4
Drilling and Sampling Technologies and the Potential for Contamination
TECHNOLOGIES TO ACCESS SUBGLACIAL AQUATIC ENVIRONMENTS
A key issue in the exploration of subglacial aquatic environments is how to recover data and samples that are free of artifacts or contamination without irreversibly altering the environment under study. Current ice penetration and drilling technologies to reach the aquatic subglacial realm have been developed for projects to recover ice cores mainly for paleoclimate research or to most efficiently reach the subglacial bed for direct glaciological observation using fast access ice sheet drilling. Fast access ice sheet drilling generally proceeds without the recovery of a continuous ice core, and, in most cases, is used to reach scientific targets deep within or below the ice surface. This approach may be useful for the exploration of subglacial aquatic environments if drilling platforms such as (1) wire-line drilling, (2) hot-water drilling, (3) coiled-tube drilling, and (4) hybrid systems can be adapted for the purpose of fast ice sheet drilling. Other technologies, such as ice corers and thermo- or melt probes may also be adaptable for subglacial aquatic environments (SCAR SALE 2006). At present, each of these techniques has its own advantages and disadvantages, but a common technological challenge for all deep ice holes is to stabilize the hole against the lithostatic pressure within the borehole to prevent the hole from closing. Currently, this is accomplished by filling the holes with a fluid that will not freeze whose density is similar to the ice. The fluid acts to counterbalance the overburden pressure keeping the hole open.
Ice coring is an established method using standard mining and oil industry technology to reach the base of many ice sheets (SCAR SALE 2006); however the main objective of ice coring is to recover ice cores, not simply to drill a hole. Holes are generally left after an ice core has been extracted, and they vary in diameter between roughly 10 and 20 cm depending on the drilling equipment used. All deep ice core drills are electromechanical devices that recover ice cores and drill cuttings with each run. The drawbacks to using this technique are that usually two to four field seasons are required to drill to the bed and this technology requires the use of an antifreeze drilling fluid. Consequently, the use of existing coring systems to access subglacial aquatic environments may be limited. However, the borehole may
be used for sample retrieval from a subglacial lake if certain precautions are taken to ensure that the drilling fluids do not enter the subglacial aquatic environments.1
Hot-Water Drills
Using hot water to melt holes into the ice is a well-established technique that has been used by several countries such as the United States (AMANDA, ICECUBE, Caltech), Australia, Germany, and the United Kingdom. Hot-water drills operate by pumping hot water from a heater through a hose under high pressure to a drill head, which melts the ice. This technique generates a hole filled with water, which is pumped to the ice surface where it is reheated and pumped back into the hole. The drawbacks of this technique are that holes are open for only a few hours because the water refreezes, the hole closes, and no cores are recovered.
Keeping holes open for more than a few hours necessitates constant reaming and input of heat, which requires significant amounts of fuel. Ideally, such holes could be considered clean because the water used to melt the holes comes from the melted ice itself and is constantly recirculated through the heaters and high-pressure pumps. However, this circuit through machinery is also a possible source of contamination. Soot or materials dissolved from the walls of the high-pressure hose may contaminate the waters that are pumped back into the hole.2 These potential contaminants could be removed by introducing nanotechnology filters in the steam path and through the high-pressure (e.g., 8-10 MPa) steam itself. It is even conceivable to introduce a full autoclave system into the water circuit. Although this technique has been used to quickly drill several kilometers of ice and may be able to access some subglacial aquatic environments, more method development will be needed for this technique to achieve the ice depths that are required to reach many subglacial aquatic environments located beneath thicker ice.
Melt Probes
Melt or thermo-probes represent another technology that shows promise to access subglacial aquatic environments. It should be noted however, that to date, the melt probe concept has not yet achieved this. Originally designed by Philbert & Philbert as a system to remotely measure temperature within the Greenland ice sheet, melt probes have so far reached a maximum depth of about 1600 m. The probes have a heated element at the tip, which melts ice as it descends. The water refreezes behind the probe, which is tethered to the surface via cable. Such melt probes could conceivably reach subglacial aquatic environments in a sterile fashion. Once in position, these probes could collect remote measurements and provide data communication with the surface.
At present, there is no technology available for retrieval of such melt probes, which precludes using this equipment for sample recovery. The major disadvantage of using melt probes is that they cannot effectively penetrate ice with particulates and may stop or be diverted from the preferred course. As the borehole deepens, these impurities are concentrated within the borehole because there is no way to circulate the fluids away from the probe (SCAR SALE 2006).
Fast Access Drilling
There are options, as yet untried, for rapid access drilling to the bed using technology from oil drilling industries, such as coiled-tube drilling or even wire-line drilling using conventional drill rigs. These technologies, however, are not presently capable of penetrating ice sheets to greater depths and are not suitable for the conditions likely to be encountered during drilling subglacial aquatic environments. These technologies need to be adapted before they can successfully be used to access subglacial aquatic environments. Development of a new ice sheet drilling platform that will provide access to deep samples of ice and subglacial materials and enable fast completion of arrays of boreholes distributed over large areas (tens and hundreds of kilometers in one season) was the focus of a workshop sponsored by the National Science Foundation.3 The FASTDRILL workshop identified four potential drilling platforms, which may be adapted for the purpose of fast ice sheet drilling.
Wire-Line Drilling
Wire-line drilling is a standard mining and oil industry technology already widely used in cold climates with many relatively inexpensive and standard accessories available for deployment in support of polar research. This technique is currently being used in Antarctica in the Antarctic Geological Drilling (ANDRILL) project. Although this system is limited to ~2.5 km in its current configuration, it may be useful for relatively shallow subglacial aquatic environments. To reach deeper environments, such as Lake Vostok, the maximum drilling depth would have to be increased. In addition, further analysis is necessary to evaluate whether the weight and size of such drills will preclude their deployment with aircraft (LC-130) and prevent moving this system in the field.
Hot-Water Drilling
Hot-water core drilling is another fast drilling technique that can also recover cores. In the past it has been the primary fast access technology in ice-sheet environments mainly to depths <1.5 km (Engelhardt et al., 2000) and is the most established technology for making access boreholes to the bottom of ice sheets. The drill system consists of small hot-water jets contained in an annulus that melts the ice around a core and can be used in conjunction with coreless hot-water drilling. This system has minimal infrastructure and would be quite mobile, which may permit several sites to be sampled in a season. However, in its current configuration, this technique has
3 |
FASTDRILL: Interdisciplinary Polar Research Based on Fast Ice-Sheet Drilling. Report of an NSF-Sponsored Workshop Held at University of California, Santa Cruz, October 23-25, 2002. A PDF version of the report can be found at http://es.ucsc.edu/%7Etulaczyk/Report_I_V.pdf. |
serious limitations for the exploration of subglacial aquatic environments. A lack of control of borehole dimension and geometry, difficulty in obtaining subglacial cores, and the lack of continuous coring capability are major disadvantages. In addition, the lack of casing makes is difficult to maintain a sterile environment around the core and the hot water may cause thermal shock to the outer part of the core if it is recovered. Moreover, power requirements for hot-water drilling of access boreholes through deep ice (2-4 km) are very high.
