4
Farm-System Dynamics and Social Impacts of Genetic Engineering
The dissemination of genetically-engineered (GE) crops, like the adoption process associated with other farm-level technologies, is a dynamic process that both affects and is affected by the social networks that farmers have with each other, with other actors in the commodity chain, and with the broader community in which farm households reside. As noted in Chapter 1, farmer decisions to adopt a technology are influenced not only by human-capital factors, such as the educational level of the adopter, but by social-capital factors, such as access to information provided by other farmers through social networks (Kaup, 2008). That necessarily implies that farmers receive information from others—for example, on the risks and benefits of a particular technology—and that they share their own knowledge and experience through the same networks. Such findings confirm the relevance of social factors in influencing how genetic-engineering technology is adopted, what the impacts of its adoption are, and the significance of farmers’ active participation in both formal and informal social networks with other actors in commodity chains and communities.
However, little research has been conducted on the social impacts of the adoption of genetic-engineering technology by farmers, even though there is substantial evidence that technological developments in agriculture affect social structures and relationships (Van Es et al., 1988; Buttel et al., 1990). Because further innovations through genetic engineering are anticipated, such research is needed to inform seed developers, policy makers, and farmers about potential favorable benefits for adopters
and nonadopters and unwanted or potentially unforeseen social effects (Guehlstorf, 2008). With such information, the likelihood of maximizing social benefits while minimizing socials costs is increased. To demonstrate the necessity for increasing commitments to the conducts of research on the social effects of GE-crop adoption, this chapter synthesizes what is known in the scientific literature about the social impacts of farm-technology adoption and the interactions between farmers’ social networks. The chapter also identifies future research needs.
SOCIAL IMPACTS OF ON-FARM TECHNOLOGY ADOPTION
The earliest academic research in the United States on the social impacts of technology adoption at the farm and community levels was focused on mechanical technologies. More than a century ago, the use of machines in U.S. agriculture not only displaced labor but widened socioeconomic discrepancies between skilled and unskilled laborers (Quaintance, 1984). Academic interest in the socioeconomic consequences of agricultural mechanization was particularly strong in the 1930s and 1940s in the southern United States (Buttel et al., 1990) and again in the 1970s throughout the country. Berardi (1981) summarized the findings of the literature and found that mechanization was associated with decreases in the agricultural labor force, particularly those among the least educated and least skilled workers and in minority groups; with better working conditions and less “drudgery” for the remaining work force; with a decrease in farm numbers and an increase in farm size; with increased capital costs for agricultural producers; and with a decline in the socioeconomic viability of agriculture-dependent rural communities. Data also suggested that the technological development of U.S. agriculture had contributed to declines in farm labor, in community dependence on agriculture, and in rural community viability although other on-farm and off-farm factors also contributed to these changes (Van Es et al., 1988).
In the 1980s, social scientists broadened their research on the impacts of technology adoption on farms and farm communities to include studies of the potential and actual impacts of biological (pregenetic engineering) technologies in agriculture. Many observers assumed that, unlike the earlier wave of mechanical agricultural technologies, genetic-engineering technology would not be biased towards large-scale farming operations. Such an assumption was supported by analyses of the production capabilities of agricultural biotechnology. For example, it was noted that no interaction effect was observed between genetic predisposition to produce milk and the use of the growth hormone bovine somatotropin (BST) to increase milk production in dairy cows (Nytes et al., 1990). However,
other studies that directly examined farm-level social change revealed that, despite the presumption of scale-neutrality, it was difficult to isolate the impacts of biological innovations from those of other technological innovations in agriculture because biological innovations were often developed and disseminated in conjunction with other technologies that may not have been scale-neutral (Kloppenburg, 1984).
Additional research conducted on the social impacts of biotechnology in animal agriculture, specifically on the use of BST, noted that rates of adoption of BST were moderate and that, although adoption did not require large herds, scale effects were observed because BST use was more effective in high-producing cows, which were more likely to be found in large herds with complementary feeding technologies (Barham et al., 2004). Beck and Gong (1994) also observed the existence of a scale effect with adoption of BST, with adopters more likely to have larger herds, as well as being younger and having more formal education. Additionally, it was suggested that the quality of farm management had an impact on the benefits accruing to the adoption of BST (Bauman, 1992). The use of BST also was thought to lead to lower prices and thus to result in increased economic pressure on smaller producers (Marion and Wills, 1990). In other words, the body of research on the socioeconomic consequences of the use of biotechnologies, including Green Revolution technologies, indicated that “scale neutrality is not inevitable, but a possibility that depends on institutional context” (DuPuis and Geisler, 1988: 410). To put it another way, the social context of the adoption process and the impacts on that context are interconnected, from which it follows that the social impacts of genetic-engineering technology on farms and communities differ among cultures, commodities, and historical periods.
Thus, though seed varieties are generally conceptualized as being scale-neutral, the adoption of any technology may be biased toward large firms that can spread the fixed costs of learning over greater quantities of production (Caswell et al., 1994). In developing countries, the economics of genetic-engineering technology do not appear to vary with farm size (Thirtle et al., 2003). However, scale may affect accessibility to technology. Small farmers have less influence in input supply and marketing chains with which to secure access to desired technologies. Thus, there can be a scale bias in the development and dissemination processes associated with herbicide-resistance technology that puts small farmers at a disadvantage. In contrast, as noted in Chapter 3, insect-resistance technology can replace insecticide applications that require fixed capital investments, such as for tractors and sprayers. In this regard genetic-engineering technology has the potential to favor small farmers, who would benefit more from a technology that required less fixed capital investment. The scale effects of transgenic varieties may also depend on the pricing (such as quantity
discounts) set by seed companies, which typically assess a technology-user fee.1
An early empirical study was carried out by Fernandez-Cornejo et al. (2001) using 1998 U.S. farm data. They found that, as expected, the adoption of HR soybean was invariant to size, but adoption of HR corn was positively related to size. They explained this disparity as due to the different adoption rates: 34 percent of the farms had adopted HR soybean at the time, implying that adoption of HR soybean had progressed passed innovator and early-adopter stages into the realm where adopting farmers are much like the majority of farmers. On the other hand, adoption of HR corn was quite low at the time (5 percent of farms), implying that adoption was largely confined to innovators and other early adopters who in general tend to control substantial resources and who are willing to take the risks associated with trying new ideas. Thus, they claimed that the impact of farm size on adoption is highest at the very early stages of the diffusion of an innovation (HR corn), and becomes less important as diffusion increases. This result confirms Rogers’s (2003) observations that adoption is more responsive to farm size at the innovator stage, and the effect of farm size in adoption generally diminishes as diffusion progresses. Early adopters, by virtue of early adoption, also are able to capture a greater percentage of the economic benefits of the technology adoption process.
Clearly, one cannot extrapolate the social impacts of the adoption of GE crops based solely on an assumption that the productive capabilities of genetic-engineering technology, when isolated from the interaction with other factors, should be scale-neutral. In other words, previous research on the social impacts of agricultural technologies suggests the possibility that the early dissemination of genetic-engineering technology would be associated with farm size, and that the use of GE crops could have differential impacts across farm types, farm size, and region, despite the fact that GE crops are presumed to be scale-neutral.
