 7 deaths), nerve sheath tumors (RR 18.8, 25 deaths), and other neural tumors. A strong dose-response relation was found, with the RR approaching 20 after doses of the order of 2.5 Gy. No estimate of risk per dose is presented in this study. Since then, a descriptive study of 253 meningioma cases diagnosed
in the above cohort has been published (Sadetzki and others 2002), but there were no risk estimates. A more recent study (Sadetski and others 2005) conducted a survival analysis using Poisson regression to estimate the excess relative and absolute risks for brain tumors. After a median follow-up of 40 years, ERRs/Gy of 4.63 and 1.98 (95% CI 2.43, 9.12 and 0.73, 4.69) and EARs/Gy per 104 PY of 0.48 and 0.31 (95% CI 0.28, 0.73 and 0.12, 0.53) were observed for benign meningiomas and malignant brain tumors, respectively. The risk of both types of tumors was positively associated with dose. The estimated ERR/Gy for malignant brain tumors decreased with increasing age at irradiation from 3.6 for exposures below the age of 5 to 0.5 for exposures at ages 10 or above (p = .04), while no trend with age was seen for benign meningiomas. The ERR for both types of tumor remains elevated 30-plus years after exposure.
Modan and colleagues (1989) reported on an additional 5-year follow-up (until 1986) of the Israeli tinea capitis cohort. While the previously observed increases in the incidence of head and neck tumors (mainly brain, CNS, and thyroid tumors) continued, an elevated risk of breast cancer was observed for the first time in this cohort, based on 13 new cases in 1982–1986. The estimated average dose to the breast was low—0.016 Gy. The increase was seen only among women who were 5–9 years of age at the time of radiation exposure (10 cases). No estimate of risk per dose is presented.
Ron and colleagues (1989) reported on the risk of thyroid cancer following irradiation in childhood for tinea capitis, based on an extended follow-up (until 1986). The dose reconstruction method is based on the approach described above for the brain and CNS study. To adjust the dose for possible head movement during treatment, individual dose estimates were multiplied by a factor of 1.5 as suggested by results of dosimetric studies. Average doses to the thyroid were 0.13, 0.09, and 0.06 Gy, respectively, for children aged less than 5, 5–10, and 10–15 years at the time of exposure. Overall, 98 thyroid tumors were identified among the exposed and 57 among the two control populations. An estimated dose of 0.09 Gy was related to a fourfold increase (95% CI 2.3, 7.9) in the risk of thyroid cancer and a twofold increase in benign tumors. The dose-response was consistent with linearity. The risk diminished with age at exposure, and the RR appeared to be constant over time. The ERR was estimated to be 30 Gy−1 and the EAR was 13 per 104 PY per gray.
Modan and coworkers (1998) also reported a 4.5-fold increase in the incidence of malignant salivary gland tumors (p < .01) and a 2.6-fold increase of benign tumors in subjects irradiated for tinea capitis. A clear dose-response association with both cancer and benign tumors was demonstrated. No estimate of risk per dose level was presented.
In New York, about 2200 children who received X-ray treatment for tinea capitis during the 1940s and 1950s and a comparable group of 1400 treated without X-rays were followed by mail questionnaire to evaluate the incidence of skin cancer (Shore and others 1984). The average length of follow-up was 26 years. Delivered doses ranged from 3 to 6 Gy depending on the portion of the scalp, with lower doses to the skin of the face and neck (0.1–0.5 Gy). In the irradiated group, 41 subjects had a diagnosis of basal carcinoma of the scalp or face, compared to 3 in the control group. The prevalence of multiple lesions was high in the exposed group. The minimum latent period was long (about 20 years); skin cancers were more pronounced on the face, where the potential for exposure to ultraviolet is higher, and were restricted to Caucasians although one-quarter of the study population was African American. No estimate of risk per dose is presented.
Enlarged Thymus Gland
Patients in Rochester, New York who received X-ray treatment between 1926 and 1957 in infancy (before 6 months of age) for an enlarged thymus gland and their nonirradiated siblings have been followed up periodically through the use of a mail questionnaire (Shore and others 1985, 1993a, 1993b; Hildreth and others 1985). Information on X-ray treatment factors was extracted from medical records and supplemented by interviews with the treating physicians. These, along with anatomic measurements for infants, allowed estimation of doses to various nearby organs. The thyroid doses were estimated by irradiating a radiological phantom of an infant. The irradiated group had a statistically significant increase of both benign and malignant thyroid tumors (Shore and others 1985) and extrathyroid tumors (Hildreth and others 1985), particularly benign tumors of the bone, nervous system, salivary glands, skin, and breast (women only) and malignant tumors of the skin and breast.
In the most recent paper on thyroid cancer, which reports on follow-up to 1986, the cohort included 2657 exposed subjects and 4833 unexposed siblings with at least 5 years of follow-up (Shore and others 1993a). The average duration of follow-up was 37 years. Thyroid doses could be estimated for 91% of the subjects. The thyroid dose distribution was skewed, ranging from 0.03 to more than 10 Gy, with a mean of 1.4 Gy and a median of 0.3 Gy. There were 37 pathologically confirmed thyroid cancers among the irradiated group and 5 among the sibling controls. A linear dose-response was found in this cohort with an ERR of 9.0 at 1 Gy (90% CI 4.0, 24.0). An increased risk was seen even at low doses, with a significant positive slope in the dose range 0–0.3 Gy, based on four exposed cases. The risk ratio decreased over time, but was still highly elevated 45 years after exposure. There was no evidence of a decrease in the absolute excess risk over time (EAR varying from 2.1 per 104 PY per gray 5–14 years postirradiation to 6.0 per 104 PY per gray after 45 years or more). Analyses of interactions suggested that all Jewish subjects and women with older ages at menarche
or at first childbirth were at greater risk of radiation-induced thyroid cancer.
The risk of benign thyroid adenomas was also studied in more detail (Shore and others 1993b). There were 86 pathologically confirmed thyroid adenomas among the irradiated group and 11 in the sibling controls. The estimated ERR was 6.3 Gy−1 (90% CI 3.7, 11.2) overall and 7.8 Gy−1 when restricted to subjects with doses less than 6 Gy. Adenoma rates were elevated even at lower doses, with a significant increase in the lowest-dose group (<0.25 Gy). The risk continued to be elevated to the end of follow-up.
Analyses of the risk of breast cancer in relation to radiation dose were also carried out in this population. Hildreth and colleagues (1989) reported on the follow-up to 1985 of 1200 women who received X-ray treatment and their 2469 nonirradiated sisters. Twenty-two breast cancer cases were diagnosed in the irradiated group and twelve in the control group. The estimated average dose to the breast was 0.69 Gy. A linear dose-response was observed, with an ERR of 2.48 Gy−1 (95% CI 1.1, 5.2) and an EAR of 5.7 per 104 PY per gray (95% CI 2.9, 9.5).
Skin Hemangioma
Two Swedish cohort studies have been performed of patients treated for skin hemangioma in infancy. In the first study (Lundell and others 1994), the cohort consisted of 14,351 infants (less than 18 months of age) treated between 1920 and 1959 at Radiumhemmet, Stockholm, who were followed up for cancer incidence over the period 1958–1986. Radiotherapy was given with -particles, X- and/or -rays, and usually, with some type of 226Ra applicator. Individual organ doses were calculated using treatment information and, for 226Ra needles and tubes, phantom simulations. Seventeen thyroid cancers were registered in this cohort during the follow-up period. The mean dose to the thyroid was 1.07 Gy (range <0.01, 4.34 Gy). A significant excess thyroid cancer incidence was seen in this cohort, starting 19 years after treatment and persisting at least 40 years after irradiation. A significant dose-relationship was observed, with an ERR of 4.92 Gy−1 (95% CI 1.26, 10.2) and an EAR of 0.90 per 104 PY per gray.
Lundell and Holm (1995) also studied the risk of other solid tumors in this cohort. Statistically significantly increased SIRs were seen for cancer of the pancreas and tumors of the endocrine glands, based on small numbers of cases (9 and 16, respectively). For lung cancer (mean dose 0.12 Gy), a nonsignificant ERR of 1.4 Gy−1 was reported (confidence interval not given) and an EAR of 0.33 per 104 PY per gray, based on 11 cases. For stomach cancer (mean dose 0.09 Gy), both the ERR and the EAR were negative (values not reported), based on five cases.
Lundell and colleagues (1996) reported more specifically on the risk of breast cancer among women from this cohort. The mean absorbed dose to the breast was 0.39 Gy (range <0.01, 35.8 Gy). During the follow-up period, 75 breast cancer cases were found in the cohort. A significant linear dose-response relationship was observed, with an ERR of 0.38 Gy−1 (95% CI 0.09, 0.85) and an EAR of 0.41 per 104 PY per gray. This was not modified by age at exposure or by dose to the ovaries. The ERR increased significantly with time since exposure, however, with an ERR at 1 Gy of 2.25 (95% CI 0.59, 5.62) 50 years or more after exposure. The EAR was 22.9 per 104 PY per gray.
In an analysis of leukemia mortality in the same cohort, 20 deaths from leukemia were observed (11 in childhood and 9 among adults). The weighted bone marrow dose was 0.13 Gy on average (range <0.01–4.6 Gy). There was no association between radiation dose and leukemia (childhood or adult) in this cohort. Among those who received more than 0.1 Gy, the ERR was estimated to be 5.1 Gy−1 (95% CI 0.1, 15) for childhood leukemia, −0.02 Gy−1 (95% CI −0.8, 1.9) for adult leukemia, and 1.6 Gy−1 (95% CI −0.6, 5.5) overall.