Coiled Tubing Technology
Coiled-tubing technology is now a fairly mature technology used by the oil and gas industry, especially in the Arctic. This technology can be used as a more capable version of a hot-water drill, with greater control during drilling and flexibility in deploying different downhole tools. Although it is commercially available, its suitability for FASTDRILL applications needs to be evaluated in polar ice sheet drilling.
Hybrid Systems
It appears that at the present time no single technology exists that provides a means to access subglacial aquatic environments in a clean manner. It may be possible, however, to combine different drilling technologies to reach this goal. For example, it may be possible to use coiled tubing with a hot-water tip for melting through ice and then switch to wire-line technology for recovery of long cores. Development of a hybrid drill, combining some characteristics of two or three basic drilling technologies, may also provide a way forward.
Coiled-Tube Drilling for Ice
Recent technological advances in coiled-tube drilling by the commercial oil and gas industry may lead to the development of a system capable of rapidly drilling through 3-4 km of ice in several days. This proposed system, coiled-tube drilling for ice (CTDI) uses a metal or advanced-composite tube to deliver fluid to a hydraulic motor that drives a cutting bit within the borehole. An advantage of this system is that it combines the best aspects of mechanical drilling with the speed of coiled-tube drilling. Ice chips generated by drilling are removed as the drilling fluid is pumped through a continuous hose. This technique can provide continuous drilling, unless ice cores are desired.
Expectations are that this technique will permit drilling rates as fast as 40 m hr−1 in polar ice, which would mean that this system would be capable of drilling through 3-4 km of ice in six to eight days, including setup time. The system would be aircraft (LC-130) transportable and sled mounted for rapid mobilization and demobilization. The drill speed and portability of this system would enable an array of deep boreholes to be drilled in a single season. Tubing sizes used to drill a 54-mm borehole can be as small as 25-mm inside diameter, thereby reducing the volume of borehole fluid. This is a clear advantage in case of a blow-out, where only minimal fluid will be ejected. The drill system would be able to produce semipermanent uniform-diameter holes with minimal thermal disturbance and be capable of acquiring rock cores, frozen sediment cores, and short ice cores. The modular and flexible design proposed for this system would facilitate the addition of new tools to satisfy future research needs. The
capabilities of the CTDI would fill the void between existing deep ice core drills and hot-water drills.
The disadvantages are that the small-diameter boreholes make instrument deployment difficult. The drill would be mobile to some extent; however, it should be noted, that to reach a depth of 3800 m, the drill configuration would weigh tens of metric tons.
Additional Considerations
Regardless of drilling technology, the problem of how to penetrate and sample subglacial aquatic environments without the introduction of borehole stabilizing fluids into these environments remains a challenge. The decisive factor is the pressure difference between the inside and the outside of the boreholes. Initially the obvious choice is to work with an underpressured hole leading to a rise of subglacial water inside the borehole for sampling. Due to the prevailing temperature gradient, this will lead to refreezing of the water rising into the borehole. If the water is to be sampled as a liquid this must be done very quickly, conceivably in heated containers, and transported to the surface. Alternatively, the frozen water may be sampled by additional drilling. This approach has been proposed to recover samples from Lake Vostok (Box 1.1). In all cases where water was encountered at the bed (i.e., Byrd and the European Project for Ice Coring at Dronning Maud Land [EPICA DML]4 boreholes; the North Greenland Ice Core Project [NGRIP] borehole), the underbalanced hole option worked to keep the fluids in the hole, but the redrilled samples of refrozen subglacial water from NGRIP were found to be contaminated with drilling fluid (J. Priscu and S. Bulat, personal communication). Redrilling of the EPICA DML hole has yet to occur.
Another approach to reach subglacial aquatic environments is to use conventional electromechanical ice core drilling techniques to reach an environmentally safe depth above the top of a lake. This safe distance would preclude the possibility that microfractures in the ice would allow the borehole fluid to migrate along an intergranular vein network and enter the lake. Once the appropriate depth is reached, then other equipment and technology specifically designed for this operation would be employed. This approach was used by the Russian-led consortium to position the borehole above Lake Vostok in anticipation of entering the lake during the International Polar Year (IPY, 2007-2009).5 The safe distance for Lake Vostok was calculated to be 30 meters above the lake surface (Russian Federation 2006).
This value was determined by considering the conditions that favor ice fracture—large stress differences and low confining pressure—and the permeability of ice with respect to the drilling fluid. Large fractures, or crevasses, form where stress differences are large and confining pressures are small; however, over subglacial lakes, stress differences are expected to be small and the confining pressure is extremely large due to the 4 km of overlying ice. The deviatoric stresses are believed to be small because the 220 m of accreted (lake) ice at Vostok forms an ice massif blocked against the eastern edge of the lake and overridden by the moving glacier. A shear layer (likely active) is found above the interface (around 3540 m depth) between the glacial and accreted ice.
This suggests that fracture processes are suppressed or inhibited in the deepest section of the accreted ice (to be drilled).
The latter conclusion is fully supported by the high crystallographic structure of the ice crystals, as revealed by X-ray diffraction measurements (Montagnat et al. 2001), which is possible only if the lake ice does not plastically deform under the in situ conditions. Hence, the formation of open crevasses often observed in grounded ice can hardly be expected in the case of basal ice at Vostok (Russian Federation 2006).
The permeability of the accreted ice to the drilling fluid is expected to be low, because the accreted ice crystals show a lack of distortion, which essentially rules out a significant diffusion of the drilling fluid through the ice lattice (Montagnat et al. 2001). However the presence of liquid water along the grain boundaries provides a route for liquid transport. The veins form a continuous network of microscopic channels that remain liquid at subfreezing temperatures. Hence, contamination from the drilling fluid may be associated with both the downward advection of intercrystalline water and the diffusion of the drilling fluid through the vein system. Estimates of the total advection of the drilling fluid from the borehole toward the ice-water interface will be about 1 m over millennial time scales (Russian Federation 2006). In addition, helium data (Jean-Baptiste et al. 2001) support the presence of an upward component of velocity in the accretion ice layer of about 6 mm per year, which can be the result of hydrostatic compensation in this region (Souchez et al. 2004). This upward movement of ice, if present, would overcompensate a very slow downward advection of the fluid (Russian Federation 2006).
NEEDS FOR TECHNOLOGY DEVELOPMENTS
Some technologies currently exist that can be used immediately in the exploration of subglacial aquatic environments. These include airborne radar, magnetic, andgravity surveys, land-based seismic surveys; and certain operational sensors that could be deployed within lakes exist for some of the more fundamental properties. Other technologies however, will require development. For example, experimental sensor arrays need to be developed for the detection of dissolved gases such as H2S, CH4, N2O, N2, and argon, as well as major anions and cations and bioreactive redox couples such as ammonium and dissolved manganese. Currently available sensors will also need to be field-tested for compatibility with the expected temperature and pressure conditions of subglacial lake environments and environmental restrictions.
The participants of SCAR SALE (2006) stressed that the size of the sensor packages may not be suitable for the size of lake access holes and that limitations on the borehole size may require the miniaturization of existing technologies. It is conceivable that at least some of the subglacial aquatic environments may be substantially overpressure. Further technological developments, such as introducing pressure-tight locks in the bottom parts of a borehole may eventually be advisable to deal with this possibility as well as to sample subglacial lake bottom sediments.