In an article that attempted to predict some of the environmental, economic, and social effects of genetic engineering of crops, it was argued that the use of GE crops was “clearly capable of causing major ecological, economic, and social changes” (Pimentel et al., 1989: 611). Nonetheless, over the last decade, there has been virtually no empirical research conducted on the social impacts of the use of GE crops on farms and rural communities. The lack of research may have to do in part with the scarcity of funds available for such research as well as a relative lack of interest in social issues on the part of environmental groups (Chen and
1 |
Examples of empirical studies on the effect of farm size on GE-crop adoption are given in “An Early Portrait of Farmers Who Adopt Genetically Engineered Crops” in Chapter 1. |
Buttel, 2000), and other groups and organizations that might be expected to support such research. Nonetheless, the results of research referred to above on the social repercussions of agricultural technologies, including non–genetic-engineering biotechnology in crops and biotechnology in animal agriculture, would suggest that there are impacts, that these impacts could be favorable or adverse, and that adverse impacts could be alleviated through the adoption of appropriate policies. For example, based on earlier research on the introduction of new technologies in agriculture, it might be hypothesized that certain categories of farmers (those with less access to credit, those with fewer social connections to university and private sector researchers, etc.) might be less able to access or benefit from existing GE crops. There is also the possibility that the types of genetic advances being marketed do not meet the needs of certain classes of farmers, and that the full spectrum of the potential of genetic-engineering technology is not being achieved. Furthermore, the possibility exists that communities where farmers play an important social, political, and economic role could be impacted as well. However, for the purpose of this report, no conclusion on the social impacts of the adoption of GE crops can be drawn on the basis of empirical evidence. Research on such impacts clearly should be accorded a high priority as genetic-engineering technology evolves. Without such research, the potential for genetic-engineering technology to contribute to the sustainable development of U.S. agriculture and rural communities cannot be adequately assessed. Thus, we recommend that such research be sponsored and pursued actively and immediately.
SOCIAL NETWORKS AND ADOPTION DECISIONS
The adoption of genetic-engineering technology and its performance on the farm are functions of the knowledge of agricultural decision makers, who include farmers, input suppliers, commodity traders, farm-management consultants, and extension agents. In making technology-adoption decisions, farmers rely principally on information about the relative performance of competing technologies and on information about best practices for optimizing yields and controlling costs, given the technologies that they use. The performances of firms and technology, therefore, depend upon the information used by various commodity-system actors. Just et al. (2002) have shown that the internal competences of decision makers affect the degree to which they rely on different types and sources of information.
Farmers rely on a variety of intermediaries—such as extension agents, commodity groups, commercial vendors, agricultural media, and other farmers—for information. For example, farmers often turn to commodity
associations for information about regulations and regulatory changes. Many of the intermediaries that farmers communicate with use public information, especially data and research results provided by the U.S. Department of Agriculture (USDA) Economic Research Service and the National Agricultural Statistics Service and by state extension services, particularly for information about the economic outlook of agriculture and specific industries. Intermediaries use formal channels of information more than farmers (Just et al., 2002) and then make that information available to farmers.
Farmers obtain about half their information from informal sources (i.e., sources whose professional duties do not include provision of information) (Just et al., 2002), including people in the end-users’ civic, community, professional, and commercial networks, like neighbors, colleagues, customers, and suppliers. Farmers’ reliance on informal sources may reflect low availability of or access to information from formal channels, issues of affordability of private information, and credibility (Just et al., 2002).
Those findings suggest that farmers’ attitudes toward GE crops are likely to be affected by a number of information providers. USDA’s Cooperative Extension Service, commodity groups, and agricultural media are particularly influential in informing farmers’ views on the technical aspects of genetic-engineering technology, its economic implications, and its prospects. Although the influence of those sources has not been widely appreciated, they have played a key role in the adoption of the technology. As Wolf et al. (2001) and Just et al. (2002) demonstrated, informal sources of information are just as likely as formal sources to accelerate or to slow the rate of GE-crop adoption. It would also be reasonable to hypothesize that patterns of information use would be linked to the ability of farmers to use the technology effectively and maximize its potential.
INTERACTION OF THE STRUCTURE OF THE SEED INDUSTRY AND FARMER DECISIONS
The U.S. seed industry has experienced extensive structural change in the last few decades. The changes have affected decisions at the farm level by shaping the choices available to corn, soybean, and cotton farmers.
As Fernandez-Cornejo and Just (2007) have summarized, plant-breeding research until the 1930s was conducted primarily by the public sector (for example, USDA and state agricultural experiment stations), and most commercial seed suppliers were small, family-owned businesses that multiplied seed varieties that had been developed in the public domain. Seeds embody the scientific knowledge needed to produce a new plant variety with desirable attributes—such as higher yield, disease or
pesticide resistance, or improved quality—so seed innovators face both the risk of imitation by competing seed firms and the risk of seed reproduction by farmers themselves (Fernandez-Cornejo, 2004). The development of hybrid corn in the first half of the 20th century provided breeders with greater protection of intellectual-property rights (IPR) because seeds saved postharvest produced substantially smaller yields than the hybrid plants from which they were gathered. With that incentive, the number of private firms engaged in corn breeding grew rapidly.
The proliferation of firms was followed by consolidation in part because U.S. law evolved to provide incentives to innovators for research and development by giving them exclusive control of their innovations through patent laws and other forms of enforceable legal protection (Fernandez-Cornejo, 2004). Two principal forms of legal protection for seed innovators are plant variety protection (PVP) certificates issued by the Plant Variety Protection Office of USDA and patents issued by the Patent and Trademark Office (PTO) of the U.S. Department of Commerce. Both grant private crop breeders exclusive rights to multiply and market their newly developed varieties. Patents provide more control because PVP certificates have a research exemption that allows others to borrow a new variety for research purposes (Fernandez-Cornejo and Schimmelpfennig, 2004). IPRs for seed innovators were strengthened by the U.S. Supreme Court’s 1980 Diamond v Chakrabarty decision, which extended patent rights to GE microorganisms, important tools and products of biotechnology. A series of rulings by PTO’s Board of Appeals and Interferences widened the scope of patent protection for GE organisms by including plants and nonhuman animals. Those rulings extended IPRs to a wide array of new biotechnology products in the form of utility patents (also referred to as patents for invention). Products protected under the rulings include seeds, plants, plant parts, genes, traits, and biotechnology processes (Fuglie et al., 1996; Fernandez-Cornejo, 2004).
