4
Characterization
The orbit of a near-Earth object (NEO) determines whether it will or will not strike Earth. Sufficiently accurate orbital information additionally determines the place and time of an impact, should one be predicted to occur. However, the physical outcome of an impact and its effects on people and property depend on many factors. Mitigation efforts (Chapter 5) are likewise predicated on the basis of many more properties of the NEO than its orbit alone.
The chief factors governing the effects of an NEO impact are the NEO’s mass and speed at impact. These properties determine the amount of energy delivered by the strike (this energy is proportional to the NEO’s mass multiplied by the square of its speed at impact). Other factors include the angle of the NEO’s approach to Earth’s surface and the NEO’s density, diameter, composition, and internal structure. Different mitigation strategies require a knowledge of different NEO properties, as discussed in Chapter 5.
Characterization encompasses the determination of all relevant properties of an NEO beyond its orbit. Some properties of an NEO that can be determined remotely, such as its brightness at several wavelengths, can be related to its diameter and composition. Other NEO properties, such as mass, porosity, or strength, may require a visit by a spacecraft and in situ investigation to determine.
This chapter examines the properties of an NEO that can be determined from ground investigations, using both optical telescopes and radar, as well as the utility of in situ studies by spacecraft. Additional information on both impact effects and properties of small asteroids as a class can be obtained from the study of airbursts that occur when these objects enter Earth’s atmosphere.
GROUND-BASED REMOTE CHARACTERIZATION
Ground-based characterization efforts can establish some aspects of the physical nature of individual NEOs and of the NEO population. However, detailed knowledge of the physical properties of the NEO population lags far behind the current rate of NEO discoveries: Considerable effort is required to collect information about these bodies not only to obtain a better understanding of the NEO population, but also to understand how the physical and compositional properties vary from one NEO to another. Such information is important for assessing the hazard potential of individual NEOs that may threaten Earth and the viability of proposed mitigation strategies.
A majority of the work supported under NASA’s NEO Observations Program to date has focused primarily on the detection and orbit determination of NEOs. These are necessary steps in the effort to assess the potential impact threat from such objects: The object’s orbit determines whether or not it is a threat to Earth.
The optical brightness of an NEO also provides a very rough estimate of its equivalent diameter, as noted in Chapter 3. For example, the albedo (reflectivity) of the NEO must be known or assumed in order for its size to be estimated. The variations in albedo from one NEO to another (Binzel et al., 2002) are such that the average assumed value leads, in “extreme” cases, to an uncertainty in diameter of about a factor of two. Furthermore, because small asteroids can be irregular in shape, it is possible to get a biased idea about the size of a small asteroid if it is observed on only one or two occasions from atypical vantage points as the asteroid rotates.
Radar investigations are exploring the physical properties of individual NEOs, including their sizes, shapes, surface roughness, rotation periods, and rotation pole orientations, as well as whether they have satellites. In addition, time variations of brightness as NEOs spin (“light curves”) are being used to identify body shapes, rotation periods, pole orientation, and the presence of satellites.
The change in the amount of light reflected by an NEO as a function of wavelength (color) of the light provides information on the composition of the NEO. Such “spectra” range in precision from the measurement of the brightness in a few broad wavelength bands, a technique permitting a classification of NEOs into a small number of groups of similar composition, to studies that acquire brightness information over a large number of narrow wavelength intervals. Such spectra can be compared to suites of laboratory spectra of meteorites and minerals to accurately determine the composition of the surface of an NEO. Which technique can be used is determined by the brightness of the object, the size of the telescope used for observation, and the time devoted to such observations. Classification and detailed spectral studies have begun to yield information on the types of minerals present in these objects, which thus lends qualitative insights into their physical strengths, internal structures, and bulk densities.
NEOs are more challenging to observe than are planets and their moons. NEOs tend to come into telescopic range for only short times (approximately a few days to weeks) and they often appear either low in the sky, along the star-crowded Milky Way, or during times when the Moon creates background light; conditions at discovery are thus not always optimal for detailed characterization efforts. Nevertheless, the best opportunity to characterize a given NEO occurs when it is optically bright during close Earth approaches, often when the NEO is discovered. Because the telescopes used to discover NEOs spend their time searching for them, follow-up observations for characterization must be done by other telescopes that can afford to devote the necessary time to this effort. However, few optical telescope facilities routinely provide observing time for the physical characterization of NEOs. (Radar characterizations of NEOs are discussed below.) Even these few efforts are not well coordinated. Therefore, many observable NEOs are not characterized in the detail necessary to allow the development of a better understanding of these objects as a population or the study of the individual objects that present the greatest threats to Earth.
Finding: The best opportunities for the physical characterization of most NEOs occur during close Earth approaches when these objects are optically bright. Existing programs of ground-based optical observations for the characterization of NEOs are few in number and are not coordinated among different observing teams. Many observable NEOs are not characterized.
THE ROLE OF RADAR IN THE CHARACTERIZATION OF NEAR-EARTH OBJECTS
Radar observations are complementary to optical measurements. The power of radar derives principally from the precision of its measurements: In optimum conditions, radar can determine the distance (“range”) to a target many millions of kilometers away with approximately 10-meter accuracy, and simultaneously measure speed in the direction toward Earth (“radial velocity”) to within 1 millimeter per second, while optical techniques locate the object’s angular position in the sky to about a few tenths of a second of arc (the angle formed by a penny viewed face on from about 15 kilometers away) under the best conditions. Both radar-derived range and velocity data and optically derived angular positions are used to estimate the orbit, which enables the computation of past and future trajectories.