Sample recovery poses its own set of challenges. Many of the standard oceanographic techniques for remote collection using water sampling bottles (e.g., Rosette Samplers with Niskin bottles) and sediment retrieval by coring devices (piston corers, gravity corers, box corers, grab samplers, etc.) use very large devices that are too large to fit through a drill hole; specialized sampling devices and techniques may have to be developed (SCAR SALE 2006). Sediment trap technologies and other water particu-
late collection devices are adaptable to subglacial environments. Another suggestion posed by SCAR SALE (2006) is the use of in situ, large-volume filtering devices to concentrate particulates for analysis in a clean surface laboratory following retrieval of the sampling device.
Geological drilling technologies remain to be fully developed; however, the current ANDRILL Program is establishing technology and techniques (a 2000-m-long drill string through up to 300-m thickness of floating ice on the sea) that are moving closer to what is required for subglacial lake sediment recovery. Existing ice drilling techniques appear to be capable of meeting the field requirements for penetration of 4 km or more of ice, penetrating low-temperature (subzero) and high-pressure environments.
The challenge for ice drilling is demonstration of the ability to do so with minimal and/or acceptable levels of contamination. Techniques are needed to ensure the purity of the drilling water fluid to avoid contamination from surface microbes. There may be solutions to this requirement in methods currently proposed to sterilize (ultraviolet radiation, ozonolysis) ballast waters in ships that prevent the introduction of non-indigenous species.
Future technologies required for the exploration and sampling of subglacial lakes (focusing on Lake Vostok) were the topic of a workshop funded by NSF (Rock and Bratina 2004).6 The main goal of this workshop was to identify the technological challenges to sampling and exploring the waters and sediments of Lake Vostok and to generate recommendations to overcome these barriers. A number of findings and recommendations were developed and are summarized in Table 4.1. The workshop found no significant technological barriers to simple entry, exploration, and sampling of the lake in the region of the borehole. Interestingly, one of the major findings and recommendations was that required cleanliness levels needed to be set in order for the technology development to advance.
Collecting samples and data away from the region of the borehole incrementally increases the technical challenge. This type of research will require the use of robotic vehicles that are either tethered (remotely operated vehicles—ROVs) or not (autonomous underwater vehicles—AUVs). One of the biggest barriers to performing research in the subglacial environment using robotic vehicles will be designing the vehicles to be able to fit down a small-diameter borehole. The workshop (Rock and Bratina 2004) considered the possibility of remote assembly of smaller vehicle parts (e.g., below the ice) but concluded that this would be prohibitively challenging.
There is, however, a large and growing body of expertise in performing surveys with ROVs and AUVs in marine environments that can be drawn on for dealing with issues such as vehicle design, navigation, communication, and power. Enabling these vehicles to collect samples far from the borehole was deemed a significant challenge and beyond current technology. The workshop recommendations (Rock and Bratina 2004) encouraged the subglacial research community (through NSF) to draw on the expertise in the ocean science community to overcome these challenges and said that NSF should encourage the development of new and creative approaches to the exploration of subglacial aquatic environments.
6 |
Final Report from the NSF sponsored workshop Lake Vostok: Defining a Technology Roadmap for Exploration and Sampling, March 27-29, 2003, Palo Alto, California. A PDF version of the report can be found at http://salegos-scar.montana.edu/docs/Workshopdocs/Vostok_Technology_Workshop_Report.pdf. |
TABLE 4.1 Findings and Recommendations from Rock and Bratina (2004)
Findings |
Recommendations |
1. No fundamental engineering barriers exist that would prohibit entry of a (simple) probe into the lake (although significant engineering development might be required) |
1. NSF should task a group to develop these specifications immediately (e.g., an American Society for Microbiology board or committee) |
2. Quantifiable specifications that define acceptable levels of contamination do not currently exist and must be generated before detailed engineering efforts can proceed |
2. All early missions that penetrate the ice should include an engineering feed forward component designed to collect relevant data |
3. Critical data defining the operational environment for instruments (and vehicles) deployed within Lake Vostok are lacking |
3. NSF should team with the ocean science community to exploit existing capabilities (e.g., vehicle and sensor technology) wherever possible |
4. Significant development will be required to develop an underwater vehicle to deploy within the lake (e.g., through a small-diameter borehole) |
4. NSF should team with the ocean science community to develop the capability to perform sample collection using AUVs |
5. Significant research and development is required to create the technology required to collect samples at locations remote from a borehole (e.g., the grounding zones) |
5. NSF should encourage new and creative approaches to the broad range of technological challenges associated with the exploration of Lake Vostok. Questions include: Are there creative or different ways to access the lake? Are there novel ways to provide long-term power to equipment in the lake? Are there new techniques to assemble a sensing system within the ice or within the lake? |
The future technology workshop participants found that another impediment to the planning and design of technologies for subglacial exploration is the lack of knowledge about the aquatic environments (e.g., how corrosive is the water?). As a result, some technologies for more advanced stages of the research may not be able to be developed reliably until after first entry and preliminary data collection occur.
POTENTIAL FOR CHEMICAL CONTAMINATION
Chemical contaminants could enter a subglacial lake during exploration as liquid constituents of the drilling fluid and as solutes or particulates in that fluid. Direct chemical contamination could also occur from the drilling and sampling apparatus, for example, from water-soluble oils used in metal working during the fabrication of the instruments and equipment and from phthalate ester additives that are used extensively in the plastics industry. The nature and magnitude of chemical contamination from each of these sources will be highly specific to the type of drilling and sampling operation.
Drilling Fluids
Drilling fluids have varied in the past. At present there are basically two types of drilling fluids. One fluid is kerosene based (Shell Exxol D40/D60) with an appropriate amount of hydrochlorofluorocarbons (HCFCs) added to reach a density of 900 to 920 kg m−3; the other is n-butyl acetate. Both types of drillings fluids have served their purpose well, but the Montreal Protocol and certain health hazards with the n-butyl acetate lead to a continued search for more environmentally benign substances. These substances however must retain low viscosity at the lowest temperatures encountered. Several new drilling fluids have been proposed in the past several years. The latest developments in this sector are tests of fatty acid esters such as ESTISOL 240 by Danish drillers in Greenland (J. P. Steffenson, personal communication) and non-toxic, non-aromatic paraffin hydrocarbons including Isopar-K by U.S. drillers in Antarctica (WAIS Divide Ice Core Project). Thorough testing of these fluids still remains to be performed to fully characterize the temperature dependence of miscibility behavior (for multi-component fluids), viscosity, and the nature of fluid/chip interactions under an appropriate range of temperatures and pressures.
Regardless of which drilling fluid is used, none is sterile when purchased. This is especially true with kerosene-based fluids because there are a number of organisms live in this type of drilling fluid. To help combat potential microbial contamination, bactericides (e.g., BHT—butylated hydroxytoluene) have been added to drilling fluids. The drilling fluid proposed for the West Antarctic Ice Sheet (WAIS) Divide project is ISOPAR-K with a HCFC 141b densifier. This fluid has 3 parts per million (ppm) of BHT added to Isopar liquids by the manufacturer (J. Priscu, personal communication).
