2
Selection or Development of an Animal Model
Because bioterrorism includes malicious exposure of populations to a naturally occurring or human-modified infectious agent, such agents are by definition pathogens or toxins. Moreover, the “bio” prefix is appropriate as they are generally bacterial, viral, or fungal pathogens, or toxins of biological origin. The Animal Models for Testing Interventions against Aerosolized Bioterrorism Agents Workshop (held July 6th – 7th, 2005) focused on inhalation of a subset of these agents, the so-called select agents. Experiments designed to identify effective therapeutic countermeasures against an inhaled select agent requires knowledge of the bioaerosol and the experimental animal subjects.
Development or selection of an animal model that approximates the human disease process is dependent on a robust knowledge of the natural history and pathogenesis of the disease (Figure 2-1). However, a single well-characterized animal model may not suffice when testing countermeasures. For example, an animal model that proves predictive of the disease in healthy human adults may not approximate the disease course in immune-deficient adults, children, the elderly, infants, pregnant woman and the progeny they carry, or other susceptible subpopulations. Indeed, no single animal model will exactly replicate responses seen in humans, requiring extrapolation of endpoint-based findings in animals to humans. Accurate extrapolations are possible only when species-specific differences in pathogenesis at the cell, tissue, and organ level; pulmonary anatomy and physiology; and host-pathogen interactions are understood.
There are practical issues that need to be considered when selecting an animal model. Initial countermeasure strategies will likely focus on novel applications of existing, approved pharmaceutical agents. The pharmacodynamics and pharmacokinetics of these agents will have been
previously determined in preclinical studies using a relatively small number of animal models, most typically rodents, rabbits, dogs, and nonhuman primates. It is therefore anticipated that these commonly used species will be among the first to be used for the development of efficacious medical countermeasures for bioterrorism agents. It is also anticipated that nonhuman primates in particular may be extensively used in these studies, given that nonhuman primates are phylogenetically closest to humans (Sibal and Samson 2001). Nonhuman primates are not a homogeneous group, however. The primate order is composed of (1) prosimians (e.g., lemurs, loris, tarsiers) and (2) anthropoidia consisting of New World primates (e.g., marmosets, spider monkeys, cebus) and Old World primates further divided into monkeys (e.g., macaques, baboons) and greater and lesser apes (gorillas, chimpanzees, orangutans, and gibbons, respectively). Overall, there are more than 200 species of nonhuman primates, with approximately 30 species used in research (NRC 1998).
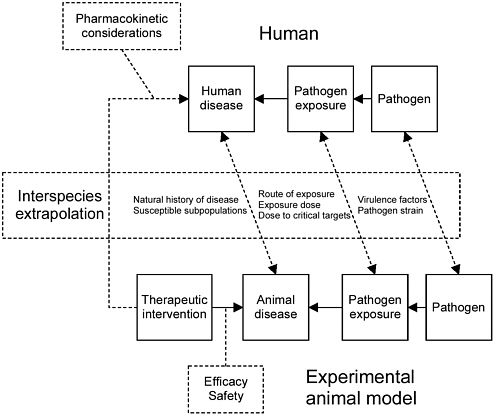
FIGURE 2-1 Factors that need to be considered when selecting an appropriate animal model.
PATHOGENESIS
The pathogenesis of certain human pathogens, including the hepatitis viruses, papillomavirus, polio virus, HIV-1, and measles virus, that can be studied in nonhuman primates cannot be easily studied in mice because mice are not normally susceptible to infection by these viruses. However, the identification and cloning of the human cellular receptors for some of the viruses have made it possible to create transgenic animals that express the corresponding receptors, thus potentially increasing their susceptibility to viral infection. For example, expression of CD46, the major cellular receptor for laboratory strains of measles virus in transgenic rodents, has been used to study immunosuppression caused by this virus (Manchester and Rall 2001).
