5
Key Findings, Remaining Challenges, and Future Opportunities
The first generation of genetically engineered (GE) crops has mostly delivered effective pest control for a few major crops because farmers producing these crops spend a lot of time and money on the task, because the firms developing the new seed technologies saw considerable profit potential in doing so, and because adding the traits was relatively straightforward, requiring transformation of a genome at only a single location. The first generation of GE crops continues a reliance on pesticide technology—in-plant toxins or resistance to herbicides—to mitigate pest problems primarily in corn, cotton, and soybean. Thus, the application of genetic-engineering technology to crops has not developed novel means of pest control, such as developing plant mechanisms to resist pest damage, nor has it reached most minor crops.
The next set of challenges for the application of GE-crop technology is to expand to additional crops and to address additional desirable traits, such as drought tolerance, enhanced fertilizer utilization to reduce nutrient runoff, nutritional benefits, renewable energy production, and carbon sequestration. A number of those applications are under development by the private sector, some by the public sector. Clearly, the future agenda for genetic-engineering technology is extensive and of great importance for improvements in agricultural productivity and sustainability in a rapidly changing world.
This chapter opens by summarizing the major findings of our assessment of the farm-level environmental, economic, and social impacts of GE crops. We then identify key remaining challenges that will frame future
development and commercialization of genetic-engineering technology in crops. The discussion turns next to the future agenda of GE-crop applications, including general patterns of crop-trait development, implications for future weed-resistance management, and the potential role of GE crops for biofuels. The penultimate section highlights two subjects of research that the committee believes deserve more resources and effort: water quality and social impacts of GE crops. In closing, we discuss options for strengthening public and private research and development to exploit the potential of genetic-engineering technology to contribute more fully to environmental, economic, and social objectives.
KEY FINDINGS
The evidence shows that the planting of GE crops has largely resulted in less adverse or equivalent effects on the farm environment compared with the conventional non-GE systems that GE crops replaced. A key improvement has been the change to pesticide regimens that apply less pesticide or that use pesticides with lower toxicity to the environment but that have more consistent efficacy than conventional pesticide regimens used on non-GE versions of the crops. In the first phase of use, herbicide-resistant (HR) crops have been associated with an increased use of conservation tillage, in particular no-till methods, that can improve water quality and enhance some soil-quality characteristics. That farmers who practice conservation tillage are more likely to adopt GE crops suggests the two technologies are complementary.
At least one potential environmental risk associated with the first phase of GE crops has surfaced: Some adopters of GE crops rely heavily on a single pesticide to control targeted pests, and this leads to a buildup of pest resistance regardless of whether GE crops or non-GE crops are involved. The governmental regulation of GE Bt crops through refuge requirements seems to have proved effective in delaying buildup of insect resistance with two reported exceptions, which have not had major consequences in the United States. Grower decisions to use repeated applications of particular herbicides to some HR crops have led, in some documented cases, to evolved herbicide-resistance problems and shifts in the weed community. In contrast with Bt-crop refuge requirements, no public or private mechanisms for delaying weed resistance have been extensively implemented. If the herbicide-resistance problem is not addressed soon, farmers may increasingly return to herbicides that were used before the adoption of HR crops. Tillage could increase as a pest-management tactic as well. Such actions could limit some of the environmental and personal safety gains associated with the use of HR crops. The newest HR varieties likely will have tolerance to more than one herbicide, and
this would allow easier herbicide rotation or mixing, and, in theory, help to improve the durability of herbicide effectiveness. These new stacked varieties will be one more tool to help manage the evolution of weed as well as insect resistance.
The potential for gene flow via cross-pollination between current major GE crops and wild or weedy relatives is limited to cotton in small spatial scales in the United States because the other major GE crops have no native relatives. How this changes in the future will depend on what GE crops are commercialized, whether related species with which they are capable of interbreeding are present, and the consequences of such interbreeding for weed management. Gene flow (i.e., the adventitious presence) of legal GE traits in non-GE crops and derived products remains a serious concern for farmers whose market access depends on adhering to strict non-GE standards. It would appear that the resolution of the issue may require the establishment of enforceable thresholds for the presence of GE material in non-GE crops that do not impose excessive costs on growers and the marketing system.
The literature reviewed in this report indicates that a majority of U.S. farmers who grow soybean, corn, or cotton have generally found GE varieties with herbicide-resistance and insect-resistance traits advantageous because of their superior efficacy in pest control; their concomitant economic, environmental, and presumed personal health advantages; or their convenience. The extent of the benefits varies among locations, crops, and specific genetic-engineering technologies.
After some early evidence of yield disadvantages for some GE varieties in the United States, studies have now shown either a moderate boost in yields of some crops or a neutral yield effect. Some emerging evidence suggests that the attractiveness of genetic-engineering technology for soybean, cotton, and corn has increased the global acreage planted to these crops over what would have been planted otherwise and thereby increased global commodity supplies (World Bank, 2007; Brookes and Barfoot, 2009). Consequently, the adoption of some of the GE crops around the world has put downward pressure on the prices received by U.S. farmers who are growing these crops, holding other factors constant. At the same time, livestock producers and consumers who purchase GE feed and food products may have benefited from the downward price pressure. However, the U.S. and world agricultural economies have been influenced by other factors that tend to increase commodity prices, making empirical verification of the effects difficult.
The economic effects of GE-crop adoption on nonadopters are mixed. To the extent that the use of genetic-engineering technology changes the types and amounts of inputs used, adopters of GE crops can influence the pesticide market. Changes in the price and availability of pesticides
affect nonadopters as well. Farmers of non-GE crops in the vicinity of GE-crop farms may experience landscape-level effects from reduced pressure from pests targeted by GE traits. Marketing of non-GE crops may also be affected by GE crops, favorably or unfavorably. For example, products derived from GE and non-GE crops can mix through gene flow or supply contamination. On the other hand, GE crops may create a market premium for non-GE products.
The historic social repercussions of introducing new technologies in agriculture, such as mechanization and the widespread planting of hybrid corn, have been studied extensively, and the results of the studies provide a basis for understanding the general effects of introducing GE varieties of crops. Despite the salience of those effects, however, there has been little investigation of farm-level and community-level social impacts of GE crops. The new seed technologies raise important potential social issues about farm structure, the input and seed choices available to farmers, and the genetic diversity of seeds. Among the known social facts associated with the dissemination of GE crops are the continued consolidation of the seed industry and its integration with the chemical industry. Another is the change in relationships between farmers and their seed suppliers. Testimony to the committee suggested that farmers of major crops have fewer opportunities to purchase non-GE seed of the best-yielding cultivars even when a GE trait is not perceived to be required in a particular cropping situation. As genetic-engineering technology matures and moves into its next phase, it is imperative that the full array of social issues involved be identified and investigated in depth.
