9
Application of Vehicle Technologies to Vehicle Classes
INTRODUCTION
In conducting its assessment of technology applicability to different vehicle classes, the committee was guided by the following question included in the statement of task: “What are the estimated cost and potential fuel economy benefits of technology that could be applied to improve fuel economy of future passenger vehicles, given the constraints imposed by vehicle performance, functionality, and safety and emission regulations?” Note that applying technology to improve fuel economy and reduce fuel consumption should not be interpreted to mean simply attaching a component or subsystem that then achieves a subsequent reduction in fuel consumption. Such reductions in fuel consumption typically evolve through an incremental, evolutionary application of components, subsystems, and new power train or vehicle technologies.
Previous chapters of this report have provided technical summaries of current and advanced technologies that are currently being applied to vehicles, or developed for future vehicle applications. Other reports from the National Research Council (NRC) have also looked at the impacts of technologies for reducing fuel consumption—Appendix H provides a summary of other recent NRC studies related to light-duty vehicle technologies. Many of these technologies could, in principle, be applied to almost any vehicle. How ever, the intended use of the vehicle, its price range, consumer characteristics, emissions and safety standards compliance, and packaging constraints influence which technologies will see market penetration on different vehicle types.
Many of the technologies have already seen significant penetration into European or Asian markets where regulatory and market pressure, including significant taxation that results in high fuel prices for consumers, have encouraged early adoption. Others, such as turbocharged, direct-injection gasoline engines, have gained significant attention in the United States because fuel consumption can be reduced with minimal redesign of the total vehicle system.
DEVELOPING BASELINE VEHICLE CLASSES
The concept of dividing U.S. passenger vehicles into so-called classes is both an outcome of regulatory segmentation for the purpose of varying standards and a means whereby vehicle sales categories are differentiated by vehicle size, geometry, and intended use. The NRC CAFE report segmented U.S. passenger vehicle sales into 10 classes that were a subset of the larger number of type and weight classes that the U.S. EPA uses as part of its vehicle certification process (NRC, 2002). These 10 classes are as follows:
-
Small SUV,
-
Medium SUV,
-
Large SUV,
-
Minivans,
-
Small pickups,
-
Large pickups,
-
Subcompact cars,
-
Compact cars,
-
Midsize cars, and
-
Large cars.
The statement of task directs the current committee to evaluate these vehicle classes and update the technology outlook for future model introduction. However, shifts in consumer preference and vehicle sales have been significantly influenced by the recent instability in fuel prices, vehicle financing costs, U.S. and global economic conditions, and regulatory uncertainty. Significant shifts in vehicle sales between 2002 and 2007 showed a continuing increase in SUV sales, with sales of small pickups essentially disappearing (EPA, 2008a). However, in 2008, large increases in fuel price (above $4 per gallon of gasoline) resulted in a greater than 50 percent reduction in the sale of midsize and large SUVs. Subsequent U.S. and global instability in the financial markets, followed by a period of recession, has resulted in an overall reduction of vehicle sales in the United States of more than 20 percent from 2008 to 2009.
Therefore, the choice of vehicle classes for future consideration as part of this assessment of potential fuel economy technologies should focus on vehicle size, weight, interior passenger volume, intended use, and the potential for implementation of next-generation power trains, including hybrid electrics. Based on various factors outlined below, the following classification of light-duty vehicles in the United States was determined by the committee to be an appropriate basis for future technology development and introduction into production.
-
Two-seater convertibles and coupes—Small vehicles by interior volume whose function is high-performance and handling. The average 2007 model-year vehicle for this class was developed from EPA (2008a) and has the following characteristics: a six-cylinder, four-valve, dual overhead cam engine with intake cam phasing and a 6-speed automatic transmission. The average vehicle for this class is used as the base vehicle in the estimation of fuel consumption reductions for multiple technologies as discussed later in this chapter.
-
Small cars—Mini-, sub-, and compact cars, standard performance, mostly four-cylinder, mostly front-wheel drive (FWD), including small station wagons. The average 2007 model-year vehicle for this class was developed from EPA (2008a) and has the following characteristics: a four-cylinder, four-valve, dual overhead cam engine with intake cam phasing and a 6-speed automatic transmission. The average vehicle for this class is used as the base vehicle in the estimation of fuel consumption reductions for multiple technologies as discussed later in this chapter.
-
Intermediate and large cars—Standard performance, mostly FWD, mostly six-cylinder, including large station wagons with less than 0.07 hp/lb of vehicle weight. The average 2007 model-year vehicle for this class was developed from EPA (2008a) and has the following characteristics: a six-cylinder, four-valve, dual overhead cam engine with intake cam phasing and a 4-speed automatic transmission. The average vehicle for this class is used as the base vehicle in the estimation of fuel consumption reductions for multiple technologies as discussed later in this chapter.
-
High-performance sedans—Passenger cars with greater than or equal to 0.07 hp/lb of vehicle weight that are not two-seaters. The average 2007 model-year vehicle for this class was developed from EPA (2008a) and has the following characteristics: a six-cylinder, four-valve, dual overhead cam engine with intake cam phasing and a 6-speed automatic transmission. The average vehicle for this class is used as the base vehicle in the estimation of fuel consumption reductions for multiple technologies as discussed later in this chapter.
-
Unit-body standard trucks—Non-pickup trucks with unibody construction and hp/lb of vehicle weight ratios of under 0.055 including crossover vehicles, SUVs, and minivans. Most vehicles employ front-wheel drive. The average 2007 model-year vehicle for this class was developed from EPA (2008a) and has the following characteristics: a six-cylinder, four-valve, dual overhead cam engine with intake cam phasing and a 6-speed automatic transmission. The average vehicle for this class is used as the base vehicle in the estimation of fuel consumption reductions for multiple technologies as discussed later in this chapter.
