5
Sustaining the Core Research Program
A great strength of the astronomy and astrophysics research enterprise in the United States is that support comes from a variety of sources. These include federal and state governments as well as private universities, foundations, and individual donors. The federal program, with which this report is most concerned, is managed by NSF, NASA, and DOE, with additional, directed federal funding coming through the Smithsonian Institution1 and the Department of Defense.2 The research enterprise consists of two main components: (1) unique facilities, missions, and institutions, which are discussed in Chapter 6, and (2) the broadly distributed core activities discussed in this chapter—such as research grants to individuals and groups that support observation, theory, computation, data handling and dissemination, technology development, and laboratory astrophysics—that are the true foundations of the astrophysics enterprise.
Maintaining the correct balance between large and small projects, between projects and core activities, and also among the elements of the core program is a challenge that requires evaluation in the context of the current and future scientific landscape. In its review of the current health of these activities, the committee identifies modifications or augmentations that, because of an evolution of funding,
of science, or of infrastructure, are needed to maintain the balance that is essential for a vibrant astronomy and astrophysics research program.
INDIVIDUAL INVESTIGATOR PROGRAMS
Individual investigator programs are paramount in realizing the science potential of existing facilities, in pathfinding for future space missions and ground-based projects, and in training the current and future workforce. A healthy enterprise in astronomy and astrophysics requires a vigorous research grants program.
The fundamental products of astronomy (or any other science) are the discoveries resulting from research—new testable and tested ideas. The data analysis and dissemination and theoretical work performed by both individual scientists and science teams are ultimately responsible for the amazing results witnessed in astronomy in the past few decades. One of the most important secondary products is people who are trained in the broad discipline of science and who have skill in quantitative thinking and analysis, numerical computation, instrumentation and engineering, teaching, and project management.
Astronomers use complex and sophisticated tools and facilities such as satellites (e.g., the Hubble Space Telescope, the Chandra X-ray Observatory, the Spitzer Infrared Observatory, the Fermi Gamma-ray Space Telescope), ground-based facilities (e.g., NRAO plus ALMA, NOAO plus Gemini telescopes), and computing (high-performance networks, large-scale clusters, and software) to produce these products. However, supporting the development, construction, and operation of astrophysics facilities is far from all that is required to produce the superb results and discoveries that have driven the field and captured the public’s imagination. It is the combination of improved capabilities and facilities and the resources to use them effectively that has led to the remarkable scientific advances in astronomy. Scientific progress thus depends on and requires that individual investigators be supported, including being granted the resources that train students and postdoctoral fellows.
A significant challenge for the astrophysics program is how to maintain support for individual investigators pursuing a broad range of activities in a landscape where specific, large programs provide a fluctuating level of funding for associated analysis and theory. Realizing the scientific potential of existing facilities is of primary importance, but so is placing the broad range of results in appropriate context, providing young scientists with opportunities to develop their potential, and enabling the creative thinking that lays the foundations for the future.
As in most fields, the primary mechanisms for supporting research and training are competed grants programs. NASA funds both general mission-enabling grants programs and those supporting the specific science from operating satellites, such as the guest observer programs associated with Hubble, Chandra, Spitzer,
TABLE 5.1 NASA Astrophysics Division-Sponsored Proposal Opportunities for 2007
Program |
Proposals Received |
Proposals Selected |
Oversubscription Rate |
Astronomy and Physics R&A (APRA) |
146 |
52 |
2.8 to 1 |
Hubble Space Telescope |
821 |
189 |
4.3 |
Chandra X-ray Observatory |
663 |
177 |
3.7 |
Spitzer Space Telescope |
720 |
258 |
2.8 |
XMM-Newton |
330 |
102 |
3.2 |
INTEGRAL |
30 |
25 |
1.2 |
Kepler Participating Scientists |
37 |
8 |
4.6 |
Origins of Solar Systems (with Planetary Science Division) |
104 |
27 |
3.9 |
Astrophysics Theory and Fundamental Physics (ATP) |
181 |
37 |
4.9 |
GALEX Guest Investigator – Cycle 4 |
99 |
35 |
2.8 |
Astrophysics Data Analysis |
98 |
41 |
2.3 |
Fermi Guest Investigator – Cycle 1 |
167 |
42 |
4.0 |
Swift Guest Investigator – Cycle 4 |
144 |
49 |
2.9 |
Suzaku Guest Investigator – Cycle 3 |
120 |
50 |
2.4 |
TOTAL |
3,660 |
1,092 |
3.4 |
SOURCE: NASA Astrophysics Division. |
and Fermi. NSF supports a general astronomy and astrophysics grants program as well as more specialized programs such as the CAREER awards and the Astronomy and Astrophysics Postdoctoral Fellow program. DOE supports centrally administered grants programs, those administered through specific DOE laboratories, and awards for young investigators.