Optical data alone, taken over a span of a few days after an asteroid is discovered, typically yield orbital predictions whose accuracy in distance and radial velocity can be improved by factors of up to several thousand when combined with radar data from the same interval. This rapid improvement provides an early and accurate assessment of future threat and is one of the most important roles for radar observation of NEOs. Radar observa-
tions, when feasible to make (see below), can extend reliable orbit prediction by centuries and, for threatening objects, can distinguish between a potential hit and miss much sooner than is possible with optical observations alone. For objects observed only when discovered, radar has added an average of 300 years to the interval over which accurate orbit prediction is possible (Ostro and Giorgini, 2004). Even for objects observed for many years, radar distance and radial velocity measurements can reduce uncertainties significantly and improve NEO orbits (Ostro and Giorgini, 2004).
A radar telescope is not an instrument that can be used to discover NEOs; however, it requires that the orbits be known well enough to “point” the telescope in four dimensions.1 It is a powerful tool for rapidly improving the knowledge of the orbit of a newly found object and thus for characterizing its potential hazard to Earth. In addition to orbit improvement, the interaction of radar signals with the surface of the NEO yields information about its physical characteristics. For example, radar observations can be used to estimate the roughness of the top several tens of centimeters of a NEO’s surface. Radar reflectivity measurements can distinguish between stony and metallic compositions and may be used to estimate the porosity of NEO surfaces.
Understanding asteroid composition is important for developing mitigation techniques. Radar observations have been used not only to estimate asteroid compositions but also to distinguish smoothly rotating from tumbling asteroids, as well as objects that appear to be monolithic fragments broken off from an originally larger parent. Some targets appear to be weakly bound “rubble piles,” while others display either spheroidal, highly elongated, or irregular shapes.
Similarly, radar observations yield direct information as to whether the NEO has a satellite and, if so, provide data about the size, rotation, and surface scattering properties of each member. In many cases where the echo is strong enough, radar may provide detailed images of an asteroid’s shape at both large and small scales (Figures 4.1, 4.2, and 4.3).
When observations of many rotational phases and geometrical aspects can be obtained, radar images can be used to reconstruct an asteroid’s size, shape, and spin state with a level of detail otherwise obtainable only by a spacecraft rendezvous. An asteroid’s shape provides fundamental information on its origin and geologic history and provides clues to its internal structure and bulk porosity. Three-dimensional shapes are available for about 25 NEOs from radar data, while several dozen more are potentially obtainable from data already in hand.
Detailed three-dimensional models open the window to other useful scientific investigations, such as estimated surface slopes and regolith distributions, as well as enabling the advance planning of spacecraft missions in close orbit about an NEO. These investigations may enhance spacecraft navigation and targeting on the NEO and are useful for realistic simulations of impacts and orbit-change scenarios involved in mitigation planning.
Arecibo Radar Observatory
The Arecibo Observatory, located near Arecibo, Puerto Rico, is part of the National Astronomy and Ionosphere Center (NAIC) operated by Cornell University under contract with the National Science Foundation (NSF). Its chief feature is a fixed 305-meter-diameter spherical antenna, of which 225 meters are illuminated by radar waves in a way that allows coverage within 20° of directly overhead. Due to its location 18° north of the equator, Arecibo can observe objects between latitudes of −1° and +38°, and about 33 percent of the sy may be observed by allowing Earth’s rotation to move the telescope to point toward the desired celestial target. Arecibo can track an individual object for up to 2.9 hours per day. When combined with its 900 kilowatt (kW) of average transmitting power of waves with a length of 13 centimeters, this system is by far the most sensitive research radar in the world—about 20 times more sensitive than the Goldstone Solar Radar System described below, but at the cost of significantly reduced sky coverage.
Figures 4.1, 4.2, and 4.3 show examples of the quality of imagery that can be obtained with Arecibo’s radar. These images contain thousands of pixels covering the target NEO; their highest resolution greatly exceeds that available from any optical telescope on the ground or in near-Earth space and is matched only by “flyby” and
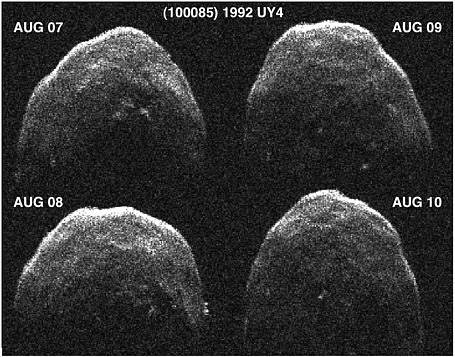
FIGURE 4.1 Arecibo radar images of the 2-kilometer-diameter near-Earth asteroid 1992 UY4 from 4 days of data obtained in August 2005. Illumination is from the top; range increases downward, and the wavelength of the echoes of the radio waves increases to the left; the Doppler frequency shift due to rotation affects the left-right positions of pixels. The resolution of each image is about 7.5 meters in each direction. The images reveal that 1992 UY4 is about 2 kilometers in diameter, with a rounded, slightly asymmetric shape, and that it has numerous topographic features. SOURCE: Courtesy of L.A.M. Benner, NASA, JPL.
exceeded only by rendezvous spacecraft missions. Because of its greater sensitivity, Arecibo provides significantly more frequent opportunities for high-resolution imaging than does Goldstone. Opportunities for radar imaging with a caliber comparable to those shown here occur several times annually. Within its latitude coverage, Arecibo can detect objects at twice the distance as can Goldstone for similarly sized objects and has contributed two-thirds of all radar range and radial velocity measurements on NEOs obtained in the last decade.
Observing time at Arecibo is awarded on a competitive basis from proposals that are normally submitted quarterly. Arecibo is also available for “urgent” target-of-opportunity observations on short notice and, in a small number of instances, has been used for radar observations of NEOs within 24 hours of their discovery.