The second Swedish hemangioma study included 11,807 patients treated with 226Ra between 1930 and 1965 at Sahlgrenska University Hospital in Göteborg (Lindberg and others 1995). The cohort was followed up for cancer incidence over the period 1958–1989. Doses to 11 organs were calculated on the basis of 226Ra activity, location of the hemangioma, and estimated absorbed dose rate in these organs per unit activity in a phantom the size of a 5–6-month-old child. No correction was made for different body sizes according to the age of the child at the time of treatment. A total of 248 malignancies were observed during the study period. A significantly increased risk of cancer was seen overall, as well as tumors of the CNS (34 cases), thyroid (15 cases), and other endocrine glands (23 cases). The mean absorbed dose to the thyroid in this cohort was 0.12 Gy; the ERR for thyroid cancer was estimated to be 7.5 Gy−1 (95% CI 0.4, 18.1) and the EAR 1.6 per 104 PY per gray.
Karlsson and others (1997) studied the risk of intracranial tumors in this cohort further in a cohort and a case-control study. Dose estimation was similar to that described above for subjects in the cohort study, although a correction was made for different age groups (0–4, 5–11, 12–18, and 18+ months). Activity was considered to be uniformly distributed over the treatment area. In the case-control study, the dose at the exact tumor site was calculated by considering the exact distance between the treatment location, according to the record, and the site of the tumor. For the controls, the dose was calculated at the location of the tumor in the corresponding case. In the cohort, 47 intracranial tumors developed in 46 individuals. An excess was found for many histopathological subgroups but was significant only for gliomas and meningiomas. The mean dose to the brain was 0.072 Gy (median 0.023 Gy; range <0.001–2.4 Gy). There was an excess of brain tumors in all dose categories, but no clear dose-response relationship. When analyses were restricted to subjects treated before the age of 7 months, both a linear and a
linear-quadratic model described the data. The estimated ERR for the entire cohort was 1.05 Gy−1; the EAR was 1.20 per 104 PY per gray. In the case-control study, the mean absorbed dose at the site of the tumor was 0.031 Gy for cases and 0.09 for controls. The estimated OR was 1.65 Gy−1 (95% CI 0.63, 4.32).
Pooled analyses of the data on breast cancer and intracranial tumors from the two Swedish hemangioma cohorts were also carried out. In the pooled breast cancer analyses (Lundell and others 1999), 245 breast cancer cases diagnosed between 1958 and 1993 were available. The ERR was estimated to be 0.35 Gy−1 (95% CI 0.18, 0.59) and the EAR 0.72 per 104 PY per gray (95% CI 0.39, 1.14). There was no evidence of an effect of time since exposure on the ERR; the EAR, however, increased with time since exposure. Neither age at exposure, dose rate, nor ovarian dose appeared to have an effect on the ERR.
In the pooled analysis of intracranial tumors (Karlsson and others 1998), 88 tumors were found in 86 individuals between 1958 and 1993. There was a significant dose-response relationship, and increasing age at exposure decreased the magnitude of the risk. The ERR was 2.7 Gy−1 (95% CI 1.0, 5.6) overall; 4.5 Gy−1 for exposure before the age of 5 months; 1.5 Gy−1 for exposures between 5 and 7 months; and 0.4 Gy−1 for exposures at older ages. The overall EAR was 2.12 per 104 PY per gray (95% CI 0.27, 4.38). There was no effect of time since exposure on the ERR, while the EAR increased with time since exposure.
Cancer mortality was studied in a cohort of 7037 patients less than 15 years of age treated for a skin hemangioma between 1940 and 1973 at the Institut Gustave Roussy, near Paris, France (Dondon and others 2004). Among them, 4940 had received radiotherapy. The cohort was followed up from 1969 to 1997, during which time 16 patients died of cancer; 14 of these had received radiotherapy. A nonsignificant excess of cancer-related mortality was observed for irradiated patients compared to the general population (SMR 1.53; 95% CI 0.86, 2.48). The excess was highest among those treated with 226Ra (RR 2.53; 95% CI 0.84, 7.07), in comparison to those who did not receive radiotherapy. No estimate of risk per dose is presented.
Enlarged Tonsils and Other Benign Conditions
In 1974, a prospective follow-up program was set up at the Michael Reese Hospital in Chicago to screen patients who had received X-ray treatment between 1939 and 1962 during childhood for benign head and neck conditions (primarily enlarged tonsils). During the screening, more than 35% of the subjects were found to have thyroid nodules (Schneider and others 1985). Analyses of dose-response relationships for thyroid cancer and thyroid nodules in this cohort were conducted by Schneider and colleagues (1993) with a mean follow-up of 33 years. Individual doses were estimated for study subjects on the basis of treatment records and experiments with an anthropomorphic phantom of a 6-year-old child, together with conversion factors for children of different ages. The average dose to the thyroid was estimated to be 0.6 Gy. Overall uncertainty in thyroid dose estimates for an individual subject is of the order of 50% and is related to the child’s movements during treatment and deviations in height and weight. The cohort included 4296 patients, of whom 3843 had estimated dose to the thyroid and 2634 could be followed up. A total of 1043 nodules and 309 thyroid cancers were diagnosed in the cohort. The ERR/Gy was 3.0 overall; it decreased with increasing age at exposure (from 3.6 for exposures below the age of 1 year to 1.4 for exposures between ages 5 and 15). There was no apparent difference between men and women. The slope of the dose-response relationship appeared to reach a maximum 25–29 years after exposure, but response continued to be elevated 40 years after exposure. The ERR appeared to be greater for cases diagnosed before 1974, when the screening program started (ERRs 9.2 and 1.8, respectively, for the period before 1974 and for 1974 and later, based on 109 and 200 cases), but this difference was not significant (p = .4).
From 1975 through 1982, a follow-up study was performed in Sweden for patients treated with X-rays for cervical tuberculous adenitis (Fjalling and others 1986). Of these patients, 444 underwent thyroid examination on average 43 years after their initial treatment. 101 had undergone surgery for thyroid nodules, including 25 for thyroid cancer. The absorbed dose to the thyroid was estimated to range from 0.4 to 51.0 Gy. A dose-response relationship was seen both for thyroid cancer and for nodules. No estimate of risk per dose is presented.
Thyroid Diseases
No study has focused specifically on populations exposed to 131I in childhood or adolescence for the treatment of hyperthyroidism. As indicated in the earlier section on treatment of adult benign thyroid diseases, the number of subjects under age 20 at diagnosis in the hyperthyroidism cohorts is very small. In a review paper, Shore (1992) carried out an analysis of risk in those exposed below age 20 in the Swedish and U.S. studies. The total population was estimated to be 602, with an approximate average follow-up of 10 years and a mean dose to the thyroid of about 88 Gy. Two cases of thyroid cancer were reported compared to about 0.1 expected. The estimated ERR was 0.3 Gy−1 (90% CI 0.0, 0.9) and the EAR 0.1 per 104 PY per gray (90% CI 0.0, 0.2).
Summary
Studies of children treated with radiation (X-rays and γ-rays) for benign disease also provide valuable information about the carcinogenicity of low-LET radiation. Studies of patients treated for tinea capitis, enlarged thymus, and benign head and neck diseases have provided much of the quantita-
tive information on the risk of thyroid cancer related to external radiation in children. Studies of children treated for hemangioma (with average doses ranging from 0.09 to 0.4 Gy, depending on the target organ) have provided radiation risk estimates for cancers of the lung, breast, thyroid, and stomach, as well as for leukemia. These estimates are reviewed in detail, and compared with risk estimates derived from other medical exposure studies, in the section of this chapter “Evaluation of Risk for Specific Cancer Sites.”
No study has focused specifically on populations exposed to 131I in childhood or adolescence for the treatment of hyperthyroidism.
Diagnostic Radiation Among Adults
Chest Fluoroscopy for Follow-up of Pulmonary Tuberculosis
A cohort study of 64,172 tuberculosis patients was carried out in Canada to assess the risk of cancer associated with multiple fluoroscopies (Miller and others 1989). In this cohort, 25,007 patients were exposed to highly fractionated radiation from repeated fluoroscopic examinations used to monitor lung collapse from pneumothorax treatment. Howe (1995) studied the risk of lung cancer in this cohort. Absorbed lung doses from fluoroscopy were estimated for each patient for each year since admission for tuberculosis. This involved obtaining counts from medical records of the number of fluoroscopies each year. This number was combined with information on dose per fluoroscopy obtained from the output of typical fluoroscopes used during the relevant period, the estimated organ dose per unit of surface exposure based on human phantom experiments, and interviews with physicians who administered pneumothorax during the relevant period. The average lung dose per fluoroscopy session was estimated to be 11 mGy. The mean total dose to the lung was 1.02 Gy (range 0–24.2 Gy), and the mean number of fractions was 92. During the study period (1950–1987), 1178 lung cancer deaths occurred. There was no evidence of an association between risk of lung cancer and dose: the ERR at 1 Gy was 0.00 (95% CI −0.06, 0.07). The authors conclude that their study supports the hypothesis of a substantial reduction in risk related to fractionation for low-LET radiation.
