2
The Changing Climate
J. MURRAY MITCHELL. JR.
National Oceanic and Atmospheric Administration
2.1
WEATHER STATISTICS AND CLIMATE DYNAMICS
The term “weather” refers to the total panoply of atmospheric conditions as they vary from moment to moment and from place to place on the earth.
The term “climate” always connotes “average weather” but properly includes consideration of weather extremes, joint frequency distributions, and many other measures of weather variability in both time and space. The classical view of climate leaves it at that: a purely statistical concept in which climate is nothing more than the sum of its parts (i.e., the collective of weather as experienced at a point or over a designated area of the earth, over a period of years). Such a view of climate, useful for many practical purposes, is better referred to as climatography.
Another, more enlightened view of climate differs from the classical view in that it recognizes climate as a basic physical entity and weather as the momentary, transient behavior of the atmosphere striving to satisfy the requirements dictated by the climate for horizontal and vertical transfer of mass, momentum, and energy. In this view, weather is the means to a climatic end: the maintenance of a global-scale atmospheric heat engine striving to transport solar heat from where it is in superabundance (principally the tropics) to where it is in deficit (principally the polar regions). This view of climate, far more illuminating in the context of understanding (rather than merely describing) overall atmospheric behavior, introduces the notion of climate dynamics.
We can briefly characterize climate and its origins in the following way.
The climate of any locality on the earth is fundamentally determined by a hierarchy of environmental influences, which range in scale from the general astronomical setting of our planet down to minute details of the local environment within and immediately surrounding that locality. The atmosphere itself plays a very important intermediary role in the way that these diverse influences combine at the point to help to establish its climate and also contributes an important influence of its own to local climate.
This hierarchy of climatic influences involves a wide variety of environmental factors. Some of these factors affect the general characteristics and distribution of climate over very large regions of the earth’s surface (the so-called macroclimate), while others affect climate with regard to its more
detailed characteristics and distribution within smaller and smaller regions (mesoclimate and microclimate).
Generally speaking, the size of the region over which the climate (or some characteristic of climate) is systematically influenced by a given factor—whether natural or man-made—bears a rather close relationship to the geographical dimensions of the factor itself. For example, the distribution of solar radiation over the whole earth, dictated by the seasonally varying geometry of the earth’s orbit and axis of spin relative to the sun, affects climate (together with atmospheric circulation) on the scale of the whole earth. On the other hand, the climatic influence of a small lake, for example, extends over an area not much larger than the lake itself. Thus we can conclude that, in general, global-scale qualities of climate are governed by global-scale environmental factors and not by local-scale factors, except under special circumstances for which local-scale factors are operating in unison over large regions of the earth. When considering the climatic impacts of power-plant cooling towers, for example, questions of the geographical scale of such impacts arise that have to be kept in mind.
2.2
THE RECORD OF PAST CLIMATES
For more than a century it has been known from a variety of geological evidence that the earth’s climate was not always what it is today. It has been only in the last few years, however, that highly significant developments in the new and rapidly developing science of quantitative paleoclimatology have begun to provide us with insights into many details of the earth’s climatic history, especially that of the last million years. These insights lend valuable perspective to the climate of the present.
Briefly stated, the climate of the earth is now known beyond any doubt to have been in a more or less continual state of flux. Changeability is evidently a characteristic of climate on all resolvable time scales of variation, from that of aeons down to those of millennia and centuries. The lesson of history seems to be that climatic variability must be recognized and dealt with as a fundamental quality of climate and that it would be potentially perilous for modern civilization to assume that the climate of future decades and centuries will be free of similar variability.
With this lesson in mind, some salient features of the earth’s climatic history are worth outlining here.