Goldstone Solar System Radar
The Goldstone Solar System Radar, located in the Mojave Desert in southern California, is part of NASA’s Deep Space Network (DSN) and is operated by the Jet Propulsion Laboratory under contract with NASA. Comprising a fully steerable 70-meter-diameter antenna that can transmit 500 kilowatts of waves with a length of nearly 4 centimeters, this radar has a significant capability for observing echoes from NEOs. It can see approximately 80 percent of the total sky over the course of a day (i.e., every part north of −35° latitude).The Goldstone antenna’s primary mission is spacecraft communications, and it is available for astronomy observations only a few percent
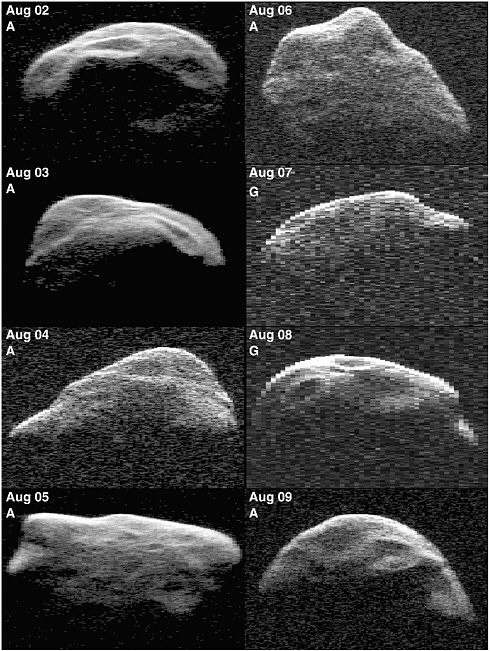
FIGURE 4.2 Arecibo (A) and Goldstone (G) radar images of near-Earth asteroid 1999 JM8. Illumination is from the top; range increases downward, and the wavelength of the echoes of the radio waves increases to the left. This asteroid is a very slow rotator, with a period of about a week. Each panel corresponds to a sum of images from the referenced 1999 August date. With a diameter of about 7 kilometers, 1999 JM8 is among the largest known near-Earth asteroids. SOURCE: L.A.M. Benner, S.J. Ostro, M.C. Nolan, J.-L. Margot, J.D. Giorgini, R.S. Hudson, R.F. Jurgens, M.A. Slade, E.S. Howell, D.B. Campbell, and D.K. Yeomans, 2000, Radar observations of asteroid 1999 JM8, Meteoritics and Planetary Science 37:779-792. Copyright 2002 by the Meteoritical Society.
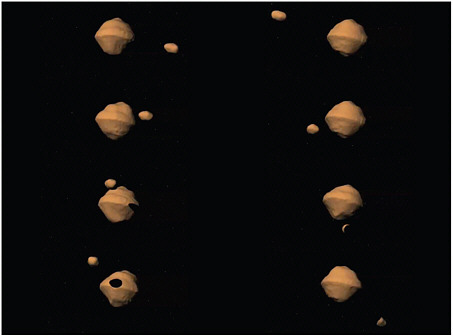
FIGURE 4.3 Renderings of binary near-Earth asteroid 1999 KW4 showing its satellite making one orbit. The figure shows three-dimensional models in shaded relief, reconstructed from a set of radar images obtained at Arecibo Observatory and Goldstone Observatory in 2001. The models are shown in their proper orientation as viewed from Earth. Radar imaging has shown that about 15 percent of NEOs larger than 200 meters in diameter have one (or sometimes two) satellites. SOURCE: S.J. Ostro, J.D. Giorgini, and L.A.M. Benner, 2006, Radar reconnaissance of near-Earth asteroids, pp. 143-150 in Near Earth Objects, Our Celestial Neighbors: Opportunity and Risk (A. Milani, G.B. Valsecchi, and D. Vokrouhlicky, eds.), Proceedings of the 236th Symposium of the International Astronomical Union, Prague, Czech Republic, August 14-18, Cambridge University Press. Copyright 2007 International Astronomical Union.
of its time. Goldstone is the only one of NASA’s three 70-meter telescopes (the others are in Spain and Australia) equipped with a high-power transmitter. The long-term future of Goldstone is uncertain; the DSN is considering decommissioning all of its 70-meter telescopes after 2015 and switching to an array of 34-meter-diameter telescopes. Whether a radar capability comparable to the present Goldstone capabilities would continue is unclear.
Capabilities of Arecibo and Goldstone
Because it is fully steerable, Goldstone can track objects significantly farther north and south than can Arecibo, and for up to several times longer per day. Limits on Goldstone’s coverage are also imposed by the requirement that targets be 20° above the horizon. Opportunities known well in advance are scheduled months or even years ahead. However, the Goldstone radar competes for telescope time with numerous NASA spacecraft missions that have higher priority and that often limit the time available for radar observations. The antenna is also available for short-notice target-of-opportunity observations if the flight projects have sufficient scheduling flexibility to accommodate changes, and if radiation clearance can be obtained in time from the numerous military and other government organizations whose airspace surrounds Goldstone. NEO radar observations have been scheduled in as few as 2 days after a request, but recent urgent requests have been at least 2 weeks in advance. In general, Arecibo has significantly greater flexibility for responding to short-notice target-of-opportunity observing requests than Goldstone has.
Radar images obtained at Arecibo and Goldstone can, respectively, now achieve resolutions as fine as 7.5 meters and 19 meters per pixel. Owing to its greater sensitivity and finer range resolution, Arecibo provides significantly more high-resolution NEO imaging opportunities than does Goldstone.