Howe and McLaughlin (1996) also reported analyses of breast cancer mortality in relation to radiation dose in this cohort. Estimates of dose to the breast were derived as above. The mean dose to the breast varied across provinces: it was 2.1 Gy (range 0–18.4) in Nova Scotia and 0.79 Gy (range 0–14.4) elsewhere. This difference was related to a difference in practices, with a much larger proportion of the examinations in Nova Scotia being carried out in an anterior-posterior orientation, and hence resulting in a higher breast dose than elsewhere. A total of 681 (103 in Nova Scotia and 578 elsewhere) deaths from breast cancer were identified during the study period. A strong dose-response association was seen in this study, with the ERR decreasing significantly with increasing age at exposure. The ERR appeared to be constant from 5 to 39 years after exposures, with a suggestion of a decrease after that. The ERR for exposure at age 15 was estimated to be 0.90 Gy−1 overall but differed significantly between regions: it was 3.56 Gy−1 (95% CI 1.85, 6.82) in Nova Scotia and 0.40 Gy−1 (95% CI 0.13, 0.77) elsewhere. The EAR 20 years later for exposure at age 15 was estimated to be 10.3 per 104 PY per gray (95% CI 6.37, 16.2) in Nova Scotia and 1.22 per 104 PY per gray (95% CI 0.42, 2.34) elsewhere; the overall estimate is 3.16 per 104 PY per gray. The difference in risk between Nova Scotia and elsewhere does not have an obvious explanation. Doses in Nova Scotia were generally less fractionated than elsewhere, and the dose per fraction was higher; hence this could be a dose-rate or fractionation effect. Comparisons of results with estimates from the study of atomic bomb survivors, however, show that the ERRs/Gy are fairly consistent and do not therefore suggest a major effect of fractionation.
The mortality experience of 6285 women patients who received repeated fluoroscopic examinations to monitor lung collapse for treatment of tuberculosis between 1925 and 1954 in Massachusetts was studied by Davis and colleagues (1989). A control cohort of 7100 nonirradiated tuberculosis patients was also studied. No significant increase in the mortality from all cancers, from lung cancer, or from leukemia was seen in the exposed cohort. Increases in mortality from breast and esophageal cancers were observed based on 62 and 14 deaths, respectively.
The incidence of breast cancer was studied further among 4940 women from the above cohort who could be followed from 1970 or 1980 (depending on the subcohort) until 1989 (Boice and others 1991b). Estimates of absorbed dose to the breast were derived by taking into account the number of lung collapse treatments, calendar year of exposure, age at exposure, and exposure settings of the fluoroscopy machines in use at the time. Physicians who conducted the examinations—and patients themselves—were interviewed about the fluoroscopy procedures during the lung collapse sessions. Among exposed women, the mean dose to the glandular tissue of the breast was estimated to be 0.79 Gy and the mean number of fluoroscopies was 88. The average length of follow-up of the cohort was 22 years. Among the 2573 women examined with fluoroscopy, 147 breast cancers developed during the follow-up period (SIR 1.29; 95% CI 1.1, 1.5). No increased risk of breast cancer was seen among the 2367 women treated by other means. Breast cancer risk increased significantly with increasing radiation dose. The overall ERR was estimated to be 0.61 Gy−1 (95% CI 0.3, 1.01) and the EAR 10.7 per 104 PY per gray (95% CI 6.0, 15.8). The risk decreased significantly with increasing age at exposure; the ERRs were estimated to be 1.0, 0.7, 0.1, and 0.1 Gy−1, respectively, for exposures at age 15, 20, 35, and 45 years. The excess breast cancer risk was not apparent until 15 years after exposure and remained high for the period of observation (over 50 years).
Other Uses of Diagnostic X-Rays in Adults
Preston-Martin and coworkers (1988) carried out two population-based, case-control studies of cancer risk in relation to prior exposure to diagnostic X-rays, one of tumors of the parotid gland and the other of chronic myeloid and monocytic leukemia. Significant associations between reported numbers of X-rays (and estimated doses) were seen for both diseases. Results from these studies should be interpreted with caution, however, because the information on past exposures was self-reported, obtained by questionnaire, and therefore subject to recall bias and uncertainty.
In a U.S. case-control study of 565 leukemia patients, 318 NHL patients, 208 multiple myeloma patients, and 1390 matched controls, the history of diagnostic X-ray exposure was ascertained from medical records held by two health plans (Boice and others 1991a) and, hence, was not subject to recall bias. Each diagnostic X-ray procedure was assigned a probable dose to the active bone marrow (averaged over the whole body) based on an extensive literature review. After excluding examinations within 2 years of diagnosis, no association was found between dose of radiation from diagnostic X-rays and the risk of leukemia or NHL. The risk of multiple myeloma, however, was increased among those patients who were frequently exposed to X-rays. No estimate of risk per dose is presented.
Inskip and colleagues (1995) carried out a case-control study of thyroid cancer among residents of the Uppsala Health Care Region in Sweden to assess the relationship between diagnostic X-ray exposure and the risk of thyroid cancer. The study included 484 cases diagnosed between 1980 and 1992 and an equal number of age-, sex-, and country of residence-matched controls. Lifetime residential history of study subjects was compiled and radiological records were searched at all hospitals serving regions where study subjects had lived. Approximate radiation doses to the thyroid gland were estimated for different types of X-ray examinations based on historical measurements made in Sweden and the United States. No association was seen between estimated radiation dose and the risk of thyroid cancer.
Diagnostic Iodine-131 Exposures
Holm and colleagues (1988) studied the incidence of thyroid cancer in a cohort of 35,074 patients who had received diagnostic 131I exposures for suspected thyroid disorders in Sweden between 1951 and 1969; 50 thyroid cancer cases were observed between 1958 and 1985, compared to 39.4 expected in the general population (SIR 1.27; 95% CI 0.94, 1.67). The risk of thyroid cancer increased with increasing 131I activity. Those who were examined for a suspected thyroid tumor tended to have received higher 131I activities, however, and there was no increased incidence among those examined for other reasons (SIR 0.62; 95% CI 0.35, 1.00, based on 16 cases). The increased risk was highest 5–9 years after examination, and there was no evidence of a dose-response 10 years or more after exposure.
In a follow-up paper, Holm and colleagues (1989) reported on cancer risk in this population over the same period. For each patient, information on delivered 131I activity, date of administration, 24-h thyroid uptake, and reason for examination was abstracted from medical records. Dose estimates were derived from this information using ICRP (1988) conversion factors. The average activity delivered to the thyroid was 1.9 MBq, which would result in radiation doses of less than 10 mGy to organs other than the thyroid. A total of 3746 cancers occurred in this cohort in the first 5 years after examination. No significant increase was seen in the incidence of cancer overall (SIR 1.01; 95% CI 0.98, 1.04). Significantly increased SIRs were noted, however, for endocrine tumors other than the thyroid, lymphoma, leukemia, and nervous system tumors. Cancer risk appeared to increase with increasing dose of 131I in years 5–9 after examination only and was significantly elevated only for subjects who received more than 2.7 MBq. No dose-related increase was seen 10 or more years after follow-up.
Hall and colleagues (1992) reported on combined analyses of leukemia incidence among 46,998 Swedish patients who had received 131I for either diagnostic or therapeutic purposes (these include most of the 35,074 subjects from Holm and others (1988) and the 10,552 from Holm and others (1991). The mean absorbed dose to the bone marrow was estimated to be 14 mGy (range 0.01–2.23). The average duration of follow-up was 21 years: 195 leukemias occurred more than 2 years after exposure and the SIR was 1.09 (95% CI 0.94, 1.25). Similar but not significantly increased risks were seen for CLL and non-CLL. A significant excess of CLL was observed among those who received more than 100 mGy to the red bone marrow. The leukemia risk did not vary with level of 131I radiation dose.
Hall and colleagues (1996) also reported on follow-up up to the end of 1990 of thyroid cancer incidence among 34,104 of the patients who had received 131I diagnostic exposures for suspected thyroid disorders in Sweden between 1951 and 1969 (Holm and others 1988). Dose to the thyroid was estimated as in Holm and coworkers (1989), taking into account information on thyroid mass from patient records and scintigrams. The mean dose to the thyroid was estimated to be 1.1 Gy, and 67 thyroid cancers were identified during the study period. The SIR was 1.35 (95% CI 1.05, 1.71). The excess thyroid cancer risk was restricted to those patients who had been examined for suspicion of thyroid tumor. Risk was not related to radiation dose to the thyroid, time since exposure, or age at exposure. Among the 2408 patients under age 20 at the time of the examination, a small excess risk was seen (3 cases observed versus 1.8 expected).
Summary
Studies of populations with diagnostic radiation exposures are, in principle, more suited than studies of therapeutic exposures to the evaluation of health risk following low doses of radiation. However, most of the studies on diagnostic X-rays reviewed do not provide risk estimates and hence are not informative for the purpose of this report. The exception is the studies of patients who received repeated chest fluoroscopies to monitor lung collapse in the treatment of tuberculosis. Careful dose reconstruction to the lung was carried out in a study in Canada and to the breast in studies in Canada and Massachusetts, allowing the quantification of risk to these organs. It is noted that average doses in studies of patients receiving fluoroscopies are high, however, of the order of 1–2 Gy to the lung and breast because of the very large number of such procedures the patients had to undergo. Estimates from these studies are reviewed in detail, and compared with risk estimates derived from other medical exposure studies, in the section “Evaluation of Risk for Specific Cancer Sites.”
Studies of diagnostic 131I exposures reviewed did not provide estimates of risk per unit dose.
Diagnostic Radiation Among Children
Scoliosis
In 1989, Hoffman and colleagues reported a doubling in the incidence of breast cancer in a pilot study of 1030 women who had received multiple diagnostic X-rays between 1935 and 1965 for evaluation of scoliosis during childhood and adolescence. These results were explored further in the U.S. Scoliosis Cohort Study (Doody and others 2000), which included 5573 women patients with scoliosis who had been referred to one of 14 orthopedic centers in the United States. The cohort included only cases of scoliosis diagnosed before age 20 between 1912 and 1965. Information on personal characteristics and scoliosis history was abstracted from medical records of participating institutions, together with radiology reports, radiograph jackets, and radiology log-books to determine for each examination the date, field, view, position, size of the radiograph, and other factors necessary to determine dose to the breast.