REMAINING CHALLENGES FACING GENETICALLY ENGINEERED CROPS
Potential crop-biotechnology developments stir discussion around five issues. The treatment and resolution of those issues hold implications for long-term sustainability for farmers, including both adopters and nonadopters of GE crops.
First, the success of genetic-engineering technology in the United States has altered the seed industry by spurring consolidation of firms and integration with the chemical industry. Those developments continue to alter seed and pest-control options in the market, expanding pest-control options for some farmers and possibly limiting them for others, including those who do not grow GE crops. The resulting concentrated and legally enforceable private control of plant genetic material contrasts sharply with public plant-breeding programs that traditionally have fostered public access to discoveries, especially if GE varieties are developed for crops from which farmers have traditionally saved seed for the next year’s crop
(such as wheat, barley, and oat). Although corporate consolidation may offer greater economies of scale, it also is accompanied by the possibility of less competition and higher seed expenses, which may lower farmer returns, reduce pest-control options, and limit the benefits of commercialization of genetic-engineering technology to a few widely grown crops. There is not yet clear evidence of a lack of competition among the large companies that produce GE-crop technologies, but these trends should be monitored, and their effects ameliorated to remedy social losses that result.
Second, how the intensive use of current and prospective GE organisms will directly affect the natural environment differently from other agricultural production systems is incompletely understood (Ervin et al., 2003). Relatively few studies have provided integrated assessments of the indirect effects of GE crops on pest damage to non-GE crops and on the full suite of ecosystem services on the landscape scale. For example, the concurrent effects on regional water quality of shifting tillage and pesticide regimens with the introduction of GE crops on regional water quality conditions remain poorly documented and understood. Knowledge of the spatial and temporal effects on ecological health—favorable or unfavorable—assumes greater importance as the evolution of herbicide resistance in weeds alters patterns of herbicide use to make up for the loss of glyphosate’s efficacy on some species, and as novel GE plants, such as those for energy and nonfood uses, approach commercialization. Evaluation and monitoring of the ecological health of soils, water quality and quantity, and air quality will provide the information needed for developing the most productive yet sustainable agricultural systems for the future (Ervin et al., 2003).
Third, progress in developing GE varieties for most “minor” crops (e.g., fruits and vegetables) and for other “public goods” purposes not served well by private markets has been slow. Minor crops play important roles in the agricultural sectors of many states. Some minor crops, such as sunflowers and grain sorghum, have been considered to be poor candidates for the HR traits because of the existence of near-relative weeds (grain sorghum) or native ancestral populations (sunflowers). However, that risk does not explain the relative dearth of research and development (R&D) on GE varieties of minor crops as a whole, especially fruits and vegetables. The high fixed investment, patent protection involving GE traits, and the regulatory expense of commercializing GE seeds have lessened the ability of small companies and public-sector crop-breeding programs to develop commercially viable GE varieties of most minor crops. It seems clear that more effort should be devoted to the enhancement of minor crops with the best available genetic-engineering techniques even though the commercial rewards per crop may be small. Private and public
R&D programs for GE crops have not yet led to the commercialization of many additional plant traits, such as improved tolerance to drought and increased fertilizer-use efficiency that decreases nutrient runoff, a contributor to nonpoint-source water pollution. This is not to suggest that GE seeds represent a “silver bullet” technology to solve all of these problems. Such GE trait developments may or may not turn out to be the most cost-effective approach to solving these issues, but exploration is necessary to evaluate their relative efficacy. Even though the basic technology is over 20 years old, agricultural biotechnology in some regards is still in its infancy. The rate of progress of genetic-engineering technology for some purposes suggests that more time and resources and new institutional relationships, such as public–private R&D collaborations, are desirable for the technology to reach its potential. Traits that have not yet received much attention, such as improved nutrient absorption and enhanced food value of crops, should be emphasized in the future so that we can gain the knowledge needed for weighing how the advances of genetic-engineering technology could present options for addressing all aspects of food supply, energy security, and environmental challenges.
Fourth, the presence of transgenic material in non-GE products should be addressed. The current definition of organic food in the United States excludes the use of GE materials in production and handling processes, so organic farmers must take steps to ensure that their production methods do not expose their crops to GE traits. To avail themselves of market premiums for certified organic crops, they must incur costs for keeping their products separate from GE crops. Food producers who market products as non-GE face similar challenges to prevent co-mingling of GE and non-GE crops during storage and distribution. In those ways, the introduction of GE crops influences the decisions and operations of farmers and food producers who do not use the technology.
Fifth, U.S. farmers who grow GE crops may face market restrictions from some countries or retail firms on the importation or sale of the crops or products made from the crops. Under some international agreements, some countries impose these restrictions because of perceived food safety or environmental risks or for other reasons. Assuming that those actions satisfy international treaty rules, such market-access restrictions to some extent slow the development of a global market for GE crops. One effect of the trade restrictions has been to limit the market demand for GE crops.
The potential of GE-crop varieties to address the world’s emerging food-supply, energy, and environmental problems hinges on how those challenges are resolved. Success in resolving them may allow genetic-engineering technology to become even more transformational in fostering sustainable agricultural systems for farmers. This important agenda frames the discussion of future GE-crop applications.
FUTURE APPLICATIONS OF GENETICALLY ENGINEERED CROPS
In addition to expanding existing GE traits into other crops, the reach of genetic-engineering technology could be extended through the development and commercialization of new traits. Traits beyond those designed to control pests could have substantial benefits in fields other than agriculture, such as food and energy security. This section summarizes the present pattern of R&D of novel GE traits in the private and public sectors and highlights areas in which new traits could be especially useful to improving agricultural sustainability.