-
Unit-body high-performance trucks—Crossover vehicles, SUVs, and minivans with hp/lb of vehicle weight ratios of 0.055 or greater. Most have rear-wheel drive (RWD) or all-wheel drive (AWD) and unibody construction, and most are luxury vehicles. The average 2007 model-year vehicle for this class was developed from EPA (2008a) and has the following characteristics: a six-cylinder, four-valve, dual overhead cam engine with intake cam phasing and a 6-speed automatic transmission. The average vehicle for this class is used as the base vehicle in the estimation of fuel consumption reductions for multiple technologies as discussed later in this chapter.
-
Body-on-frame small and midsize trucks—Pickups less than or equal to 1,500 lb payload capacity (CEC class 14) and SUVs of up to 175 cubic feet of passenger volume plus cargo volume with RWD or AWD. The average 2007 model-year vehicle for this class was developed from EPA (2008a) and has the following characteristics: a six-cylinder, two-valve, single overhead cam engine with a 5-speed automatic transmission. The average vehicle for this class is used as the base vehicle in the estimation of fuel consumption reductions for multiple technologies as discussed later in this chapter.
-
Body-on-frame large trucks—Pickups of greater than 1,500 lb payload but less than 10,000 lb GVW, and SUVs with 175 cubic feet or greater of passenger plus cargo volume with RWD or AWD, including all standard vans, cargo and passenger. The average 2007 model-year vehicle for this class was developed from EPA (2008a) and has the following characteristics: an eight-cylinder, two-valve, overhead valve engine with a 4-speed automatic transmission. The average vehicle for this class is used as the base vehicle in the estimation of fuel consumption reductions for multiple technologies as discussed later in this chapter.
These eight classes allow an evaluation of similar base vehicles designs, where the vehicle size, baseline chassis configuration, aerodynamic characteristics, vehicle weight and type of drive train (FWD, RWD, and AWD) are similar. This grouping should result in vehicle classes where similar calibration criteria are associated with similar vehicle performance characteristics. A greater number of classes would
also be possible if there was a desire to narrow the variability in vehicle characteristics in each class.
ESTIMATION OF FUEL CONSUMPTION BENEFITS
Incremental reductions in fuel consumption through the application of technologies were estimated by the committee. As discussed earlier in this report, input came from many sources including component suppliers, vehicle manufacturers, and the review of many published analyses conducted by, or for, the U.S. Department of Transportation National Highway Traffic Safety Administration (NHTSA), U.S. Environmental Protection Agency (EPA), California Air Resources Board (CARB), and other agencies or trade associations. The committee also contracted with several consultants to provide input.
Relative reductions in fuel consumption can result from several factors, many of which are interrelated:
-
Reduction in the tractive force needed to propel the vehicle (reduced rolling resistance, aerodynamic drag, vehicle weight, etc.);
-
Improvement in the energy conversion efficiency of the fuel in the engine into maximum usable energy through increased thermal efficiency (compression ratio increase for gasoline engines, lean combustion, diesel, etc.);
-
Reductions in the engine and power train energy losses that consume portions of the available energy before and after combustion (gas exchange losses, power train friction, accessory losses, etc.);
-
Optimization of operational parameters that allow the engine to run in regions of highest efficiency (increased number of transmission gears, CVTs, improved lugging characteristics, aggressive shift logic, etc.); and
-
Some form of hybridization that allows other forms of energy capture, storage, and management to reduce the total energy consumed over the driving cycle.
The committee thinks that the most accurate method of analyzing potential reductions in fuel consumption, which considers the extent to which any of the efficiency improvements or energy loss reductions identified above can be realized while maintaining energy balance criteria, utilizes full system simulation (FSS). This analysis technique, as described in Chapter 8, represents the state of the art in predicting vehicle performance, fuel consumption, direct CO2 emissions, and other regulated and non-regulated emissions. However, FSS analyses require detailed vehicle, engine, transmission, accessory, and other subsystem data, typically expressed in the form of data maps that quantify power, torque, fuel consumption, and exhaust emissions over the complete range of operation. Historically, such data (which may not yet exist for the most advanced technologies) have been considered proprietary by automobile manufacturers (referred to as original equipment manufacturers; OEMs) and suppliers, such that only those companies associated with the design, development, and production of such systems have had the data to conduct such analyses. However, partnerships currently exist between the automotive industry and the U.S. government such that more complete experimental data will be made available in the future.
Another factor in successfully modeling full vehicle systems is the need to understand and capture the tradeoffs that OEMs must make in developing final production calibrations of vehicles and their power trains. Calibration is the process of power train and vehicle performance optimization that focuses on achieving predetermined performance, drivability, fuel consumption, durability, fuel octane sensitivity, and many other parameters while still complying with statutory requirements such as those for levels of emissions, onboard diagnostics (OBD), and safety standards. In particular, many potential technologies that can be applied for improving fuel consumption could influence performance parameters such as 0-60 mph acceleration times, vehicle passing capability, towing capability, transmission shift quality, or noise and vibration characteristics. Different manufacturers must thus determine their customer-preferred compromises and calibrate the vehicle control algorithms accordingly. Based on the number of potential parameters that may be varied in modern passenger car engines, tens of thousands of combinations are possible. Therefore, manufacturers and calibration service companies have developed optimization strategies and algorithms to fine-tune these variables while achieving an OEM’s criteria for performance and drivability within the constraints of emissions, fuel economy, and other standards. Calibration logic is normally a highly confidential process that requires the experience of companies involved in the production release of vehicles (OEMs, Tier 1 suppliers, production engineering services companies, etc.) to accurately assess the necessary performance, fuel consumption and exhaust emission, and drivability tradeoffs for accurate modeling.