In recent times, funding for these essential programs has flattened or even declined3 at NASA and NSF, especially when considered relative to the growth of the field. Notably, DOE funding for astrophysics research increased from $34.4 million per year in 2004 to $45.2 million per year in 2008. Table 5.1 shows that in 2007 the oversubscription rate for various elements of NASA’s Astrophysics Division grants program varied but generally exceeded 2.5:1 and was as high as 4.9:1. Figure 5.1 shows that during the past decade, NSF’s proposal success rate for AST grants fell from a high of 37 percent in 2002 to a low of 23 percent in 2008, significantly lower than the more than 50 percent success rate of the early 1990s.
These data show that grant support for individual astronomers and astrophysicists has not grown as fast as the field over the past 15 years. At the current proposal success rate of less than 1 in 5 for NSF’s AAG program or some of the NASA R&A grants programs, even proposals rated “excellent” cannot be supported. There is a strong case for increasing the funding of these programs such that those
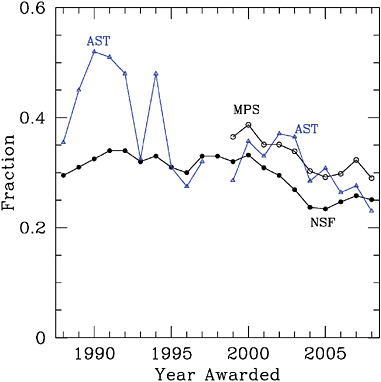
FIGURE 5.1 Proposal success rate for NSF as a whole, for NSF’s Directorate for Mathematics and Physical Sciences (MPS), and for NSF’s Astronomical Sciences (AST) Division, from 1988 to 2008.
proposals deemed worthy of funding by review panels, program managers, and advisory groups can be supported. Furthermore, the current situation is not a healthy position from which to carry out the more ambitious recommendations of Astro2010, given the needs for technical resources and personnel training. The goal is to achieve an appropriate balance between the optimal scientific exploitation of data obtained from the missions and facilities funded by NASA and NSF, and the mission/facility support itself.
In the committee’s judgment, it is absolutely necessary for the health of the whole astronomy and astrophysics enterprise to increase the support of individual investigators: those who write the papers, who train the students and other junior researchers, and who in the end produce the results to drive the field forward and ignite the public’s imagination. Reallocation of resources may have to come at the expense of support of existing missions/facilities and new projects.
In Chapter 7 the committee recommends upward adjustments in the funding levels of certain individual researcher and group grants programs at NSF
and NASA. Funding opportunities and the changing needs of larger programs sometimes require advice on significantly shorter intervals than the long-term advice provided here on program balance. In the past decade, for example, changing priorities at NASA overall, combined with the Columbia disaster, resulted in an abrupt funding redistribution that ultimately led to a significant imbalance in NASA’s astrophysics program, which in turn created issues with continuity of small-scale funding.4 For such unforeseen changes in circumstance, the AAAC can, as discussed in Chapter 3, provide tactical advice to DOE, NASA, and NSF on the support of individual and group grants funding, including the balance between grants programs, mission/facility operations, and the design and development of new missions/facilities.
THEORY
Emerging Trends in Theoretical Research
The role of theory in astrophysics has evolved in ways that reflect the increasing complexity of observations. Today, theoretical astrophysicists use analytical methods to devise speculative scenarios that account for new observations, they carry out detailed computational simulations of complex systems, and they develop new methods and frameworks for testing models against observational data. Together these methods propel progress, often in unforeseen ways. For example, the discussion of gravitational microlensing in the 1980s led to new observational constraints on the nature of dark matter in the 1990s and now provides a powerful pathway to the discovery of exoplanets. Similarly, recent observations of the cosmic microwave background have provided precision measurements of the age and content of the universe, but only because the theoretical framework had been developed over the preceding several decades, starting with new, bold theories about the exponential expansion rate of universe in its first few moments. Moreover, theory informed the design of experiments and enabled measurements to be extracted. The result is a spectacularly successful “standard model” of the universe, which experiments recommended in this report will test even more stringently.