Certain studies do not require the use of genetically altered rodents. For example, infant botulism—a neurological syndrome caused by the absorption of toxin produced by toxigenic organisms that colonize the intestinal tracts of infants under one year of age—can be replicated and studied in neonatal mice and rats (Moberg and Sugiyama 1980). In any case, reasonable application of well-characterized rodent models may accelerate development of efficacious and safe countermeasures for bioterrorism agents.
HOST-PATHOGEN INTERACTIONS
The selection of the appropriate animal model needs to also consider host-pathogen interactions. Host-pathogen interactions can result in a number of outcomes, including elimination through physical defenses or immune mechanisms, host-mediated or pathogen-mediated damage and disease, development of persistent infections and incapacitation, or death (Casadevall and Pirofski 1999). The ability to identify useful medical countermeasures for the treatment or prevention of disease associated with bioterrorism agents will depend on a robust knowledge of the pathogen and of the host response to that pathogen.
Selection of the appropriate strain of the agent is critical as strain characteristics may influence host responses. Various strains of microbes express different characteristics that can dramatically influence the virulence of the microorganism. In the case of Bacillus anthracis, the main virulence factors are the anthrax toxin proteins that, along with the capsule, are expressed largely under the control of the atxA gene (Abrami and others 2005; Brey 2005). In nonhuman primates, live attenuated pigmentation-deficient strains of Yersinia pestis (plague) are more virulent than is the wild-type strain when delivered as an aerosol (Welkos and others 2002). Disease pathogenesis varies following different routes of exposure. For example, the capsule is required for dissemination of inhaled B. anthraci spores within the host (Brey 2005); dissemination of these spores is enhanced by migration of phagocytic dendritic cells (Brittingham and others 2005).
But while selection of the appropriate strain of a given agent is clearly critical, this factor alone does not ensure clinical relevance. Experience with lentiviruses causing immunodeficiency provides one example where differences in host responses have been especially problematic. Simian immunodeficiency virus (SIV), feline immunodeficiency virus (FIV), and human immunodeficiency virus (HIV) share many molecular and structural features. Each of these viruses targets T cells and reduce CD4+ cell numbers in the host (Levy 1996), and xenoinfections can be produced experimentally. For example, cynomolgus monkeys infected with FIV reportedly develop depletion of CD4+ cells and weight loss (Johnston and others 2001). HIV-1 however, fails to replicate and cause disease except in humans or chimpanzees (Haigwood 2004). Thus the failure of alternative animal models, including other nonhuman primate models, to develop infection following HIV-1 infection has limited development of vaccines and other immunization approaches for this virus (Hu 2005).
Significant differences in host response have also been noted for select bioterrorism agents. For example, several animal models have been investigated for use in testing vaccines protective against anthrax infection, including mice (Welkos and others 1986; Ezzell and others 1984), guinea pigs (Fellows and others 2001; Jones and others 1985), rabbits (Little and others 2004; Fellows and others 2001; Pitt and others 2001), and rhesus and cynomolgus monkeys (Vasconcelos and others 2003; Ivins and others 1998). Of these, macaques are the closest model to humans as they have analogous major-histocompatability complex (MHC) class I and II and cross-reactive IgG (Kennedy and others 1997). Differences in host response to an agent may also be influenced by the strain, age, or sex of the animal. For example, the A/J mouse strain is very susceptible to infection with unencapsulated anthrax strains (Welkos and others 1986)—the animal lacks a functional Hc gene, which encodes for complement component C5. The C5 protein attracts neutrophils and induces the release of histamine from mast cells and basophils. The absence of C5 results in a delay in the influx of macrophages to the site of anthrax infection, thereby allowing the bacteria to overwhelm the host before a suitable immune response can be mounted (Flick-Smith and others 2005).