Patterns of Genetically Engineered Products in Development
The GE crops now being planted by U.S. farmers were developed over long gestation periods. Given that fact, the current portfolio of GE crops does not wholly capture the range of new crops being readied for commercialization that could cover the U.S. farm and global landscape in the future. For instance, companies are developing crops with more intricate pest-control mechanisms (see Box 5-1), but they are also engineering
BOX 5-1 New Traits Reduce Refuge Requirement and Introduce Second Mode of Herbicide Resistance On July 20, 2009, the Environmental Protection Agency (EPA) announced final approval for commercialization of SmartStax™ corn hybrids. These hybrids produce multiple proteins for the control of lepidopteran pests and for the control of corn rootworm (two of these proteins must be used together to induce significant rootworm mortality). The hybrids also have transgenes conferring resistance to glyphosate and glufosinate. Because of the multiple toxins affecting single pests, this group of hybrids was approved by EPA with a refuge requirement of 5 percent in the Corn Belt and 20 percent in the Cotton Belt (US-EPA, 2009). Those refuge requirements are reduced from the original requirements of 20 percent in the Corn Belt and 50 percent in the Cotton Belt for previous transgenic Bt corn hybrids. Resistance to the different broad-spectrum herbicides may help in delaying the evolution of resistance in weeds in areas where glyphosate-resistant populations have not been identified. However, growers must assume appropriate stewardship for these herbicides in order to manage the future evolution of herbicide-resistant weed populations. |
traits that improve crop tolerance to stress or that provide benefits directly to consumers. Recent research also indicates that novel forms of pest control may be part of the next generation of GE crops. As an example, Baum et al. (2007) report positive laboratory findings that ribonucleic-acid (RNA) interference technology, a plant-based method for pest management, results in larval stunting and mortality in several coleopteran species controlled by Bt crops. Predicting future crop biotechnologies is somewhat difficult because companies, for reasons of competitiveness and patent protection, may not fully share the specifics of planned releases. However, three recent global surveys of product-quality innovations in the development phase of genetic-engineering technology help in discerning general trends in GE-crop development and, in particular, why quality-improving innovations from genetic-engineering technology have not yet been more numerous (Graff et al., 2009).
The combined findings of the surveys show that less than 5 percent of the innovations reach the regulatory and commercialization phases. As might be expected, R&D activity has been uneven among different trait categories because of variation in the difficulty of achieving selected transformations and in the economic value of the traits. For example, traits governing content and composition of macronutrients—proteins, oils, and carbohydrates—and traits that control fruit ripening have more readily reached later stages of R&D, and few products with enhanced micronutrients, functional food components, or novel esthetics have approached commercialization (Graff et al., 2009). The analysts concluded that product-quality innovation appears more responsive to demand in intermediate markets for processing and feed attributes than to demand in retail markets for improved or novel products. They also noted that many of the observed traits offer potential efficiency gains in agriculture and improvements in natural-resource systems and a potential to reduce environmental impacts both because they will decrease input requirements and because they will reduce adverse externalities of crop production, processing, or consumption. For example, new traits may increase the efficiency of livestock-feed digestibility and by so doing increase the value of the feed to farmers. Traits that improve nitrogen-use efficiency that are on the horizon will bring value to farmers and could contribute to reducing agriculture’s effects on water quality. The potential for environmental improvement from such traits depends on the degree to which they improve input-use efficiency and the extent to which farmers expand production because of lower unit costs.
The rate of product-quality innovations in genetic-engineering technology identified in the surveys decreased considerably after 1998. The authors noted that the cause of the decline remains conjectural. It coincided with the decrease in the number of transgenic field trials conducted
in the United States and Europe, with the exit from the market of a number of small biotechnology companies that were not deeply involved in the first generation of GE pest-control crops, and with changes in the regulatory environment in Europe that restricted the introduction of GE varieties there.
Data on field tests of genetic-engineering innovations corroborate the slowdown. A critical part of new variety development is field testing to ensure that the desired traits will perform under commercial production conditions and that no important environmental risks are associated with release of a GE organism. The release of new GE varieties or organisms into the environment is regulated, in part, through field-release permits monitored by the U.S. Department of Agriculture (USDA) Animal and Plant Health Inspection Service (APHIS) (Fernandez-Cornejo and Caswell, 2006).1 The overall number of field releases of plant varieties for testing purposes is a useful indicator of R&D efforts in GE crops. By the end of 2008, about 15,000 applications had been received by APHIS since 1987, and nearly 14,000 (93 percent) had been approved (ISB and USDA-APHIS, 2009). Annual applications peaked in 1998 with 1,206, and annual approvals peaked in 2002 with 1,141 (Figure 5-1). Most applications approved for field testing in 1987–2008 involved major crops, particularly corn with 6,648 applications approved, followed by soybean (1,554), cotton (912), potato (817), tomato (637), wheat (413), alfalfa (385), and tobacco (363). Applications approved during this time included GE varieties with herbicide resistance (25.1 percent), insect resistance (20.1 percent), improved product quality (flavor, appearance, or nutrition) (18.2 percent), and agronomic properties, such as drought resistance (13.4 percent) (Figure 5-2).
After sufficient field testing, an applicant may petition APHIS for a determination of “nonregulated” status to facilitate commercialization of a product. If, after extensive review, APHIS determines that unconfined release does not pose a substantial risk to agriculture or the environment, the organism is “deregulated” and can be moved and planted without APHIS authorization (Fernandez-Cornejo and Caswell, 2006). Petitions for deregulated varieties peaked in 1995–1997 with 14-15 petitions per year and have been below 10 petitions every year thereafter (ISB and USDA-APHIS, 2009). As of June 5, 2009, APHIS had received 119 petitions for deregulation and had approved 76. The deregulated varieties had HR traits (38 percent), IR traits (28 percent), product-quality traits (15 per-
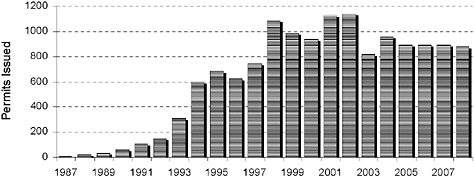
FIGURE 5-1 Number of permits for release of genetically engineered varieties approved by APHIS.
SOURCE: ISB and USDA-APHIS, 2009.
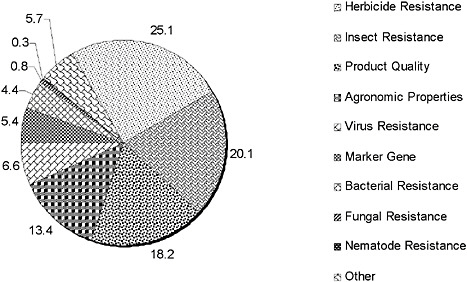
FIGURE 5-2 Approved field releases of plant varieties for testing purposes by trait (percent).
SOURCE: ISB and USDA-APHIS, 2009.
cent), virus-resistance traits (11 percent), and agronomic traits (6 percent) (ISB and USDA-APHIS, 2009).
Another study of the APHIS GE-crop release data investigated the private or commercial and public-goods aspects of traits being tested (Welsh and Glenna, 2006). The authors analyzed releases in 1993–2002 to answer two research questions:
-
“To what extent have universities mimicked the for-profit sector in agricultural biotechnology by focusing their transgenic research on a relatively few proven genotypes (traits)?”