Enhanced IPR protection has brought rapid increases in private research and development (R&D), and indirectly assisted technology developers in setting prices above marginal costs (Goldsmith, 2001). Private spending on R&D in crop varieties increased by a factor of 14 in real terms from 1960 to 1996 (Fernandez-Cornejo, 2004), whereas public (federal and state) spending changed little (Figure 4-1; Fernandez-Cornejo and Schimmelpfennig, 2004). At the same time, IPR protection may have spurred market concentration in the seed industry. The potential profits of seed firms made possible through IPR protection may strengthen the incentive to invest and thus provide greater opportunities to large firms (Lesser, 1998). Many seed firms have been acquired by corporations that have the resources needed to achieve large economies of scale in R&D (Fernandez-Cornejo, 2004). For example, Lesser (1998) stated that more
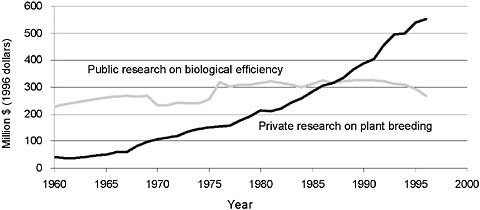
FIGURE 4-1 Public and private research expenditures on plant breeding. Biological efficiency includes breeding and selection of improved plant varieties.
SOURCE: Fernandez-Cornejo, 2004.
than 50 seed firms were acquired by pharmaceutical, petrochemical, and food firms after the passage of the Plant Variety Protection Act.2 In contrast, Lesser (1998) also noted that weakness of IPR protection may lead to mergers and acquisitions. In any case, by 1997, the share of U.S. seed sales controlled by the four largest firms reached 69 percent for corn (up from 60 percent 1973), 47 percent for soybean (up from 7 percent in 1980), and 92 percent for cotton (up from 74 percent in 1970) (Table 4-1; Fernandez-Cornejo, 2004). Though it is difficult to obtain recent detailed published market share information, it appears from company reports and other sources that the trend of increased concentration in the structure of the seed industry continued in recent years.3 Farm survey data for corn and soybean indicated that by 2007 the share of the four largest firms reached 72 percent for corn and 55 percent for soybean (Figure 4-2; Shi and Chavas, 2009).
Concentration of R&D output can also be used to measure the concentration in innovation activity in the seed industry (Fulton and Giannakas, 2001). In genetic-engineering technology, a measure of R&D output is the
TABLE 4-1 Estimated Seed Sales and Shares of Major Field Crops, United States, 1997
Company |
Total ($ billions, current) |
Corn Market Share |
Soybean Market Share |
Cotton Market Share |
Percentage of Acres |
||||
Pioneer Hi-Bred International |
1.18 |
42.0 |
19.0 |
— |
Monsanto/Stoneville |
0.54 |
14.0 |
19.0 |
11.0 |
Novartis/Syngenta |
0.26 |
9.0 |
5.0 |
— |
Delta & Pine Land |
0.08 |
— |
— |
73.0 |
Dow Agrosciences/Mycogen |
0.14 |
4.0 |
4.0 |
— |
Golden Harvest |
0.09 |
4.0 |
— |
— |
AgrEvo/Cargill |
0.09 |
4.0 |
— |
— |
Others |
1.12 |
23.0 |
53.0 |
16.0 |
Total |
3.50 |
100.0 |
100.0 |
100.0 |
SOURCES: Hayenga, 1998; Fernandez-Cornejo, 2004. |
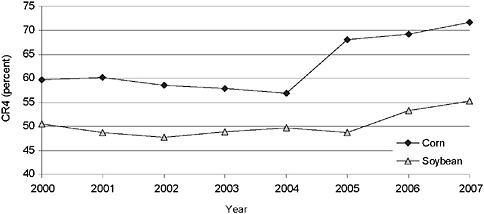
FIGURE 4-2 Share of planted acres of corn and soybean seeds by largest four firms (CR4).
SOURCE: Stiegert et al., 2009.
number of GE cultivars approved by USDA for release into the environment for field testing. In particular, Fernandez-Cornejo (2004) adapted the four-firm concentration-ratio measure, commonly used to quantify industry concentration in terms of sales, to examine R&D concentration on the basis of regulatory approvals of GE crop varieties. Table 4-2 shows the percentage of field releases obtained by the leading four firms
TABLE 4-2 Four-Firm Concentration Ratio in Field-Release Approvals from USDA Animal and Plant Health Inspection Service, by Crop, 1990–2000
|
1990 |
1991 |
1992 |
1993 |
1994 |
1995 |
1996 |
1997 |
1998 |
1999 |
2000 |
Corn |
67 |
67 |
65 |
82 |
82 |
67 |
60 |
73 |
73 |
80 |
79 |
Soybeans |
100 |
100 |
94 |
68 |
72 |
94 |
82 |
82 |
71 |
87 |
85 |
Cotton |
100 |
100 |
100 |
89 |
79 |
85 |
91 |
64 |
98 |
98 |
96 |
SOURCE: Fernandez-Cornejo, 2004. |
in 1990–2000. The top four firms controlled well over 50 percent of the approvals; this suggests consolidation in R&D and potential barriers to entry for competitors. As Fulton and Giannakas (2001) noted, expenditures on R&D and expenditures made to obtain regulatory approvals are sunk costs—costs that cannot be recouped. If such sunk costs are present, markets are not contestable, so there are potential barriers to entry.4
As Fernandez-Cornejo (2004) observed, on the basis of the four-firm concentration ratio of approvals, the extent of corn-seed R&D concentration has been relatively constant at around 72 percent, which is fairly consistent with the four-firm concentration ratio in corn in terms of sales. Cotton-seed R&D is the most centralized, and this is also consistent with market-concentration measures.
Patent ownership shows a pattern of concentration similar to that evident in other R&D measures (Fernandez-Cornejo, 2004). Most of the biotechnology patents awarded to private firms are held by a small number of large companies. As of 1996–1997, Pioneer (soon after DuPont/Pioneer) held the largest number of patents for corn and soybean, followed by Monsanto (Brennan et al., 1999). The leading firms in the sector have received IPR protection not only by virtue of their respective R&D investments but through mergers and acquisitions. For example, Pioneer was one of the first four companies active in the emerging corn-seed market in the early 1930s. As shown in Figure 4-3, Pioneer (Pioneer Hi-Bred International, Inc.) made a series of acquisitions in 1973–1980 that strengthened its overall position in the seed market. The chemical firm DuPont bought 20 percent of Pioneer in August 1997 and bought the remaining 80 percent in 1999 for $7.7 billion. As a DuPont company, Pioneer continues to operate under the Pioneer name and remains headquartered in Iowa (Fernandez-Cornejo, 2004).
Although the increase in seed-industry concentration has raised concerns about its potential impact on market power, and ultimately on
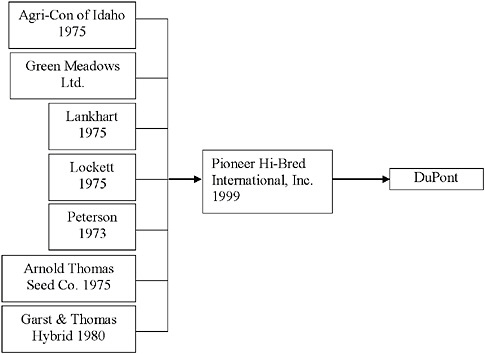
FIGURE 4-3 Evolution of Pioneer Hi-Bred International, Inc. / E.I. du Pont de Nemours and Company.