Partial discrete approximation (PDA) and lumped parameter modeling techniques, as described in Chapter 8, examine and estimate incremental reduction in fuel consumption associated with applications of discrete technologies or subsystems and their effect on reducing energy losses. They represent a more time- and cost-effective method of estimating potential reductions in fuel consumption and may incorporate routines that attempt to tabulate or account for aggregation of energy-loss reductions that focus on fluid mechanical losses, frictional losses, and heat transfer losses. However, the ultimate accuracy of such analyses relies on a sufficiently broad set of empirical or system-simulation data that do not necessarily provide enough detail to understand the base test vehicle distribution of energy losses. Calibration of such models against actual test vehicles provides a benchmark of the modeler’s attempt to match performance
data, but does not provide the same level of explanation of the subsystem contribution to total vehicle energy losses that is accomplished in the FSS cases. Furthermore, the influences of variations in calibration strategies owing to such factors as driver comfort; noise, vibration, and harshness (NVH)-related issues; and performance/emissions tradeoffs are typically not considered in such analyses.
With either modeling approach, it is imperative to understand the role that any previously applied technologies play in reducing energy losses and/or improving the thermodynamic efficiency of the power train.
APPLICABILITY OF TECHNOLOGIES TO VEHICLE CLASSES
Not all of the technologies identified in Chapters 4 through 7 of this report can be justifiably applied to all vehicle types. Applicability of the technologies to the various vehicle classes requires an analysis of parametric tradeoffs which considers functionality, intended use, impact on warranty, ease of implementation, product cycle timing, market demand, cost-effectiveness, and many other factors. Some technologies may be discounted for technical reasons, for example, the limitations of continuously variable transmissions (CVTs) in transmitting high torque on vehicle classes with larger engines where towing or off-road capability is a primary product feature. Others may be excluded based on the intended purpose of the vehicle. For example, low-rolling-resistance tires appear to be a cost-effective means of reducing fuel consumption, potentially justifying their use on all vehicles. However, in higher performance classes of vehicles, where tire grip is an important product feature or for SUV applications where the vehicle may travel off-road, the use of such tires is likely restricted.
Table 9.1 shows the committee’s estimation of incremental reductions in fuel consumption that may be expected from the application of different technologies and ranges associated with the reductions. In general, the committee estimated what it considered to be the average fuel consumption reduction for a technology before it attempted to estimate the range. These data, shown in the form of ranges, are in some cases dependent upon the level of technology applied to a vehicle before the next increment is taken. As identified above, these data represent estimates by the committee developed from evaluating published data, and analyses conducted by the NHTSA, the EPA, and others. Appendix I contains results from some of these other studies, although the reader should refer to the original source for the assumptions and study approaches used in these other studies. The expert judgment of members of the committee whose careers have focused on vehicle and power train design and development were also incorporated in the estimates. Examination of the data in Table 9.1 suggests that significant variations in estimates of the potential for reducing fuel consumption are due to the lack of detailed simulation data on actual or theoretically modeled vehicles or power trains against which to refine the estimates. This variability in estimates for fuel consumption reductions also reflects the fact that different OEMs may obtain different benefits from the same technology due to differences in implementation and calibration. Also, positive benefits may vary depending on the particular engine/transmission/vehicle architecture. These factors have been considered by the committee in its range of estimates or its decision to include or exclude the potential application of technologies into different technology paths. Note that the ranges associated with these technologies do not reflect the possibility that, over time, the average fuel consumption benefit could tend toward the high end of the range as the lessons learned from the best examples of the technology spread across the industry and as the impacts of higher CAFE standards increase. Although the committee recognizes that the implementation of these technologies with fuel consumption benefits at the higher end of the ranges could occur, it is difficult to assert that this will occur or to what degree this would impact the average consumption benefit over time.
The issue of how multiple technologies might interact when used to reduce fuel consumption is critical. FSS analyses conducted by Ricardo, Inc., for the EPA and for the committee shed some light on the issue of synergistic interaction of multiple technologies that may attempt to reduce energy losses of a similar type, such as pumping losses (Ricardo, Inc., 2008, 2009). These analyses show the need to carefully understand the contribution of technologies in reducing losses whose impact may be only a 1 to 2 percent reduction in fuel consumption. The Ricardo, Inc., analyses also show that the type of vehicle and power train influences the extent to which different technologies reduce fuel consumption, especially between vehicles of different classes with different intended uses. This effect is discussed in Chapter 8, where the primary effect of synergies was shown to dominate the potential for improvement. Accordingly, secondary effects of influences that interact across technology improvement paths were found to be minor.
ESTIMATING INCREMENTAL COSTS ASSOCIATED WITH TECHNOLOGY EVOLUTION
Chapter 3 describes the methodologies used for the estimation of incremental costs associated with the introduction of advanced technology for reducing fuel consumption. A range of estimated costs was also prepared and is outlined in the technology sections presented in Chapters 4 through 7. Table 9.2 shows the collection of these cost estimates for all technologies included in this report. The cost estimates represent estimates for the current (2009/2010) time period to about 5 years in the future. As with the data on fuel consumption reductions, incremental cost information was provided to the committee by OEMs, Tier 1 suppliers, and studies published by trade associations, governmental agencies, manufacturing consultants, and earlier NRC reports. Appendix I
TABLE 9.1 Committee’s Estimates of Effectiveness (shown as a percentage) of Near-Term Technologies in Reducing Vehicle Fuel Consumption
|
NOTE: Some of the benefits (highlighted in green) are incremental to those obtained with preceding technologies shown in the technology pathways described in Chapter 9. |
contains results from some of these other studies, although, again, the reader should refer to the original source for the assumptions and study approaches used in these other studies. During the data gathering process, it became clear that the estimated incremental cost ranges were, in many cases, very large, depending on the boundary conditions identified by the organization offering the information. Generally, the committee notes that cost estimates are always more uncertain than the fuel consumption impact estimates, and the estimates presented here should be considered very uncertain until more detailed studies are completed. A boundary condition in the cost estimations is an assumption of long-term, high-volume production, whereby analysts attempt to normalize all incremental costs into a scenario where the capitalized development cost becomes a small portion of the final unit production cost. This is accomplished by assuming that production volumes are several hundred thousand units per year and remain in production for many years.