Chemically, kerosene-based drilling fluids, such as those used in the Vostok 5G site and EPICA (Shell Exxol D40), are heterogeneous mixtures of mostly alkanes; however, other kerosene-based drilling fluids also contain benzene, toluene, xylene, alkylbenzenes, and various polycyclic aromatic hydrocarbons (PAHs) including naphthalene and alkylnaphthalenes (Irwin et al. 1997). Alkyl PAHs are toxic and constitute a high percentage of the total PAHs in kerosene; for example, these compounds constitute 97.6 percent of the PAH concentrations detected in fuel-contaminated sediments (Irwin et al. 1997). Other drilling fluids include n-butyl acetate, as used at the Dome Fuji site in Antarctica and on the Greenland ice sheet at the GISP2 borehole (Table 4.2).
Contamination from kerosene is typically measured by gas chromatography-mass spectrometry (GC-MS), and each fluid will have its own distinctive fingerprint. Considerable attention has been given to decontaminating Vostok ice cores from kerosene and, by association, any organisms associated with the drilling fluid.
A careful of analysis of ice core decontamination protocols by Christner et al. (2005a) included GC-MS analysis of the kerosene content of successive layers of ice core. Hydrocarbon-derived ions with a mass between 70.7 and 71.7 were quantified relative to ion fragments from an internal standard and converted to kerosene values from a standard dilution curve. The outer layers of the cores had high kerosene concentrations, while the inner core was not significantly different from blanks (Figure 4.1).
Presumably much lower limits of detection could be achieved by using larger sample volumes or by targeting specific constituents with more sensitive analytical methods. For example, the lowest amount of benzene that can be detected by using a GC-photoionization detector method (0.2 μg L−1) is two orders of magnitude (100 times) lower than the concentration that can be detected by GC-MS (20 μg L−1), (see the following
TABLE 4.2 List of the Deepest Bore-Holes in Ice with Drilling Fluids Used
Depth (m) |
Fluid |
Notes |
1391 |
Aqueous ethylene glycol solution; Fuel DF-A + trichlorethylene |
|
2164 |
Aqueous ethylene glycol solution; Fuel DF-A + trichlorethylene |
Drill was stuck in the year during resumption of the drilling |
812 |
Aqueous ethanol solution |
Hole was plugged by ice chips |
2037 |
Fuel Jet A-1 + perchlorethylene |
Drill was stuck |
2202 |
Fuel TS-1 + CFC 11 |
Drill was stuck |
2546 |
Fuel TS-1 + CFC 11 |
Drill was stuck |
3029 |
Solvent D60 + CFC 113 |
|
3053 |
n-butyl acetate |
Kevlar cable was damaged |
1196 |
Fuel Jet A-1 + perchlorethylene |
|
554 |
n-butyl acetate |
|
1371 |
Solvent D60 + HCFC 123 Shell Exxol D60 + HCFC 141b |
The drill was stuck, not recovered |
3086 |
Shell Exxol D60 + HCFC 141b Small amounts of aqueous ethanol solution in deepest 100 m |
Subglacial water rose in borehole at completion |
2500 |
n-butyl acetate |
The drill was stuck, not recovered |
3623 |
Fuel TS-1 + CFC 11; Fuel TS-1 + HCFC 141b |
|
1003 |
n-butyl acetate |
|
723 |
Fuel TS-1 + HCFC 141b |
|
3270 |
Shell Exxol D60 + HCFC 141b Insignificant amount of aqueous ethanol water solution |
First drill stuck in 781 m, not recovered |
2774 |
Shell Exxol D40 + HCFC 141b Insignificant amount of aqueous ethanol water solution |
Subglacial water rose in borehole at completion approx. 160 m |
948 |
Shell Exxol D60 + HCFC 141b |
|
3028 |
n-butyl acetate |
|
? |
Shell Exxol D60 + HCFC 141b |
|
? |
ISOPAR-K + HCFC 141b densifier |
|
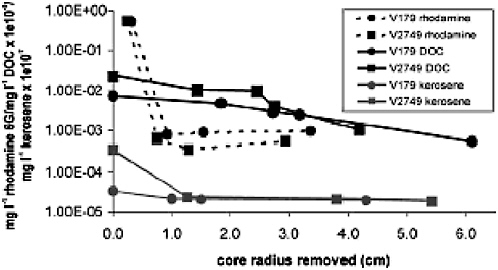
FIGURE. 4.1 Decontamination of Vostok accretion ice cores as measured by reduction in an added fluorescent tracer (rhodamine), dissolved organic carbon concentrations, and kerosene concentrations. SOURCE: Reprinted from Christner, B. C., Mikucki, J. A., Foreman, C. M., Denson, J. and Priscu, J. C., 2005a, Glacial ice cores: a model system for developing extraterrestrial decontamination protocols, Icarus 174:572-584. Copyright (2005), with permission from Elsevier.
web site for analytical methods and detection limits for this and other pollutants: http://www.pacode.com/secure/data/025/chapter16/s16.102.html). Total organic carbon measurements could also provide a guide to contamination above baseline levels, with a detection limit of 10 μg L−1 (Christner et al. 2006).
Miscible Fluids and Solutes
The drilling fluid may contain various additives and tracer recoveries (e. g., rhodamine as in Figure 4.1). For example, Chlorofluorocarbon-11 (CFC-11) has been added as a densifier at the Vostok site. CFCs have been detected as contaminants in McMurdo Dry Valley lakes at concentrations up to 3.5 ng L−1 (Tyler et al. 1998). The detection limits reported in that study using a purge-and-trap gas chromatography system were 5 pg kg−1 for CFC-11 and CFC-12 and 10 pg kg−1 for CFC-113.
Additional contaminants may be dissolved in the fluids, including persistent organic compounds such as polychlorinated biphenyls (PCBs). The limit of detection for most congeners, for example, in groundwater is 0.1-0.5 μg L−1 (ppb) using U.S. Environmental Protection Agency (EPA) Method 608/SW-846 Method 8082.
Persistent fluorinated contaminants such as long-chain perfluorinated acids and heptadecafluorooctane sulfonamide (FOSA) are a new class of pollutants associated with chemical manufacturing of some products, and their appearance has now been detected throughout the world including polar bears in the Arctic (Martin et al. 2004). These compounds can be detected in picogram quantities by liquid chromatography-mass spectrometry (LC-MS).
Chemical Contaminants from the Drilling and Sampling Equipment
Chemical contaminants from drilling and sampling equipment will depend on the composition of the equipment, its fabrication history, and cleaning protocols prior to use. Machine oils (cutting fluids) are routinely used in metal working to cool the cutting tools and to wash away the metal filings (http://www.mfg.mtu.edu/testbeds/cfest/ fluid.html#cfintro_name). They may be mixed with lubricants and typically contain many additives including hydroxyalkylamines as emulsifiers, chlorinated paraffins to control viscosity, corrosion inhibitors such as sodium nitrite, and biocides to prevent microbial growth. The addition of nitrites can result in the production of toxic N-nitroso compounds (nitrosamines) such as nitrosodiethanolamine (NDELA), which can occur in high concentration in water-based cutting fluids. The detection limit for NDELA and other nitrosamines in aqueous systems is 20 pg by GC-MS (Wigfield et al. 1988).
Phthalate esters are widely used as plasticizers in polyvinyl chloride (PVC), polyvinyl acetates, cellulosics, and polyurethanes. Their solubility in water varies by orders of magnitude depending on the length of their alkyl chain. A large collection of literature exists on their distribution and bioaccumulation throughout the environment. Limits of detection vary with the method applied and can be less than 50 ng L−1 in water using solid phase extraction (Staples 2003).