-
“To what extent have universities mimicked the for-profit sector in agricultural biotechnology by focusing their transgenic research on the relatively few major (commercially dominant) agronomic crops?”
They categorized the crop releases into major traits—herbicide resistance, insect resistance, and product quality—and “other” GE traits. Product quality includes the alteration of a particular crop’s characteristics to make it more valuable to food, feed, or energy manufacturing firms, such as a higher lysine concentration in corn that would be useful to livestock producers. Other GE traits were nematode resistance, fungus resistance, bacterium resistance, virus resistance, and agronomic properties (such as yield). They also categorized the releases by whether they were major crops (soybean, corn, wheat, alfalfa, and cotton) or minor crops (such as other field crops, vegetables, and fruits planted on smaller acreages).
The results indicated that the research profiles of universities in 1993–2002 were less dominated by the major traits than were the profiles of for-profit firms. A similar pattern emerged for major crops: About 71 percent of notices filed with APHIS by for-profit firms entailed research on major crops compared with 32.6 percent filed by universities. Moreover, work on minor crops differed among universities depending on their region (for example, apples in the Northeast and citrus in the Southeast). The authors examined whether the relationships changed during three periods: 1993–1995, 1996–1998, and 1999–2002. The research profiles of the for-profit firms remained fairly uniform, but universities looked more like for-profit firms in the later periods. That trend was especially pronounced for major traits: Almost 73 percent of notices filed by universities entailed at least one major trait in 1999–2002 compared with around 35 percent in earlier periods. The proportion of research on major crops by universities also increased.
To probe those relationships in more depth, Welsh and Glenna constructed a commercial index for each release. The index was computed by assigning scores of 1 for research on a major crop and 1 for research on a major trait. Research on a minor crop was scored as –1 and research on a minor trait was scored as –1. Possible index values were therefore 2, 1, 0, –1, and –2. A higher index value indicated greater research emphasis on more commercially relevant crops and traits, and a lower value indicated more emphasis on crops and traits with smaller markets. Plotting the value of the index for universities and private-sector firms over time revealed that universities and private-sector firms showed increasingly similar research trajectories, which emphasized major crops and major
traits in genetic-engineering technology. The time-series relationship was also found to be statistically significant (Welsh and Glenna, 2006).
Results of those three studies of GE-trait developments indicated that some GE products in various phases of development serve purposes other than pest-control traits dominant today, but they have not been commercialized. The reasons for this vary with the crop and the trait, but include a small anticipated market, lack of access to technology, uncertainty about consumer acceptance, potential spillover to weedy relatives or gene transfer to non-GE cultivars, and high regulatory costs. During the process of innovation, commercialization, and adoption, all organizations weigh the costs and benefits of their decisions (Bradford et al., 2006). It is not surprising that the GE crops that were commercialized first provided substantial net benefits to the innovators, the seed companies, and adopting farmers.
Implications of Genetically Engineered Crops for Weed Management
An unmet challenge for GE crops documented in Chapter 2 revolves around sustaining the efficacy of particular herbicides. Maintaining their efficacy holds important implications for future farm economics and environmental sustainability. A key concept in understanding herbicide-resistance effects in weeds is the open, unregulated access of all farmers to the common pool of pest susceptibility. The presence of this condition leads to individual decisions that may impose user costs on the whole population of farmers, to suboptimal overall management, and to increasing total social costs (Hardin, 1968).
The data show that GE-crop cultivars resistant to glyphosate dominate in the corn, soybean, and cotton production regions across the United States. Results of a six-state survey to assess crop rotations by growers of GE crops showed that rotation of a GE crop with a non-GE crop was most commonly followed by rotation of a GE crop with a GE crop (Shaw et al., 2009). Rotation of a GE with a non-GE crop was more common in the Midwest than in the South. With the high acreage of glyphosate-resistant crops being planted, substantial changes in herbicide use occurred; notably, fewer herbicides were used (Young, 2006); the most common current herbicide tactic reported is one to three applications of glyphosate (Givens et al., 2009). A related change in production practice attributable to the adoption of HR crops that has implications for weed management was the increased adoption of conservation tillage (Givens et al., 2009). The overall grower assessment of the effect of HR crops on weed population densities was that there were fewer weeds because of the use of glyphosate on HR crops (Kruger et al., 2009). Few growers felt that weed populations would shift to species that had evolved resis-
tance to glyphosate, understood the importance of alternative tactics to control weeds, or perceived the role of selection pressure caused by the use of glyphosate on HR crops (Johnson et al., 2009). Given that changes in weed communities in response to production practices have been a consistent problem in agriculture, future weed challenges are likely to increase quickly (Baker, 1991; Owen, 2001; Heard et al., 2003a, 2003b). To summarize, growers valued the immediate benefits of weed control without appreciating the long-term risks attributable to the tactics they used. That behavioral response might be expected given many farmers’ desire to meet short-run financial needs and the fact that other growers may not take similar control actions.
Recent survey data show that growers emphasize the convenience and simplicity of the glyphosate-based cropping systems while discounting the importance of diversification of weed-management practices (Owen, 2008b). That prevailing attitude has several likely results: Given growers’ apparent unfamiliarity with or lack of concern about the implications of selection pressure for weed communities, weed shifts are inevitable; and growers’ short-term interest in killing weeds rather than managing them will result in the loss of crop yield and farm profits in the long run unless innovation in weed-control technology occurs. The efficacy of glyphosate on a broad spectrum of weeds, its ability to kill larger weeds, and the fact that glyphosate can be applied to HR crops almost regardless of crop stage of growth all reinforce growers’ perception of the simplicity and convenience of glyphosate-based programs.
Weed management implies that there is a critical period of weed control (CPWC) when weed interference must be eliminated to protect crop-yield potential (Nieto et al., 1968; Kasasian and Seeyave, 1969; Swanton and Weise, 1991; Kneževič et al., 2002, 2003). The CPWC is the same whether the crop is GE or non-GE. There is considerable information about the proper timing of glyphosate applications that will provide protection of yield potential and the related economic loss of crop yield in response to untimely glyphosate application (Gower et al., 2003; Dalley et al., 2004; Cox et al., 2005, 2006; Stahl, 2007). Many growers may recognize the relationship between early weed interference with HR crops and the resulting economic loss to profitability or may face high individual cost or risk in changing their behavior. The perception of success with glyphosate results in the repeated use of this herbicide without consideration of alternative strategies. Recurrent application of any herbicide will cause shifts in the weed community that support the evolving dominance of weeds that are not susceptible. Growers must use diversified weed-management practices, recognize the importance of understanding the biology of the cropping system, and give appropriate consideration to more sustainable weed-management programs (Kneževič et al., 2003; Owen and Zelaya,
2005; Owen, 2008a). Furthermore, unless growers collectively adopt more diverse weed-management practices, individual farmer’s actions will fail to delay herbicide resistance to glyphosate because the resistant genes in weeds easily cross farm boundaries. Some form of private or public collective action may avert this classic management of the commons problem in which individual actions to apply glyphosate have adverse spillover effects on the entire community of farmers (Hardin, 1968). Further research that results in new HR traits and other efficient means of weed control likely will lessen this problem.