Geological evidence leaves little doubt that, during the past billion years or so, the prevalent condition of global climate was one of relative warmth—as much as 10°C warmer than now—and almost totally free of polar ice (see Figure 2.1). T his warm condition was, however, punctuated by relatively short ice-age intervals of the order of 10 million years’ duration and separated by a few hundreds of millions of years. Begin-
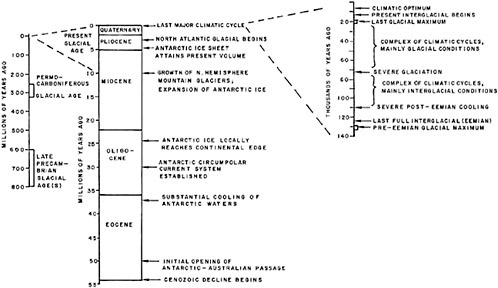
FIGURE 2.1 History of glacial ages over the last 1,000,000,000 years. Intervals when extensive polar ice sheets occurred are indicated as glacial ages on the left. An outline of significant events in the Cenozoic climate decline is given in the middle, and the significant climate events during the last major glacial-interglacial cycle are given at right, Source: U.S. Committee for the Global Atmospheric Research Program, Understanding Climatic Change: A Program for Action, National Academy of Sciences, Washington, D.C., 1975.
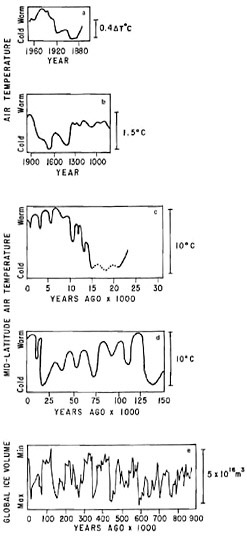
FIGURE 2.2 General trends in global-scale climate for the past million years: (a) changes in the five-year average surface temperatures over the region 0–80° N; (b) winter severity index for eastern Europe; (c) generalized northern hemisphere air-temperature trends, based on fluctuations in Alpine glaciers, changes in tree lines, marginal fluctuations in continental glaciers, and shifts in vegetation patterns recorded in pollen spectra; (d) generalized northern hemisphere air-temperature trends based on midlatitude sea-surface temperature, pollen records, and worldwide sea-level records; (e) fluctuations in global ice-volume recorded as changes in isotopic composition of fossil plankton in a deep-sea core. Source: GARP Publications Series No. 16, The Physical Basis of Climate and Climate Modeling, Report of the International Study Conference in Stockholm, 29 July-10 August 1974, World Meteorological Organization, International Council of Scientific Unions, Geneva, 1975.
ning roughly 50 million years ago, something happened to bring about a gradual deterioration of climate. This deterioration culminated, about 2 million years ago, in the arrival of a new mode of climate, characterized by a long sequence of perhaps as many as 20 major glacial-interglacial oscillations, which presumably continues to grip the world today.
The history of glacial-interglacial events is revealed in some detail for the past one million years by oxygen-18 analysis of deep-sea sediments and by several other kinds of paleoclimatic indicators. In that period of time, and most particularly during the last half-million years, the principal variations of ice volume and other conditions of climate appear to have been of a quasi-periodic nature, with a characteristic wavelength close to 100,000 years (see Figure 2.2). Lesser ice volume changes seem also to have occurred, with characteristic periods of about 20,000 and 40,000 years. The world has been in a relatively warm extreme-interglacial phase during the past 10,000 years, similar to other relatively brief warm phases that have occurred earlier at roughly 100,000-year intervals. In these interglacial phases, ice has been confined primarily to the Antarctic and Greenland ice sheets. In the intervening glacial phases, continental ice sheets have developed primarily in high northern latitudes, which covered a maximum of about 9 percent of the earth’s surface and reached a volume of about 75 × 106 km3—some three times present-day figures. The variations of ice conditions have been accompanied by midlatitude temperature changes of the order of 6 to 10°C and by sea-level changes of the order of 100 m.