Manufacturers of the radiograph machines that had been used in the study centers completed a questionnaire concerning machines and parameters during the study period. Dose to the breast was estimated for each examination for which the breast was in the beam. For each examination, the breast was classified as preteen (<13 years old) or teen and adult combined ( 13 years) depending on the age of the patient at the time; dose to the breast was estimated at a depth of 1 cm for preteen breasts and at 2.5 cm otherwise. The average number of examinations per patient was 24.7 (range 0–618), and the mean cumulated dose to the breast was 0.11 Gy (range 0–1.7 Gy); 631 women had no radiographic examination. The mean age at diagnosis of scoliosis in the cohort was 10.6 years, and the average length of follow-up was 40.1 years. A total of 77 breast cancer deaths were observed in the cohort compared to 45.6 expected based on U.S. national rates. The risk increased significantly with cumulative radiation dose; the unadjusted ERR was 5.4 Gy−1 (95% CI 1.2, 14.1); adjustment for type of treatment or age at first radiographic examination reduced the risk, as did restriction of analysis to women who had at least one radiographic examination (ERR 2.7; 95% CI −0.2, 9.3). Findings from this study must be interpreted with caution because dose to the breast could be underestimated (records were available only of radiographs from participating institutions and did not include those made before referral to these institutions). Further, a number of factors may confound the association between radiation dose and risk of breast cancer, such as the severity of disease, which may affect reproductive history and hence breast cancer risk.
CT scans
As indicated in the introduction to this chapter, there is concern about the potential health effect of repeated CT scan exposures, particularly in childhood. No epidemiologic study of populations exposed to CT was available to the committee.
Brenner and colleagues (2001) and Brenner and Elliston (2004) have evaluated the possible consequences of CT exposures based on estimated doses to specific organs. They conclude that lifetime risks of cancer are not negligible.
Exposure In Utero
Prenatal X-rays were first associated with increased risk of childhood leukemia and cancer in the 1950s in the Oxford Survey of Childhood Cancers (OSCC), a U.K.-wide study begun in 1955 (Stewart and others 1958). Results were based on a case-control study of 1416 childhood cancer deaths and the same number of controls, in which mothers of the study subjects were asked about their child’s history of radiographic examinations (in utero and after birth). This association was confirmed by MacMahon (1962) in a study of a cohort of 734,243 children born in the northeastern United States between 1947 and 1954, in which 584 subjects had died of cancer in childhood and information about prenatal X-rays was obtained from medical records, thus eliminating the possibility of recall bias.
The OSCC is the largest study of childhood cancer after prenatal exposure to X-rays. It has continued and been expanded to cover all children dying from malignant disease in the United Kingdom under the age of 16 (Bithell and Stewart 1975; Knox and others 1987; Gilman and others 1989); in 1981, it included 15,276 matched case-control pairs. The magnitude of the association appears to have diminished over time (Muirhead and Kneale 1989), but so has the dose
of radiation to which pregnant women have been exposed during examinations (Doll and Wakeford 1997). A decrease over time also was reported in the northeastern U.S. study (Monson and MacMahon 1984).
The possible effect of prenatal exposure has been studied in a number of other populations in the United States and Europe. Results of the case-control studies have been combined in meta-analyses by Bithell (1989, 1990). Although dominated by the OSCC, results of these studies show a significant RR of 1.4 for in utero radiation in association with childhood cancer (Doll and Wakeford 1997).
Controversy continues, however, about the existence and size of the risk following prenatal exposure. Boice and Miller (1999) noted that the increases were restricted to case-control studies and were not seen in cohort studies; they also commented on the similarity of relative risks for leukemia and solid cancers, suggesting an underlying bias in the case-control studies. In their review, Doll and Wakeford (1997) discuss these arguments. In regard to cohort studies, they combine the results of cohort studies for which relative risks can be calculated reliably and note that, when the atomic bomb survivors are excluded, an increased risk is obtained that is consistent with the combined results of case-control studies. They note further that the incomplete follow-up of the Japanese atomic bomb survivor cohort in the years after the bombings may be partially responsible for the apparent inconsistency of results concerning the effects of prenatal exposures. The argument that radiation risks for leukemia and solid cancers differ is based on observations of exposure in childhood and later years. Doll and Wakeford (1997) note that the carcinogenic effects of radiation exposure in utero and in childhood are not expected to be the same because the cells that give risk to most of the typical childhood cancers other than leukemia persist and are capable of dividing for only a short time, if at all, after birth. Doll and Wakeford further conclude that the idea of a causal relationship is supported by the increase in RR with increasing number of X-ray examinations conducted in the third trimester of pregnancy and the significant decline in RR with year of birth, paralleling the decline in fetal doses that occurred over the same period (UNSCEAR 1972).
Based on the results of the Oxford survey and other studies of the effects of maternal irradiation, UNSCEAR (1996) reported a statistically significant leukemia risk (up to age 15 years) and estimated a 40% increase in risk of childhood cancers (up to 15 years) at doses of 10–20 mGy (low LET). Risk estimates have been derived since then by a number of authors and committees (UNSCEAR 1996; Doll and Wakeford 1997; Wakeford and Little 2003). In the most recent analyses, Wakeford and Little (2003) derive an ERR for childhood cancer following prenatal exposure of about 50 Gy−1, with an EAR of about 8% Gy−1. They comment, however, that statistical, dosimetric, modeling, and other uncertainties associated with these risk estimates are appreciable. They also note that when these uncertainties and those associated with equivalent risk coefficients from the Japanese atomic bomb survivor cohort exposed in utero are taken into account, the risk estimates for childhood cancer from these two sources of data are compatible and they conclude that “doses to the fetus in utero of the order of 10 mSv discernibly increase the risk of childhood cancer.”
Diagnostic 131I Exposures
The use of 131I for diagnostic purposes in childhood is rare; hence information on risk is very sparse. In the cohort of 34,104 patients who had received 131I diagnostic exposures for suspected thyroid disorders in Sweden between 1951 and 1969, reported by Hall and colleagues (1996), only 2408 patients were under age 20 at the time of the examination. Among these, a small excess risk was seen (3 cases observed vs. 1.8 expected).
Summary
Information on radiation risks following diagnostic radiation exposure in childhood comes from a study of women who received multiple diagnostic X-rays for the evaluation of scoliosis during childhood and adolescence. This study, in which important efforts were made to reconstruct dose to the breast, has provided an estimate of the risk of radiationinduced breast cancer. This estimate is reviewed, and compared with risk estimates derived from other medical exposure studies, in the following section.
Studies of prenatal exposure to diagnostic X-rays have, despite long-standing controversy, provided important information on the existence of a significantly increased risk of leukemia and childhood cancer following diagnostic doses of 10–20 mGy in utero.
Only one study has examined the effects of using 131I for diagnostic purposes in childhood. A small excess of thyroid cancer risk was seen—based on very small numbers—and no risk estimate is provided.
EVALUATION OF RISK FOR SPECIFIC CANCER SITES
This section reviews radiation risk estimates for five types of malignancies (lung cancer, female breast cancer, thyroid cancer, leukemia, and stomach cancer). The results of analyses of the risk of heart disease following medical radiation exposures are also reviewed.
Lung cancer was chosen because it is the most common malignancy among humans. Breast cancer was selected because breast tissue in young women is responsive to low levels of low-LET radiation, and thyroid cancer was chosen because of the inherent radiosensitivity of the thyroid gland. Leukemia was chosen because bone marrow is sensitive to low levels of low-LET radiation, and stomach cancer was selected because of its high incidence in many parts of the
world, including Japan. Breast cancer, thyroid cancer, and leukemia were evaluated in the BEIR V (NRC 1990) report.
As far as feasible, the effects of both external and internal (131I) low-LET radiation exposures are considered separately. The focus on low-LET irradiation is related to the goal of BEIR VII to investigate the magnitude of risk from these radiation types. Although the focus of this report is the effects of low doses (i.e., doses less than 100 mGy), results of medium- and high-dose studies are also reviewed because they provide important insights into modifiers of radiation risks that cannot, at present, be studied in populations with lower-dose medical exposures.
The information presented in the previous section of this chapter was used to identify the studies that are informative for radiation risk estimation and have provided estimates of risk per gray in a comparable fashion (either as ERR or as EAR). The estimates have been taken from the original publications. When such estimates were not available in the original study reports, these studies have not been included in this section, with the exception of the study of breast cancer in cervical cancer survivors (for which risk estimates were taken as derived by UNSCEAR 2000). No estimation was attempted by the committee because there is considerable uncertainty in deriving risk estimates based on only an average dose in populations with dose distributions that are often skewed and include subjects with very high, cell-killing doses.
The estimates from these studies are summarized in Tables 7-2 to 7-6 and Figures 7-1 to 7-6. In the following sections, differences and similarities among risk estimates are discussed, and conclusions are drawn, where possible, about radiation risks for each of the cancer sites of interest. Since the conditions of exposure, the characteristics of the study populations, and the extent and quality of the dosimetry and follow-up differ widely, the risk estimates derived for individual studies are not strictly comparable. They do, however, illustrate the range and significance of estimates obtained and provide some indication of the influence of the study-specific factors involved.