Potential for Biofuels
Amid diminishing reserves of fossil fuels, heightened concern about climate change, and growing demand for domestic energy production, biofuels have emerged as an important supplementary fuel that may have considerable potential in supplying future energy needs. First-generation biofuels are serving as fuel extenders, displacing only a small percentage of gasoline consumption in the United States (Energy Information Administration, 2007). Nevertheless, because some existing biofuel crops can be produced in a manner that reduces greenhouse-gas emissions relative to fossil fuels and because they reduce dependence on volatile oil import markets, governments around the world have supported production of biofuel crops with subsidies and mandates (Koplow, 2007). However, although, as noted, some biofuel technologies can reduce greenhouse-gas emissions (Farrell et al., 2006), that might not always be the case, depending on production practices (Fargione et al., 2008; Searchinger et al., 2008). Furthermore, when they increase the demand for food crops, biofuel production can contribute to higher prices and shortages of food like those witnessed in 2008 (Tyner and Taheripour, 2008; Sexton et al., 2009).
In the United States, ethanol is produced principally from corn, approximately 80 percent of which is grown with GE varieties. Similarly, biodiesel in the United States is produced almost entirely from soybean; about 92 percent U.S. acres in soybean produce GE varieties. If those GE crops have increased yields, they may reduce biofuel costs.
If developed appropriately, new GE-crop technologies could play an important role in ameliorating further the adverse economic and environmental impacts of biofuels (Sexton et al., 2009). Indeed, some governments, like that of the United States, have tailored their biofuels policy to include, among other instruments, some support for next-generation technologies to overcome existing limitations (e.g., Rajagopal et al., 2007; Rajagopal and Zilberman, 2008). For example, genetic-engineering technology may help to improve the agronomic characteristics of plants used
for cellulosic ethanol, which are not yet widely commercialized. Whereas ethanol is produced today only from the starch in plants, developments in microbiology allow the cellulosic material to be converted to biofuel. Genetic-engineering technology holds the potential to generate higher yields of these crops and to improve the amount of liquid fuel obtainable per plant by altering plants’ genetic code in beneficial ways. It may provide these benefits without environmental damage. Because many of the plants that may provide fuel in the future have not been commercially farmed, it may be possible to improve plant genetics to maximize their energy-yield potential, minimize the costs of converting cellulosic plant material to liquid fuel, and devise best-management practices or mitigation for the environment.
RESEARCH PRIORITIES RELATED TO GENETICALLY ENGINEERED CROPS
Water-Quality Monitoring and Evaluation
Nonpoint pollution is the leading cause of water-quality impairments across the United States, extending into ocean estuaries, bays, and gulfs (US-EPA, 2007). Agriculture remains the largest source of these nonpoint pollution flows by volume, and much of the pollution stems from cropland operations. The predominant contaminants include sediment from land erosion and nutrient and pesticide residues not used or retained for growing crops. For example, a recent analysis estimated that agriculture contributes 70 percent of the nitrogen and phosphorus that enters the Gulf of Mexico and that corn production accounts for the majority of the nitrogen and corn and soybean production account for one-fourth of the phosphorus (Alexander et al., 2008). Particularly in view of the huge dead zone that has formed in the Gulf of Mexico, lessening such pollution has high national priority.
As explained in Chapter 2, evidence has begun to emerge that GE crops are often associated with changes in cropping practices that should lead to an improvement in the nation’s water quality. The changes include shifts to conservation tillage or no-till techniques that leave more residues on the cropland surface and thereby reduce water runoff that contains sediment, nutrient, and pesticide contaminants. They also include the use of pesticides, such as glyphosate, that are less toxic and more quickly degrading than conventional crop herbicides and insecticides. The latter effects could also reduce contamination of groundwater and wells on farms from spills and mixing operations.
Because monitoring and research resources have been inadequate, those potential water-quality impacts of GE crops have not been doc-
umented. The committee received testimony from the U.S. Geological Survey that explained the lack of comprehensive data and analysis that could identify and estimate the magnitude of the potential improvements in water quality (Gilliom and Meyer, personal communication). Those effects are among the largest environmental effects of agriculture, and we recommend that more resources be devoted to the important tasks of spatial and temporal monitoring of how agricultural practices influence water quality. Monitoring changes associated with the adoption of GE cultivars is important given that the rapid, widespread adoption of those cultivars may have large impacts on water quality by changing agricultural practices. The resulting information could influence the design of future environmental policies and agriculture programs. Such critical intelligence would help to improve the efficacy and cost-effectiveness of achieving regional and national water-quality standards, and thereby improve farm sustainability.
Social Issues in the Use of Genetically Engineered Crops
Accumulated research in the social sciences has verified that the processes of technological development and diffusion do not take place in a social vacuum. Choices made by those who create new technologies and decisions made by others regarding whether to use the technologies are influenced by political, economic, and sociocultural factors. The social impacts of the use of the technologies are influenced by the same factors. A particular technology can have dissimilar social impacts depending on the context within which it is adopted. It is reasonable to hypothesize, on the basis of the existing body of knowledge, that the adoption of particular genetic-engineering technologies has a variety of social, economic, and political impacts, and that these impacts would not be the same at all times and in all regions and cultures.
As noted in Chapter 4, the amount of research on the social processes and effects associated with the development and use of GE crops has been inadequate and has not matched what took place previously in the cases of agricultural mechanization or even the use of bovine somatotropin in dairy production. Thus, there is little empirical evidence to which the present committee can point to that delineates the full array of social impacts of the adoption of GE-crop technology. That includes a lack of research on the social impact on farmers of companies legally enforcing their intellectual-property rights in GE seeds. Research that has been conducted on the role of industry–farmer social networks in influencing the development of new seed technologies, legal issues related to GE-crop technology, resistance to the use of the technology in organic production systems, and the development of the open-source breeding movement
does suggest, however, that genetic-engineering technology is socially contested by some groups. Those findings and trends reinforce the need for research on the social processes associated with genetic-engineering technology, including its social impacts, to inform the decision-making processes of technology developers, farmers, and policy makers. The committee recommends the development of this research agenda, which should lead to findings important for addressing the sociocultural issues that will arise in connection with broader adoption of GE crops in the future.