Since recovery of the earth from the last major glacial stage about 10,000 years ago, global climate has been found from a variety of paleoclimatic indications to have varied within narrower limits (see Figure 2.3). An intercontinental survey of mountain glacier moraines and tree lines has revealed three periods of glacier expansion (each of about 1000 years’ duration), alternating with three periods of glacial contraction (each of 1000 to 2000 years’ duration) in the past 8000 years. There is evidence, derived primarily from pollen analyses of lake and bog sediments, that significant shifts of the earth’s vegetation zones accompanied these glacial variations, in what is sometimes referred to as the “neoglacial cycle.” General temperature levels are believed to have varied by about 1 or 2°C during the course of this cycle.
Narrowing our attention to the past millennium, we can begin to call into play various additional climatic indices such as human chronicles, tree-ring sections, and annually laminated ice cores from Greenland and Antarctica, to add further detail to our reconstruction of climatic history (see Figure 2.4). The period from about A.D. 1430 to A.D. 1850 was one of comparatively cold climate and expanded mountain glaciation. A member of the “neoglacial cycle,” noted above, this cold period is commonly referred to as the “Little Ice Age.” Earlier centuries were milder, although probably not everywhere as warm as the climate of today. From long instrumental records and human chronicles, typical fluctuations of 30-year averages of climatic variables over the past several centuries have been as follows: Major circulation features, such as the centers of subpolar lows and subtropical highs, and the position of the Intertropical Convergence Zone, have varied by 2 or more degrees of latitude. The positions of midlatitude troughs and ridges have shifted east or west
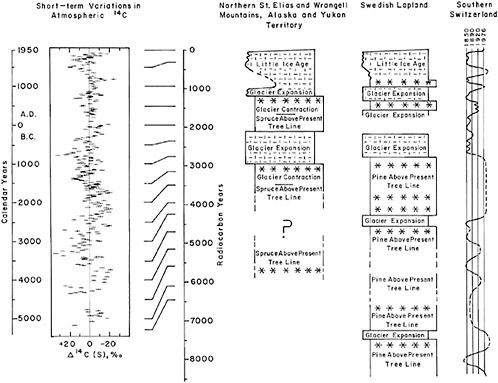
FIGURE 2.3 Holocene short-term atmospheric 14C variations, glacial events, and tree-line fluctuations. Short-term 14C variations are from Suess (1970; Figures 1 and 2), Glacial and tree-line events in St. Elias and Wrangell Mountains are from Denton and Karlén (1977); those in Lapland are from Karlén (1973, 1976; Karlén and Denton, 1976). Glacial variations in southern Switzerland are from Röthlisberger (1976).
References:
Denton, G. H., and W. Karlén (1977). Holocene glacial and tree-line variations in the White River Valley and Skolai Pass, Alaska and Yukon Territory, Quaternary Res. 7, 63.
Karlén, W. (1973). Holocene glacier and climatic variations Kebnekaise Mountains, Swedish Lapland, Geografiska Ann. 55, 29.
Karlén, W . (1976). Lacustrine sediments and tree-limit variations as indicators of Holocene climatic changes in Lapland, northern Sweden, Geografiska Ann. 58, 1.
Karlén, W., and G. H. Denton (1976). Holocene glacial variations in Sarek National Park, northern Sweden, Boreas 5, 25.
Röthlisberger, F. (1976). Gletscher- und Klimaschwankungen im Raum Zermatt, Ferpècle und Anolla, Die Alpen 52 (3), 59.
by 10 to 20° longitude. Regional variations of temperature have been of the order 1–2°C, and those of precipitation, of the order of 10–20 percent. Much larger variations have been determined to occur over periods shorter than 30 years. If repeated in the near future, variations of these magnitudes would probably have highly significant impacts on water resources and agricultural productivity.