Lung Cancer
Lung cancer is the leading cause of cancer mortality in industrialized countries, and its incidence is rising in many developing countries. Cigarette smoking is accepted to be the primary cause of lung cancer. Also, ionizing radiation
TABLE 7-2 Risk Estimates for Cancer Incidence and Mortality from Studies of Radiation Exposure: Lung Cancer
has been implicated as a lung carcinogen (UNSCEAR 2000b).
Of all the studies reviewed above, only seven provide dose-specific estimates of ERR and only one provides an estimate of the EAR. Table 7-2 and Figure 7-1 summarize the results from these studies. In the figure, results are shown for all studies as well as those restricted to an average dose to less than 1 Gy.
In the incidence studies, the ERR/Gy ranges from 0.15 Gy−1 in survivors of HD to 1.4 Gy−1 in patients treated for hemangioma in infancy. In mortality studies, estimates range from 0.00 per gray among tuberculosis patients exposed to fluoroscopy to 0.43 Gy−1 in patients treated with radiation for peptic ulcer. Although risk estimates from these studies vary, confidence intervals are very large and the estimates shown are therefore statistically compatible.
In interpreting the results of these studies, differences in study populations and exposure patterns must be taken into account: the hemangioma study (which had 11 cases of lung cancer) included only patients who were exposed in infancy, while the average age in other cohorts ranged from 28 in tuberculosis patients exposed to fluoroscopic X-rays to 50 years among breast cancer survivors. Exposure rate and exposure pattern also vary across studies, with hemangioma patients having received a low-dose-rate, protracted exposure (over a period of 1 d to more than 2 years) and tubercu-
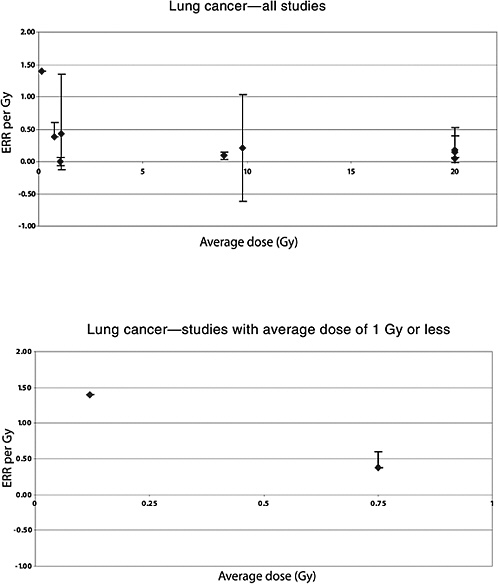
FIGURE 7-1 Distribution of study-specific estimates of ERR per gray for lung cancer according to average dose to the lung. Results are shown for all studies as well as studies in which the average dose to the lung was less than 1 Gy.
losis patients having received a highly fractionated dose (with 92 fluoroscopy sessions on average) at a low dose rate.
The ERR/Gy from the studies of acute high-dose-rate exposures are compatible and in the range 0.1–0.4 Gy−1. The study of HD survivors showed little evidence for nonlinearity of the dose-response, despite the fact that the majority of patients received very high doses to the lung (in excess of 30 Gy). A multiplicative interaction was seen in this study between radiation dose and tobacco smoking, with a smaller ERR/Gy among women than men (difference not statistically significant).
It is difficult to evaluate the effects of age at exposure or of exposure protraction based on these studies because only one study (the hemangioma cohort) is available in which exposure occurred at very young ages and protracted low-dose-rate exposures were received. Risk estimates from that study are higher than those seen in other studies, but the difference is not statistically significant.
The study of tuberculosis patients, based on a very large number of lung cancer deaths, appears to indicate that substantial fractionation of exposure leads to a reduction in risk.
Female Breast Cancer
Breast cancer is the most commonly diagnosed cancer and cause of cancer mortality among women in North America and Western Europe. Incidence rates are lower in Asian countries. Ionizing radiation is well documented as a cause of breast cancer in women, especially when exposures occur in childhood and around puberty (UNSCEAR 2000b).
Of all the studies reviewed in the medical uses of radiation section above, only 11 provide dose-specific estimates of ERR and/or EAR. Table 7-3 and Figure 7-2 summarize the results from these studies. In the figure, results are shown for all studies as well as restricted to studies in which the average dose to the breast was less than 1 Gy.
In the incidence studies, the ERR/Gy ranges from 0.15 Gy−1 in women who received very high doses for HD radiotherapy (mean among cases = 25 Gy) to 2.5 Gy−1 in populations irradiated for enlarged thymus in infancy. The range in mortality studies is similar: from 0.08 Gy−1 among patients irradiated for treatment of ankylosing spondylitis to 2.7 Gy−1 in women repeatedly exposed to X-rays to monitor scoliosis. As indicated previously, in the international cervical cancer follow-up study (Boice and others 1988) the significant reduction of risk seen among women with intact ovaries was probably attributable to the cessation of ovarian function related to radiotherapy; only the risk estimates in women with no ovaries are considered here. Similarly, the results from Travis and colleagues are restricted to women who had chest radiotherapy only (and hence exclude women with high doses to the ovaries).
Although the risk estimates from these studies vary considerably, confidence intervals are very large and the estimates shown are therefore statistically compatible, except for the study of HD patients where the doses to the breast (up to more than 60 Gy) will have led to cell killing and hence a reduction of risk per gray.
The situation is somewhat different for EAR. Few studies have reported risk estimates in terms of EAR. The estimates shown in Table 7-3 and Figure 7-2 are quite variable, and several of the confidence intervals do not overlap, indicating heterogeneity in risk estimates across these studies. In reviewing these results, differences in study populations and exposure patterns must be taken into account. These include the following:
-
The thymus and hemangioma studies relate mainly to patients who were irradiated in infancy; in the scoliosis study, the mean age at first exposure was 10.1 years, while in the other studies, the majority of subjects were adults at the time of radiation exposure. Mean ages ranged from 25 to 52 years, respectively, in the Massachusetts fluoroscopy study and the cervical cancer survivor study.
-
Exposure patterns ranged from very protracted low-dose-rate or fractionated exposures in the scoliosis and tuberculosis studies, where diagnostic radiation was used to monitor the evolution of the disease, to high-dose-rate, acute or much less fractionated exposures received for treatment of disease.
-
Exposures in childhood and adolescence, particularly in the time around puberty (Doody and others 2000), have been shown to be associated with higher risks of radiation-induced breast cancer than exposures later in life. Figure 7-3 shows the relation between ERR/Gy and average age at exposure. The ERR appears to decrease with increasing age at exposure.
Exposure in infancy led to an ERR of 2.5 Gy−1 in the Rochester thymic irradiation study, based on 22 exposed cases, but the ERR in the pooled analysis of data on hemangioma patients was much lower, 0.35 Gy−1. A previous report of a much higher ERR in the Stockholm hemangioma cohort 50 or more years after exposure was not confirmed in the pooled analysis.
Exposure at age 15 in the Massachusetts fluoroscopy study was estimated to result in an ERR/Gy of 1.0, and the ERR for breast cancer mortality was 2.7 Gy−1 in the scoliosis study (with average age at first exposure of 10.1 years) and 0.9 Gy−1 overall in the Canadian fluoroscopy study for exposures between 15 and 25 years of age. Exposures at older ages tended to result in lower risk estimates, ranging from 0.33 among cervical cancer patients with no ovaries to about 0.61 in women exposed to fluoroscopy, and to about 1.63 following high-dose-rate irradiation for benign breast disease. The ERR was lower, but not statistically incompatible, in the ankylosing spondylitis study, probably related to the fact that the dose to the ovary was high among these women (ERR not shown in Figure 7-3).
Exposure fractionation does not appear to be an important determinant of risk per gray in the fluoroscopy studies.