ADVANCING POTENTIAL BENEFITS OF GENETICALLY ENGINEERED CROPS BY STRENGTHENING COOPERATION BETWEEN PUBLIC AND PRIVATE RESEARCH AND DEVELOPMENT
The rapid adoption by U.S. farmers of the first generation of GE soybean, cotton, and corn varieties illustrates the speed and scope with which agricultural systems can be improved if appropriate products and systems are available. This report documents how GE varieties contribute to the sustainability of agriculture related to the production of those major crops. Expanding the effects to additional crops and further improving the technology will require an expansive program of R&D. Private companies are already working to develop additional traits that will improve the productivity and sustainability of agriculture in the United States and worldwide. However, both the private and public sectors must play vigorous, if often times different, roles if the full potential of genetic-engineering technology to foster a more sustainable agriculture is to be realized. In developing analogous traits for other crops, such as GE varieties of “minor” crops and additional GE traits to meet broader public environmental and social objectives (e.g., improved water quality and carbon sequestration), the active involvement of universities and nongovernment institutions will be crucial given the flexibility of such institutions in selecting research objectives when funding is available. Developing the most appropriate agenda for such research will require extensive stakeholder involvement, including input from adopters and nonadopters of GE crops, environmental and social interest groups, and industry representatives.
Public investment and innovation in genetic-engineering technology includes several phases, such as discovery, scaling up of innovations, regulatory research, commercialization, production, and marketing. Life-science innovations increasingly take place within the educational– industrial complex, where research universities and public research institutions are engaged mainly in the early stages of innovation, and startup and major corporations are engaged more in product development (Graff
et al., 2009). Much of the academic research addresses basic problems with uncertain outcomes that may result in new commercial innovations or in knowledge that has either pure public-good properties in the economic sense, such as basic-research discoveries that are nonrival and non-exclusive (Just et al., 2008), or properties that are not easy to appropriate, so revenues cannot be collected. Thus, such academic research will receive underinvestment by the private sector and warrants public-sector support (Dasgupta and David, 1994; Dasgupta, 1999).
Not all discoveries from academic research in genetic-engineering technology result in nonrival and nonexcludable products. For example, some scientists patent their innovations; these are then excludable products that can be licensed to other scientists, nonprofit organizations, or industry. A national survey in 2004 revealed that about 25 percent of responding scientists had filed for a patent since 2000. About 15 percent of the scientists had been issued a patent, and just under 8 percent had licensed their invention for use by private or public parties (Buccola et al., 2009). The responding scientists expressed slight support for patenting compared to the belief that publicly supported scientists should focus on knowledge with nonexcludable benefits (a mean of 2 versus a mean of 5 on a 6-point scale) (Buccola et al., 2009). Some scientists may be inclined to patent their discoveries in case they contribute to future, commercially developed technology.
In those cases where university discoveries and innovations are basic proofs of concepts, the private sector often undertakes the development effort, upscaling manufacturing capacity and commercializing the technology. Companies that invest in development of university innovations frequently buy rights to university patents to secure intellectual-property protection and monopoly power once the products are developed. Without that protection, the firms may be unwilling to invest the capital necessary to move the technology to the commercialization stage.
There is likely to be underinvestment in commercialization of biotechnology innovation by the private sector because companies with implicit monopoly rights associated with patents aim to maximize their own profit without taking full account of consumers’ welfare gains that result from the lower prices associated with an innovation. Firms cannot capture the potential benefits external to farmers and consumers, such as reduction in downstream pollution. The underinvestment in research makes a case for public-sector development of GE varieties as long as social benefits exceed social costs or social-equity objectives defined by elected representatives are achieved (de Gorter and Zilberman, 1990; Just et al., 2008). The situation suggests that public-sector research should emphasize the development of genetic-engineering technologies in specialty crops and innovation of other kinds, such as traits that may lead to a reduction in
greenhouse-gas emissions from crop or livestock production or novel varieties that conserve water resources. The ability of the public and private sectors to develop new gene technologies depends on the costs of innovation, which may include access to intellectual-property rights and regulatory requirements. A tailored and targeted regulatory approach to GE-crop trait development and commercialization that meets human and environmental safety standards while minimizing unnecessary expenses could enhance progress on this front (Ervin and Welsh, 2006).
In reinforcement of those conceptual points, recent studies of genetic-engineering R&D have concluded that publicly funded research programs can complement private-sector R&D efforts in developing the full potential of agricultural biotechnology (Graff and Zilberman, 2001; Glenna et al., 2007; Buccola et al., 2009). There are several reasons for that conclusion. First, federal and state support encourages more basic research, whereas industry and foundations support more applied research in U.S. universities (Buccola et al., 2009). Downstream (i.e., more applied) research tends to be legally and economically more excludable than upstream (i.e., more basic) research. Publicly funded research offers the highest potential for achieving public goods, such as the basic science of genetic mechanisms, broadly accessible platform technologies, and nonmarket environmental services (Buccola et al., 2009). Second, industry collaborations with academic scientists have affirmed the necessity of a strong independent university research sector in helping to provide credible evaluations of new technology (Glenna et al., 2007). Third, both publicly and privately supported research assist in the transfer of basic discoveries in genetic-engineering technology, such as plant-genome characterizations, into useful crop-plant applications (Graff and Zilberman, 2001). For example, publicly supported basic research on Arabidopsis thaliana has provided an enormous store of information on basic plant biology, which in turn has enhanced our ability to produce commercially important advances in crops of all kinds. New institutional mechanisms are needed to provide an uninterrupted “pipeline” from basic research to field application (Graff et al., 2003). Fourth, commercialization of orphan and minor crops requires a special role for public R&D because the improvement of such crops often will not lead to sufficient profit to attract private-sector investment, even though the crops are important to many farmers and consumers. Fifth, public funding of academic research and government research fosters investigation that is too risky, or not sufficiently profitable, to be attractive to the private sector.
The evidence assembled in this report makes clear that the first generation of GE soybean, cotton, and corn varieties has generally been economically and environmentally advantageous for U.S. farmers who have adopted the technologies. The next generation of genetic-engineering tech-
nologies being reported by industry suggests that it is intent on enhancing those benefits and going beyond to new traits, such as drought and heat tolerance and enhanced fertilizer utilization that may indirectly reduce nutrient runoff, and new applicability to minor crops, renewable energy, climate change, and nutritional qualities. The public sector must complement industry by developing genetic-engineering technologies for crops that have insufficient markets to justify R&D and regulatory expense and to develop socially valuable public-goods applications. We envision the research and technology agenda to include individual private and public activities as well as private–public collaborations and to encompass work on the three essential components of sustainable development: environmental, social, and economic. Furthermore, we recommend that this agenda be undertaken in a program of research, technology development, and education that maximizes the potential synergies between the two sectors and their strengths and that limits redundancies and tradeoffs. Such an integrated approach would have universities, government, and nonprofit organizations leading in the development of traits that deliver public goods, including basic discoveries and such environmental issues as improved regional water quality. The private sector would continue to lead in the commercialization of GE crops for which there are adequate market incentives.