Rubber hosing has the potential to release a variety of contaminants. For example, styrene, used in the synthetic rubber industry and in the production of other polymers, is required to be monitored in U.S. drinking water supplies if levels exceed 500 ng L−1 (http://www.freedrinkingwater.com/water-contamination/styrene-contaminants-removal-water.htm).
POTENTIAL SOURCES OF BIOLOGICAL CONTAMINATION
Any form of invasive sampling of a subglacial lake will result in some level of perturbation to the environment in question. Since many of the subglacial aquatic environments are likely to contain a low biomass of microbes it is of critical importance to minimize the introduction of exogenous microbes, whether living or dead, and even of exogenous nucleic acids to (1) prevent changes in the native microbial composition and (2) allow for proper investigation of the native microbial community (and not contaminants) of the subglacial lake environment.
Potentially, a variety of allochthonous microbes (“contaminants”) could be introduced into subglacial lake environments via drilling and sampling activities. These allochthonous microbes may include microorganisms native to the surface environment or introduced from humans or equipment brought to the sampling area. Microbes immured in glacial ice for long periods of time might conceivably proliferate once they are released back into the modern-day biosphere through natural melting processes or through research activities. The majority of this release is likely to be of prokaryotic organisms, although possible introduction of microeukaryotes and viruses cannot be discounted (e.g., Rogers et al. 2004; Smith et al. 2004). For example, tomato mosaic virus, a member of the highly stable tobamovirus group, has been isolated from decontaminated Greenland ice cores ranging in age from <500 to 140,000 years before the present (Castello et al. 2005). Human and animal pathogens are also likely to be present in glaciers and ice sheets (Shoham 2005). Even if these microbes and pathogens
did not grow, their presence might confound measurements based on macromolecules (e.g., DNA, proteins).
Unless these microbes are introduced along with substrates that provide them a nutritional edge, it is less likely that allochthonous microbes would outcompete autochthonous (already present) ones. Microbes recovered from subglacial aquatic environments may not survive in surface environments due to a variety of factors. For example, it is more likely that microbes released from the overlying ice sheet into the atmosphere would survive more readily than microbes recovered from subglacial lakewater, given that long-term preservation would be more effective in deep frozen ice than in liquid-water conditions.
In addition, subglacial microbes may be light sensitive, may not be able to adapt to a more nutritionally rich environment, may not be able to survive under lower pressures or they may be more sensitive to new populations of grazers or other predators. These features would place them at a disadvantage relative to other taxa if they were transferred into less severe environmental conditions, where they would likely be outcompeted by the native microbiota. As discussed in Chapter 3, even microbes isolated in ice for 1 million years are unlikely to have a genome exhibiting significant genetic divergence because of the slow pace of prokaryotic evolution.
The work of Karl et al. (1999) on water from accretion ice, however, does suggest that some fraction of recovered microbes could remain viable at the surface. Spores that might be either introduced to or removed from subglacial aquatic environments represent a potentially different situation because they are able to survive under many extreme conditions over very long time frames (millions of years) (Vreeland et al. 2000). For example, spores present in a subglacial lake environment such as Lake Vostok might have been deposited millions of years ago prior to the formation of the lake and, if returned to the surface, could become viable once more.
OTHER POTENTIAL SOURCES OF CONTAMINATION—PARTICULATES IN THE FLUID
This category includes biotic constituents such as bacterial cells, viruses, and fungi. Drilling fluids at the Vostok site (Alekhina et al. 2007) and at the EPICA sites (S. Bulat, personal communication) are known to contain many microbial taxa, including genera such as Sphingomonas that have also been detected in hydrocarbon-contaminated soils in Antarctica and elsewhere and on Greenland ice cores (Alekhina et al. 2007). Technologies that are under development for the detection of life in subglacial lakes (e.g., fluorescence-based systems; see Bay et al. 2005) may also provide sensitive detection of dissolved and particulate organic contaminants.
Abiotic particulates are probably best monitored via turbidity sensors that could be lowered down the access holes. A variety of optical backscattering instruments are used routinely for water column profiling in limnology and oceanography, and these have detection limits at or below 1 NTU (nephelometric turbidity unit).7
Combined Microbial and Chemical Contamination
Drilling fluids can be heavily contaminated not only with microorganisms but also with the substrates that they use for growth (Alekhina et al. 2007). The release of this inoculum plus growth medium into the relatively warm (by comparison with the ice hole and surface environment) liquid-water conditions of a subglacial lake could result in a short-term phase of increased metabolism and growth. A variety of hydrodynamic circulation processes are likely to operate in Lake Vostok and other subglacial waters, and localized inputs of contamination could be widely dispersed, including between lakes via subglacial hydrological networks.
Isotopic Contamination
Naturally occurring stable isotopes and radioisotopes are routinely analyzed in biogeochemical studies and provide many insights into the structure and functioning of aquatic ecosystems. The relatively small transfer of unusual isotopes during lake access (e.g., from drilling fluids) or by release of deep water and sediments into the upper water column (see below) could potentially compromise the geochemical interpretation of some isotopic signatures. These problems have been encountered in the McMurdo Dry Valley lakes where a variety of radioisotopic and stable isotopic techniques have been used in situ, and where 14C contamination of some waters has precluded the use of radiodating techniques.
Intrasystem Contamination
In two NSF-sponsored workshops concerning environmental management of the McMurdo Dry Valleys, it was recognized that research activities targeting one component of the environment had the potential to cause contamination or damage of another. Accordingly, an Environmental Code of Conduct was developed that included specific measures to minimize or avoid such effects on the aquatic microbial ecosystems of this region (Vincent 1996; Wharton and Doran 1999). For example, the anoxic bottom waters of the McMurdo Dry Valley lakes have a high nutrient and metal content that, if discharged to the surface waters or overlying lake ice, could potentially enrich or inhibit the microbial communities growing in those strata. The Code of Conduct now requires that all sampled water, including that used to rinse and clean bottles, routinely be removed from the valleys.
In subglacial lakes there also may be large differences in chemical properties among liquid-water strata, including the possibility of anoxia at depth in the water column and in the sediments (Siegert et al. 2003). Releases of water during sample removal could enrich or inhibit communities in the water column or overlying ice. As in lakes elsewhere (Wetzel 2001), the sediments underlying subglacial lakes are likely to contain orders-of-magnitude higher concentrations of microbiota, nutrients, and metals. The benthic microbial communities also are likely to have a very different phylogenetic composition than those in the water column, and transfers during sampling could compromise subsequent measurements of water and ice samples (e.g., DNA clone library analysis).
The glacial ice is likely to contain a variety of viral, bacterial and fungal species (e.g., Ma et al. 2005; Christner et al. 2005b) including organisms that could potentially
grow in the solute-rich water sequestered between the triple junctions of ice crystals (Price 2000). These microbiota could be transferred deeper into the system through sampling and could potentially grow (e.g., in meltwaters produced during hot-water drilling). In the Lake Vostok core, different sections of the accretion ice contain materials frozen in from different parts of the lake, allowing contamination from one part of the lake to another. This latter effect is likely to be small; however, if hydrodynamic processes are operating across the lake, the effect could be magnified. Furthermore, the melting of overlying glacial ice is a continuous natural source of microbiota for Lake Vostok and probably other subglacial lakes.