PULMONARY ANATOMY AND PHYSIOLOGY
The Upper Respiratory Tract
Upper airway anatomy, which differs significantly among species, can influence respiratory tract dosimetry of an inhaled toxin. Humans and certain nonhuman primates such as rhesus monkeys have relatively simple nasal anatomy in regions of the nose lined by the olfactory mucosa (Harkema 1990; Warwick and Williams 1973). In contrast, the rat, which is the animal species most frequently used in conventional toxicology studies, has a highly complex set of ethmoid turbinates (Méry and others 1994), as does the mouse, dog, and rabbit (Figure 2-2). Animal-model selection can be further complicated, as there
are known species differences in the distribution of luminal epithelial-cell populations along the nasal airway, in the intranasal airflow patterns (Morgan and Monticello 1990), and in the function of the nasal mucociliary apparatus (Harkema 1990). Species differences in nasal airway anatomy and airflow patterns influence the relationship between exposure concentration and pathogen dose delivered to either the lung or nasal cavity.
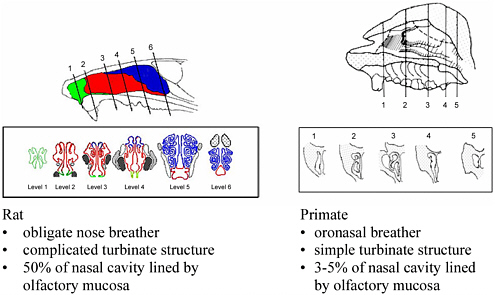
FIGURE 2-2 Nasal anatomy in rats and macaque monkeys and impact on airflow and particle distribution. Nasal epithelial subtypes are shown for the rat.
The size of the inhaled bioaerosol (or therapeutic agent) can also influence the sites at which deposition occurs. Because the nasopharynx compartment is a portal of entry into the lungs, particle removal by the nasal cavity will lower the fraction of the bioaerosol entering more distal airways. Removal of inhaled aerosols by the nasal cavity has been examined using inert particles. Nasal deposition of polystyrene particles with a mass median aerodynamic diameter (MMAD; see glossary) of 1 to 2.8 μm ranged from 12 to 20 percent in children and adults (Becquemin and others 1991), whereas rats have appreciably higher nasal deposition rates for comparably sized particles (Kelly and others 2001). Bacterial bioaerosols are likely to have an effective MMAD on the order of 1-10 μm (Louveau and others 2005; Nicas and others 2005; Jahrling and others 2004; Zaucha and others 2001) resulting in similar deposition patterns as that seen with similarly sized polystyrene particles. Viral bioaerosols; however, will be considerably smaller with aerodynamic diameters ranging from approximately
0.01 to 0.4 μm. Nano-sized particles are more effectively deposited in the nasal cavity (Martonen and others 2003). Differences in nasal anatomy and physiology also influence the delivery of a nasally administered pharmaceutical agent (Ugwoke and others 2001) that may be developed as a bioaerosol countermeasure.
The mode of breathing (e.g., obligate nasal versus oronasal), overall geometry of the nasal passages, relative nasal surface areas, proportions of nasal surfaces lined by various epithelia, mucociliary clearance patterns, and inspiratory airflow routes are also very different between rodents and humans. These anatomical differences may likewise influence the fate of an inhaled bioaerosol and thus influence the response seen in different animal species. This difference in response may become important for inhalation studies with spores or aggregates of bacteria that may deposit within the nasal cavity. Nasal deposition of bioaerosols is considered important diagnostically. For example, the use of nasal swabs in the diagnosis of human inhalational anthrax provides a dramatic example where deposition in the nasal cavity is clinically relevant in humans (Kiratisin and others 2002). In general, the complex structure of the rodent turbinates results in increased nasal deposition when compared with either nonhuman primates or humans.
The Lower Respiratory Tract
Differences in the structure of the lower respiratory tract are also an important consideration when choosing an animal model. There are marked differences in the tracheobronchial airways and lungs of various mammalian species, both at the macroscopic and microscopic levels, that entail not only obvious differences in size but also striking architectural differences. The latter include the branching system of the conducting airways (bronchi and bronchioles), the gross lobation of the lung, the amount of pleura and interlobular connective tissue, and the structural design of the pulmonary centriacinus, where the most distal conducting airways intersect with the alveolar gas-exchange regions in the lung parenchyma (Tyler 1983).