Although this assumption may be quite appropriate to normalize overall annual societal costs, it does not necessarily recognize the initial development-based costs and quality hurdles that may prevent a manufacturer from pursuing new product or technology areas. For example, such analyses would not consider factors that may inhibit or prevent the
introduction of diesel technology into passenger vehicles due to the significant investment and general lack of familiarity of North American automotive OEMs and suppliers in the production of small, light-duty diesels and the durability of necessary exhaust aftertreatment systems. This serves as a reminder that, while overall costs to the industry of new technologies is an important consideration, it is the individual manufacturers that bear the risk in adapting a technology to a specific vehicle and this risk may not be fully captured in a metric of overall industry costs.
The committee was briefed on the very detailed and transparent teardown cost assessment methodology being utilized by the EPA as part of the process for estimating the cost of fuel economy technologies. Cost estimation using the teardown approach is discussed in Chapter 3. The committee finds this approach an improvement over one where cost estimates are developed through expert knowledge and surveys of suppliers and OEMs, which have been the basis for most published studies and the majority of this report. Furthermore, the committee recommends that the use of teardown studies be expanded for future assessments when cost-effectiveness is an important evaluation criterion.
ASSESSING POTENTIAL TECHNOLOGY SEQUENCING PATHS
When manufacturers consider a strategy for implementing technologies that reduce fuel consumption, a normal business decision process must tradeoff many different parameters, including cost-effectiveness (fuel consumption reduction versus production cost), the ability to be integrated into product planning cycles, intended product use, reliability, impact on vehicle performance characteristics, and customer acceptance. To conduct the current assessment, the committee employed a method whereby cost-effectiveness (fuel consumption reduction divided by high-volume production incremental cost), vehicle intended use, base power train configuration, and technology state of readiness were considered in estimating potential technology paths for the eight vehicle classes described earlier.
As previously stated, an attempt to perform FSS on every vehicle model with all combinations of technologies is not practicable. Such a process would necessitate the analysis of (at least) tens of thousands of vehicle and power train technology combinations. It would require potentially confidential engine, transmission, accessory, and hybrid power train system maps; vehicle data such as friction as a function of vehicle speed; aerodynamic parameters; and many others parameters that are either proprietary or would require significant vehicle testing to generate for all of the combinations that are possible.
In some published studies, OEMs have supported such analyses for a limited number of vehicles that were chosen as sufficiently representative for discussion of the technology benefits and associated costs. For example, Sierra Research, in its report for the Alliance of Automobile Manufacturers (Auto Alliance), used the DOE-supported VEHSIM model to estimate fuel consumption reduction for various technologies using a composite of engine maps provided by manufacturers. Although the committee recommends a more practical approach to apply FSS for future regulatory actions, which is discussed later in this chapter, the exclusive use of FSS simulation for the assessment of all technologies considered under this study was not possible. The committee believes that sufficient experimental data can be gathered by the government to support future analysis and regulatory activities through consortia that include both regulatory agencies and automotive manufacturers and suppliers.
With this background, the committee evaluated potential technology paths that could be considered by a manufacturer, depending upon the manufacturer’s actual state of technology and production capability. Rather than creating decision trees from which an extremely large number of possible technology combinations could be created for each vehicle class, the committee estimated possible technology evolution paths for each class that develop from the average baseline vehicle. These pathways are summarized in Figures 9.1 to 9.5.
The baseline attributes were determined on a class-by-class basis using the 2007 EPA test list. If 51 percent of the vehicles in a given class had variable valve timing (VVT), then the baseline, class-representative vehicle was given VVT, and this technology would not be added in the path. Because the characteristic vehicle in each class represents the average attributes for that class, there will be some vehicles in that class that have more or less technology content. The below-average vehicles may require additional technologies and associated costs to address future standards while the above-average vehicles may not. Using the average attributes should provide a good overall representation of technology benefits relative to the baseline fleet within a class of vehicles. The technologies of the baseline vehicles are listed in the title bar of each technology path.
In the absence of a very large number of FSS analyses, but guided by a limited number of FSS runs performed for the committee by Ricardo, Inc. (2009), the committee evaluated possible sequences of technology implementation for different classes of vehicles. The development of the technology sequences shown in Figures 9.1 to 9.5 also was done with input from OEMs, Tier 1 suppliers, other published analyses, and the expert judgment of committee members. In developing the ranges of fuel consumption reduction, the committee recognized that the potential reduction for each incremental step is highly dependent on the extent to which system losses could have been reduced by previous technologies. These pathways attempt to include such factors as cost-effectiveness (percent fuel consumption reduction/incremental cost), logical sequencing based upon preexisting technology, technical limitations, and ease of implementation (requirements for major or minor manufacturing changes, including production line considerations). Subjective judgment by the committee also played a role in the pathway definition process.
Although the committee believes that some potential reduction is possible with each of the technologies considered, the extent to which a system energy loss can be reduced is highly dependent on all of the system interactive effects, the extent to which the baseline technology package has already reduced different categories of energy losses, and the production calibration parameters chosen by each manufacturer for the final release of each vehicle. Evaluating the energy losses associated with these technology pathways is discussed later in this chapter.
Review of Figures 9.1 through 9.5 shows that in certain cases (intermediate and large cars; unit-body standard trucks; two-seater convertibles, coupes, and high-performance sedans; unit-body performance trucks) the technology pathways are the same because of the similar base vehicle power train. However, the tradeoffs made as a result of varying performance metrics as these vehicle types go through their product evolution would result in different levels of fuel consumption improvement depending on the specific vehicle application.
Each range in potential fuel consumption reduction is an attempt by the committee to estimate the potential variation in energy loss reduction that might be possible when applying the technology to different power train and vehicle packages, taking into consideration known system features that will likely be optimized for different classes of vehicles with different intended uses. An example would be the bias inherent in production calibration of light-duty trucks or SUVs where reasonable towing capability is required.