SOURCE: Fernandez-Cornejo, 2004.
the sustainability of farms, empirical results for U.S. cotton and corn seed industries over the period covering 1970–1998 (which includes only 2 years of GE-crop adoption) suggest that increased concentration during that time period resulted in a cost-reducing effect that prevailed over the effect of enhanced market power (Fernandez-Cornejo, 2004). Goldsmith (2001) argued that even though GE-seed prices were above the competitive price, the actions of biotechnology supply firms apparently were not adversely affecting the welfare of U.S. farmers.
However, concerns have been raised that, in time, such market power could lead to decreased variability in the types of seeds being produced for the market, as well as increased prices, which could limit the ability of farmers to purchase those seeds most suited for local environmental conditions. In addition, it is conceivable that the continued market power of biotechnology supply firms could lead to increased input costs for farmers, which in turn could have an unfavorable effect on the socioeconomic sustainability of farms. A recent study by Shi and Chavas (2009) has found that vertical integration (ownership of control of different
stages of production) in the U.S. soybean seed industry had a substantial effect on soybean prices. Shi et al. (2008) analyzed the pricing of corn seed with stacked traits for the years 2000–2007 and found “significant departures from component pricing (where seeds are priced as the sum of their component values). The evidence supports sub-additive pricing. It shows that the marginal contribution of each component to the seed price declines with the number of components.” The authors also have indicated that “such a finding is consistent with the presence of economies of scope in seed production. Indeed, synergies in R&D investment (treated as fixed cost) across seed types can contribute to reducing total cost.”
In response to these concerns and others, USDA and the U.S. Department of Justice launched a series of workshops in 2010 to examine competition and regulatory issues in the agriculture industry (USDA, 2010). This is a first step towards updating and continuing research on how market structure in the seed industry may be impacting seed prices and availability to variability in genetic resources. In addition, studies of how seed-industry concentration, as well as the practice of cross-licensing, could interact with farmers’ planting options and decisions, overall yield benefits, crop genetic diversity, and economic returns would be very valuable.
Although the private sector owns the majority of agricultural-biotechnology patents, the public sector still owns a substantial share. In a study of assignment of U.S. plant-biotechnology patents granted from 1982 to 2001, Graff et al. (2003) found that 41 percent of the patents were owned by large biotechnology companies, 35 percent by startups, and 24 percent by the public sector. The public-sector ownership is weaker in some categories, such as Bt and other insect-resistant traits (10 percent) and plant enzymes (8 percent), but stronger in other categories (42 percent in flowering and 56 percent in pathogen resistance). The capacity of the public sector to obtain freedom to operate in transgenic crops is often also constrained by the fragmentation of technology ownership among numerous institutions. Improved access to information about IPRs and reduced transaction costs to obtain rights to use patents could increase the public sector’s contribution to the development of transgenic varieties. Concerns have also been raised that technology use and stewardship agreements prevent scientists in the public sector from conducting independent assessments of GE varieties marketed by the private sector. In February 2009, in response to a notice in the Federal Register on a meeting of the Federal Insecticide, Fungicide, and Rodenticide Act Scientific Advisory Panel, 26 entomologists submitted a general comment that, because of those restrictions, the data that the Environmental Protection Agency received regarding GE crops were inherently limited (US-EPA, 2008). If such restrictions exist, farmer welfare could be adversely affected
by the lack of complete information regarding GE traits or crops. However, the degree to which technology use agreements may hamper public research is unclear and strongly disputed by private-sector seed companies (Monsanto, 2010). This issue merits careful investigation by neutral researchers to understand what, if any, effects such agreements have on public research.
SOCIAL AND INFORMATION NETWORKS BETWEEN FARMERS AND INDUSTRY
Agriculture is unique among American industries in that federal law allows farmers to cooperate in some collective activities while competing in the output market. That has enabled farmers to act collectively as a counterweight to the large firms on which they rely to sell their output (Cochrane, 1993). Farmers have developed cooperatives to coordinate research and marketing efforts to benefit from economies of scale in these activities (Sexton, 1986). That collective-action capacity is important for adoption of genetic-engineering technology in that it gives farmers the ability to influence crop traits that are introduced.
Farmers may attempt to block seed technologies if they anticipate that those new technologies will not enhance, or perhaps even endanger, farm profit. Innovation in any crop can increase farm revenue if the elasticity of demand for the crop is sufficiently high that increases in output compensate for decreases in prices. But if the elasticity of demand is low, increased output may lead to a fall in revenue and a decrease in profit unless the technology delivers cost savings. In the latter case, farmers may attempt to block the introduction of a technology. Farmers may also block a technology if its introduction would result in lower prices in national or international markets.
A large body of literature on the political economy of research argues that farmers use political pressure to shape public research funding. Ruttan (1982) argued that farmer pressure may have led to underinvestment in public research in the United States, and de Gorter and Zilberman (1990) linked overall spending on research to the political power of such groups as consumers and producers. Graff and Zilberman (2007) argued that farmers’ interests partly motivated Europe’s effective ban on GE crops, which in turn affected the access of U.S. farmers who were growing GE crops to European markets.
Another example of farmers’ collective action is how farmers’ concerns influenced Monsanto’s decision to halt its efforts to introduce and market herbicide-resistant (HR) wheat. Some farmer coalitions in the United States and in Canada played a role in that decision because they feared losing access to European and Asian markets that would not accept
HR wheat. It was thought that the introduction of that GE crop might have closed markets to U.S. and Canadian farmers who planted non-GE wheat because of the difficulty in segregating wheat on fields and in grain elevators and trucks (e.g., Anonymous, 2001; Pollack, 2004). Many international buyers, including millers and bakers in important Asian and European markets, had made it clear that they wanted to purchase identity preserved (IP), non-GE commodities (Vandenberg et al., 2000). In the case of white wheat, Japan and South Korea, two countries that have GE-food labeling laws, were importing more than one-fourth of U.S. white wheat exports (Squires, 2004). Japanese millers and bakers were well aware that a large percentage of Japanese consumers were expressing negative attitudes toward the use of genetic-engineering technology in foods and believed that their government’s regulation of GE food was too lax (Toyama et al., 2001). Indeed, Japan was the United States’s largest market for non-GE corn and soybean: Nearly 90 percent of the IP, non-GE corn and soybean produced in the United States was being exported to Japan (Wilson et al., 2003). Faced with such demands from the market-place and with farmer concern about how those demands might influence sales in those markets, Monsanto decided to defer the introduction of HR wheat in 2004.
However, farm organizations are not monolithic; indeed, the issue of HR wheat divided the farming community (Graham and Martin, 2004). Even after Monsanto chose to suspend its HR-wheat program, wheat producers and support groups, such as the National Association of Wheat Growers, exhibited a strong interest in glyphosate-resistant wheat (Jussaume et al., 2004). Monsanto’s HR trait had been inserted into only one variety of wheat grown in a subset of U.S. and Canadian regions. It was opposed primarily by farmers who did not plant the potential HR variety and by some farmers who could have planted it but were afraid of losing access to European and Asian markets. Furthermore, during its deliberations, the present committee heard that North Dakota wheat farmers who were against the introduction of HR wheat because of concern about losing market access in Europe may now believe that they are disadvantaged by not having HR varieties and would like to see government and industry action that would lead to its development (Wilson, personal communication). Pest pressure is heterogeneous among growing regions, so support for or opposition to damage control through GE traits will vary according to farmers’ abilities to benefit from them. Thus, the potential for collective action to restrain the power of the seed industry is a function of farmers’ common interests, which are often variable.