Several important trends are increasing the scope of theoretical activity and enhancing the roles of theorists:
-
The boundary between astrophysics theory and high-energy physics theory has become increasingly blurred as astrophysical observations play a growing role in particle physics phenomenology. Much of the information we
-
have about physics beyond the standard model of particle physics comes from astrophysics; particle and astrophysical theorists are collaborating to push back the frontiers of fundamental physics. As an example, particle physics theory provides the prime candidates for potential dark matter particles (weakly interacting massive particles and axions) with properties that are constrained by both high-energy physics and cosmology.
-
Large numerical simulations are increasingly central to progress in astrophysics. Rapid advances in computational capabilities enable the large-scale computations needed to understand the complex phenomena being uncovered by current telescopes. They will be essential for predicting and understanding gravitational wave signals, and will enable three-dimensional simulations of supernova explosions and of the formation of the first stars in the universe, for example (Figure 5.2).
-
As the cost and scope of new observational facilities have grown, theorists have played an increasing role in their conceptual development, in making the science case for funding them, and in analyzing the results. Examples include new gravitational wave observatories and modeling of the distribution of stable planetary systems to inform future searches.
-
Theorists provide visualizations of complex physical phenomena that facilitate deeper understanding, that are appealing to the general public, and that attract talented young people to the field.
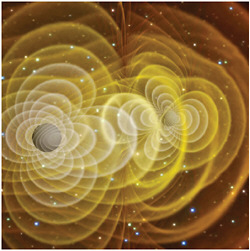
FIGURE 5.2 Simulated image of gravitational radiation from two merging black holes using NASA’s Columbia supercomputer. A movie of this simulation can be found at http://www.nas.nasa.gov/News/Archive/2006/08-09-06.html. SOURCE: Chris Henze, NASA.
Theoretical Challenges for the Next Decade
A healthy theory program advances science on a broad front and supports a range of targeted activities as well as the exploration of radical new ideas that inspire missions for the distant future. For this decade, the Astro2010 Science Frontiers Panels (SFPs), and Chapter 2 of this report, have identified questions on the forefront of astrophysics, several of which present specific and significant theoretical challenges.
The Panel on Cosmology and Fundamental Physics raises the questions, How did the universe begin? Why is the universe accelerating? What is dark matter? What are the properties of neutrinos? New observations are central to providing the necessary constraints to address these questions, but theories are ultimately being put to the test.
One of the upcoming challenges associated with the Panel on Stars and Stellar Evolution is the three-dimensional simulation of the magnetic field observed in the solar corona using the Solar Dynamics Observer and other solar observatories. The quality of the data now being garnered presents a strong challenge to simulators. Success in explaining the behavior of the solar magnetic field will pay large dividends as astrophysicists attempt to understand how fields behave in other environments.
The prime research topics identified by the Panel on the Galactic Neighborhood involve study of the circumgalactic and interstellar media seen as complex ecosystems. For both topics, sophisticated simulations go hand in hand with the observational program. A third question concerns the fossil record of star formation as a means of understanding the first stars and the subsequent assembly of galaxies like our own. Here the theories of stellar evolution and stellar dynamics are crucial. The fourth research area, the use of the galaxy to study dark matter (Figure 5.3), has already attracted the attention of a large community of theoretical physicists.
Central questions raised by the Panel on Galaxies Across Cosmic Time are the following: How do cosmic structures form and evolve? How do baryons cycle in and out of galaxies, and what do they do while they are there? How do black holes grow, radiate, and influence their surroundings? (Figure 5.4), and What were the first objects to light up the universe, and when did they do it? As discussed below, analytic theory and computational modeling will take a central role in addressing these questions.
Supernovae are the most energetic explosions in the universe since the big bang and the furnaces in which most of the chemical elements from which we are made are forged. Visible from halfway across the universe, these spectacular cosmic events provide some of the strongest evidence that the universe is accelerating. As pointed out by the Panel on Stars and Stellar Evolution, understanding why and how stars
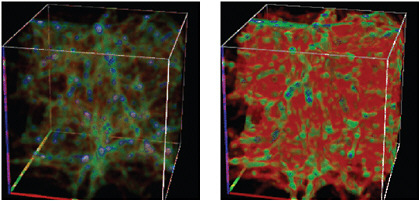
FIGURE 5.3 Two views of dark matter distribution. SOURCE: Edmund Bertschinger, MIT.