In the first half of the twentieth century, the world was enjoying a full recovery, at least temporarily, from the Little Ice Age (see Figure 2.5). There are indications that, at that time, the circumpolar westerlies contracted toward the poles, and that, in the northern hemisphere at least, the amplitude of the planetary waves underwent a decrease. Also at that time, a general warming of the earth occurred,
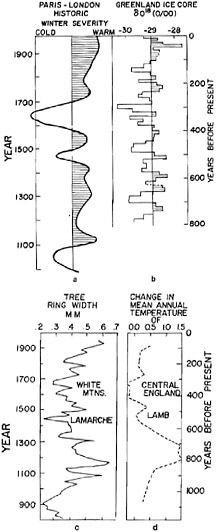
FIGURE 2.4 Climatic records of the past 1000 years. (a) The 50-year moving average of a relative index of winter severity compiled for each decade from documentary records in the region of Paris and London (Lamb, 1969). (b) A record of δ18O values preserved in the ice core taken from Camp Century, Greenland (Dansgaard et al., 1971). (c) Records of 20-year mean tree growth at the upper treeline of bristlecone pines, White Mountains, California (LaMarche, 1974). At these sites tree growth is limited by temperature with low growth reflecting low temperature. (d) The 50-year means of observed and estimated annual temperatures over central England (Lamb, 1966).
References:
Lamb, H. H. (1969). Climatic fluctuations, in World Survey of Climatology, 2, General Climatology, H. Flohn, ed., Elsevier, New York, pp. 173–249.
Dansgaard, W. S., S. J. Johnsen, H. B. Clausen, and C. C. Langway, Jr. (1971). Climatic record revealed by the Camp Century ice core, in The Late Cenozoic Glacial Ages, K. Turekian, ed., Yale U.P., New Haven, Conn., pp. 37–56.
LaMarche, V. C., Jr. (1974). Paleoclimatic inferences from long tree-ring records, Science 183, 1042.
Lamb, H. H. (1966). Climate in the 1960s, Geog. J. 132, 183.
which was most pronounced in the Atlantic sector of the sub-Arctic. A rapid worldwide retreat of mountain glaciers and a poleward extension of the ranges of many flora and fauna took place then.
There is considerable evidence that, between the 1940’s and about 1970, the climatic changes of the earlier part of this century had tended to undergo a reversal. Temperatures had mostly fallen, especially in the Arctic and the Atlantic sub-Arctic, where sea ice has been increasing. The circulation of the northern hemisphere appears to have shifted in a manner suggestive of an increasing amplitude of the planetary waves and of greater extremes of weather conditions in many areas of the world. (The situation in the southern hemisphere has not been so well documented.) These events have culminated, at times in the last several years, in the emergence of anomalous conditions in the monsoon belt of the tropics and in widespread drought in the Sahel zone of Africa and in northwest India (see Figure 2.6). To what extent these calamitous recent events are related to each other as manifestations of a globally coherent fluctuation of climate is not clear. In any event, they dramatize the fact that climatic variability, whether globally coherent or not, is to be expected no less on time scales of months and years than on time scales of centuries and millennia. An evident faltering of these tendencies of climate, in just the last five or ten years, attests also to the ephemeral nature of all climatic “trends.” Such is the nature of climate and climatic variations.
2.3
ORIGINS OF CLIMATE VARIABILITY
Mindful as we are of the remarkable variability of climate in the remote past, the lesser variability of present-day climate catches us by surprise only in the sense that we lack a satisfactory explanation for it, and we do not know how to
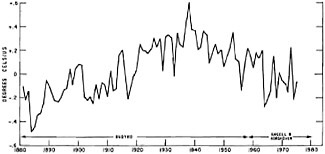
FIGURE 2.5 Recorded changes of annual mean temperature of the northern hemisphere as given by Budyko (1969) and as updated after 1959 by H. Asakura of the Japan Meteorological Agency (unpublished results).
Reference:
Budyko, M. I. (1969). The effect of solar radiation variations on the climate of the earth, Tellus 21, 611.