Farmer cooperatives may also undertake efforts to bring about the introduction of seed traits that the private sector, for economic or other reasons, is not motivated to introduce. They may pool resources to under-
take their own research or to defer the regulatory and development costs that private firms face when they introduce new seed technologies. Farmers have worked with universities to introduce new technologies (Bradford et al., 2006), and similar collaborations could be effective in the development of genetic-engineering technology. In California, grape growers have suffered considerable losses from Pierce’s Disease, so they have contributed funds to the Public Intellectual Property Resource for Agriculture to support research for a genetic-engineering solution to the problem (PIPRA, 2006). More generally, pooled funds from farmers can lead to the introduction of desired traits for specialty crops when private seed companies lack the incentives to develop the traits alone (for more discussion of seed-access issues, see Chapter 3). As the price of wheat goes up, for instance, farmers in some regions may approach companies or universities to develop seed varieties to address specific constraints on productivity (Wilson, personal communication). They may also work with initiatives like the Specialty Crop Regulatory Assistance program, a fledgling collaboration of the federal government, scientists in public universities and the private sector, and farmers designed to assist technology developers in negotiating GE specialty crops through the requirements and expense of the regulatory process.
Despite the ability of farmers to organize collectively to counter-balance seed companies and processors, some farmers are concerned about the evolution of seed-technology innovation from a public good to a private good that is controlled by firms that have market power derived from patents on specific products. Innovation in most agricultural inputs is embodied in the technology, as in tractors and fertilizers. The technology is developed and sold by the private sector. But because it had historically been difficult to capture benefits from research efforts in seed technology before the advent of hybrid corn seed, the private sector underprovided seed-technology innovation, and the public sector took the lead in providing improved seed varieties in many crops (especially wheat, soybean, cotton, barley, and oat). Consequently, farmers who grew those crops—particularly wheat, barley, and oat—became accustomed to free or low-cost access to seed, and some farmers may consider open access to seeds a right.
In the case of GE seed, however, companies can make use of patent protection to enforce contracts that disallow reuse of the seed grown in farmers’ fields. Farmers must instead purchase seed from firms to reward their research investment and effort. The patents enable the private sector to set prices for the protected technology and to restrict the flow of knowledge. The private sector had already begun to invest more in seed technology for major crops such as corn in the 1930s and soybean in the 1970s. Because of the difficulty and expense of removing lint from the seed,
cotton farmers had traditionally purchased their seed from ginners and seed distributors. Consequently, the introduction of GE seeds by the private sector and the patented nature of the technology in the case of commercially available corn, soybean, and cotton may not have appeared to be strikingly different from the established relationship between seed companies and farmers of those commodities.
However, the developmental trajectory of GE-seed technology is leading to concern that access to seeds without GE traits or to seeds that have only the specific GE traits of particular interest to farmers may become increasingly limited. Additional concerns are being raised about the lack of farmer input and knowledge regarding which seed traits might be developed. The push to develop seed varieties with a series of stacked traits, some of which may not be of use to some farmers with respect to short-term productivity (leaving aside the issue of improved resistance management discussed in Chapter 2), raises the issue of access to seeds that have equivalent yield potential but only the desired GE traits or no GE traits at all. Although the committee was not able to find published research that documents the degree of U.S. farmers’ access to and the quality of non-GE seed, testimony provided to the committee suggested that access to non-GE or nonstacked seed could become limited for some farmers and that available non-GE or nonstacked seed may not have the same yield characteristics as GE cultivars (Hill, personal communication). Research is needed to investigate the extent to which U.S. farmers are having difficulty purchasing high-yielding, non-GE seed. Public-sector institutions could address this concern by improving the design of licensing contracts with seed companies so that property rights of privately developed traits or cultivars will revert to university research programs if private companies do not use the technologies.
Boehlje (1999) has suggested that U.S. agriculture is going through a structural change in which activities that will enhance product differentiation and added value to farming are being emphasized. As part of that evolution, many agricultural sectors (poultry, swine, and some fruits and vegetables) have come to be dominated by contracting arrangements between major agribusiness companies and farmers or by large vertically integrated agribusiness firms. Those large companies have the resources and scale to finance research in the development of GE traits. The emergence of alliances between biotechnology companies and large agribusiness firms, and even large farmers’ cooperatives to produce proprietary GE varieties, appears possible, but future research is needed to determine whether such relationships can lead to the development of differentiated products (Boehlje, 1999)—including those with traits that enhance direct value to consumers, such as improving health or convenience, or that respond to the environmental and management needs of
specific groups of farmers—and whether such relationships will limit farmers’ access to the types of GE traits they value.
INTERACTIONS OF LEGAL AND SOCIAL ISSUES SURROUNDING GENETIC ENGINEERING
Legal issues constitute an important sociopolitical dimension that influences the adoption of genetic-engineering technology and its impacts on farmers and communities. The legal issues are complex, and a complete treatment of them is beyond the expertise of any of the authors of this report. We briefly touch here on the issues of seed saving, gene flow, and organic standards.
Seed Biotechnology
Courts in the United States and Canada have consistently upheld the rights of companies that sell patented seeds and genes through technology-use agreements to prohibit seed-saving practices that involve seed sold through those contracts (Kershen, 2004; Anonymous, 2008). Although that property right has been established, some continue to express concern about the ethical issues surrounding the patenting of life forms and over the effects of technology-use agreements on seed-saving practices. Research on whether those concerns are warranted and what the impacts are on farm sustainability are needed. Concerns are also being raised about the lack of farmer involvement in GE-trait development for traits that could address production problems identified by farmers and over the implications of current patenting procedures on power relationships between biotechnology firms and farmers (Phillipson, 2001). However, the social and economic effects of the exercise of such property rights, especially actual or potential litigation on both adopters and nonadopters of GE crops, have not been thoroughly investigated by social scientists. The lack of academic analyses of those issues may be due in part to the fact that companies, in any sector, that use the courts to enforce their property rights view legal actions and any out-of-court settlements as proprietary information. One interesting response by those who are concerned about the possible effects of the private control of genetic resources has been the open-source breeding movement.5
Gene Flow
A second set of legal issues related to genetic-engineering technology has to do with gene flow, particularly from fields of GE crops to those managed by people not using GE crops (for more on the potential for gene flow between GE and non-GE crops and on the challenges of coexistence of GE and non-GE crops, see Chapter 2). As in cases that involve restrictions on farmers against seed saving, these issues can be viewed as property-rights issues. Does gene flow impinge on the rights of producers and consumers who wish to grow and eat foods that do not include GE material (Conner, 2003)? That is of particular concern to some farmers who wish to produce organic or non-GE crops. Even though organic certification by the U.S. government is determined by the process used to grow the crop, some farmers are concerned that their products may not be accepted by markets in other countries or by food distributors and consumers who establish their own standards, irrespective of process.