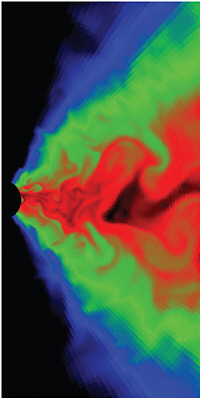
FIGURE 5.4 False-color simulated image of the density of matter accreting from a spinning gas disk onto a black hole. The image shows a cross-sectional cut through one side of the disk, with the black hole represented as a black semicircle on the left side. A striking feature is the large, chaotic fluctuations in the density caused by convective motions in the disk. SOURCE: J. Stone, Princeton University.
explode as supernovae requires three-dimensional computations similar to those used to study fuel efficiency in cars and the design of new rockets but in far more exotic and challenging conditions (Figure 5.5).
Finally, understanding planet formation, an issue central to the Panel on Planetary Systems and Star Formation, is one of the most challenging tasks in astrophysics. A comprehensive theory of planet formation requires following the growth of dust grains in the protoplanetary disk into small rocky bodies, the growth of these bodies into planets, and the subsequent development of oceans and atmospheres—a study spanning some 42 orders of magnitude in mass and a vast array of processes ranging from the sticking properties of dust grains, through the dynamics of bodies in shearing gas flows, to gravitational stability of planetary orbits on billion-year timescales.
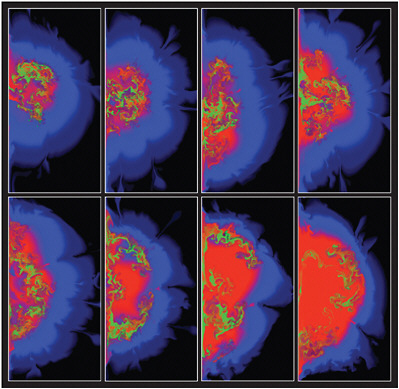
FIGURE 5.5 Theoretically predicted chemical structure 100 seconds after the explosion of a massive carbon/oxygen white dwarf. The blue regions show intermediate-mass elements (e.g., silicon, sulphur, calcium), green indicates radioactively stable iron-group elements, and red indicates 56Ni, the isotope that powers the supernovae for the next few months. SOURCE: Reprinted by permission from Macmillan Publishers Ltd: Nature, D. Kasen, F.K. Röpke, and S.E. Woosley, The diversity of type Ia supernovae from broken symmetries, Nature 460:869-872, 2009. Copyright 2009.
Individual Investigator Programs in Theory and Computation
Astrophysical theory is intellectually vibrant, and productivity is high: about 45 percent of published papers are on theory, and about one-third of publishing astronomers are pursuing theory (see Figure 4.9). Based on the recent record, there is a compelling case that investments in theory by the agencies will be amply repaid in the form of new mission and experiment concepts and enhanced scientific return from operating facilities. Astrophysical theory draws some of the world’s best intellectual talent into the U.S. scientific enterprise. Because some of the most important theory contributions in the next decade will come from broadly based theory not specifically tied to large activities, the role of general individual investigator programs will continue to be as important as ever. These programs form the traditional base of theoretical astrophysics in which Ph.D. students are trained, new ideas arise, and future observational or experimental efforts are seeded.
Astrophysics and cosmology theory is supported through a number of programs at the federal agencies. At NSF, general astrophysics theory is funded through the AST Astronomy and Astrophysics Research Grants (AAG) program,5 as well as through the NSF Division of Physics (NSF-PHY) via its Frontier Centers and individual investigator grants in cosmology and particle physics theory. At NASA, the Astrophysics Theory Program (ATP) supports most general theory efforts. In addition, the Hubble, Spitzer, Chandra, and Fermi Observatories accept theoretical investigations as part of their guest investigator programs. Other critical support comes from NASA Prize Postdoctoral Fellowship programs (Einstein, Hubble, Sagan). The DOE’s Office of High Energy Physics also supports theoretical and computational astrophysics efforts. Table 5.2 summarizes current funding levels.