TABLE 7-3 Risk Estimates for Cancer Incidence and Mortality from Studies of Radiation Exposure: Female Breast Cancer
Reference |
Study |
Radiation Type |
Average Dose (Gy) |
Dose Range |
Cases |
Controls/Population |
ERR per Gy |
LB |
UB |
EAR/104 PY/Gy |
LB |
UB |
Comments |
Incidence |
|||||||||||||
Boice and others (1988) |
Cervix |
External + 226Ra |
0.3 |
|
953 |
1,806 |
−0.20 |
<−0.2, |
0.3 |
|
Overall |
||
|
145 |
284 |
0.33 |
<−0.3, |
5.8 |
Women with no ovaries |
|||||||
Hildreth and others (1989) |
Thymus |
|
0.69 |
[0.02–7.5]a |
22 |
1,201 |
2.48 |
1.1 |
5.2 |
5.7 |
2.9, |
9.5 |
|
Boice and others (1991b) |
Fluoroscopy |
External |
0.79 |
[0.02–5]a |
147 |
2,573 |
0.61 |
0.3 |
1.01 |
10.7 |
6.0 |
15.8 |
Overall |
|
1.00 |
|
Exposure at age 15 |
||||||||||
|
0.70 |
Exposure at age 25 |
|||||||||||
0.10 |
Exposure at age 35 |
||||||||||||
Mattsson and others (1995) |
Benign breast disease |
External |
5.8 |
0–50 |
47 |
|
1.63 |
0.77 |
2.89 |
|
|||
Shore and others (1986) |
Postpartum mastitis |
External |
3.8 |
[0.6–14]a |
51 |
601 |
0.40 |
0.2 |
0.7 |
||||
Lundell and others (1996) |
Hemangioma |
Mostly 226Ra |
0.39 |
<0.01–5.8 |
75 |
9,675 |
0.38 |
0.09 |
0.85 |
0.4 |
|
|
Overall 50+ years after exposure |
0.22 |
|
16 |
|
2.25 |
0.59 |
5.62 |
22.9 |
|
|
||||
Lundell and others (1999) |
Hemangioma |
Mostly 226Ra |
0.29 |
<0.01–35.8 |
236 |
17,202 |
0.35 |
0.18 |
0.59 |
0.7 |
0.4 |
1.1 |
|
Travis and others (2002) |
HD survivors |
External |
22 |
<0.1–61.3 |
67 |
122 |
0.15 |
0.04 |
0.73 |
|
Women with chest radio-therapy only |
||
Mortality |
|||||||||||||
Weiss and others (1994) |
Ankylosing spondylitis |
External |
0.59 |
0.07–1.27 |
42 |
|
0.08 |
−0.3, |
0.65 |
NA |
NA |
Note—high dose to the ovary |
|
Howe and others (1996) |
Fluoroscopy |
External |
2.13 |
0–18.4 |
103 |
|
3.56 |
1.85 |
6.82 |
10.3 |
6.4 |
16.2 |
Nova Scotia: for exposures at age 15—EAR 20 years after exposures |
|
0.79 |
0–14.4 |
578 |
|
0.40 |
0.13 |
0.77 |
1.2 |
0.4 |
2.3 |
Other provinces: for exposures at age 15—EAR 20 years after exposures |
||
|
681 |
|
0.90 |
NA |
3.2 |
|
Overall: for exposures at age 15—EAR 20 years after exposures |
||||||
Doody and others (2000) |
Scoliosis |
External |
0.11 |
0–1.7 |
70 |
4,942 |
2.7 |
−0.2 |
9.3 |
|
Among women with at least one radiographic examination |
||
NOTE: The number of cases and controls (or population size in cohort studies) as well as the mean dose and range relate only to exposed persons. Empty cells indicate data not available from publication. LB = lower bound; UB = upper bound of CI. aFrom Preston and others 2002. |
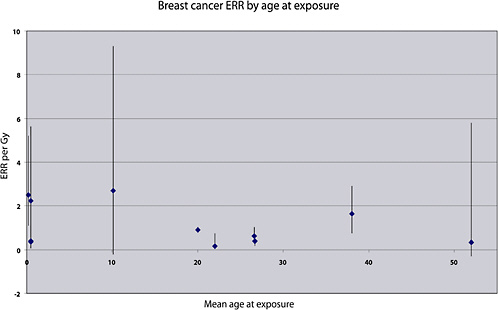
FIGURE 7-3 Distribution of study-specific estimates of ERR/Gy for breast cancer according to average age at exposure.
Protraction of low-dose-rate exposure in the hemangioma cohort may account for the reduced risk following exposures in infancy in this cohort, although analyses within this study do not indicate a significant association.
Preston and colleagues (2002b) carried out a pooled analysis of eight cohorts to estimate radiation-induced breast cancer risk and evaluate the role of modifying factors. The analyses included studies of the following populations: Japanese atomic bomb survivors (Thompson and others 1994), the original and extended Massachusetts TB fluoroscopy cohorts (Boice and others 1991b), the New York acute post partum mastitis cohort (Shore and others 1986), the Rochester infant thymic irradiation cohort (Hildreth and others 1989), the Swedish benign breast disease cohort (Mattsson and others 1993), and the Gothenburg and Stockholm skin hemangioma cohorts (Lindberg and others 1995). The analyses included 1502 breast cancer cases among 77,527 women, about half of whom were exposed to radiation, with 1.8 million person-years of follow-up. No simple unified summary model adequately described the excess risk in all of these studies.
The excess risks for the thymus, tuberculosis, and atomic bomb survivor cohorts showed similar temporal trends, depending on attained age in the ERR model and on both age at exposure and attained age in the EAR model. The excess rates appeared to be similar in these cohorts, with a combined EAR estimate of 9.9 per 104 PY per gray (95% CI 7.1, 1.4) at age 50, suggesting similarity of risks following acute and fractionated low-dose-rate exposure. The ERR/Gy was greater among Japanese atomic bomb survivors; this difference may be partly attributed to the lower background rates of breast cancer in Japan.
The excess rates were higher for the mastitis and benign breast disease cohorts with EAR estimates of 15 (95% CI 7.7, 24) and 32 (95% CI 21, 47) per 104 PY per gray, respectively, suggesting that women with some benign breast conditions may be at an elevated risk of radiation-induced breast cancer.
The hemangioma cohorts showed lower risks (EAR: 5.1 per 104 PY per gray; 95% CI 1.3, 11), suggesting a reduction of risks following protracted low-dose-rate exposures.
Thyroid Cancer
Thyroid cancer is one of the less common forms of cancer. Its incidence is relatively high before age 40, it increases
comparatively slowly with age, and it is about three times higher in women than men. Ionizing radiation is a well-documented cause of thyroid cancer (UNSCEAR 2000b).
Of all the studies reviewed in the medical uses of radiation section above, only six provide dose-specific estimates of ERR and/or EAR. Table 7-4 and Figure 7-4 summarize the results from these studies. In Figure 7-4, results are shown for all studies as well as restricted to studies in which the average dose to the thyroid was less than 1 Gy.
All of the studies shown are studies of children who received radiotherapy for benign conditions. All results relate to thyroid cancer incidence. Because of the relatively good prognosis of most papillary thyroid cancers, studies of thyroid cancer mortality add little information about radiation risks.
In studies of external radiation exposure, the ERR/Gy ranges from 3 Gy−1 in children exposed for enlarged tonsils and other benign head and neck disorders to 30 Gy−1 among those exposed in Israel for the treatment of tinea capitis. Similarly, the estimates of EAR vary from 0.9 per 104 PY per gray in the hemangioma study to 13 in the tinea capitis study. Although risk estimates from these studies vary considerably, the confidence intervals tend to be large; it is likely that the estimates shown are statistically compatible.
Ron and colleagues (1995a) conducted combined analyses of data from seven studies including five cohort studies—the atomic bomb survivors study, the Rochester thymic irradiation study (Shore and others 1993a), the Israeli tinea capitis study (Ron and others 1989), and the Michael Reese and Boston enlarged tonsil studies (Pottern and others 1990; Schneider and others 1993)—as well as two case-control studies of thyroid cancer nested respectively within the International Cervical Cancer Survivor Study (Boice and others 1988) and the International Childhood Cancer Survivor Study (Tucker and others 1991). The analyses included a total of 707 cases, the majority of which (apart from the A-bomb and cervical cancer survivors) were below age 15 at time of exposure.
For subjects exposed below age 15, a linear dose-response was seen with a leveling or decrease in risk at the higher doses used for cancer therapy. The pooled ERR was 7.7 Gy−1 (95% CI 2.1, 28.7), based on a random effects model that took into account the heterogeneity of risk across studies. The EAR was estimated to be 4.4 per 104 PY per gray (95% CI 1.9, 10.1). Both of these estimates were significantly affected by age at exposure, with a strong decrease in risk with increasing age at exposure and little apparent risk for exposures after age 20. The ERR appeared to decline over time
TABLE 7-4 Risk Estimates for Cancer Incidence and Mortality from Studies of Radiation Exposure: Thyroid Cancer
Reference |
Study |
Radiation Type |
Average Dose (Gy) |
Dose Range |
Cases |
Controls/Population |
ERR/Gy |
95% CI |
EAR/104 PY/Gy |
LB |
UB |
Comments |
Incidence |
||||||||||||
Ron and others (1989) |
Tinea capitis |
External |
0.09 |
|
98 |
10,834 |
30.0 |
|
13.0 |
|
||
X-ray |
|
|||||||||||
Shore (1992) |
Meta-analysis of hyperthyroidism studies |
131I |
88 |
|
2 |
602 |
0.30 |
(0, 0.9)a |
0.1 |
(0.0, 0.2)a |
|
|
Schneider and others (1993) |
Benign head and neck |
External |
0.6 |
|
309 |
234 |
3.00 |
|
Overall |
|||
X-ray |
0.6 |
109 |
|
9.20 |
Before 1974 and screening program |
|||||||
|
0.6 |
200 |
|
1.80 |
After 1974 |
|||||||
Shore and others (1993a) |
Enlarged thymus |
External |
1.36 |
0.03–10 |
37 |
2,657 |
9.00 |
(4, 24)a |
2.9 |
(2.1, 3.9)a |
|
|
X-ray |
|
|||||||||||
Lundell and others (1994) |
Hemangioma |
Mostly |
1.07 |
<0.01–4.34 |
17 |
14,351 |
4.92 |
(1.26, 10.2) |
0.9 |
|
||
|
Ra |
|
||||||||||
Lindberg and others (1995) |
Hemangioma |
226Ra |
0.12 |
|
15 |
11,807 |
7.50 |
(0.4, 18.1) |
1.6 |
|
||
Ron and others (1995a) |
Pooled analysis of 7 studies |
External |
|
|
700 |
58,000 |
7.7 |
(2.1, 28.7) |
4.4 |
(1.0, 10.1) |
|
|
X-ray |
|
|||||||||||
NOTE: The number of cases and controls (or population size in cohort studies), as well as the mean dose and range, relate to exposed persons only. Empty cells indicate data not available from publication. LB = lower bound; UB = upper bound of CI. a90% CI. |
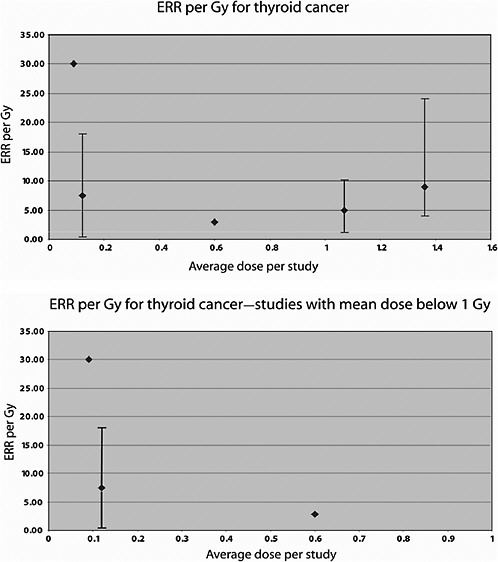
FIGURE 7-4 Distribution of study-specific estimates of ERR/Gy for thyroid cancer according to level of average thyroid dose. NOTE: The estimate from populations exposed to 131I is excluded.
about 30 years after exposure but was still elevated at 40 years.