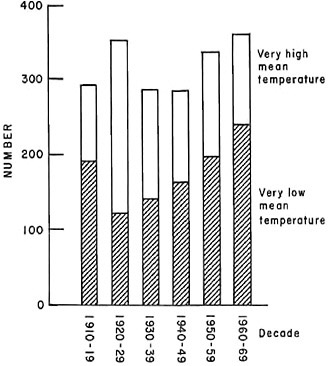
FIGURE 2.6 Total number of very low and very high monthly mean surface temperatures observed in each decade since 1910, at 65 stations distributed over the world. Length of each column indicates total number of temperatures in the decade that lie outside the range expected 90 percent of the time in all 60 years of record at each station. The hatched part of each column indicates total number of very low temperatures and the unhatched part the number of very high temperatures. Source: Japan Meteorological Agency (1974). Report of a Study on Recent Unusual Weather and Climatic Trend in the World and the Outlook for the Future. (Summarized edition, in English.) Mimeographed, 13 pp.
predict it in advance. Today, we find ourselves uneasy because through our use of energy we may be significantly disturbing the natural climate system. This uneasiness is justified. For surely we cannot hope to grasp all the ramifications of the forces we set in motion when we alter our atmospheric environment through the massive use of energy, if we fail to understand the source of natural climatic variations that will be taking place at the time. By the same token, if we fail to understand the source of natural climatic variations, how can we know when and whether our use of energy is responsible in any way for new developments in the climate that might be disadvantageous to society?
If we agree to the proposition that an understanding of natural climatic variability is a prerequisite to the intelligent assessment of the climatic impacts of energy use, we are faced with a problem: our understanding of natural climatic variability is not yet at hand, nor is it likely to be at hand for many years. In order to achieve it, an enormously difficult task lies before us in developing credible mathematical models of the climate system (see Figure 2.7). We cannot hope for success without a national and international commitment to devote the considerable resources of various kinds that are required to tackle so complex and so challenging a scientific problem.
At this stage, some assessment can nevertheless be made as to the nature of the problem to be solved, if we are to understand climatic variability and to predict it.
First, we can point to the fact that those parts of the climate system that we already understand—at least in principle—constitute a highly complex fluid-dynamical system, involving the coexistence of water in all three of its phases, in which there are many highly nonlinear interactions. While these nonlinear interactions are the source of many difficulties faced in developing successful climate models, they are also to be recognized as the source of the very climatic variability that we seek to understand.
Second, our knowledge of the climate system is thus far confined primarily to the atmospheric part of the system. While the atmosphere is perhaps the most complex part of the system, the atmosphere alone cannot account for the bulk of climatic variation, except on very short time scales. To take into account the longer time scales, it is necessary to consider the interaction of the atmosphere with other parts of the fluid envelope of the earth, which impart correspondingly longer relaxation phenomena to the climate system. To begin with, numerical simulation schemes (models) of the atmosphere-ocean-sea ice system are required, following the lead of some relatively crude models now under development. It is hoped that such models, although they admittedly do not contain all the elements of the complete climate system, will ultimately succeed in capturing the essence of climatic variability on time scales of months, years, and decades. It is possible that much of the variability on still longer time scales (centuries and perhaps even millennia) can also be captured in joint atmosphere-ocean-sea ice models that include deep-ocean processes.
Ultimately a capability to model climatic variability on the time scales of glacial-interglacial events would be highly desirable, although the relevance of such models to the problems of climate in the time frame of direct concern to us here may seem more remote. In any case, the modeling of climatic variability on such long time scales would require incorporation of ice-sheet dynamics into the climate system.
Present understanding of the causes of climate variability is meager at best. Most causal hypotheses fall into one of two schools of thought.
One school of thought holds that climate varies mainly as a result of environmental changes outside the climate system. A familiar example is the notion that climate responds in a more or less deterministic manner to variations of total solar irradiance, presumed but never proven to accompany the 11-year or longer sunspot cycles. It cannot be ruled out that such external influences on climate are real, but even if they are real there is much evidence to indicate that they can account only for a small fraction of the total variability of climate, the bulk of which requires a different explanation.