POTENTIAL FOR TESTING AND ASSESSING CONTAMINATION: EXPERIENCES FROM DEEP-BIOSPHERE SAMPLING
The advent of molecular and genomic approaches to biological measurements has continued to broaden our concepts and understanding of microbial diversity. It is now commonplace to sample the presence and diversity of microbial communities from many environments throughout the biosphere. Of particular importance to these investigations, especially those occurring in areas where biomass is predicted to be low (such as the deep biosphere), is the prevention of contamination of the environment and subsequent samples with exogenous life or nucleic acids.
Most protocols examined to date have focused on preventing (or at least minimizing) contamination of samples extracted from the environment in question and, by extension, providing a measure of protection to the environment itself. These protocols center on (1) using advancements in drilling technology to obtain the necessary depths and (2) incorporating the use of sterile liners and other sterile devices for extracting samples from rock, sediment, or water.
Most protocols for sediment cores recommend further processing of the core under sterile conditions and removing interior portions for actual microbiological analysis. The inclusion of tracer studies to track possible contamination during any deep-biosphere sampling has also been viewed as an important approach to these studies. Interestingly, much less emphasis has been placed on determining the risks or effects of reverse contamination—where the surface environment or personnel operating drilling equipment or collecting samples are exposed to microorganisms from the deep environment under investigation.
Terrestrial Deep Subsurface Drilling
Investigation of microbial life in the deep biosphere represents an important undertaking in the search for the nature and diversity of microbial life. The majority of experience gained thus far in sampling has occurred in the terrestrial deep subsurface (>100-m depth) including groundwater, aquifer sediment, and rock matrices. The ability to conduct sampling in these environments has been aided by advances in specialized drilling technologies. In terrestrial settings, rotary drilling is a common method when drilling to depths >100 m, although cable tool drilling has also been used to obtain high-quality samples for microbial analysis from unconsolidated sediments from at least 200 m and has the advantage that no recirculating drill fluids are necessary. Push-type core barrels such as split-spoon core barrel devices are the most common samplers used in cable drilling to obtain core samples of formation materials for microbiological
sampling. These devices are advantageous; they can be disassembled readily for cleaning, and sterile inner core liners can be used. Rotary drilling and coring is a commonly used technology for obtaining samples from deep subsurface environments. Similar to ice coring, this system also requires the use of drilling fluids, which can be problematic due to the presence of bacteria (Hurst 1997).
Alternative methods that have demonstrated some success in reducing microbial contamination involve the use of inert gases (e.g., argon, nitrogen), although their application can be most effective only at more limited depths because of the extreme hydrostatic pressures that must be overcome when boreholes fill with groundwater. A Gel-Coring system (Baker-Hughes, Casper, Wyoming) has been used with some success. With this method, a core liner is first filled with a viscous, inert gel, which surrounds the core as it advances, forming “platelets” that confine the core, thereby decreasing its exposure to oxygen and drilling fluids (Hurst 1997).
To estimate sample quality, including contamination by drilling fluids, inert tracers can be used. Commonly used tracers principally for geochemical analyses are bromide, rhodamine, and fluorescein. Tracers that have been applied in terrestrial subsurface sampling for microbial analyses include a microorganism with an uncommon but easily detected phenotype (e.g., a distinguishing pigment). Obvious concerns exist with (and regulations may prohibit) the introduction of another microbial life form in many sampling situations. Alternatives include the use of fluorescent microspheres. Microspheres are readily available in a range of sizes and charges and with different fluors that are easily detectable via epifluorescence microscopy (Hurst 1997).
Deep Subsurface Ocean Drilling
The Integrated Ocean Drilling Program (IODP), operating from 2003 to the present (built from the successful legacy programs, the Deep Sea Drilling Program [DSDP] and the Ocean Drilling Program [ODP]), represents an international scientific research plan focused on the exploration of the deep biosphere of the Earth’s crust and sediments from deep beneath the ocean (http://www.iodp.org). As a part of this program, several studies have been conducted to examine methods of monitoring microbial contamination of sediment cores and rock recovered from the deep ocean subsurface. The methods employed were adaptations of techniques used in land-based drilling as described above. Results of this work tested during the Leg 185 cruise (Smith et al. 2000) are summarized below.
The use of chemical and particulate tracers was evaluated to track microbial contamination (Smith et al. 2000). These tracers were introduced while drilling (1) unconsolidated sediments using an advanced piston corer (APC), (2) sedimentary rock using an extended core barrel and rotary core barrel (RCB), and (3) igneous rock using the RCB and diamond core barrel. Perfluorocarbon tracers ( PFTs) that could be measured by gas chromatography and a particulate tracer of fluorescent microspheres that could be measured by epifluorescent microscopy were tested. The importance of verifying that the PFT had been delivered effectively with each sample was noted (otherwise the lack of measurable PFT in the core is merely a false negative). Conclusions from these evaluations were that both tests should be included for monitoring contamination.
Because the PFT analysis can be performed quickly, it represents a mechanism that could be used for the early detection of possible contamination during the sampling process. In the tests described, the lower limit of detection was 6 × 10−12 g of PFT
and higher sensitivity was expected with the use of smaller bore GC columns or possibly a less volatile PFT (Smith et al. 2000). Results of PFT measurements suggested that drilling fluid infiltration was in the range of nanoliters per gram of core material, leading researchers to conclude that depending on the coring methods used and the formations sampled, contamination may represent at most 1-10 bacteria per gram of core material. Intrusion of fluorescent microspheres into the core interior was not detected in the APC-cored unconsolidated sediments or RBC-cored consolidated sediment or rock. However, microspheres were detected in thin sections tested from the igneous rock samples, leading researchers to conclude that processing of the rock after sample extraction was the likely source of contamination (Smith et al. 2000). PFT and fluorescent microsphere tracers have seen continued use in deep sea subsurface sampling conducted by the IODP (D’Hondt et al. 2004), and methodologies for contamination-free sampling and monitoring continue to be investigated (Lever et al. 2006).
POTENTIAL FOR TESTING AND ASSESSING CONTAMINATION: EXPERIENCES FROM INTERPLANETARY RESEARCH
Requirements for cleaning are driven by mission requirements (as set out by the National Aeronautics and Space Administration [NASA]) and are based upon landing or regional concerns on the target planet or moon. Current sterilization levels are based on criteria established during the Viking pre-launch biological research conducted in the 1970s and are accepted and implemented by NASA as current requirements. Examples of specific measures to control contamination include reduction of the spacecraft’s biological burden, a spacecraft organic inventory and material archive, and documentation of spacecraft trajectories and restrictions on returned samples.