Three studies provided data on exposure protraction or fractionation (thymus, tinea capitis, and Michael Reese); analyses indicate that a small nonsignificant decrease in risk may be related to exposure fractionation in these studies.
A meta-analysis of hyperthyroidism studies provides a risk estimate of thyroid cancer in relation to 131I exposure in childhood (Shore 1992). The ERR estimate from that study is 0.3 Gy−1, lower than that from studies of external exposures, but based on only two exposed cases. This study therefore provides little information about the risk of thyroid cancer in relation to exposure to this nuclide. Studies of the effects of 131I exposure later in life are reviewed in the preceding section, although no dose-related estimate of risks have been provided. These studies, taken together, provide little evidence of an increased risk of thyroid cancer following 131I exposure after childhood.
Leukemia
Leukemia is one of the less common malignancies, but substantial epidemiologic and experimental information exists on the leukemogenic effects of ionizing radiation (UNSCEAR 2000b).
Of all the studies reviewed in the “Medical Uses of Radiation” section, six provide dose-specific estimates of ERR and/or EAR. Table 7-5 and Figure 7-5 summarize the results from these studies. In the figure, results are shown for all studies as well as restricted to studies in which the average dose to the active bone marrow was less than 1 Gy. Results shown are for leukemia excluding CLL in all studies except the tinea capitis and uterine bleeding studies.
The ERRs/Gy shown in Table 7-5 range from 0.88 Gy−1 in women who received an average dose to the active bone marrow of 7 Gy from radiotherapy for cervical cancer to 12.4 Gy−1 in subjects treated for ankylosing spondylitis (average dose 4.4 Gy). All other estimates, from studies with average doses ranging from 0.1 to 2 Gy, are relatively close, in the range 1.9 to 5 Gy−1, and are statistically compatible.
Three studies have provided estimates of EAR per 104 PY per gray. Risk estimates between these studies are relatively close, ranging from 1 to 2.6.
In most of the studies included here, the majority of subjects were adults at the time of exposure (with average ages at exposure between 45 and 52 years in the uterine bleeding, benign breast disease, and cervical cancer survivor studies). Only the tinea capitis and hemangioma studies provide information about exposures in childhood. In the hemangioma study—where all subjects were irradiated in infancy—the overall ERR/Gy is similar to that seen in other studies; it is notable, however, that this is driven mainly by a higher ERR for childhood leukemia; the ERR for adult leukemia in this study was very close to zero. In the tinea capitis study, in which all exposures were below age 15, no ERR is shown; the EAR is similar to that seen in the other studies.
In one study (Inskip and others 1993), an effort was made to estimate separately the effects of external exposures, 226Ra, and the combination of the two. Estimates of risk from 226Ra alone or in combination with external radiation are
TABLE 7-5 Risk Estimates for Cancer Incidence and Mortality from Studies of Radiation Exposure: Leukemia Excluding CLL
Reference |
Study |
Radiation Type |
Average Dose (Gy) |
Dose Range |
Cases |
Controls/Population |
ERR per Gy |
95% CI |
EAR/104 PY/Gy |
LB |
UB |
Comments |
Incidence |
||||||||||||
Boice and others (1985) |
Cervix |
External |
7 |
|
143 |
745 |
0.88 |
(SE: 0.69) |
|
|||
X-rays + intracavitary |
|
|||||||||||
226Ra |
||||||||||||
Ron and others (1988b) |
Tinea capitis |
External |
0.3 |
|
14a |
10,834 |
|
|
0.9 |
|
||
X-ray |
|
|||||||||||
Inskip and others (1990b) |
Uterine bleeding |
226Ra |
0.53 |
|
34a |
4,483 |
1.90 |
(0.8, 3.2) |
2.6 |
|
||
Inskip and others (1993) |
Benign gynecological disease |
Overall |
|
39 |
8,352 |
2.90 |
|
1.2 |
||||
Rad + ext |
2.03 |
9 |
1,437 |
2.10 |
(0.5, 8.3) |
1.0 |
(0.3, 1.9) |
|
||||
226Ra |
2.31 |
26 |
5,508 |
3.70 |
(−1, 15) |
1.5 |
(0.3, 2.9) |
|||||
|
External |
0.59 |
4 |
1,407 |
0.50 |
(−0.6, 3.3) |
0.1 |
(−0.2, 0.6) |
||||
Lundell and Holm (1996) |
Hemangioma |
Mostly Ra |
0.13 |
<0.01–4.6 |
20 |
14,624 |
1.60 |
(−0.6, 5.5) |
|
Overall |
||
0.13 |
<0.01–4.6 |
9 |
14,624 |
5.01 |
(0.1, 15) |
Childhood leukemia only |
||||||
|
0.13 |
<0.01–4.6 |
11 |
14,624 |
−0.02 |
(−0.8, 1.9) |
Adult leukemia only |
|||||
Mortality |
||||||||||||
Weiss and others (1995) |
Ankylosing spondylitis |
External |
4.38 |
1.27–6.99b |
35 |
1,745c |
12.4 |
(2.25, 52.1) |
|
|||
X-ray |
|
|||||||||||
NOTE: The number of cases and controls (or population size in cohort studies) as well as the mean dose and range relate to exposed persons only. Empty cells indicate data not available from publication. LB = lower bound; UB = upper bound of CI. aAll forms of leukemia combined. b10–90% range. cSubcohort with reconstructed doses. |
similar; the ERR/Gy for external exposures appears to be lower, but this result is based on only four cases exposed.
Stomach Cancer
Incidence rates for stomach cancer vary considerably throughout the world, with particularly high rates in Japan. Many countries have seen decreases in incidence and mortality over the past 50 years or so, believed in large part to be due to healthier diets with increased fruits and vegetables and less salt.
Of all the studies reviewed on medical uses of radiation, five provide dose-specific estimates of ERR and/or EAR. Table 7-6 and Figure 7-6 summarize the results from these studies. In the figure, results are shown for all studies as well as restricted to studies in which the average dose to the active bone marrow was less than 1 Gy.
Among the studies of populations with external radiation exposure and/or 226Ra, the estimates of ERR/Gy range from negative (in the hemangioma study) to 1.3 Gy−1 in the study of benign breast disease. The confidence intervals are wide, and they all overlap, indicating that these estimates are statistically compatible. An ERR of 1.32 Gy−1 (not significantly different from zero) was seen among patients treated for hyperthyroidism with 131I.
Radiation and Circulatory Diseases
Although radiation exposure is well established as a risk factor for cancer, a clear understanding of the relationship between radiation exposure and other diseases is lacking. It has been postulated that the cardiovascular system is resistant to radiation-induced injury (Stewart and others 1995). However, it appears that tissue damage may occur as a result of both therapeutic (Stewart and Fajardo 1984) and A-bomb radiation exposure (Villeneuve and Morrison 1997; Shimizu and others 1999). Capillaries represent the most radiosensitive component of the cardiovascular system, with characteristic changes including detachment of endothelial cells and thrombosis. Arterial changes resulting from radiation exposure depend on vessel size, with small and medium-sized arteries undergoing changes in all vessel layers, and large arteries appearing to be relatively radioresistant, although radiation exposure may predispose larger vessels to the development of atherosclerosis (Louis and others 1974).
Radiation exposure has also been implicated in the development of cerebrovascular injury (O’Connor and Mayberg 2000). Specific conditions postulated to arise from irradiation include vasculopathy, intracranial aneurysm formation, cerebral radiation necrosis, intracranial atherosclerosis, and stroke (Trivedi and Hannan 2004).