As is the case with the grants programs in general, proposal success rates in theory have declined over the past decade. The recent success rate in NASA’s ATP is only 15 to 20 percent, significantly lower than funding rates for theory within its Planetary Exploration program, for example. Given the central importance of theory to the enterprise, and the crucial role played by individual investigator grants, the committee recommends in Chapter 7 that the grants programs at both NSF and NASA be augmented.
The Rapid Rise of Astrophysical Computing
The dramatic impact of computation on astronomy and astrophysics is manifested in many ways. Modern numerical codes are now being used to simulate and
TABLE 5.2 Support for Astrophysics and Cosmology Theory
Program |
Budget (million $) |
NSF-AST Astronomy and Astrophysics Research Grants |
15.1 |
NSF other (AAPF, CAREER, Cyberinfrastructure, and others) |
5.3 |
NSF-PHY Astrophysics and Cosmology Theory |
1.2 |
NSF-PHY Physics Frontier Centers |
(several) |
NASA Astrophysics Division Astrophysics Theory Program |
12.4 |
NASA Astrophysics Division Great Observatories Guest Observer Programs |
2.2 |
DOE Scientific Discovery through Advanced Computing |
0.7 |
DOE High Energy Physics Theory |
10 |
DOE Nuclear Physics Theory |
3 |
understand the formation of structure in the universe, the explosion of massive stars, the evolution of our solar system over billions or trillions of years, and how a complex experiment works. They are also essential to processing astronomical images whose sizes now exceed 1 billion bytes (a gigabyte) into data that are usable by the astronomical community. The largest codes may have in excess of a million lines and run on supercomputers that have more than 100,000 cores, generating data sets that occupy 1 trillion bytes (a terabyte) of storage. These codes are now an indispensible part of the astronomical enterprise. However, they often require teams—scientists, computer professionals, applied mathematicians, and algorithm specialists—to create, maintain, and constantly develop them.
NSF, NASA, and DOE have made substantial investments in high-performance computing (HPC) over the past decade, making available close to a petaflop of sustained computing power to the astrophysics community. Such facilities enable cutting-edge theoretical calculations and analyses that push the astrophysics frontier. Future progress in supercomputer power will come from further parallelization, with the largest systems evolving from 104 to 105 processor cores today to perhaps 108 to 109 cores by the end of the decade.6
These capabilities will enable qualitatively new physical modeling.7 Exploiting the new computer systems will require new software codes and sustained support for focused research groups. At the same time, strategic balance should be maintained between investment in HPC and hardware resources for individual investi-
gators and university-department-scale clusters, which are critical for exploratory and smaller-scale projects and for the training of students.
Research Networks in Theoretical and Computational Astrophysics
A large number of the theoretical challenges posed by the Science Frontiers Panels are of a scale and complexity that require sustained, multi-institutional collaborations of theorists, computational astrophysicists, observers, and experimenters. There is currently no mechanism to support these coordinated efforts at the required level in the United States; however, successful models for such coordinated efforts exist in Europe.8 Opportunities used to exist for such medium-scale group efforts in the NASA ATP, but more recently ATP has been focused on individuals and small single-institution groups. Appropriately focused and led research collaborations and networks are “efforts of scale” that can make long-term investments in personnel, computing, and scientific networking uniquely effective in tackling some of the most difficult problems in modern astrophysics.
RECOMMENDATION: A new program of Research Networks in Theoretical and Computational Astrophysics as discussed in Chapter 7 should be funded by DOE, NASA, and NSF. The program would support research in six to eight focus areas that cover major theoretical questions raised by the survey Science Frontiers Panels.
The networks would be devoted to a specific problem or topic that is believed to be ripe for a breakthrough within 5 years. Selection criteria would include the degree of cross-institutional synergy in the network and its planned role in training and mentoring the next generation of researchers. Funding would normally be for a 5-year period, and the entire program would be subject to a senior review after 5 years. These networks fulfill a role different from that of NASA’s ATP and NSF’s AAG program and should not be funded at their expense. For NSF’s AAG program the success rate for theory proposals is roughly 37 percent.
DATA AND SOFTWARE
The scientific richness and extent of astronomical data sets are increasing rapidly. The sizes of modern databases have grown over the past decade into
the petabyte (1 million gigabytes) range, with a current growth rate of roughly 0.2 petabytes per year. Challenges for data archiving will increase dramatically in the future. The committee’s top-ranked ground project, the Large Synoptic Survey Telescope (LSST), expects its archive to grow by a petabyte per month. A complete SKA operated in the manner of the VLA or ALMA would operate in the thousand-petaflop, or exaflop (1,000 petaflops), scale compared with the petaflop of sustained power consumed by current astronomical computing. Proper maintenance and accessibility of these archives are essential to optimizing scientific return, especially for LSST studies of transient and time-variable phenomena for which rapid availability of validated data will be critical.