Reduction includes sterilization and cleaning by approved and certified techniques. Sterilization requires that the entire landed system be sterilized at least to Viking post-sterilization biological burden levels or to levels of biological burden reduction driven by the nature and sensitivity of the particular life detection experiments, whichever are more stringent. Subsystems that are involved in the acquisition, delivery, and analysis of samples used for life detection must be sterilized to these levels, and a method for preventing recontamination of the sterilized subsystems is also required. Terminal sterilization methods (the terminal Viking bake-out process) were conducted after the lander had been sealed inside the aeroshell and bioshield capsule. The entire capsule was placed inside a thermal chamber (specially designed for the sterilization process) and the methods were verified by proxy; a sterilized spacecraft or component is never assayed directly. The acceptable terminal sterilization bioburden level was derived from the Viking pre-sterilization level of 3 × 105 spores per vehicle, which was reduced after bake-out by four orders of magnitude to a calculated value of 30 spores per vehicle. Such an approach using heat treatment is clearly impracticable in general for Antarctic sampling at present, although it may prove possible to apply it to the final sampling assembly if this is sealed inside a shell for transfer down the drill hole.
Cleaning requirements for spacecraft or parts that do not require terminal sterilization are driven by the pre-bake-out bioburden levels determined analytically on the Viking landers to be 3 × 105 spores per vehicle. Bioburden reduction techniques include clean-room assembly practices, dry heat to reduce the number of microbes, and physical cleaning of spacecraft components and surfaces. Clean-room assembly requirements
include Class 100K (International Organization for Standarization [ISO] Class 8) or better for spacecraft and payload assembly. Personnel controls include requirements for hoods, face mask or beard covers, sterile gloves, booties, taped wrists and ankles to prevent gaps between gloves and suits, and frequent (daily) change-out of clean-room garments. Additional assembly measures include attentiveness to bioload requirements including certification of manufacturing process such as cleaning procedures and products associated with clean room garments, tool sterilization and cleaning, and certification training in planetary protection practices for everyone associated with the spacecraft. When necessary physical barriers within the clean room are used to prevent cross-contamination from noncertified personnel or non-spacecraft-related activities.
Dry heat microbial reduction techniques require that specified heat, humidity, and exposure duration parameters are met, so that treated components may “take” a predicted order-of-magnitude bioburden reduction as specified by NASA Procedure Requirements (NPRs). Microbial reduction is often applied to parts that cannot easily be cleaned and assayed, such as thermal blankets or multilayer insulation constructed in such a manner that the material has a calculated high burden density but cannot readily be cleaned using manual methods.
Physical cleaning of spacecraft is accomplished mostly by wiping surfaces using isopropyl alcohol and sterile clean-room cloths. Physical removal of bacteria via wiping is implemented rather than using bacterial agents to kill surface bacteria. If the part or component can withstand autoclaving, this is also a suitable alternative. Prior to mating or installing components, surfaces are assayed to determine spore counts. The verification sampling is generally conducted in the assembly area, with the resulting sample transported to the laboratory for analysis. Assays are culture-based methods and require traditional plating techniques with a minimum three-day incubation period before final data are available. Verification assays are taken at “last access” for mated surfaces, but exposed areas on the spacecraft are routinely monitored as well to verify that the vehicle meets the required cleanliness levels through the months of assembly. Any time a surface is de-mated or rework occurs on the vehicle, the re-assay is required.
Many samples of bacteria were collected during the assay development phase of the Viking mission (Viking Collection and Teflon Ribbon Isolate Microbial Collection). These samples were taken via swab or wipe methods or using Teflon ribbons from spacecraft surfaces and assembly environments and were evaluated biochemically prior to storage. The isolates were lyophilized and sealed in glass ampoules and stored at Kennedy Space Center until recently when the collections were moved to the Jet Propulsion Laboratory. The Viking collection contains 1296 isolates; the Teflon Ribbon Isolate Collection contains 234 isolates. Currently there are no requirements to archive bacteria, although a limited effort is under way at the Jet Propulsion Laboratory to build a planetary protection bacterial archive based on clean-room and spacecraft samples obtained during the assembly process.
Planetary protection does require an archive of some materials used in spacecraft assembly (see requirement summary below), but there are no specific requirements to archive bacteria for each mission. However, with recent discussions of sample return and the ever-increasing understanding of planetary surfaces, an interest has resurfaced in typing and archiving pre-launch bioburden samples.
WHAT LEVELS OF CLEANLINESS ARE FEASIBLE WHEN ACCESSING SUBGLACIAL ENVIRONMENTS?
Planetary protection cleanliness requirements are achieved in a closed environment, generally in class 100K clean rooms or other facilities that have controlled environments. Spacecraft and associated hardware are cleaned, assayed to confirm bioburden levels, and maintained clean throughout building and processing. The external environment of the spacecraft is controlled up to the time of launch to prevent recontamination. While the process is dynamic due to test and rework of hardware, for the most part hardware is accessible for recleaning and assaying. Once certified as clean, mated surfaces that are no longer accessible remain so by definition unless separated due to rework or reassembly requirements. In this environment it is possible to quantify the bioburden on the spacecraft and to maintain cleanliness requirements as dictated by planetary protection protocols.
Current levels of cleanliness associated with planetary protection standards are not feasible in the open environment associated with subglacial lake exploration. While it may be possible to control the quantity of cells associated with drilling and sampling operations initially, it is not possible to control the likely transfer and distribution of cells during the drilling and sampling process. Using current technologies, it is likely that operations will transfer cells between different strata in the borehole. Drilling and sampling equipment can be precleaned prior to penetration, but the extreme depths required for drilling and the fact that hardware cannot readily be accessed for recleaning make stringent bacterial cleanliness requirements such as those implemented in space research unachievable.
In addition the water environment of the lakes facilitates cell transfer from any object that may find its way into liquid water. If the lake has unique biological ecosystems, transfer may also occur as a robotic sampling device moves from ecosystem to ecosystem. In general, cleanliness requirements will have to be addressed in terms of (1) cleaning hardware (and quantification of bacterial levels and diversity) prior to penetration, (2) maintaining hardware cleanliness levels as much as possible during penetration, and (3) designing research techniques that minimize the possibility of cell transfer between different levels in the ice and the lake bed itself.
BIOLOGICAL CONTAMINANT DETECTION
The detection of whole cells has traditionally relied on a variety of techniques including culture-based methods in which different media are used to grow organisms from a sample that can then be counted microscopically or as colonies on plates. These techniques are limited in scope because only those organisms capable of growing on a particular media formulation can be cultured, and these may constitute only a small fraction of the total community (Kämpfer et al. 1996). Microscopic counts of spores have also been used as proxies of bacteria (see Potential for Testing and Assessing Contamination: Experiences from Interplanetary Research). An important set of techniques for counting intact cells is the use of fluorescent dyes such as SYBR Gold, 4’,6-diamidino-2-phenylindole (DAPI), and acridine orange (AO). These methods use epifluorescence microscopy or flow cytometry to quantify the fluorescent-stained cells, and they are appropriate to the ongoing analysis of Antarctic glacial ice and drilling fluids for environmental monitoring and management. Application of this approach
to samples from the lower strata of Vostok glacial ice has given counts of the order of 102 DNA-containing cells per milliliter of melted ice (Christner et al. 2006, Figure 2A), and this forms the basis for the committee’s Recommendation 7.