The other school of thought holds that most, if not all, of climatic variability originates from purely internal stochastic
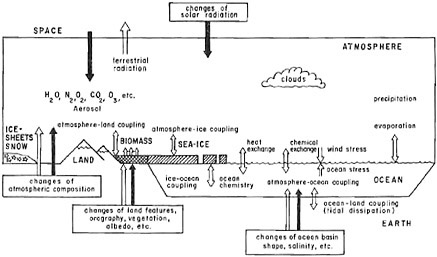
FIGURE 2.7 Schematic illustration of the components of the coupled atmosphere-ocean-ice-land surface-biomass climatic system. The full arrows are examples of external processes, and the open arrows
are examples of internal processes in climatic change. Source: GARP Publications Series No. 16, The Physical Basis of Climate and Climate Modeling, Report of the International Study Conference in Stockholm, 20 July-10 August 1974, World Meteorological Organization, International Council of Scientific Unions, Geneva, 1975.
variation of the climate system itself. Indeed, present and planned climate modeling efforts generally subscribe implicitly or explicitly to this view. This is as it should be, for it is only through experiments with climate models capable of dealing with internal stochastic processes that one can hope to gain a realistic measure of the capacity of the climate system to vary through internal dynamics as well as external forcing.
In this connection, it has to be emphasized that in a highly interactive system, which involves as wide a range of reaction times as that of the real climate system of the earth, many opportunities exist for the internal stimulation of slowly varying modes of system behavior. In the joint atmosphere-ocean part of the climate system, for example, oceanic thermohaline reaction times are likely to be one or more orders of magnitude slower than the slowest (thermal) reaction time of the atmosphere. Under these circumstances, the stimulation of what are essentially random variations of the ocean part of the system would, to the extent that such variations are transmitted to the atmospheric part of the system, give rise to what are perceived as nonrandom variations in the atmosphere. There is ample reason to believe that interannual variations of climate to a considerable extent owe their existence to ocean-atmosphere interactions of this “random” form. More generally, what may appear to be a nonrandom form of variability in one part of the climate system may be recognizable as having its origin in a strictly random (albeit dynamically constrained) form of variability in another part of the system. If such internal sources of climatic variability are not recognized for what they are, the variability might easily be mistaken for an effect of variable external forcing.
It is conceivable that all climatic variations, even including the ice ages, owe themselves to “random” processes in those parts of the climate system that possess extremely slow reaction mechanisms. The plausibility of this view of climatic variation, together with its implications for climate predictability, remains to be explored.
2.4
A LOOK TO THE FUTURE
Our very superficial understanding of climate dynamics translates to a very modest capability for prediction of climate. One of the principal motivations for climate-dynamics research is to gain enough insight into climate system behavior to enable the skillful prediction of climate. Yet, it has to be recognized that our understanding is not yet adequate to assure us that climate can be predicted with practically useful skill for an extended period of time into the future.
In the face of the many urgent societal needs for information about future climates, it seems appropriate to inquire further into the present situation with regard to climate prediction.
On the one hand, it can be observed that the pioneers in the field of modern climate-dynamics research have been reluctant to engage in any kind of speculation about future climatic developments. This is so despite the fact that those pioneers are probably in the best position of all to speculate intelligently about the future. This reticence is not difficult to understand. It is born of a healthy respect for the limitations of present generations of climate models, which necessarily omit too much of the physics that they will eventually have to include before real-time predictions are likely to bear much relationship to reality.
On the other hand, one can point to the fact that in the last 20 years or so, a number of deterministic long-range projections of climate have found their way into the scientific literature. The authors of such projections have based them on various kinds of simplistic reasoning, sometimes involving “blind” statistical extrapolation of what are essentially unverified periodicities in past climate and other times involving certain external physical processes (e.g., solar activity) that they have supposed to have an overriding influence on the course of global climate. It cannot be flatly asserted that all of these projections are without merit. Unfortunately, however, where merit exists it will be likely
to remain obscure until climate-dynamics research finally progresses to a point where it can confirm that merit. By then, the modeling framework available to illuminate climatic dynamics would make possible a much more comprehensive assessment of reality.