For example, the left human lung has two connected lobes, the superior and inferior, while the right lung is composed of three connected (fused) lobes— superior, middle, and inferior. In contrast, the right lungs of laboratory mammals, including nonhuman primates, are divided into four distinctly separated lung lobes (cranial, middle, caudal, and accessory). The left lung lobes of nonhuman primates are divided into cranial (with cranial and caudal portions) and caudal lobes, but rats and mice have a single undivided left-lung lobe. In humans, the visceral pleura on the outer surface of the lung lobes are thick and the interlobular connective tissue within the lobes is extensive. But in laboratory mammals, including nonhuman primates and rodents, the pleura of the lung are thin and the extent of interlobular connective tissue, if any, is modest.
In the human lower respiratory tract, there are seven generations of bronchi, and the tracheobronchial branching system is relatively symmetrical (i.e., the
parent airway divides into two smaller airways with relatively equal diameter). This is in contrast to most laboratory mammals, such as monkeys, dogs, rats, and mice, which have monopodial branching patterns consisting of daughter branches of unequal diameter (Tyler 1983). The lower respiratory tract in humans also contains several generations of nonrespiratory bronchioles— conducting airways without alveolarized outpockets (Weibel 1963). Considerably fewer generations of these small conducting airways are present in other mammalian species, including monkeys.
The most distal nonrespiratory bronchiole is defined as the terminal bronchiole, which connects to the aveolarized (respiratory) airways. This focal area where conducting and respiratory airways join is called the centriacinus, a common site of injury caused by inhaled toxic gases and particles. In humans and some laboratory mammals (e.g., monkey, dog), the terminal bronchioles end into several generations of respiratory bronchioles that are defined as bronchioles with a few, widely scattered, intramural alveoli (or alveolar outpocketings). This is in contrast to several small laboratory mammals (e.g., rat, mouse), whose terminal bronchioles end directly into one short segment of respiratory bronchiole or into airways with walls completely covered by alveoli (i.e., alveolar ducts) (Tyler 1983).
Though similar epithelial-cell types (e.g., ciliated, mucous, basal) line the tracheobronchial airways of mammalian species, the percentage of these cell types may differ markedly among these species and needs to be taken into consideration when selecting an animal model for inhalation studies. A striking example is the percentage of mucous goblet cells that are found in surface epithelium lining the tracheobronchial airways of human and nonhuman primates compared to the corresponding fraction in laboratory rodents (mice and rats). The primary secretory cell in the tracheobronchial airways of humans and nonhuman primates is the mucous goblet cell, while in similar airways of laboratory rodents the percentage of these secretory cells is very low compared to that of other secretory cell types (i.e., serous and Clara cells). In addition, while submucosal glands, which also contribute mucus to the airway luminal surface, are found throughout the tracheobronchial airways of humans and nonhuman primates, these glands are restricted to a very small portion of the proximal trachea in laboratory mice and rats. The density of mucus-secreting cells in either the surface epithelium or submucosal glands of the tracheobronchial airways will determine the amount and character of the airway lining fluid which serves as a critical protective interface protecting the airway epithelial cells from potential injury (or infection) from inhaled infectious agents.
Aerosol Deposition in the Respiratory Tract
Although it is clear that each species has its unique respiratory tract structure, an important consideration in selecting an appropriate animal model is how that structure affects particle (bioaerosol) deposition. Raabe and others
(1988; 1977) published aerosol-deposition data in common laboratory animals and in particular on the regional deposition of monodisperse particles (1-10 μm diameter) in Fischer 344 rats, Hartley guinea pigs, and New Zealand rabbits. The data describe deposition in several regions, including the naso-pharynx, larynx, trachea, bronchi, and pulmonary structures. A conclusion was that deep-lung deposition of particles with diameters greater than 3 μm is negligible, but that upper airway deposition is significant.
Schlesinger (1985) reviewed aerosol-deposition efficiencies in the human (for oral and nasal breathing), hamster, monkey, dog, guinea pig, rat, and mouse. The data for tracheobronchial deposition show relatively low, constant deposition in the experimental animals in the particle-size range of 0.1 to 5.0 μm. Alveolar deposition reached a peak at about 1 μm in experimental animals, but at about 2-4 μm in humans. Despite these differences, Schlesinger concluded that “the relationship between particle size and total respiratory tract deposition is quite similar in humans and most of the experimental animals presented.” This conclusion must be qualified since total deposition may not reflect regional dose at the target site of interest.