A simple, multiplicative aggregation of the potential fuel consumption reduction is presented below each path in Figures 9.1 through 9.5 as a means to roughly estimate the total potential that might be possible. A probabilistic methodology based on the mean square rule was applied to estimate the confidence intervals for the aggregation of fuel consumption improvements and costs. Appendix J provides the mathematical explanation for this methodology. It assumes that the confidence intervals on each individual estimate of technology effectiveness or cost are the same. It also assumes that ranges in estimates are independent of each other and that errors are normally distributed. The approach then maintains a confidence interval for the aggregation of the low or high ends of the estimates that is equal to the confidence intervals estimated for the individual technologies. The committee assumes that the ranges for the individual costs and effectiveness represent a 90 percent confidence level. As such, the ranges were increased in technical areas where, in the opinion of the committee, more uncertainty existed with initial estimates.
It should also be noted that when the combination of fuel consumption improvement predictions and associated incremental costs is considered, the probability drops to 81 percent that any actual production technology introduction would fall within the ranges bounded by both the fuel consumption and cost ranges. This reduction is due to the (multiplicative) product of two 90 percent probabilities. Although prepared in response to the committee’s statement of task, these data are approximate in nature and as such should not be used as input to analyses where modeling accuracy is important. They are provided here as rough estimates that can be used in a qualitative comparative sense when comparing the relative cost-benefits of spark-ignition (SI)-related technologies that are potential candidates for FSS analyses. The committee’s estimates can also be used for a qualitative comparison of SI-related technologies to other candidates such as light-duty diesel or hybrid vehicles.
The results show that significant reductions in fuel consumption are possible with technologies that are already in production in U.S., European, or Asian markets. For example, for the intermediate car, large car, and unibody standard truck classes, the average reduction in fuel consumption for the SI path is 29 percent at a cost of approximately $2,200; the average reduction for the compression-ignition (CI) engine path is 38 percent at a cost of approximately $5,900; and the average reduction for the hybrids path is about 44 percent at an average cost of approximately $6,000. In general, diesel engine and hybrid vehicle technology options offer greater improvement potential compared to the SI pathway, but at a higher incremental cost. However, as evidenced by the increasingly wide range in estimated fuel consumption reduction and incremental cost, actual fuel consumption improvement can vary significantly depending on an individual manufacturer’s product strategy. Further, it may be that the needs to reduce vehicle fuel consumption as mandated by recent legislation will result in OEMs implementing these technologies in such a way that the benefits fall toward the high end of the range. It should be noted that among its provisions related to fuel economy, the Energy Independence and Security Act (EISA) of 2007 required periodic assessments by the NRC of automobile vehicle fuel economy technologies and their costs. Thus, follow-on NRC committees will be responsible for responding to the EISA mandates, including the periodic evaluation of costs and fuel consumption benefits of individual technologies and the combined impacts of multiple technologies.
When developing the effectiveness numbers, attempts were made by the committee to incrementally adjust the effectiveness numbers of certain technologies that would normally be preceded by another technology. This process allowed the committee to approximate the inclusion of the synergistic effects resulting from the combination of certain technologies that were deemed to usually be packaged together. In an attempt to evaluate the incremental effectiveness numbers for the SI pathway derived by the committee, comparisons were conducted using the FSS data from the Ricardo report prepared for the committee (Ricardo, Inc., 2009), the EPA-provided lumped parameter model, and various other SAE papers where combinations of technologies were assessed. A comparison to the Ricardo data is shown in Figure 9.6. Packages involving CVTs were excluded because the committee agrees with the EPA (EPA, 2008b) that
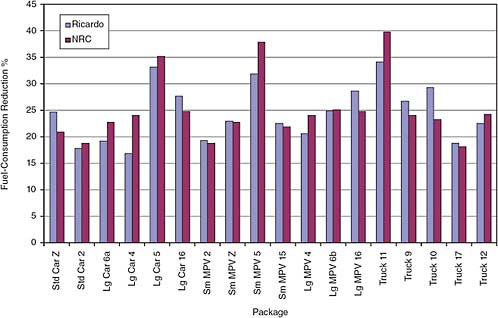
FIGURE 9.6 NRC estimates of effectiveness in reducing the fuel consumption of various light-duty vehicles compared with Ricardo, Inc. (2009) estimates based on data obtained with full system simulation.
Ricardo, Inc., used an abnormally small fuel consumption effectiveness value for this type of transmission.
As can be seen in Figure 9.6, the packages’ fuel consumption reduction results generally follow the relative comparisons between the packages analyzed by Ricardo, Inc. This is likely due to the engineering judgment of the members of the committee whose experience in power train engineering could be applied to the assessment. However, the absolute levels of potential improvement can vary significantly between the committee estimates and Ricardo analyses. Furthermore, a comparison of the step-by-step incremental estimates that would result from the application of single technologies was not conducted. Therefore, it is not possible to determine whether the demonstrated correlations were a result of accurate incremental estimates, or whether a combination of over- and underestimates resulted in a rough approximation, where such occurs.
In any case, the Ricardo, Inc., packages represent only a subset of the greater number of technology combinations that would result from proceeding down the entire pathway evaluated by the committee. This underscores the importance of using FSS to account for the larger number of technology synergies and ensure that system loss reduction is not overstated.
Due to the approximate nature of the estimates of incremental improvements in fuel consumption, the committee recognizes the potential to overestimate the potential reduction in energy losses, despite consideration given to the total system energy consumers. Therefore, as another check on the predicted aggregation of potential technologies, the committee contracted with EEA to apply its lumped parameter modeling approach to evaluate the committee’s estimates. Simplified lumped parameter models of vehicle energy use (e.g., Sovran and Bohn, 1981) provide a means of evaluating whether the fuel consumption benefits estimate for combinations of technologies by the multiplication methods result from forcing categories of energy losses (pumping and friction) to physically impossible levels. Appendix K provides a description of the EEA lumped parameter model as well as a description of the results in terms of the tractive energy requirements and the engine efficiency for the SI and diesel test cycles. These results indicate that the results from the multiplication method used here likely do not greatly overstate the benefits because this method does not explicitly take into account the theoretical limits of pumping loss reduction.