A recent JPL internal study found that, despite its restricted pointing capabilities, Arecibo is capable of observing up to two-thirds of newly discovered potentially hazardous nearby NEOs because these nearby objects move so rapidly across the sky that many pass through Arecibo’s latitude “window” before they exceed detectable range. The corresponding figure for Goldstone (whose detectable range on a given object is about one-half of Arecibo’s) is nearly the same. Arecibo is able to detect 12 percent more of the larger objects (about 700 meters in diameter) than Goldstone can, but 5 percent fewer of the smaller objects (about 70 meters in diameter) because of the smaller Arecibo “window” and shorter times for observation, as noted above.
In practice, most NEOs are observable at both Arecibo and Goldstone, but for the relatively small fraction that remain south of −1° or north of +38°, Goldstone is the only radar capable of observing them. Radar obserations at the two telescopes are often scheduled on different days (with those at Goldstone often on dates when targets are too far south or north for Arecibo), and which increases coverage of the different surface regions of the NEO, which is very important for three-dimensional shape determination. The capabilities of Arecibo and Goldstone are thus complementary, and many observing campaigns have made use of their synergy. Another primary advantage of having two radar facilities is that one can serve as a backup for the other. Mechanical problems or other demands on the facilities (particularly the need to use Goldstone to communicate with NASA spacecraft) mean that both facilities are rarely available simultaneously.
In the past several years, 20 to 30 NEOs have been observed with radar annually (average = 24), and since the first detection of an asteroid by radar in 1968, 252 near-Earth asteroids and 13 comets have been detected. Table 4.1 lists the number in several size ranges that have been observed.
NEOs have been selected for radar observations primarily on the basis of objects that are expected to yield the greatest scientific return on investment, which often means by providing high-resolution images that are suitable for three-dimensional shape reconstruction. In some instances, such as with the near-Earth asteroid Apophis (see Box 4.1), which was observed solely to improve its orbit, observations of targets with weaker radar echoes are scheduled. Many more NEOs are potentially detectable by radar than are scheduled, due to limitations in available telescope time, person-power, funding, scheduling conflicts, and equipment problems.
The ability of radar to detect echoes from a cosmic object is a complicated function of the object’s distance, diameter, rotation period, and radar reflectivity, and the telescope’s size and the transmitter power, as well as the length of the transmitted waves and the sensitivity of the receivers. The most important factor is the distance: the returned signal strength depends on (1/distance)4, so echoes will be 16 times weaker when the distance to a target doubles. Figure 4.4 shows radar echo strengths at Arecibo and Goldstone for a range of distances and sizes.
How many NEOs could be observed by radar annually? If a threshold is adopted that is suitable for detection and orbit improvement (e.g., Apophis) but much weaker than is necessary for obtaining high-resolution images, then in a 1-year interval starting in May 2008 about 410 NEOs could have been detected by radar if the factors discussed above were not an issue. Of these, 140 NEOs had already been discovered before May 2008, and 270 were found during the ensuing year. During that same interval, about 760 NEOs (other than Sun-grazing comets) were discovered, so in principle 270/760 = 36 percent of all new NEOs could have been observed by radar. Given in Table 4.2 is the number of NEOs in different size intervals that were detectable.
TABLE 4.1 Sizes of Asteroids Observed Annually by the Arecibo and Goldstone Radar Observatories
Diameter (D) Range |
Number |
Percentage |
D > 1 km |
92 |
36.5 |
0.5 km < D < 1 km |
68 |
27.0 |
0.2 km < D < 0.5 km |
32 |
12.7 |
D < 0.2 km |
60 |
23.8 |
BOX 4.1 Radar Observations of the Near-Earth-Object Apophis The near-Earth asteroid Apophis, which is approximately 300 meters in diameter, was discovered in March 2004, lost, and then rediscovered in December of that year. It quickly became clear that it would make a very close approach to Earth in 2029, and initial estimates of its orbit showed a significant probability of an impact. Further observations ruled out an impact in 2029. Apophis was observed at the Arecibo Observatory in Puerto Rico as a target-of-opportunity in January 2005, August 2005, and May 2006 solely to reduce uncertainties with respect to its orbit. The radar observations reduced the volume of the statistical uncertainty for the approach in 2029 by more than 90 percent, and they also revealed a bias in the analysis of the optical observations obtained in March 2004; the net effect was to shift the predicted 2029 encounter 4.4 Earth radii closer and only 5.6 Earth radii from the surface (Giorgini et al., 2008), a distance comparable to those of many communication satellites. During the radar observations, Apophis was between 0.19 to 0.27 astronomical units (AU) from Earth (1 AU is the average distance of Earth from the Sun) and a weak radar target. It is thus now known that Apophis cannot impact Earth in 2029, but an impact, although extremely unlikely, has not been ruled out for the approach in 2036. The primary sources of uncertainty are the physical properties of the asteroid and how, through interaction with sunlight, they propagate into orbit change. Apophis is an unusual case: These properties matter so much because the uncertainties grow enormously owing to this asteroid’s expected very close approach to Earth in April 2029. Thus, although the radar observations in 2005 to 2006 significantly improved the orbit, paradoxically, because the approach is so deep in Earth’s gravity well, the uncertainties in subsequent years are greatly magnified. Ignoring these sunlight effects leads to a probability of impact in 2036 of about 0.000002, but in practice this probability cannot be computed reliably due to uncertainties imposed by Apophis’s unknown physical properties, as mentioned above. Optical observations will be obtainable in 2011 and may be sufficient to exclude the possibility of a 2036 impact. If not, then radar observations at Arecibo or Goldstone when Apophis approaches Earth within 0.14 AU in 2013 should reduce uncertainties in the knowledge of the orbit substantially, with a high probability of completely ruling out an impact in 2036, and a very small probability of indicating a possible impact. |
During those 12 months starting in May 2008, 23 NEOs were observed by radar, so the number that could have been observed was about 18 times larger and substantially more than have been observed by radar in the past 40 years. Thus, Arecibo and Goldstone are grossly underutilized as radar observatories and could make much more substantial contributions than they do currently. Furthermore, when the Panoramic Survey Telescope and Rapid Response System (PanSTARRS) 1 begins regular operations, the number of NEOs discovered and thus detectable by radar should increase dramatically.