Other dosimetrics can be considered. The ideal dose metric would need to be mechanistically associated with or closely correlated to the biological response. Internal dose may be accurately described by particle deposition alone if the toxin exerts its primary action on the epithelial surface tissues. Jarabek and others (2005; 1995) reviewed particle dosimetric adjustments for interspecies extrapolations, which underscore the importance of using quantitative morphometric measurements as input for mechanistic computer codes that calculate regional particle deposition. One important conclusion was that the magnitude of species differences in particle deposition depends strongly on whether the deposition is calculated for an entire anatomical region (e.g., tracheobronchial tree) or normalized to deposition per unit surface area. Use of dose metrics based on the number of retained particles and normalized to the number of alveoli, macrophages, or ventilatory units all gave rise to lower human equivalent concentrations than those based on the current default of particle mass normalized to pulmonary surface area.
Wolff (1996) reviewed differences in particle deposition and clearance measurements in several species, including humans, dogs, monkeys, and rats. A striking finding was that there were significant differences in pulmonary deposition of particles in the 0.1 to 10 μm diameter range; mouth breathing in humans led to large pulmonary deposition, and both dogs and monkeys had pulmonary depositions similar to that of nose-breathing humans. In addition to differences across species, strain differences may be important. Significant particle deposition efficiencies have been predicted for BALB/C mice versus B6C3F1 mice from respiratory tract morphometric measurements (Oldham and Phalen 2002).
Clearly, species differences in airway anatomy need to be considered when extrapolating results from animals to humans. Moreover, diverse responses may
arise when toxins are deposited in different airway regions (e.g., nose vs trachea or alveoli). Thus, local tissue dose needs to be considered, since similar total respiratory deposition among species does not necessarily lead to similar responses. Several tools are available to estimate the delivered dose to the airways of animals and humans (NCRP 1997; ICRP 1995). Computational models can also predict deposition and retention of particles in the lung (Brown and others 2005; Subramaniam and others 2003).
Clearance from the Respiratory Tract
Immunological or physical clearance of an agent from the lung may also be species-dependent. An inhaled pathogen or particle may become inactive or be cleared from the lung by either physical means (e.g., mucociliary clearance) or via immunological mechanisms (e.g., alveolar macrophage phagocytosis and subsequent removal). The physical processes demonstrate important species differences (Stober and McClellan 1997). The difference in rates of clearance of insoluble particles inhaled by several laboratory animals has been reviewed by Wolff (1996), who noted that rats and mice, in contrast to humans and dogs, exhibit an early and prolonged rapid clearance of material deposited in the alveolar region. This rapid clearance serves to protect the distal lung from particle accumulation, unless the particle load is great enough to overwhelm the physical-clearance mechanisms.
In addition to being influenced by mucociliary clearance, removal of a bacterial pathogen from the normal lung reflects the relative rates at which in-vivo bacterial multiplication and killing will occur. Marked differences in lung clearance have been reported with different strains of pathogens and may also be influenced by the strain of the animal model used (Jay and others 1976), as well as the immune status of the animal.
From the foregoing information, it is clear that pulmonary anatomy and physiology, at both the macroscopic and microscopic levels, differs significantly among species. This can influence the deposition of the inhaled agent within the lung, as well as the tissues and cells that will primarily be contacted by the agent. All of these differences can affect disease outcomes and whether the animal model will be predictive of the human response to a countermeasure.
It is clear that for the species and strains to be used in countermeasure research, additional information is needed on differences in respiratory-tract anatomy and particle deposition. The Committee recommends that additional data on experimental-animal airway anatomy and particle deposition and clearance be acquired to aid in developing new animal models and performing extrapolations to human populations. In particular, data are needed for various strains of mice and many species of nonhuman primates.