Several lawsuits have been filed by farmers against agricultural-biotechnology companies in part because of damage alleged to have occurred as a result of drift of genetic material to the fields of farmers who do not wish to grow crops with GE traits (Kershen, 2004). Consumer groups have also brought legal action against the federal government for approving the commercialization of GE crops that have the potential to cross with non-GE crops in the same vicinity. As was discussed in previous chapters, GE alfalfa was pulled from the market after a U.S. federal judge sided with arguments brought forward by numerous plaintiffs and found that USDA should have prepared an environmental impact statement before it deregulated the crop, which facilitates commercialization (Geertson Farms v. Johanns, 2009). In another case filed by the Center for Food Safety and other plaintiffs, a federal judge decided in September 2009 that similar steps should have been taken before GE sugar beet was commercialized (Pollack, 2009).
Issues raised by the possibility of gene flow are not only legal in nature. As noted in Chapters 2 and 3, the adventitious presence of GE material in non-GE crops raises complex environmental and economic challenges. Similarly, social problems could arise as a consequence of gene flow, particularly if GE and non-GE producers of the same commodity live in the same community. Gene-flow disputes could move beyond the merely legal and affect the overall functioning of communities where such disputes exist. This might include conflicts between farmers as well as stress related to the economic and social costs associated with lawsuits and the potential threat of lawsuits. Studies of the social effects of such disputes are needed to gauge the full impact on community well-being. The ability of GE production and non-GE production to coexist in society may depend on the health of communities. Proposals for establishing
“landscape clubs” (Furtan et al., 2007) and voluntary “GMO-free zones”6 (Jank et al., 2006) clearly depend on the existence of high levels of community cooperation, which could be undermined by disputes related to gene flow.
Organic Laws and Resistance to Genetic Engineering
One of the intriguing public debates that has emerged around genetic engineering in agriculture has been that regarding whether GE crops should be allowable in legal standards for organic agriculture. As discussed in Chapter 1, many organic growers have vehemently resisted the notion that GE crops should be allowable in organic agricultural production systems. However, scientific arguments can be made for the use of genetic-engineering technology for making organic agricultural production more sustainable. Ronald and Adamchak (2008) note that what is or is not an appropriate use of genetic-engineering technology for “organic” producers is problematic given that genetic-engineering techniques can be used to transfer genes within plant species as easily as between them. Genetic-engineering techniques also include the use of marker-assisted breeding wherein the genetic “fingerprint” of plants can be used to aid conventional plant breeding. These authors also note the potential for genetic-engineering technology to develop new varieties of crops that could be grown under conditions that reduce some of the adverse environmental impacts of growing food and that contribute to local food production. The rationale parallels the arguments used in discussing the potential of genetic-engineering technology for improving the productive capability of orphan crops in developing countries (Naylor et al., 2004).
The ideological divisions between those who favor and those who oppose the use of GE plants in organic production systems are complex, and in many cases concerns about safety and naturalness are connected to and mask socioeconomic concerns. An example of the complexity was the successful vote in Mendocino County, California, in 2004 to ban the local use of GE organisms in agriculture. The legal focus of the vote was on GE organisms, but it was clear, because of how genetic-engineering technology was linked to issues related to corporate versus local control of agriculture, that the technology was viewed by many of those supporting the measure as a social problem (Walsh-Dilley, 2009). Similarly,
some genetic-engineering proponents argue for including GE products in organic standards and labels at the same time that they argue against the labeling of foods with GE content because they consider GE and non-GE foods to be substantially equivalent products (Klintman, 2002). That position can be understood, in part, as a desire to obtain the economic benefit of some labels while avoiding the cost of being associated with other labels. Those examples underscore the important socioeconomic and sociopolitical dimensions in public debates about genetic-engineering technology. To reconcile those debates over the potential use of genetic engineering in sustainable and developing-country agriculture, it may be wise to heed the suggestion of Ronald and Adamchak (2008) and use various social, environmental, and economic criteria in making decisions on when to use and not to use genetic-engineering technology in agriculture.
CONCLUSIONS
Social dynamics and networks between farmers and within local communities play a substantial role in the decisions that farmers make with respect to the use of GE crops and likely are impacted by the use of and conflicts over those crops. Research on the adoption of other agricultural technologies has demonstrated substantial social impacts on a farm level and a community level. Those impacts include but are not limited to: decreases to and change of composition in the agricultural labor force; better on-farm working conditions; changes in farm and agricultural-industry structure; increases in capital requirements for farmers; and a decline in the socioeconomic viability of some rural communities. Comparable research on the effects of GE crops is lacking, and although it is reasonable to hypothesize that the social impacts of the spread of GE crops have been low due to the assumed scale neutrality of this technology, it is equally reasonable to assume that the social impacts have been numerous and profound. Those questions cannot be answered without short- and long-term empirical research on the social processes surrounding, and the social impacts associated with, the adoption of genetic-engineering technologies at the farm level. Such research must take into account the various contextual factors that are influencing social changes on U.S. farms and rural communities.
Research has demonstrated that farmers’ interest in genetic-engineering technology and patterns of adoption are influenced by farmers’ social networks and by farmers’ associations, private firms, and public actors, including universities. Research also has identified the continuing consolidation of the seed industry and its integration with the chemical industry. The market power of firms that supply seed has not adversely
affected farmers’ economic welfare so far, but research is needed on how market structure may affect ongoing access to non-GE or single-trait seeds and future seed prices. Furthermore, there has been comparatively little research on how changes in farmer social networks and seed-industry concentration might be affecting farmers’ planting decisions and options, overall yield benefits, crop genetic diversity, and economic returns.
A final set of social issues has to do with complex legal issues, including the adoption of and the use of genetic-engineering technology. U.S. and Canadian courts have upheld the legal rights of seed companies to prohibit seed-saving practices through the use of contracts. The issue of gene flow is complicated. One important question being raised is whether adventitious presence of genetic material from GE crops into non-GE crops impinges on the rights of producers, including organic producers, who do not wish to use specific GE traits. The legal debates may mask deeper social and ideological divisions over the use of GE plants and how to define and implement sustainable agricultural practices.
REFERENCES
Anonymous. 2001. Group spells out concerns over genetically modified wheat. CBC-News.ca, August 1. Health section. Available online at http://www.cbc.ca/health/story/2001/07/31/gm_wheat010731.html. Accessed April 1, 2009.
Anonymous. 2008. CAFC again agrees you can’t save seed; judge blocks sale of Roche Epo. Patent litigation. Biotechnology Law Report 27(3):221–222.
Barham, B.L., J.D. Foltz, D. Jackson-Smith, and S. Moon. 2004. The dynamics of agricultural biotechnology adoption: Lessons from series rBST use in Wisconsin, 1994-2001. American Journal of Agricultural Economics 86(1):61–72.
Bauman, D.E. 1992. Bovine somatotropin: Review of an emerging animal technology. Journal of Dairy Science 75(12):3432–3451.