Finding: The capabilities of the Arecibo and Goldstone Radar Observatories are complementary, and many observing campaigns have made use of their synergy. One of the primary advantages of having two radar facilities is that one can serve as a backup for the other.
Finding: The number of NEOs observed by radar per year could be increased about fivefold by obtaining sufficient observing time.
Arecibo and Goldstone radar observations of more than 20 NEOs have revealed that surface roughness depends on composition and that very rough surfaces are common. Arecibo and Goldstone radar observations have also revealed that approximately 15 percent of NEOs larger than 200 meters in diameter have satellites orbiting about
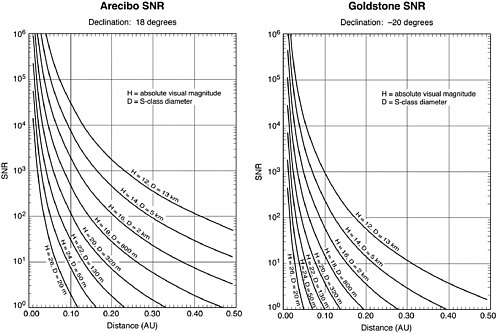
FIGURE 4.4 Signal-to-noise ratios (SNRs) for radar echoes received at the Arecibo Observatory and Goldstone Solar System Radar for several combinations of distances and sizes. “S-class” is a category of stony asteroids. SOURCE: Courtesy of Lance A.M. Benner, NASA, JPL.
TABLE 4.2 Number of Near-Earth Objects in Different Size Intervals That Are Detectable by the Arecibo and Goldstone Radar Observatories
Diameter (D) |
Number Detectable |
D > 1 km |
46 |
0.14 km < D < 1 km |
110 |
D < 0.14 km |
252 |
Total |
408 |
them (see Figure 4.3). This information is important for planning mitigation (Chapter 5). The first confirmed NEO “triple system” (a central rock has two smaller bodies in orbit around it) was discovered at Arecibo. Arecibo has discovered half of all known NEOs with satellites and observed almost all of these systems. Radar, with Arecibo in the lead, has become the most effective tool available for discovering that NEOs have satellites, and for estimating the mutual orbits, masses, sizes, and thus densities of each component.
Arecibo observations of the NEO 1950 DA suggested a small probability of impact with Earth in 2880. These observations demonstrated that the physical properties of an NEO are intimately coupled with long-term orbit prediction through the accelerations resulting from the absorption of sunlight and the asymmetric radiation of heat from the NEO due to its rotation (Giorgini et al., 2002), as well as the direct pressure exerted by sunlight on
TABLE 4.3 Numbers of Near-Earth Objects with Known Physical Properties
|
Number |
Near-Earth objects currently known |
6,278 |
Rotation periods |
450 |
Rotation pole directions |
25 |
Detected by radar |
246 |
Shapes estimated from radar data |
25 |
Shapes estimated from optical data |
14 |
Shapes estimated from spacecraft data |
2 |
Masses estimated from spacecraft data |
2 |
Masses estimated from radar data |
4 |
Bulk densities estimated from all sources |
10 |
Size estimated from all sources |
108 |
Near-surface densities estimated from radar |
17 |
the NEO. The importance of these effects depends on the NEO mass, thermal properties, size, shape, and rotation. Arecibo and Goldstone radar observations led to the first detection of such effects for asteroid Golevka and provided an estimate of its density and mass; this is one of only a handful of NEOs for which a mass estimate is available (see Table 4.3).
Operational Reliability of Arecibo and Goldstone
Until recently, Arecibo has proven a more dependable radar facility than Goldstone because of fewer equipment problems interfering with scheduled observations. That situation has recently changed, largely because of aging on-site primary power turbine generators at Arecibo (commercial power for the operation of extremely high power transmitters there is not practical). Because of turbine degradation, Arecibo has been unable to guarantee its full nominal power output of 900 kilowatts for several years; by the fall of 2008 the turbine generator had become progressively less reliable, forcing a reduction of power to approximately 500 to 600 kilowatts, and by the spring of 2009 to only about 60 kilowatts, which caused the cancellation of many NEO radar observations. The government of Puerto Rico has appropriated money for a new, more reliable generating source using diesel engines, but installation of this system is not expected to start until 2010.
Goldstone has also experienced significant equipment problems, most notably with its transmitter, which reduced operations to half power for several months in late 2008, but has recently resumed operating at its nominal power of 430 kilowatts. Keeping the approximately 45-year-old DSS-14 antenna operating is an increasingly important issue; Goldstone is scheduled to go “off-line” for 7 months of maintenance starting during 2010.
Arecibo and Goldstone Radar Operating Costs
The Arecibo and Goldstone radar systems are currently operational (with the caveats on transmittal power noted above), but neither is funded for dedicated observations of NEOs. The annual cost for Arecibo to carry out up to 300 hours of radar observations plus adequate maintenance is estimated at $2 million (approximately $1 million for the cost of purely radar operation [fuel, salaries, and so on] and $1 million for radar’s pro rata share of maintaining the antenna and facility). In 2008 Arecibo devoted about 240 hours to NEO observations. If the radar observations at Arecibo increased, say, to about 500 hours, then the associated operational cost would rise to about $3 million.