Figures 9.7 and 9.8 show the model results versus the committee estimates for eight cases (four for SI paths, and four for diesel paths). The model estimates for incremental improvements are relatively close to those of the committee, with the committee’s estimates generally exceeding those of the EEA model by a small amount. These comparisons are made between the average level of the committee’s estimates and the EEA data with no range presented. It should also be
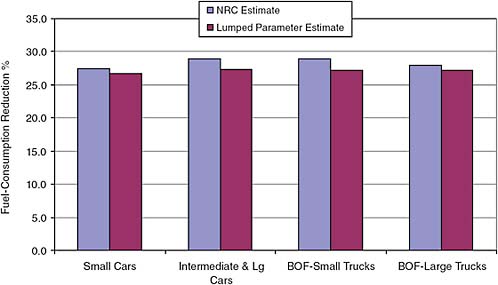
FIGURE 9.7 NRC estimates of effectiveness in reducing fuel consumption in spark-ignition engine pathways compared to EEA model outputs.
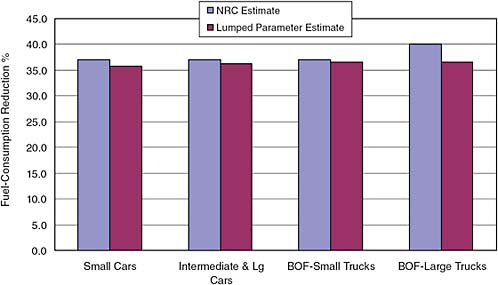
FIGURE 9.8 NRC estimates of effectiveness in reducing fuel consumption in diesel engine pathways compared to EEA model outputs.
noted that a baseline 4-speed automatic was used for both the committee’s and EEA estimates because these comparisons were conducted prior to the committee’s decision to utilize the average class transmission from the 2007 EPA test data in the technology paths.
One might conclude that the EEA modeling does, in fact, suggest that the committee’s estimates slightly overpredict the estimate. However, the same general method of comparison with known production vehicles and estimating the potential levels of energy loss reduction are employed in both the EEA lumped parameter approach and the expert opinion of the committee members. The EEA model does employ an algorithm to account for incremental reductions of energy losses, as predicted by an industry-derived set of equations (see Chapter 8). Therefore, it is not surprising that the estimations are relatively close.
However, the applications of the EEA’s or the committee’s estimation approach is done without a detailed understanding
of the actual levels of thermal efficiency of a subject vehicle’s engine, the influence of combustion chamber design on the fuel conversion efficiency, the actual levels of gas exchange or frictional losses, and all of the other parameters for which additional technologies can be applied to reduce fuel consumption. This is only possible through a combination of experimental and analytical analyses, which are necessary to predict the absolute level of fuel consumption.
Stated another way, in the opinion of the committee, neither the lumped parameter approaches evaluated by the committee nor the committee’s aggregated estimates define the actual level of energy efficiencies and/or losses of a randomly chosen vehicle with sufficient accuracy to allow accurate predictions of future technology introductions. Further more, this inaccuracy further degrades as an increasing number of technologies is employed. Therefore, the committee believes that a physics-based, FSS, in combination with experimentally generated data, is required for such predictions, especially if technology that is not currently in production is considered.
IMPROVEMENTS TO MODELING OF MULTIPLE FUEL ECONOMY TECHNOLOGIES
The application of FSS, in which the engine load, thermodynamic efficiency, operational losses of energy, and accessory loads are varied as a function of vehicle operational performance, offers the best opportunity to evaluate the effectiveness of incremental application of vehicle systems in reducing vehicle energy losses, thereby improving overall operational cycle efficiency and reducing fuel consumption. However, since different technologies may be attempting to reduce the same type of loss, for instance, pumping loss, it is necessary to evaluate the contribution of each incremental technology in reducing the different losses in each step along a potential product improvement path. Through the application of incremental technologies, one at a time, and then optimizing the predicted overall vehicle performance and fuel economy tradeoffs, it is possible to understand and quantify, at least for the vehicle model being evaluated, the interactive or synergistic effects that result. These may be positive or negative synergies, as outlined in the Ricardo report prepared for this committee (Ricardo, Inc., 2009) and discussed in Chapter 8 of this report. An example of these synergistic effects is presented in Table 9.3.
Table 9.3 shows that the total improvement in fuel consumption is gained from a combination of primary benefits attributed to a technology pair and a synergistic benefit (or detriment) as a result of the energy losses that are targeted for reduction. If one considers the engine and transmission combination, benefits in reduced pumping losses occur if a downsized, higher-specific-power engine is applied. Additional benefits can be gained from a more efficient transmission with reduced hydraulic losses or reduced friction. However, when these two are applied, there are additional benefits that arise from the ability to run the engine at a lower operating speed for a given power level, thereby increasing the brake mean effective pressure in the cylinders and further reducing the pumping losses. This contributes to the 2.17 percent improvement outlined in Table 9.3. However, it is important to note that the absolute level and relative levels of improvement outlined in Table 9.3 may vary significantly, depending on the application of the same technology sequence to another vehicle application.
As evidenced by the Ricardo, Inc., FSS analyses conducted for the committee, different vehicle types, with differing intended uses, demonstrate different optimization-of-performance characteristics. Therefore, when attempting to estimate the incremental benefits from the application of discrete technologies, the vehicle class, intended use, and associated performance metrics must be considered. Furthermore, the positive or negative synergistic effects of multiple vehicle energy-loss-reducing technologies will vary depending on the vehicle class and intended performance.
As outlined in Chapter 8 of this report, the current NHTSA method of applying technologies to vehicles applies them incrementally and individually to each vehicle in the NHTSA database, starting from the experimentally deter-
TABLE 9.3 Fuel Consumption Synergy Values for Inter-tree Technology Pairs—Results for Truck Package 11
mined value for combined fuel economy as demonstrated in the EPA vehicle exhaust emission certification process. One potential flaw in this methodology results from the process in which the lumped parameter model is used to predict the magnitude of energy loss reduction through the application of discrete technologies on an actual vehicle-by-vehicle basis. Without knowing the starting point in terms of how much the energy losses have been already been reduced, the ability to accurately project further reductions in such system energy losses, and therefore fuel consumption, can be highly erroneous.