REFERENCES
Alexander, R.B., R.A. Smith, G.E. Schwarz, E.W. Boyer, J.V. Nolan, and J.W. Brakebill. 2008. Differences in phosphorus and nitrogen delivery to the Gulf of Mexico from the Mississippi river basin. Environmental Science & Technology 42(3):822–830.
Baker, H.G. 1991. The continuing evolution of weeds. Economic Botany 45:445–449.
Baum, J.A., T. Bogaert, W. Clinton, G.R. Heck, P. Feldmann, O. Ilagan, S. Johnson, G. Plaetinck, T. Munyikwa, M. Pleau, T. Vaughn, and J. Roberts. 2007. Control of coleopteran insect pests through RNA interference. Nature Biotechnology 25(11):1322–1326.
Bradford, K., J. Alston, and N. Kalaitzandonakes. 2006. Regulation of biotechnology for specialty crops. In Regulating agricultural biotechnology: Economics and policy. eds. R.E. Just, J.M. Alston, and D. Zilberman, pp. 683–697. New York: Springer.
Brookes, G., and P. Barfoot. 2009. Global impact of biotech crops: Income and production effects, 1996-2007. AgBioForum 12(2):184–208.
Buccola, S., D. Ervin, and H. Yang. 2009. Research choice and finance in university bioscience. Southern Economic Journal 75(4):1238–1255.
Cox, W.J., R.R. Hahn, P.J. Stachowski, and J.H. Cherney. 2005. Weed interference and glyphosate timing affect corn forage yield and quality. Agronomy Journal 97(3):847–853.
Cox, W.J., R.R. Hahn, and P.J. Stachowski. 2006. Time of weed removal with glyphosate affects corn growth and yield components. Agronomy Journal 98(2):349–353.
Dalley, C.D., J.J. Kells, and K.A. Renner. 2004. Effect of glyphosate application timing and row spacing on weed growth in corn (Zea mays) and soybean (Glycine max). Weed Technology 18(1):177–182.
Dasgupta, P. 1999. Science as an institution: Setting priorities in a new socio-economic context. Paper presented at the Plenary session on science in society at the UNESCO/ICSU world conference on science (Budapest, Hungary, June 26–July 1, 1999). Available online at http://www.econ.cam.ac.uk/faculty/dasgupta/science.pdf. Accessed September 16, 2009.
Dasgupta, P., and P.A. David. 1994. Toward a new economics of science. Research Policy 23(5):487–521.
de Gorter, H., and D. Zilberman. 1990. On the political economy of public good inputs in agriculture. American Journal of Agricultural Economics 72(1):131–137.
Energy Information Administration. 2007. Biofuels in the U.S. transportation sector. U.S. Department of Energy. Washington, DC., Available online at http://www.eia.doe.gov/oiaf/analysispaper/biomass.html. Accessed January 12, 2010.
Ervin, D., and R. Welsh. 2006. Environmental effects of genetically modified crops: Differentiated risk assessment and management. In Regulating agricultural biotechnology: Economics and policy. eds. R.E. Just, J.M. Alston and D. Zilberman, pp. 301–326. New York: Springer.
Ervin, D.E., R. Welsh, S.S. Batie, and C.L. Carpentier. 2003. Towards an ecological systems approach in public research for environmental regulation of transgenic crops. Agriculture, Ecosystems and Environment 99(1–3):1–14.
Fargione, J., J. Hill, D. Tilman, S. Polasky, and P. Hawthorne. 2008. Land clearing and the biofuel carbon debt. Science 319(5867):1235–1238.
Farrell, A.E., R.J. Plevin, B.T. Turner, A.D. Jones, M. O’Hare, and D.M. Kammen. 2006. Ethanol can contribute to energy and environmental goals. Science 311(5760):506–508.
Fernandez-Cornejo, J., and M.F. Caswell. 2006. The first decade of genetically engineered crops in the United States. Economic Information Bulletin No. 11. April. U.S. Department of Agriculture–Economic Research Service. Washington, D.C. Available online at http://www.ers.usda.gov/publications/eib11/eib11.pdf. Accessed June 15, 2009.
Gilliom, R.J., and M.T. Meyer. 2009. Personal communication to the Committee on the Impact of Biotechnology on Farm-Level Economics and Sustainability. February 26. Washington, DC.
Givens, W.A., D.R. Shaw, W.G. Johnson, S.C. Weller, B.G. Young, R.G. Wilson, M.D.K. Owen, and D. Jordan. 2009. A grower survey of herbicide use patterns in glyphosate-resistant cropping systems. Weed Technology 23(1):156–161.
Glenna, L.L., W.B. Lacy, R. Welsh, and D. Biscotti. 2007. University administrators, agricultural biotechnology, and academic capitalism: Defining the public good to promote university-industry relationships. Sociological Quarterly 48(1):141–163.
Gower, S.A., M.M. Loux, J. Cardina, S.K. Harrison, P.L. Sprankle, N.J. Probst, T.T. Bauman, W. Bugg, W.S. Curran, R.S. Currie, R.G. Harvey, W.G. Johnson, J.J. Kells, M.D.K. Owen, D.L. Regehr, C.H. Slack, M. Spaur, C.L. Sprague, M. VanGessel, and B.G. Young. 2003. Effect of postemergence glyphosate application timing on weed control and grain yield in glyphosate-resistant corn: Results of a 2-yr multistate study. Weed Technology 17(4):821–828.
Graff, G., and D. Zilberman. 2001. An intellectual property clearinghouse for agricultural biotechnology. Nature Biotechnology 19(12):1179–1180.
Graff, G., D. Zilberman, and A. Bennett. 2009. The contraction of agbiotech product quality innovation. Nature Biotechnology 27(8):702–704.
Graff, G.D., S.E. Cullen, K.J. Bradford, D. Zilberman, and A.B. Bennett. 2003. The public-private structure of intellectual property ownership in agricultural biotechnology. Nature Biotechnology 21(9):989–995.
Hardin, G. 1968. The tragedy of the commons. Science 162(3859):1243–1248.