As discussed in Chapter 3, the AAAC can play a key role in providing tactical advice to DOE, NASA, and NSF on the support of data archiving and dissemination, and on data analysis software funding across the three agencies relative to the agencies’ programmatic needs as identified by Astro2010. In particular, the optimal infrastructure for the curation of archival space- and ground-based data from federally supported missions and facilities will need periodic attention.
Data Archives
Data archives are central to astronomy today, and their importance continues to grow. The science impact of these archives is large and increasing rapidly. Papers based on archival data from the Hubble Space Telescope now outnumber those based on new observations in any year and include some of the highest-impact science from the HST, as shown in Figures 5.6 and 5.7. Data from the 2 Micron All Sky Survey (2MASS) and the Sloan Digital Sky Survey (SDSS), which were both designed as archival projects, led to more than 1,400 and 2,650 refereed papers in the past decade, respectively, with the scientific output continuing to increase well after the completion of these surveys.
Publicly accessible data archives can multiply the scientific impact of a facility or mission—for a fraction of the capital and operating costs of those facilities or missions. The data explosion and the long-term need for the ability to cross-correlate enormous data sets require archival data preservation beyond the life of projects and the development of new analysis and data-mining tools. The establishment over the past decade of the National Virtual Observatory, a top recommendation of the 2001 decadal survey and part of an International Virtual Observatory initiative, has produced widely accepted standards for data formatting, curation, and the infrastructure of a common user interface. These standards have the potential to substantially enhance the collective value of archival data sets.
NASA has regarded data handling and archiving as an integral part of space missions. It has established a network of data centers to host data from NASA mis-
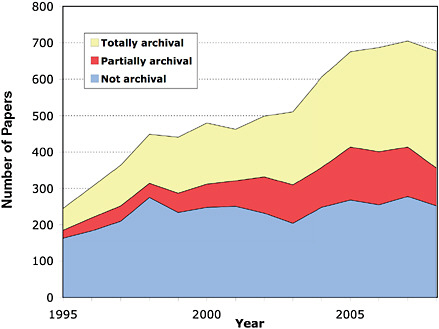
FIGURE 5.6 Number of published papers using Hubble Space Telescope data, 1995 to 2008. The publications are divided into non-archival papers written by the original investigators (blue), fully archival publications not involving any of the original investigators (yellow), and papers, some archival and some not, that include data from multiple proposals (red). The number of archival papers has exceeded the number of original-investigator-written papers since 2006. SOURCE: Courtesy of Richard L. White, Space Telescope Science Institute.
sions, and a National Research Council report9 lauded their efficiency. This support now provides the major return on the considerable investments the agency has made in the Great Observatories and other facilities over the past 20 years. The 2007 report also found that the consolidation of archives at a small number of facilities is efficient, cost-effective, and serves the scientific community well.
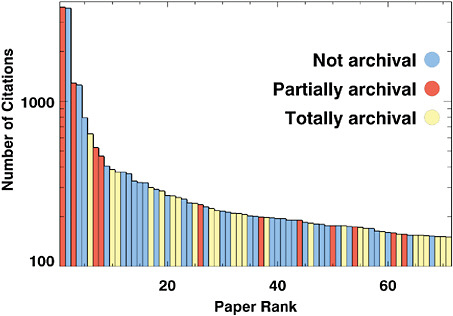
FIGURE 5.7 Highly cited Hubble Space Telescope publications, 1997 to 2000. All 71 papers with more than 150 citations as of March 2009 are included in the sample. Note that the y-axis is logarithmic. Totally archival papers make up 37 percent of the sample. SOURCE: Courtesy of Richard L. White, Space Telescope Science Institute.