Both animal and human studies have identified intimal thickening, lipid deposition, and adventitial fibroses of the
TABLE 7-6 Risk Estimates for Cancer Incidence and Mortality from Studies of Radiation Exposure: Stomach Cancer
Reference |
Study |
Radiation Type |
Average Dose (Gy) |
Dose Range |
Cases |
Controls/Population |
ERR/Gy |
95% CI |
EAR/104 PY/Gy |
LB |
UB |
Comments |
Incidence |
||||||||||||
Boice and others (1989) |
Cervix |
External |
2 |
0.5–3.5 |
348 |
658 |
0.54 |
(0.05, 1.5)a |
3.2 |
(0.1, 10.4) |
|
|
X-rays + intracavitary |
|
|||||||||||
226Ra |
||||||||||||
Holm and others (1991) |
Hyperthyroidism |
131I |
0.07 |
|
29 |
|
1.3 |
|
9.6 |
|
||
Lundell and Holm (1995) |
Hemangioma |
Mostly Ra |
0.09 |
5 |
14,351 |
<0 |
<0 |
|||||
Mattsson and others (1997) |
Benign breast disease |
External |
0.66 |
0–5.4 |
14 |
1,216 |
1.3 |
(0, 4.4) |
|
|||
Weiss and others (1994) |
Ankylosing spondylitis |
External |
3.2 |
0.52–5.8 |
127 |
1,745b |
−0.004 |
(−0.05, 0.05) |
||||
X-ray |
|
|||||||||||
Carr and others (2002) |
Peptic ulcer |
External |
8.9 |
|
11 |
1,859 |
0.20 |
(0, 0.73) |
|
Among subjects with stomach dose 10 Gy |
||
NOTE: The number of cases and controls (or population size in cohort studies) as well as the mean dose and range relate to exposed persons only. Empty cells indicate data not available from publication. LB = lower bound; UB = upper bound of CI. a90% confidence interval. bSubcohort with reconstructed doses. |
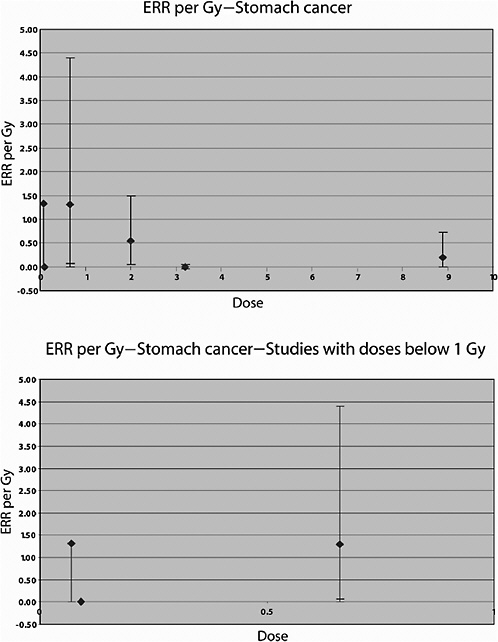
FIGURE 7-6 Distribution of study-specific estimates of ERR/Gy for stomach cancer according to level of average dose to the stomach.
vascular system following irradiation. These changes are associated with atherosclerosis and the normal aging process, although irradiation may accelerate the development of these conditions (Trivedi and Hannan 2004).
Although the dose required to produce specific conditions or vascular effects is uncertain, it appears that over extended periods, the nature of the changes induced are similar for low doses (on the order of 5 Gy) and for high doses (in the region of 40 Gy). There is a broad spectrum and severity of cardiovascular diseases, with radiation being only one of many possible risk factors that may act directly or indirectly on the vasculature. To clarify the role of radiation in the etiology of cardiovascular diseases, further studies involving long-term, low-level exposures are needed, taking into account all of the known risk factors for cardiovascular outcomes.
Excess heart disease mortality has been observed among women with breast cancer who were irradiated with cobalt-
60 (Host and Loeb 1986, not reviewed here) and among persons with HD who received mediastinal irradiation (Boivin and Hutchison 1982; Hancock and others 1993b, 1993c). Most affected patients had received at least 30 Gy to the mediastinum, although some had received less (Trivedi and Hannan 2004).
DISCUSSION
Since the publication of BEIR V (NRC 1990), new information concerning health effects of radiation exposures has become available from epidemiologic studies of populations exposed to medical uses of ionizing radiation. The longer follow-up periods in recent reports have increased the statistical power in examining dose-response relationships at the doses used for medical purposes.
Available studies of the effects of radiotherapy for malignant or benign diseases confirm the presence of a heightened risk of development of a number of primary or second primary cancers on follow-up. Because the doses in most series far exceed 100 mGy to the site of interest, they provide limited direct quantitative information on the risk of low-level radiation, particularly when they involve large doses where cell killing may lead to underestimation of the risk per unit dose. These studies provided valuable information for the study of risk modifiers, including age at exposure, attained age, and possible differences in patterns of risk across countries.
Analyses that are restricted to populations with low doses are complicated by the limitations of statistical variability as well as by limitations of sample size and study design, including dose reconstruction. Limitations also include chance, small undetected biases, and the consequences of doing multiple tests of statistical significance. Indeed, among diagnostic radiation studies, only studies of repeated chest fluoroscopy and scoliosis examinations are informative concerning the magnitude of ERR and EAR as a function of dose. It must be noted that although the dose rates in these studies are low, the cumulative doses received by tuberculosis patients are high, and even scoliosis patients followed radiologically for spine curvature received average cumulative doses of the order of 100 mGy or more.
Most of the information on radiation risks therefore still comes from studies of populations with medium to high doses, with the notable exceptions of childhood cancer risk following in utero exposures and thyroid cancer risk following childhood exposures, for which significant increases have been shown consistently in the low- to medium-dose range.
SUMMARY
In this chapter on medical radiation, particular attention has been paid to estimating the risk of cancer at specific sites—namely, the lung, breast, thyroid, and stomach, as well as leukemia risk. Information that has become available since 1989 has contributed to the examination of risks for these malignancies.
A large number of studies involving radiation exposure for medical reasons have been described and discussed. Although these studies of medically exposed cohorts have increased our general knowledge of radiation risks, not all of them contribute substantially to quantitative risk assessment. Many studies lack the sample size and high-quality dosimetry that are necessary for precise estimation of risk as a function of dose, a point that is illustrated by the large confidence intervals for many of the risk estimates shown in Tables 7-2 to 7-6 and by the limited number of studies for which risk estimates per gray are available.
Nevertheless, studies of populations exposed to therapeutic and diagnostic radiation provide information on issues that cannot be addressed with atomic bomb survivor data alone. Some examples are the evaluation of risk in Caucasian populations where baseline cancer and other disease risks may be very different from those in a Japanese cohort. Also, studies of medically exposed cohorts allow for the evaluation of risk from protracted exposures. In addition, studies of medical uses of radiation have been important in establishing the lack of radiation risk for CLL, since this cancer is very rare in Japan.
Often there is interest in comparing results from different studies to gain information on the modifying effects of factors such as baseline risks and protraction of exposure that may differ among the studies. It should be kept in mind that such comparisons can be difficult to interpret since there are nearly always several differences among the cohorts being compared. As an illustration, the ERR/Gy for breast cancer in the Life Span Study cohort has been found to be higher than the ERR/Gy in tuberculosis fluoroscopy patients (Howe and McLaughlin 1996; Little and Boice 1999; Preston and others 2002a). However, it is not clear whether this difference occurs because of the higher baseline risks in the Caucasian fluoroscopy cohorts, the lower dose rate in these patients, the lower energy of the X-ray exposure used in fluoroscopy (Brenner 1999), or some combination of these factors.
For lung cancer, the ERR/Gy from the studies of acute, high-dose-rate exposures are statistically compatible and in the range 0.1–0.4 Gy−1. It is difficult to evaluate the effects of age at exposure or of exposure protraction based on these studies because only one study (the hemangioma cohort) is available in which exposure occurred at very young ages and in which protracted low-dose-rate exposures were received. The study of tuberculosis patients appears to indicate that substantial fractionation of exposure leads to a reduction of risk.
For breast cancer, EARs appears to be similar (of the order of 9.9 per 104 PY per gray at age 50) following acute and fractionated exposures to moderate- to high-dose-rate radiation. Effects of attained age and age at exposure are impor-
tant modifiers of risk. The excess risks appear to be higher in populations of women treated for benign breast conditions, suggesting that these women may be at an elevated risk of radiation-induced breast cancer. The hemangioma cohorts showed lower risks, suggesting a possible reduction of risks following protracted low-dose-rate exposures.
For thyroid cancer, all of the studies providing quantitative information about risks are studies of children who received radiotherapy for benign conditions. A combined analysis of data from some of these cohorts with data from the atomic bomb survivors and from two case-control studies of thyroid cancer nested within the International Cervical Cancer Survivor Study and the International Childhood Cancer Survivor Study provides the most comprehensive information about thyroid cancer risks. For subjects exposed below the age of 15, a linear dose-response was seen, with a leveling or decrease in risk at the higher doses used for cancer therapy. The pooled ERR was 7.7 Gy−1 and the EAR 4.4 per 104 PY per gray. Both estimates were significantly affected by age at exposure, with a strong decrease in risk with increasing age at exposure and little apparent risk for exposures after age 20. The ERR appeared to decline over time about 30 years after exposure but was still elevated at 40 years.
Little information on thyroid cancer risk in relation to 131I exposure in childhood was available. Studies of the effects of 131I exposure later in life provide little evidence of an increased risk of thyroid cancer following 131I exposure after childhood.
For leukemia, ERR estimates from studies with average doses ranging from 0.1 to 2 Gy are relatively close, in the range 1.9 to 5 Gy−1, and are statistically compatible. Estimates of EAR are also similar across studies, ranging from 1 to 2.6 per 104 PY per gray. Little information is available on the effects of age at exposure or of exposure protraction.
For stomach cancer, the estimates of ERR/Gy range from negative to 1.3 Gy−1. The confidence intervals are wide and they all overlap, indicating that these estimates are statistically compatible.
Finally, results of two studies of patients having undergone radiotherapy for HD or breast cancer suggest that there may be some risk of cardiovascular morbidity and mortality for very high doses and dose-rate exposures. The magnitude of the radiation risk and the shape of the dose-response curve for these outcomes, if an effect exists, are uncertain.
In conclusion, studies of medically irradiated populations provide information on the magnitude of risk estimates (mainly in the medium- to high-dose range) and on the effects of factors, such as exposure pattern and age at exposure, that may modify risk. Further studies of medically exposed populations are needed to study possible gene-radiation interactions that may render parts of the population more sensitive to radiation-induced health effects. Studies of populations (particularly children and infants) with lower-to medium-dose diagnostic exposures also are needed because of the increasing use of procedures such as CT and radiological monitoring of infants.