Heard, M.S., C. Hawes, G.T. Champion, S.J. Clark, L.G. Firbank, A.J. Haughton, A.M. Parish, J.N. Perry, P. Rothery, R.J. Scott, M.P. Skellern, G.R. Squire, and M.O. Hill. 2003a. Weeds in fields with contrasting conventional and genetically modified herbicide-tolerant crops. I. Effects on abundance and diversity. Philosophical Transactions of the Royal Society of London B 358:1819–1832.
Heard, M.S., C. Hawes, G.T. Champion, S.J. Clark, L.G. Firbank, A.J. Haughton, A.M. Parish, J.N. Perry, P. Rothery, D.B. Roy, R.J. Scott, M.P. Skellern, G.R. Squire, and M.O. Hill. 2003b. Weeds in fields with contrasting conventional and genetically modified herbicide-tolerant crops. II. Effects on individual species. Philosophical Transactions of the Royal Society of London B 358:1833–1846.
ISB (Information Systems for Biotechnology), and USDA-APHIS (U.S. Department of Agriculture–Animal and Plant Health Inspection Service). 2009. Field test release applications in the U.S. Available online at http://www.isb.vt.edu/cfdocs/fieldtests1.cfm. Accessed June 5, 2009.
Johnson, W.G., M.D.K. Owen, G.R. Kruger, B.G. Young, D.R. Shaw, R.G. Wilson, J.W. Wilcut, D.L. Jordan, and S.C. Weller. 2009. U.S. farmer awareness of glyphosate-resistant weeds and resistance management strategies. Weed Technology 23(2):308–312.
Just, R.E., D.L. Hueth, and A. Schmitz. 2008. Applied welfare economics. Northampton, MA: Edward Elgar Publishing.
Kasasian, L., and J. Seeyave. 1969. Critical periods for weed competition. International Journal of Pest Management: Part A 15(2):208–212.
Kneževič, S.Z., S.P. Evans, E.E. Blankenship, R.C. Van Acker, and J.L. Lindquist. 2002. Critical period for weed control: The concept and data analysis. Weed Science 50(6):773–776.
Kneževič, S.Z., S.P. Evans, and M. Mainz. 2003. Row spacing influences the critical timing for weed removal in soybean (Glycine max). Weed Technology 17(4):666–673.
Koplow, D.N. 2007. Biofuels, at what cost? Government support for ethanol and biodiesel in the United States: 2007 update. Earth Track, Inc. Cambridge, MA.
Kruger, G.R., W.G. Johnson, S.C. Weller, M.D.K. Owen, D.R. Shaw, J.W. Wilcut, D.L. Jordan, R.G. Wilson, M.L. Bernards, and B.G. Young. 2009. U.S. grower views on problematic weeds and changes in weed pressure in glyphosate-resistant corn, cotton, and soybean cropping systems. Weed Technology 23(1):162–166.
Nieto, H.J., M.A. Brondo, and J.T. Gonzales. 1968. Critical periods of the crop growth cycle for competition from weeds. PANS: Pest Articles & News Summaries 14:159–166.
Owen, M.D.K. 2001. Importance of weed population shifts and herbicide resistance in the Midwest USA Corn Belt. In Pesticide behaviour in soils and water: Proceedings of a symposium organised by the British Crop Protection Council, pp. 407–412 (Brighton, UK, November 12–15, 2001). Farnham, Surrey, UK: British Crop Protection Council.
———. 2008a. Weed species shifts in glyphosate-resistant crops. Pest Management Science 64(4):377–387.
———. 2008b. Glyphosate resistant crops and evolved glyphosate resistant weeds—the need for stewardship. In Proceedings of the 5th International Weed Science Congress, p. 51 (Vancouver, Canada, June 23–27, 2008). ed. K. Hurle. International Weed Science Society.
Owen, M.D.K., and I.A. Zelaya. 2005. Herbicide-resistant crops and weed resistance to herbicides. Pest Management Science 61(3):301–311.
Rajagopal, D., and D. Zilberman. 2008. Environmental, economic and policy aspects of biofuels. Foundations and Trends in Microeconomics 4(5):353–468.
Rajagopal, D., S.E. Sexton, D. Roland-Holst, and D. Zilberman. 2007. Challenge of biofuel: Filling the tank without emptying the stomach? Environmental Research Letters 2(4).
Searchinger, T., R. Heimlich, R.A. Houghton, F. Dong, A. Elobeid, J. Fabiosa, S. Tokgoz, D. Hayes, and T.-H. Yu. 2008. Use of U.S. croplands for biofuels increases greenhouse gases through emissions from land-use change. Science 319(5867):1238–1240.
Sexton, S., D. Zilberman, D. Rajagopal, and G. Hochman. 2009. The role of biotechnology in a sustainable biofuel future. AgBioForum 12(1):130–140.
Shaw, D.R., W.A. Givens, L.A. Farno, P.D. Gerard, D. Jordan, W.G. Johnson, S.C. Weller, B.G. Young, R.G. Wilson, and M.D.K. Owen. 2009. Using a grower survey to assess the benefits and challenges of glyphosate-resistant cropping systems for weed management in U.S. corn, cotton, and soybean. Weed Technology 23(1):134–149.
Stahl, L. 2007. Application timing is key in optimizing returns. News and Information, May 14. Available online at http://www.extension.umn.edu/extensionnews/2005/applicationtiming07.html. Accessed July 8, 2009.
Swanton, C.J., and S.F. Weise. 1991. Integrated weed management: The rationale and approach. Weed Technology 5(3):657–663.
Tyner, W., and F. Taheripour. 2008. Biofuels, policy options, and their implications: Analyses using partial and general equilibrium approaches. Journal of Agricultural and Food Industrial Organization 6(2).
US-EPA (U.S. Environmental Protection Agency). 2007. National water quality inventory: Report to Congress; 2002 reporting cycle. October 2007. EPA 841-R-07-001. Washington, DC. Available online at http://www.epa.gov/305b/2002report/report2002305b.pdf. Accessed July 29, 2009.
———. 2009. Pesticide fact sheet: SmartStax™). Washington, DC. Available online at http://www.epa.gov/oppbppd1/biopesticides/pips/smartstax-factsheet.pdf. Accessed September 28, 2009.
Welsh, R., and L.L. Glenna. 2006. Considering the role of the university in conducting research on agri-biotechnologies. Social Studies of Science 36(6):929–942.
World Bank. 2007. World development report 2008: Agriculture for development. Washington, DC: The World Bank.
Young, B.G. 2006. Changes in herbicide use patterns and production practices resulting from glyphosate-resistant crops. Weed Technology 20(2):301–307.