Arecibo could carry out radar observations at a significantly higher rate than it does currently if additional time and funding were available. At Goldstone the situation is different, because Goldstone’s primary mission is spacecraft communication, although if the Deep Space Network decommissions the DSS-14 antenna, considerably
more time could be obtained by converting Goldstone to a dedicated radar facility, but at a greatly increased cost since the whole facility would then be charged to the radar budget.
The 2004 Goldstone NEO budget request was $2.4 million, which would have supported a robust observing program. Only $2 million was appropriated, and since then the budget has dropped to about $1 million annually. Since 2002, Goldstone has devoted an annual average of about 200 hours to observing NEOs, which constitutes 2.3 percent of all time available on this telescope. During this interval, the number of hours scheduled for NEO radar observations declined by about 50 percent, and the fraction of scheduled time that was used for data acquisition declined from about 78 to 63 percent due to increasing difficulty with maintaining different components of the system.
Recent Funding History of the Arecibo Radar
In the 1990s the NSF and NASA funded a $25 million project that increased Arecibo’s sensitivity by approximately 20-fold. NASA contributed $11 million to provide new equipment that doubled the transmitter power to 900 kilowatts. This funding followed a history of NASA support for radar observations at Arecibo dating back to the 1970s and was particularly aimed at improving radar observations of near-Earth asteroids. Following the completion of this project in the late 1990s, NASA provided about $600,000 annually for a few years to support fuel costs, salaries, and the maintenance of the Arecibo radar.
Late in 2001 NASA sent a letter to the NAIC which indicated that funding for the radar would be eliminated in calendar year 2002. This deadline was subsequently relaxed, and the NAIC was instead asked to submit a proposal to NASA for continued funding. In consultation with NSF, NASA began reducing Arecibo’s funding in fiscal year (FY) 2003 and eliminated it at the end of FY 2005. NAIC has continued to operate the radar using existing funds but at the expense of adequate maintenance of the radar system.
In late 2006, the National Science Foundation convened a senior review that issued a report on observatories funded by NSF. No solar system scientists served on the panel, which recommended annual reductions in funding at Arecibo to a level that would merely permit the completion of several (non-radar) astrophysical surveys that still had a few years to run. That panel recommended that unless funding outside the NSF could be secured, the observatory be closed and decommissioned. According to a March 2009 report by the Congressional Research Service, costs for decommissioning the facility have ranged from $170 million to $200 million (Matthews, 2009), more than the cost of a decade’s total operations of the facility. Because of budgetary commitments for essential maintenance, NAIC was forced to cut Arecibo’s operating budget by 24 percent almost immediately following the senior review, but continued to operate the radar within its reduced budget. However, due to continuing budget cuts, NAIC stated that it would soon be necessary to cease operations of the radar altogether in order to provide sufficient funds for the observatory to complete the recommended astrophysical surveys. Currently NAIC is committing to operating the radar only through FY 2010.
Finding: Radar cannot be used to discover NEOs, but it is a powerful tool for rapidly improving the knowledge of the orbit of a newly found object and thus characterizing its potential hazard to Earth.
Finding: The Arecibo and Goldstone radar systems play a unique role in the characterization of NEOs, providing unmatched accuracy in orbit determination and offering insight into size, shape, surface structure, and other properties for objects within their latitude coverage and detection range.
Finding: Congress has directed NASA to ensure that Arecibo is available for radar observations but has not appropriated funds for this work.
Recommendation: Immediate action is required to ensure the continued operation of the Arecibo Observatory at a level sufficient to maintain and staff the radar facility. Additionally, NASA and the National Science Foundation should support a vigorous program of radar observations of NEOs at Arecibo, and NASA should support such a program at Goldstone for orbit determination and the characterization of physical properties.
CHARACTERIZATION ISSUES FOR AIRBURSTS
Airbursts created by the entry into Earth’s atmosphere of NEOs with diameters up to a few hundred meters both pose a serious threat at the larger end of the size range of the NEO and offer a unique opportunity to deduce physical characteristics at the small end of the range. Observations of small airbursts have provided almost the only information existing on the bulk strength, density, and composition of small NEOs through their high-speed interaction with Earth’s atmosphere. Although kilometer-sized NEOs are not substantially affected by their atmospheric passage, knowledge of their density and probable strength is important for mitigation efforts, making the study of airburst phenomena a prime focus for characterization efforts.
The density of an NEO that enters Earth’s atmosphere is most often the main determinant of where its energy is released. Dense and physically strong bodies (e.g., solid bodies) will be more likely to penetrate the atmosphere intact and impact the surface of Earth. Although much of the energy from such impact events goes into crater formation and excavation, producing melt, ejecta, and seismic shaking and/or tsunamis in ocean events, a substantial fraction of its energy (perhaps as much as two-thirds for the event that produced Meteor Crater, Arizona; see Figure 2.1 in Chapter 2) is nevertheless deposited in the atmosphere. Objects up to a few hundred meters in diameter with low density or physically weak bodies (e.g., highly porous and strengthless rubble piles) are likely to be disrupted during atmospheric entry; all of the energy from such events will be deposited directly into the atmosphere, producing shock waves in the air and heat radiation that may cause more widespread damage on the ground than had the atmosphere been absent.
The most notable recorded airburst event occurred in a remote region of Tunguska, Siberia, in 1908 and knocked down or defoliated the trees over an area of more than 2,000 square kilometers. There is a range of estimates for the size of the object that caused this event. Several estimates place the object as approximately 100 meters in diameter. A recent study, as yet not reproduced, suggests that the event was caused by a small (approximately 30- to 50-meter-diameter) NEO exploding at relatively low altitude, about 10 kilometers up (Boslough and Crawford, 2008). Since smaller NEOs are thought to be far more numerous than larger ones, there is a reasonable expectation that the next markedly destructive Earth impact event will be an object in the size range of 30 to 50 meters in diameter.