If society is to embrace any deterministic climate projection as a basis for sound future planning, it should be clear that two criteria have to be met. First, the scientific basis of the projection must be rational. Second, the projection must be accompanied by a suitably objective and unbiased measure of its own reliability. No deterministic projections of future climate now available can be said to meet both of these criteria.
Making reliable projections of future climate of any kind will likely remain an elusive goal until our knowledge of climate dynamics is adequate to provide the answers to two sets of fundamental questions.
The first set of fundamental questions to be answered concerns the natural climate system: (1) Were man’s various impacts on environment to be held in the future at their present-day levels, how would the earth’s climate evolve from its present state in the decades and centuries ahead? (2) Is the answer to (1) knowable? Or is it the case that the natural evolution of climate is a probabilistic process in time, such that, after a relatively short interval, the state of global climate will be essentially unrelated to its present state? (3) To the extent that the answer to (1) is knowable, to what further extent is it within man’s power to find that answer?
The second set of fundamental questions to be answered concerns man himself in relation to his potential capacity for interfering in the workings of the natural climate system: (4) On the basis of each of a variety of credible alternative scenarios as to future increases and proliferation of his impacts on environment, can man assess the consequences of those changing impacts on the natural evolution of climate in the decades and centuries ahead? (5) Can man establish which, if any, alternative scenarios would lead to “unacceptable” climatic consequences and are therefore to be avoided? (6) If the answer to (5) is yes, will man come into possession of such knowledge in time to avert possible calamity?
The very essential matter of climate predictability is addressed by question (2). Should climate turn out to be inherently unpredictable after a short interval of future time, then the capacity of science ever to provide answers to the other questions posed here will be limited, perhaps severely so.
Up to this point we have mainly been referring to deterministic projections of climate, by which we mean statements as to the “expected” climate in a specific future interval of time or in each of a series of future intervals. Also to be considered are probabilistic projections of climate, in which the conditions to be projected for specified future intervals of time are not the “expected” climate as such but rather the probability density distribution of the full range of outcomes of climate that the statistics of past climates indicate to be possible. Such probabilistic projections are normally assessed through statistical inference rather than physical inference and are based essentially on past experience rather than physical understanding with regard to climatic variability. Projections of this kind are always meaningful, in the sense that even if no organized time-series structure whatever is evident in past climatic behavior, future projections can still be made. In that case, the projections would reduce to unconditional statements to the effect that the future probability of any given climatic condition (at any time in the future) is identical to that of the same climatic condition in the past.
The record of past climates deserves our very close scrutiny, to learn the full extent of organization in the time-series structure of climatic variations that could be used to frame improved probabilistic projections of future climate. To date, surprisingly little has been done along these lines.
Viewing past climates in the perspective of events of the past million years, we can at least venture the conclusion that, in the long run, natural forces will be more likely to relax our global climate toward a glacial condition than to maintain climate indefinitely in its present, unusually warm interglacial condition. When the return to a glacial condition may begin and at what rate global climate will be altered when it does begin are by no means obvious from the paleoclimatic record.
Viewing past climates in the somewhat shorter perspective of the postglacial period, the sequence of “neoglacial” events appears to be a dominant feature. It would be tempting to suggest that the last neoglacial event, the “Little Ice Age” of the fifteenth to nineteenth centuries, is behind us and that the next comparable event will not arrive for another thousand years or more. On the other hand, we should keep in mind evidence that the Little Ice Age was appreciably shorter than earlier neoglacial events. This invites an alternative view of the comparative warmth of the twentieth century, as being not a recovery from the Little Ice Age but perhaps only an interruption of it.
Beyond such vague statements as these, disturbingly little can be said about the probable course of natural climate in the decades and centuries ahead. Moreover, a number of man’s activities are being ingested by the climate system, adding further uncertainty to an already uncertain future of climate. The need for better understanding of the climate system, in all its aspects, is a clear and present one.