Beck, R.L., and H. Gong. 1994. Effect of socioeconomic factors on bovine somatotropin adoption choices. Journal of Dairy Science 77(1):333–337.
Berardi, G.M. 1981. Socio-economic consequences of agricultural mechanization in the United States: Needed redirections for mechanization research. Rural Sociology 46(3):483–504.
Boehlje, M. 1999. Structural changes in the agricultural industries: How do we measure, analyze and understand them? American Journal of Agricultural Economics 81(5):1028–1041.
Bradford, K., J. Alston, and N. Kalaitzandonakes. 2006. Regulation of biotechnology for specialty crops. In Regulating agricultural biotechnology: Economics and policy. eds. R.E. Just, J.M. Alston, and D. Zilberman, pp. 683–697. New York: Springer.
Brennan, M.F., C.E. Pray, and A. Courtmanche. 1999. Impact of industry concentration on innovation in the U.S. plant biotech industry. Paper presented at the Transitions in agbiotech: Economics of strategy and policy NE-165 conference (Washington, DC, June 24–25, 1999).
Buttel, F.H., O.F. Larson, and G.W. Gillespie Jr. 1990. The sociology of agriculture. New York: Greenwood Press.
Caswell, M.F., K.O. Fuglie, and C.A. Klotz. 1994. Agricultural biotechnology: An economic perspective. Agricultural Economic Report No. 687. U.S. Department of Agriculture–Economic Research Service. Washington, DC.
Chen, L., and F.H. Buttel. 2000. Dynamics of GMO adoption among Wisconsin farmers. Madison, WI: Program on Agricultural Technology Studies, University of Wisconsin-Madison and University of Wisconsin-Cooperative Extension.
Cochrane, W.W. 1993. The development of American agriculture: A historical analysis. 2nd ed. Minneapolis: University of Minnesota Press.
Conner, D.S. 2003. Pesticides and genetic drift: Alternative property rights scenarios. Choices: The magazine of food, farm, and resource issues 1st Quarter: 5–7. Available online at http://www.choicesmagazine.org/2003-1/2003-1-02.pdf. Accessed March 31, 2010.
de Gorter, H., and D. Zilberman. 1990. On the political economy of public good inputs in agriculture. American Journal of Agricultural Economics 72(1):131–137.
Delmer, D.P. 2005. Agriculture in the developing world: Connecting innovations in plant research to downstream applications. Proceedings of the National Academy of Sciences of the United States of America 102(44):15739–15746.
DuPuis, E.M., and C. Geisler. 1988. Biotechnology and the small farm. BioScience 38(6):406– 411.
Fernandez-Cornejo, J. 2004. The seed industry in U.S. agriculture: An exploration of data and information on crop seed markets, regulation, industry structure, and research and development. Agriculture Information Bulletin No. 786. U.S. Department of Agriculture– Economic Research Service. Washington, DC. Available online at http://www.ers.usda.gov/publications/aib786/aib786.pdf. Accessed May 26, 2009.
Fernandez-Cornejo, J., and D. Schimmelpfennig. 2004. Have seed industry changes affected research effort? Amber Waves 2(1):14–19.
Fernandez-Cornejo, J., and R.E. Just. 2007. Researchability of modern agricultural input markets and growing concentration. American Journal of Agricultural Economics 89(5):1269–1275.
Fernandez-Cornejo, J., S. Daberkow, and W.D. McBride. 2001. Decomposing the size effect on the adoption of innovations: Agrobiotechnology and precision farming. Paper presented at the American Agricultural Economic Association Annual Meeting (Chicago, IL, August 5–8, 2001). Available online at http://ageconsearc.umn.edu/bitstream/20527/1/sp01fe02.pdf. Accessed February 23, 2009.
Fuglie, K., N. Ballenger, K. Day, C. Klotz, M. Ollinger, J. Reilly, U. Vasavada, and J. Yee. 1996. Agricultural research and development: Public and private investments under alternative markets and institutions. Agricultural Economics Report No. 735. May. U.S. Department of Agriculture–Economic Research Service. Washington. DC. Available online at http://www.ers.usda.gov/publications/aer735/AER735fm.PDF. Accessed May 31, 2009.
Fulton, M., and K. Giannakas. 2001. Agricultural biotechnology and industry structure. AgBioForum 4(2):137–151.
Furtan, W.H., A. Güzel, and A.S. Weseen. 2007. Landscape clubs: Co-existence of genetically modified and organic crops. Canadian Journal of Agricultural Economics 55(2):185–195.
Geertson Farms Inc., et al. v. Mike Johanns, et al., and Monsanto Company: Memorandum and order Re: Permanent injunction. 2009. U.S. District Court for the Northern District of California. C 06-01075 CRB, Case#: 3:06-cv-01075-CRB. Decided May 3, 2007. Available online at http://www.aphis.usda.gov/brs/pdf/Alfalfa_Ruling_20070503.pdf. Accessed December 16, 2009.
Goldsmith, P.D. 2001. Innovation, supply chain control, and the welfare of farmers: The economics of genetically modified seeds. American Behavioral Scientist (8):1302–1326.
Graff, G.D., and D. Zilberman. 2007. The political economy of intellectual property: Reexamining European policy on plant biotechnology. In Agricultural biotechnology and intellectual property: Seeds of change. ed. J.P. Kesan, pp. 244–267. Cambridge, MA: CABI Publishing.
Graff, G.D., S.E. Cullen, K.J. Bradford, D. Zilberman, and A.B. Bennett. 2003. The public-private structure of intellectual property ownership in agricultural biotechnology. Nature Biotechnology 21(9):989–995.
Graham, J., and A. Martin. 2004. Biotech wheat pits farmer vs. farmer. Chicago Tribune, January 28. p. C-7, News section.
Guehlstorf, N. 2008. Understanding the scope of farmer perceptions of risk: Considering farmer opinions on the use of genetically modified (GM) crops as a stakeholder voice in policy. Journal of Agricultural and Environmental Ethics 21(6):541–558.
Hayenga, M.L. 1998. Structural change in the biotech seed and chemical industrial complex. AgBioForum 1(2):43–55.
Hill, T. 2009. Personal communication to the Committee on the Impact of Biotechnology on Farm-Level Economics and Sustainability. February 26. Washington, DC.
Jank, B., J. Rath, and H. Gaugitsch. 2006. Co-existence of agricultural production systems. Trends in Biotechnology 24(5):198–200.
Jussaume, R.A., Jr., K. Kondoh, and M. Ostrom. 2004. An investigation into the potential introduction of Roundup-Ready wheat. Paper presented at the annual meetings of the Rural Sociological Society (Sacramento, CA, August 15–18, 2004).
Just, D.R., S.A. Wolf, S. Wu, and D. Zilberman. 2002. Consumption of economic information in agriculture. American Journal of Agricultural Economics 84(1):39–52.
Kaup, B.Z. 2008. The reflexive producer: The influence of farmer knowledge upon the use of Bt corn. Rural Sociology 73(1):62–81.
Kershen, D.L. 2004. Legal liability issues in agricultural biotechnology. Crop Science 44(2):456–463.