Ground-based studies of NEOs using data on both rotation rates and satellites suggest that most NEOs larger than about 150 meters in diameter are rubble piles, while most smaller ones are monolithic, with enough long-term tensile strength to prevent them from flying apart. The larger objects that are weak rubble piles easily disintegrate during atmospheric entry and create airbursts that somewhat resemble high-altitude nuclear explosions. Smaller monoliths may still be dispersed by aerodynamic forces as these monoliths penetrate deeper into the atmosphere, and they may, or may not produce craters depending on the strength, density, and size of each monolith.
Recent data obtained by spacecraft sensors also indicate that many NEOs may be either composed of gravitationally bound rubble piles or physically weak materials. The investigation by Japan’s Hayabusa spacecraft of the NEO Itokawa suggests that this asteroid is a prime example of a rubble-pile object with significant porosity. The Hayabusa data show that Itokawa is very porous, having roughly the same porosity as sand, and would probably produce a very significant airburst if it impacted Earth.
Information from the U.S. Department of Defense’s (DOD’s) Earth-observing satellites has shown that high-altitude airbursts from relatively small (1- to 5-meter-diameter) objects occur on a regular basis. This key information shows, for the NEOs encountering Earth, how the number of these objects depends on their size. To date, none of these airbursts has produced appreciable damage. However, two well-observed airbursts have resulted in meteoritic material being recovered from the ground. The recent impacts of the Tagish Lake meteorite parent body over Canada (January 2000) and of asteroid 2008 TC3 over Sudan (October 2008) lend evidence to support the suggestion that airbursts are relatively common. In addition, these events lend some insights into the material composition of these NEOs. The meteorites recovered from these two airbursts are composed of carbon-rich materials, which suggest that their parent bodies were objects composed of physically weak materials compared to those of other meteorite types (e.g., iron-rich materials). This information, along with the substantial fraction of NEOs with satellites, suggests that many subkilometer-sized NEOs are rubble piles or composed of physically weak materials. Therefore, any such NEO found to have an Earth-impacting trajectory would likely deliver its impact energy in the form of an airburst.
Airbursts are also detected by the arrays of microbarographic sensors deployed by the DOD and the Comprehensive Test Ban Treaty (CTBT) Organization. This international network, called the International Monitoring System, consists of seismic, infrasound, radionuclide, and hydroacoustic stations. The data are not publicly available; the scientific community would benefit from unfiltered access to the data produced by these arrays.
One of the least understood aspects of the airburst phenomenon is whether and how these events play a role in the formation of tsunamis. There has been significant debate on the effects of ocean impacts, both by direct impact into and by airbursts above the water. Some investigators suspect that an airburst over an ocean may be much more devastating than a similar-sized impact event directly into the water. The modeling of direct oceanic impacts suggests that the impact splash is significant and will be detrimental to those nearby, but that the wavelength of the resultant waves generated is not of sufficient length to cause a tsunami. Other studies suggest on the contrary that even this type of impact may be enough to generate a tsunami-like phenomenon depending on the terrain that such impact-generated waves may encounter. Still others have found that, based on numerical simulations and on data from nuclear oceanic tests, tsunamis are not generated by impact events.
More recent work on airburst events over the ocean suggests that this too is an area of uncertainty. Previous investigations have treated these types of airbursts in a fashion similar to nuclear explosions that deliver their energy from a single point. If this treatment were correct, then the resultant blast waves would not produce a tsunami-type of event. However, a recent study suggests that NEOs entering the upper atmosphere and exploding there act more like a linear series of nearly simultaneous explosions (Boslough and Crawford, 2008). These blast effects are not as localized as those from the single source models, in which the momentum of the object is carried downward into the atmosphere and produces a shock wave. If the shock wave were sufficiently strong to depress a wide area of the ocean’s surface, the resultant rebound effect of the ocean would create a classic tsunami. Hence the threat from small NEO airbursts over the ocean might present their most significant hazard to humanity given that most of the world’s population is concentrated on or near oceanic coastlines.
Finding: U.S. Department of Defense satellites have detected and continue to detect high-altitude airburst events from NEOs entering Earth’s atmosphere. Such data are valuable to the NEO community for assessing NEO hazards.
Recommendation: Data from NEO airburst events observed by the U.S. Department of Defense satellites should be made available to the scientific community to allow it to improve understanding of the NEO hazards to Earth.
Finding: Preliminary theoretical studies on low-altitude atmospheric Tunguska-like airbursts from asteroids as small as 30 meters in diameter suggest that significant risk exists from these NEOs.
Finding: Current models for the generation of tsunamis by impacts into or airbursts above the ocean are not yet sufficiently reliable to establish threat levels to coastal communities.
Recommendation: Additional observations and modeling should be performed to establish the risk associated with airbursts and with potential tsunami generation.
IN SITU CHARACTERIZATION RELEVANT FOR MITIGATION
Detailed knowledge of the physical characteristics of several representative NEOs would improve understanding of the overall NEO population and help the design and implementation of the mitigation techniques that may be employed should an NEO threaten Earth (but that understanding may well not improve the knowledge of a specific object on an impact trajectory). Although the physical characteristics of an individual NEO that might strike Earth cannot be accurately predicted in advance, the knowledge of the range of possible characteristics will greatly aid in advance planning and might be essential if there is no opportunity to perform detailed characterization studies of the incoming NEO. Dedicated space missions such as Near Earth Asteroid Rendezvous (NEAR)
Shoemaker and Hayabusa have provided detailed information on two vastly dissimilar NEOs. NASA’s NEAR Shoemaker spacecraft visited one of the largest NEOs, Eros, in February 2000; the Japan Aerospace Exploration Agency’s Hayabusa probe rendezvoused with the subkilometer-sized asteroid Itokawa in September 2005. Both of these robotic missions generated much scientific interest in NEOs and revealed many intriguing surprises and new paradigms for asteroid scientists to consider. It is now apparent from just these two missions, and the suite of ground-based optical and radar observations of NEOs, that NEOs have a much wider range of internal structures, more diverse physical conditions, and more complex surfaces than had previously been realized.