Molecular biological and genomic approaches offer a suite of tools that that can be complemented with culture-based techniques and biochemical methods to provide new and powerful strategies not only for gaining fundamentally new insights into the diversity of microbial life in subglacial aquatic environments but also for detection of exogenous cells and nucleic acids. A variety of molecular-based methods already exist and have been used extensively since at least the mid 1990’s for surveys of microbial diversity and community structure (denaturing gradient gel electrophoresis [DGGE], terminal restriction fragment length polymorphism [T-RFLP], fluorescence in situ hybridization [FISH], clone libraries) that rely on the identification of nucleic acids and circumvent culturing biases. Of particular importance has been the construction of clone libraries of genetic markers that can be sequenced and analyzed using phylogenetic methods to assess microbial diversity using 16S rRNA (for prokaryotes) and 18S rRNA (for eukaryotes) gene sequences. For a review of the techniques discussed in this paragraph see Spiegelman et al. (2005). In the future, the use of other genetic markers or the use of multiple genetic markers should also be considered. There is no universal protocol for performing any of these techniques. Investigators should document their methods and results carefully, utilize appropriate controls, and work toward developing standardized protocols for contaminant detection and documentation.
Biology continues to undergo a revolution with the advent of new genomic, post genomic, and culturing techniques (Handelsman 2004; Page et al. 2004; Xu 2006). These rapidly progressing areas of science not only can be applied to studying microbial diversity and their functional roles in the environment but also hold great promise in providing rapid and sensitive methods for detecting and identifying the introduction of exogenous microbiota or nucleic acids. The ongoing development and application of these technologies with the goal of producing standardized protocols of methods and documentation of results should be strongly encouraged in the arena of biological contaminant detection.
CONCLUSIONS
The problem of how to penetrate and sample subglacial aquatic environments in the cleanest manner possible remains a challenge because any form of invasive sampling of a subglacial aquatic system will result in some level of perturbation to the environment. Current drilling technologies are not sterile and it is not possible to guarantee that subglacial aquatic environments will not be contaminated during drilling, sampling, and monitoring. Drilling approaches that result in freezing subglacial water inside the borehole have worked well to keep the drilling fluids in the borehole (Byrd and EPICA DML boreholes in Antarctica and the NGRIP borehole in Greenland), but contamination of the core recovered by redrilling was evident.
During penetration and exploration of a subglacial aquatic environment, chemical contaminants could enter the water as liquid constituents of the drilling fluid and as solutes or particulates in that fluid. Direct contamination could also occur from the drilling and sampling apparatus, for example, from water-soluble oils used in metal working during the fabrication of the instruments and equipment or from phthalate ester additives that are used extensively in the plastics industry. The nature and mag-
nitude of contamination from each of these sources will be highly specific to the type of drilling and sampling operation.
Biological contamination of subglacial aquatic environments can come from a variety of sources that contain not only microorganisms but also the substrates they use for growth (Alekhina et al. 2007). Drilling fluids may contain both microbiota and growth substrates. The release of this inoculum plus growth medium into the relatively warm (by comparison with the ice hole and surface environment), liquid-water conditions of a subglacial lake could result in a short-term phase of increased metabolism and growth. It is of critical importance to minimize the introduction of living exogenous microbes, and even of exogenous nucleic acids, to prevent changes in native microbial composition and to allow for proper investigation of the native microbial community (and not contaminants).
A variety of allochthonous microbes (“contaminants”) could be introduced into subglacial aquatic environments from microorganisms native to the surface environment or from humans or equipment brought to the sampling area. Microbes could be transferred deeper into the system through sampling and could potentially grow (e.g., in meltwaters produced during hot-water drilling). Microbes immured in glacial ice for long periods of time might conceivably proliferate once they are released back into the modern-day biosphere through natural melting processes or research activities.
In subglacial lakes, large differences in chemical properties among liquid-water strata are possible, including the presence of anoxia at depth in the water column and in the sediments. Releases of water during sample removal could enrich or inhibit communities in the water column or overlying ice. As in lakes elsewhere, the sediments underlying subglacial lakes are likely to contain orders-of-magnitude higher concentrations of microbes, nutrients, and metals. The benthic microbial communities also are likely to have a very different phylogenetic composition than those in the water column, and transfers during sampling could compromise subsequent measurements of water and ice samples (e.g., DNA clone library analysis).
In addition, research activities targeting one component of the environment may have the potential to cause contamination or damage of another. Once contamination reaches the aquatic environment, a variety of hydrodynamic circulation processes are likely to operate in the subglacial waters, and these localized inputs of contamination could be widely dispersed. This is particularly important for subglacial aquatic environments that are connected via subglacial hydrological networks. However, protocols or codes of environmental conduct may be developed to include specific measures to minimize or avoid such effects on the aquatic microbial ecosystems of these environments. If downstream sites are the initial targets of investigation, this may reduce the potential risk of contamination to other environments along a specific flow path.
Molecular biological and genomics approaches have made it routine to sample the presence and diversity of microbial communities from many environments throughout the biosphere. Of particular importance to these sampling regimes, especially those taking place in areas where biomass is predicted to be low, is the prevention of contamination of the environment and subsequent samples with exogenous life or nucleic acids. These precautions should seek to preserve both the subglacial aquatic environment and the integrity of the scientific samples.
Most protocols, that focus on preventing contamination of the environments and protecting the integrity of samples extracted from the environment have employed advancements in drilling technology to obtain the necessary depths and allow the
use of sterile liners and other sterile devices for obtaining sample cores (for solids) or liquids. Most protocols for sample cores recommend further processing of the core under sterile conditions and removing interior portions for actual microbiological analysis. The inclusion of tracer studies to complement and track possible contamination during any deep-biosphere sampling is also an extremely important step in most protocols examined.
Current levels of cleanliness associated with planetary protection standards are not feasible for the exploration of subglacial aquatic environments. Although it may be possible to control the initial number of cells associated with drilling and sampling equipment using current technologies, it is not possible to prevent the transfer and distribution of cells between different strata in the borehole during the drilling and sampling processes. Drilling and sampling equipment can be cleaned prior to entering the ice, but the extreme depths achieved during drilling and the fact that the drilling hardware is inaccessible for subsequent cleaning make stringent bacterial cleanliness requirements such as those implemented in space research unrealistic.
The hydrodynamic nature of subglacial aquatic environments facilitates cell transfer from any object that may find its way into liquid water. If the lake has unique biological ecosystems, transfer may also occur as a robotic sampling device moves from ecosystem to ecosystem. In general, requirements for cleanliness will have to be addressed in terms of (1) cleaning hardware (quantification of bacterial levels and bacterial diversity present) prior to penetration, (2) maintaining hardware cleanliness as much as possible during penetration, and (3) designing research techniques that minimize the possibility of cell transfer between different levels in the ice and the lake bed itself. Specific decontamination techniques need to be developed for any instruments deployed in a subglacial aquatic environment. The current decontamination approaches include sterilization by heat (autoclave) and/or chemical treatment (peroxide). The instrument packages must be robust to maintain their operational specifications following these decontamination protocols.
A critical aspect of subglacial lake exploration and technology development is testing, verification, and monitoring of potential contamination during all phases of the scientific program. There must be deliberate and careful scrutiny of the methodologies employed, from ice drilling to sample recovery, from both an environmental stewardship and a scientific standpoint. Stewardship issues include providing the maximum possible protection of subglacial lake environments by ensuring minimal alteration or change due to the planned scientific studies. From a scientific standpoint, it is essential that uncompromised samples be provided for study and that the presence of human-made devices does not bias the data collected. There is also concern that unusual or previously unknown biological agents be properly handled upon retrieval to avoid an unwanted release to the environment.