Stated another way, it appears most logical to begin any predictive analysis with actual vehicle experimental data, if they are available, as is the case with all vehicles certified under the EPA Test Car List. However, without knowing how successful each manufacturer has been on a vehicle-by-vehicle basis in an ongoing attempt to reduce such energy losses, it is not possible, without detailed vehicle and power train experimental methods, to determine the extent to which any such loss can be further reduced, with a reasonable level of accuracy, on an actual vehicle model.
With an understanding of the potential errors that will result from the approximation method presented above, or other lumped parameter approaches where insufficient information is known about the level of energy loss reduction that has previously occurred on a particular vehicle, the committee proposes an alternative method whereby the potential for fuel consumption reduction and its associated costs can be assessed. This proposed method would determine a characteristic vehicle that would be defined as a reasonable average representative of a class of vehicles. This representative vehicle, whether real or theoretical, would undergo sufficient FSS, combined with experimentally determined and vehicle-class-specific system mapping, to allow a reasonable understanding of the contributory effects of the applied technologies in the reduction of energy losses. The reference to a “theoretical” vehicle suggests that if, during the regulatory process, the NHTSA and the EPA conclude that a vehicle may be characterized to represent a class that may not be in production, FSS models may still be created using physics-based vehicle models combined with experimentally generated engine maps.
In any full system simulation, the engine/power train/vehicle system is defined by input data that are generated by other physics-based analyses, engineering judgment, or experimentally or empirically derived tests. Experimentally measured data for engine maps can incorporate manufacturer-predetermined calibration parameters that have taken into consideration production operational factors such as knock-preventing spark timing or air/fuel ratio adjustments, which are used to protect from component temperature extremes. Physics-based engine maps, generated from engine combustion models, may also be used, but calibration-specific parameters must also be incorporated into such models to achieve best possible predictive results. The use of such models may be necessary when evaluating advanced technologies, such as variable compression ratio, that may not be readily available from production vehicles.
Vehicle-related data, such as data on frontal area, rolling resistance, and weight also are required input for modeling of vehicle performance and fuel economy. However, these data are more readily approximated based upon simplified physics-based calculations or are published in accordance with vehicle certification testing. Therefore, although physics-based engine simulation models are available, the use of experimental engine data, as described above, greatly improves the accuracy of the modeling.
Experimental methods used to understand the effects of different technologies in an attempt to reduce system energy losses have been developed under the United States Council for Automotive Research (USCAR) Benchmarking Consortium. Actual production vehicles are subjected to a battery of vehicle, engine, and transmission tests in sufficient detail to understand how each is applied and how they contribute to the overall performance and fuel consumption factors in light-duty vehicles. Combining such experimental methods with FSS modeling, wherein all simulation variables and subsystem maps would be transparent to all interested parties (both the regulatory agencies and automotive manufacturers, for example), would allow, in the opinion of the committee, the best opportunity to define a technical baseline against which potential improvements could be more accurately and openly analyzed than the current methods employed.
The advantages of such a method include the ability to explicitly account for all energy loss categories, the ability to directly estimate fuel consumption (as opposed to the percent change in fuel consumption), and the ability to represent new technologies and combinations of technologies. It also recognizes the increasingly common utilization of FSS models by regulatory agencies and other entities outside the automotive industry. Finally, the method proposes a procedure whereby engine and vehicle experimental data can be obtained without reliance on proprietary data, such as engine maps, that have posed a barrier to effective utilization of FSS models by non-OEMs in the past.
The steps in the recommended process are as follows:
-
Develop a set of baseline vehicle classes from which a characteristic vehicle can be chosen to represent each class. The vehicle may be either real or theoretical and will possess the average attributes of that class as determined by sales-weighted averages.
-
Identify technologies with a potential to reduce fuel consumption.
-
Determine the applicability of each technology to the various vehicle classes.
-
Estimate the technology’s preliminary impact on fuel consumption and cost.
-
Determine the optimum implementation sequence (technology pathway) based on cost-effectiveness and engineering considerations.
-
Document the cost-effectiveness and engineering judgment assumptions used in step 5 and make this information part of a widely accessible database.
-
Utilize modeling software (FSS) to progress through each technology pathway for each vehicle class to obtain the final incremental effects of adding each technology.
If such a process were adopted as part of a regulatory rulemaking procedure, it could be completed on 3-year cycles to allow regulatory agencies sufficient lead time to integrate the results into future proposed and enacted rules.
Based on the eight new vehicle classes proposed by the committee, an average vehicle, either real or theoretical, would be chosen that possessed the average attributes of the vehicles in that class. It would be of average weight, footprint, engine displacement, and other characteristics. The resulting vehicle would serve as the baseline for FSS analysis. This would also allow a very important starting point for the vehicle systems from which potential improvements could be evaluated. Using detailed benchmark data, defined levels of energy losses would be used as input into the simulation model. The data used to choose the vehicle consists of the following specifications available from the EPA test car list:
-
Footprint,
-
Weight, Engine (displacement, cylinder count, horsepower, torque),
-
Valve train configuration (OHV, SOHC, DOHC),
-
Valve event modulation technology (VVT, VVL),
-
Combustion technology (SI, CI, HCCI),
-
Fuel injection method and fuel type (SEQ, GDI, DFI, gasoline, diesel),
-
Aspiration method (natural, supercharged, turbocharged),
-
Number of occupants,
-
Power/vehicle weight ratio,
-
Transmission type and gear ratio spread,
-
Driveline (FWD, RWD, AWD), and
-
EPA vehicle class.