Essential physical properties relevant for the mitigation of NEOs are best determined from dedicated spacecraft missions. Although ground-based observations can provide significant information about the physical properties of NEOs (e.g., rotation rates, size estimates, and composition), dedicated spacecraft missions to NEOs providing extended periods for observations and investigation close to NEOs obtain detailed characterizations of their rotational motions, masses, sizes, shapes, surface morphology, internal structure, mineral composition, and collisional history. The data collected from NEO characterization missions would also help to calibrate the ground- and space-based remote sensing data and may permit increased confidence in the remote classification of NEOs and their associated physical characteristics, which could inform future mitigation decisions.
Flyby missions are not well suited for these detailed types of investigations because of the limited time for performing observations during the spacecraft encounter. To attain the required details of an NEO’s physical characteristics for hazard mitigation, much more time must be spent near the NEO than is possible in a flyby in order to operate instruments making gamma-ray, x-ray, and other compositional measurements. Constraints on some surface characteristics and on the object’s mass can be obtained, but the uncertainties on the NEO’s physical properties obtained from a flyby encounter are far too large to be useful for hazard mitigation purposes. Such missions may be suitable for basic reconnaissance of the NEO population, but overall the data return relevant to mitigation is low relative to cost.
Continued efforts to obtain characterization data from ground-based studies are desirable, and spacecraft observations of representative NEOs are very important. Spacecraft characterization of any NEO for which orbit change is to be attempted is essential (see Chapter 5).
Finding: Dedicated flyby spacecraft missions to NEOs provide only limited information relevant for hazard mitigation issues.
Finding: Rendezvous spacecraft missions can provide detailed characterization of NEOs that could aid in the design and development of hazard mitigation techniques. Such in situ characterization also allows the calibration of ground- and space-based remote sensing data and may permit increased confidence in the use of the remote classification of NEOs to inform future mitigation decisions.
HUMAN MISSIONS TO NEAR-EARTH OBJECTS
During its deliberations, the committee was briefed on the possibilities of human missions to near-Earth objects. This subject also received attention during meetings of the Human Space Flight Review Committee and was mentioned as part of its “Flexible Path” option in its final report.
In the future, NASA’s Exploration Systems Mission Directorate may conduct human missions to one or more near-Earth objects. The committee identified no cost-effective role for human spaceflight in addressing the hazards posed by NEOs. However, if human missions to NEOs are conducted in the future, the committee recommends that their scientific aspects be maximized to provide data useful for their characterization.
Recommendation: If NASA conducts human missions to NEOs, these missions should maximize the data obtained for NEO characterization.
REFERENCES
Benner, L.A.M., S.J. Ostro, M.C. Nolan, J. Margot, J.D. Giorgini, R.S. Hudson, R.F. Jurgens, M.A. Slade, E.S. Howell, D.B. Campbell, and D.K. Yeomans. 2002. Radar observations of asteroid 1999 JM8. Meteoritics and Planetary Science 37(6):779-792.
Binzel, R.P., D. Lupishko, M. Di Martino, R.J. Whiteley, and G.J. Hahn. 2002. Physical properties of near-Earth objects. Pp. 255-271 in Asteroids III (W.F. Bottke, P. Paolicchi, R.P. Binzel, and A. Cellino, eds.). University of Arizona Press, Tucson.
Boslough, M., and D. Crawford. 2008. Low-altitude airbursts and the impact threat. International Journal of Impact Engineering 35:1441-1448.
Giorgini, J.D., S.J. Ostro, L.A.M. Benner, P.W. Chodas, S.R. Chesley, R.S. Hudson, M.C. Nolan, A.R. Klemola, E.M. Standish, R.F. Jurgens, R. Rose, A.B. Chamberlin, D.K. Yeomans, and J. Margot. 2002. Asteroid 1950 DA’s encounter with Earth in 2880: Physical limits of collision probability prediction. Science 296:132-136.
Giorgini, J.D., L.A.M. Benner, S.J. Ostro, M.C. Nolan, and M.W. Busch. 2008. Predicting the Earth encounters of (99942) Apophis. Icarus 193(1):1-19.
Matthews, C.M. 2009. The Arecibo Ionospheric Observatory. R40437. Congressional Research Service, Washington, D.C..
Ostro, S.J., and J.D. Giorgini. 2004. The role of radar in predicting and preventing asteroid and comet collisions with Earth. Pp. 38-65 in Mitigation of Hazardous Comets and Asteroids (M.J.S. Belton, T.H. Morgan, N.H. Samarasinha, and D.K. Yeomans, eds.). Press Syndicate of the University of Cambridge, Cambridge, Mass.
Ostro, S.J., J.D. Giorgini, and L.A.M. Benner. 2006. Radar reconnaissance of near-Earth asteroids, Pp. 143-150 in Near Earth Objects, Our Celestial Neighbors: Opportunity and Risk (A. Milani, G.B. Valsecchi, and D. Vokrouhlicky, eds.), Proceedings of the 236th Symposium of the International Astronomical Union, Prague, Czech Republic, August 14-18, Cambridge University Press, Cambridge, U.K.