Klintman, M. 2002. Arguments surrounding organic and genetically modified food labelling: A few comparisons. Journal of Environmental Policy & Planning 4(3):247–259.
Kloppenburg, J.A. 1984. The social impacts of biogentetic technology in agriculture: Past and future. In The social consequences and challenges of new agricultural technologies. eds. G.M. Berardi and C.C. Geisler, pp. 291–321. Boulder, CO: Westview Press.
Leonard, C. 2006. Monsanto, Pioneer fight for seed market. The Washington Post, December 8. Technology section. Available online at http://www.washingtonpost.com/wp-dyn/content/article/2006/12/08/AR2006120800030.html. Accessed November 3, 2009.
Lerner, J., and J. Tirole. 2005. The economics of technology sharing: Open source and beyond. The Journal of Economic Perspectives 19(2):99–120.
Lesser, W. 1998. Intellectual property rights and concentration in agricultural biotechnology. AgBioForum 1(2):56–61.
Marion, B.W., and R.L. Wills. 1990. A prospective assessment of the impacts of bovine somatotropin: A case study of Wisconsin. American Journal of Agricultural Economics 72(2):326–336.
Monsanto. 2010. Academic research agreements. Available online at: http://www.monsanto.com/monsanto_today/for_the_record/academic/research/agreements.asp. Accessed March 22, 2010.
Naylor, R.L., W.P. Falcon, R.M. Goodman, M.M. Jahn, T. Sengooba, H. Tefera, and R.J. Nelson. 2004. Biotechnology in the developing world: A case for increased investments in orphan crops. Food Policy 29(1):15–44.
Nytes, A.J., D.K. Combs, G.E. Shook, R.D. Shaver, and R.M. Cleale. 1990. Response to recombinant bovine somatotropin in dairy cows with different genetic merit for milk production. Journal of Dairy Science 73(3):784–791.
Phillipson, M. 2001. Agricultural law: Containing the GM revolution. Biotechnology and Development Monitor (48):2–5.
Pimentel, D., M.S. Hunter, J.A. Lagro, R.A. Efroymson, J.C. Landers, F.T. Mervis, C.A. McCarthy, and A.E. Boyd. 1989. Benefits and risks of genetic engineering in agriculture. BioScience 39(9):606–614.
PIPRA (Public Intellectual Property Resource for Agriculture). 2006. Providing IP support for research consortia: PIPRA forges a novel approach for Pierces’ Disease research. PIPRA. University of California, Davis, Fall (Issue 6):3.
Pollack, A. 2004. Monsanto shelves plan for modified wheat. The New York Times, May 11. p. 1, C section.
———. 2009. Judge rejects approval of biotech sugar beets. The New York Times, September 23. p. 3, B section.
Quaintance, H.W. 1984. The influence of farm machinery on production and labor. In The social consequences and challenges of new agricultural technologies. eds. G.M. Berardi and C.C. Geisler, pp. 237–248. Boulder, CO: Westview Press.
Rogers, E.M. 2003. Diffusion of innovations. New York: Free Press.
Ronald, P.C., and R.W. Adamchak. 2008. Tomorrow’s table: Organic farming, genetics and the future of food. New York: Oxford University Press.
Ruttan, V.W. 1982. Agricultural research policy. Minneapolis: University of Minnesota Press.
Sexton, R.J. 1986. Cooperatives and the forces shaping agricultural marketing. American Journal of Agricultural Economics 68(5):1167–1172.
Shi, G., and J.-P. Chavas. 2009. On pricing and vertical organization of differentiated products. Staff Paper No. 535. University of Wisconsin-Madison. Madison, WI. Available online at http://www.aae.wisc.edu/fsrg/. Accessed October 22, 2009.
Shi, G., J.-P. Chavas, and K. Stiegert. 2008. An analysis of bundle pricing: The case of the corn seed market. FSWP2008-01. University of Wisconsin-Madison. Madison, WI. Available online at http://www.aae.wisc.edu/fsrg/. Accessed October 8, 2009.
Squires, G. 2004. Wheat watch. Wheat Life. Washington Association of Wheat Growers. Available online at http://www.wawg.org/index.cfm?show=10&mid=59. Accessed August 9, 2009.
Stiegert, K.W., J.P. Chavas, and G. Shi. 2009. Analysis of strategic pricing in the U.S. transgenic cornseed market. Working paper. University of Wisconsin-Madison. Madison, WI.
Thirtle, C., L. Beyers, Y. Ismael, and J. Piesse. 2003. Can GM-technologies help the poor? The impact of Bt cotton in Makhathini Flats, KwaZulu-Natal. World Development 31(4):717–732.
Toyama, M., J.W. Heffernan, V.N. Hillers, R.A. Jussaume, Jr., and J.A. Schultz. 2001. Consumers’ concerns and behaviors related to biotechnology: Comparison between American and Japanese consumers. Presentation at the IFT Annual Meeting (New Orleans, LA, June 23–27, 2001).
US-EPA (U.S. Environmental Protection Agency). 2008. FIFRA scientific advisory panel: Notice of public meeting. Federal Register 73(238):75099–75101.
USDA (U.S. Department of Agriculture). 2010. USDA and DOJ hold first-ever workshop on competition issues in agriculture. Press release No. 0126.10. March 12, 2010. Available online at: http://www.usda.gov/wps/portal/!ut/p/_s.7_0_A/7_0_1OB/.cmd/ad/.ar/sa.retrievecontent/.c/6_2_1UH/.ce/7_2_5JM/.p/5_2_4TQ/.d/2/_th/J_2_9D/_s.7_0_A/7_0_1OB?PC_7_2_5JM_contentid=2010%2F03%2F0126.xml&PC_7_2_5JM_parentnav=LATEST_RELEASES&PC_7_2_5JM_navid=NEWS_RELEASE#7_2_5JM. Accessed March 22, 2010.
Van Es, J.C., D.L. Chicoine, and M.A. Flotow. 1988. Agricultural technologies, farm structure and rural communities in the Corn Belt: Policies and implications for 2000. In Agriculture and community change in the U.S.: The congressional research reports. ed. L.E. Swanson, p. 355. Boulder, CO: Westview Press.
Vandenberg, J.M., J.R. Fulton, F.J. Dooley, and P.V. Preckel. 2000. Impact of identity preservation of non-GMO crops on the grain market system. CAFRI: Current Agriculture, Food and Resource Issues (01):29–36.
Walsh-Dilley, M. 2009. Localizing control: Mendocino county and the ban on GMOs. Agriculture and Human Values 26(1):95–105.
Wilson, W.W. 2009. Personal communication to the Committee on the Impact of Biotechnology on Farm-Level Economics and Sustainability. February 26. Washington, DC.
Wilson, W.W., E.L. Janzen, and B.L. Dahl. 2003. Issues in development and adoption of genetically modified (GM) wheats. AgBioForum 6(3):101–112.
Wolf, S.A., D.R. Just, and D. Zilberman. 2001. Between data and decisions: The organization of agricultural economic information systems. Research Policy 30(1):121–141.