FINDINGS AND RECOMMENDATION
Finding 9.1: Many vehicle and power train technologies that reduce fuel consumption are currently in or entering production or are in advanced stages of development in European or Asian markets where high consumer prices for fuel have justified their commercialization. Depending on the intended vehicle use or current state of energy-loss minimization, the application of incremental technologies will produce varying levels of fuel consumption reduction.
Finding 9.2: Data made available to the committee from original equipment manufacturers and Tier 1 suppliers and found in various published studies suggest a very wide range in estimated incremental cost that makes assessments of cost-effectiveness very approximate. Generally, the committee notes that estimates of cost are always more uncertain than estimates of impact on fuel consumption, and the estimates presented here should be considered very uncertain until more detailed studies are completed. As noted in Chapter 3, estimates based on teardown cost analysis, currently being utilized by the EPA in its regulatory analysis for light-duty vehicle greenhouse gas emissions standards, should be expanded for developing cost impact analyses.
Finding 9.3: In response to the statement of task, the committee estimated possible technology evolution paths for each vehicle class that arise from the average baseline vehicle. A very simple, multiplicative aggregation of potential for reducing fuel consumption is presented as a means to roughly estimate the total potential that might be possible. The results from this analysis show that, for the intermediate car, large car, and unibody standard truck classes, the average reduction in fuel consumption for the SI path is about 29 percent at a cost of approximately $2,200; the average reduction for the CI path is about 38 percent at a cost of approximately $5,900; and the average reduction for the hybrids path is about 44 percent at a cost of $6,000. However, unless calibrated methods are used to accurately consider the synergistic effects of applying several technologies—effects that may reduce the same sources of power train and vehicle energy losses—these results are extremely approximate in nature and, in the committee’s opinion, should not be used as input to analyses for which modeling accuracy is important. In general, the technology tables that present incremental data for percent reduction in fuel consumption and estimated incremental cost cannot be used in their current form as input into lumped parameter-type models without methods to accurately consider the synergistic effects of applying several technologies and without significant expertise in vehicle technologies to fully understand integration issues.
Recommendation 9.1: As noted in Chapter 8, full system simulation (FSS), based on empirically derived power train and vehicle performance and fuel consumption data maps, offers what the committee believes is the best available method to fully account for system energy losses and synergies and to analyze potential reductions in fuel consumption as technologies are introduced into the market. FSS analyses conducted for the committee show that synergy effects between differing types of energy-loss-reducing technologies vary greatly from vehicle application to vehicle application.
The committee proposes a method whereby FSS analyses are used on class-characterizing vehicles, so that synergies and effectiveness in implementing multiple fuel economy technologies can be evaluated with what should be greater
accuracy. This proposed method would determine a characteristic vehicle that would be defined as a reasonable average representative of a class of vehicles. This representative vehicle, whether real or theoretical, would undergo sufficient FSS, combined with experimentally determined and vehicle-class-specific system mapping, to allow a reasonable understanding of the contributory effects of the technologies applied to reduce vehicle energy losses. Data developed under the United States Council for Automotive Research ( USCAR) Benchmarking Consortium should be considered as a source for such analysis and potentially expanded. Under the USCAR program, actual production vehicles are subjected to a battery of vehicle, engine, and transmission tests in sufficient detail to understand how each candidate technology is applied and how it contributes to the overall performance and fuel consumption of light-duty vehicles. Combining the results of such testing with FSS modeling, and thereby making all simulation variables and subsystem maps transparent to all interested parties, would allow the best opportunity to define a technical baseline against which potential improvements could be analyzed more accurately and openly than is the case with the current methods employed.
The steps in the recommended process are as follows:
-
Develop a set of baseline vehicle classes from which a characteristic vehicle can be chosen to represent each class. The vehicle may be either real or theoretical and will possess the average attributes of that class as determined by sales-weighted averages.
-
Identify technologies with a potential to reduce fuel consumption.
-
Determine the applicability of each technology to the various vehicle classes.
-
Estimate each technology’s preliminary impact on fuel consumption and cost.
-
Determine the optimum implementation sequence (technology pathway) based on cost-effectiveness and engineering considerations.
-
Document the cost-effectiveness and engineering judgment assumptions used in step 5 and make this information part of a widely accessible database.
-
Utilize modeling software (FSS) to progress through each technology pathway for each vehicle class to obtain the final incremental effects of adding each technology.
If such a process were adopted as part of a regulatory rulemaking procedure, it could be completed on 3-year cycles to allow regulatory agencies sufficient lead time to integrate the results into future proposed and enacted rules.
BIBLIOGRAPHY
EPA (U.S. Environmental Protection Agency). 2008a. Light-Duty Automotive Technology and Fuel Economy Trends: 1975 Through 2008. EPA420-R-08-015. September.
EPA. 2008b. EPA Staff Technical Report: Cost and Effectiveness Estimates of Technologies Used to Reduce Light-Duty Vehicle Carbon Dioxide Emissions. EPA420-R-08-008. Ann Arbor, Mich.
NHTSA (National Highway Traffic Safety Administration). 2009. Average fuel economy standards, passenger cars and light trucks, model-year 2011: final rule, RIN 2127 AK-29, Docket No. NHTSA 2009-0062. Washington, D.C., March 23.
NRC (National Research Council). 2002. Effectiveness and Impact of Corporate Average Fuel Economy (CAFE) Standards. National Academy Press, Washington, D.C.
Ricardo, Inc. 2008. A Study of Potential Effectiveness of Carbon Dioxide Reducing Vehicle Technologies. EPA420-R-08-004. Prepared for the U.S. Environmental Protection Agency, Contract No. EP-C-06-003, Work Assignment No. 1-14. Ann Arbor, Mich.
Ricardo, Inc. 2009. A Study of Interaction Effects Between Light Duty Vehicle Technologies. Prepared for the NRC Committee on Assessment of Technologies for Improving Light-Duty Vehicle Fuel Economy by Ricardo Inc., Van Buren, Mich., February 27.
Sovran, G., and M.S. Bohn. 1981. Formulae for the tractive energy requirements of vehicles driving the EPA schedule, SAE Paper 810184. February.