On the ground, major surveys, such as 2MASS and SDSS in the optical-infrared, the FIRST and NVSS radio continuum surveys, and the ALFA H I (neutral hydrogen) and pulsar surveys, had archives built into their programs. Radio interferometers generally have standardized observing protocols that make it straightforward to archive the data taken. Radio telescopes operating as single apertures and ground-based optical-infrared telescope user facilities have, in large measure with the exception of the Gemini Observatory, not yet developed an archiving culture, although most major facilities do “save the bits” of raw data. Partly, this lack of an archiving culture is due to the difficulty and consequent cost of archiving data taken under a multiplicity of observing modes. Although cost-benefit considerations must be weighed, given the current trends in the demand for archived data and the growth of private-public telescope partnerships, the committee finds it highly desirable for ground-based telescopes to make data archiving an integral part of their operations in the future.
Archives of either raw or processed data should contain complete calibration information, a history of data processing and/or the software to process the data, and access to tools to analyze the data. The format should be Virtual
Observatory-compliant when this is cost-effective. Astronomical librarians and data archivists should be involved in metadata design and curation. While NASA has an established policy whereby archiving and public access are required and costs are included in mission budgets, NSF has no consistent policy. Financial support made available under agency peer-review processes would enable existing observatories to implement such archives. DOE has a culture that supports modern data handling and prudent selection of which data to archive. It does not have a culture of public access but has adapted well to such practice in its collaborations with astrophysicists.
RECOMMENDATION: Proposals for new major ground-based facilities and instruments with significant federal funding should be required as a matter of agency policy to include a plan and if necessary a budget for ensuring appropriate data acquisition, processing, archiving, and public access after a suitable proprietary period.
To be practical and cost-effective, this requirement should be limited to data that are reasonably likely to be of interest to other users, for example large survey programs and other significant principal-investigator-led efforts in optical-infrared and radio astronomy. The committee further concluded that public funds could support public archiving of data from facilities that are fully funded from private sources, should such support be proposed and highly reviewed. Proposals to NSF’s Astronomy and Astrophysics Research Grants program or to the ATI program could include support for the development of software tools related to data reduction and analysis, and archiving.
Because data archives are so central to modern astronomy, it is a matter of concern that no model exists for long-term preservation (curation) of ground-based data once observing projects or facilities are no longer funded.10 To realize the full benefit of ground-based data, especially from surveys, it is therefore necessary for NSF to adopt the model of NASA’s long-lived data archive centers (like IPAC, MAST, HEASARC) and also of the Canadian Astronomy Data Center for long-term curation of data, with capabilities similar to those available through existing successful archives.
A coordinated interagency effort will be particularly important with the advent of the petabyte-scale surveys anticipated in the future. An example of an opportunity for possible synergy in combining ground-based and space data is solar physics. The rapidly growing database from existing facilities including the Solar Dynamics Observatory presents an opportunity to combine complementary data sets in order to obtain a balanced view of the dynamic Sun. This investment
is likely to pay a large dividend when the Advanced Technology Solar Telescope comes on line in 2017. The National Science Board report Long-Lived Digital Data Collections: Enabling Research and Education in the 21st Century11 and the National Academies report Ensuring the Integrity, Accessibility, and Stewardship of Research Data in the Digital Age12 both recognized the growing importance of long-term curation, and the NSB report recommended that NSF develop a global strategy to address it. The NSF Office of Cyberinfrastructure’s DataNet program (Sustainable Digital Data Preservation and Access Network Partners), which is partnering with research institutions to develop data preservation facilities of general utility to the research community and which includes participation by astronomers, is an important first step in the process.
RECOMMENDATION: NSF, NASA, and DOE should plan for effective long-term curation of, and access to, large astronomical data sets after completion of the missions or projects that produced these data, given the likely future scientific benefit of the data. NASA currently supports widely used curated data archives, and similar data curation models could be adopted by NSF and DOE.
The committee estimated the cost of achieving these data-archiving goals on the basis of an informal survey of existing archives. Data gathered by the survey’s Infrastructure Study Groups indicate that adding the data from a new survey similar to SDSS to the portfolio of an existing archive center would involve startup costs of about $0.4 million (approximately $0.15 million for personnel and $0.25 million for hardware) and an annual operating budget of about $0.2 million to $0.3 million ($0.15 million for personnel and the remainder for maintenance and upgrades). Starting a new archive from scratch would be significantly more expensive, and so it would be particularly cost-effective for NSF and DOE to coordinate with NASA to use existing archive and data distribution centers. An added scientific advantage would be having a core of resident astronomers, computer scientists, and technical support staff.13 Supporting additional archiving and long-term curation for a few existing observatories and instruments would cost roughly $15 million per decade. Numerical codes could also be curated.
Data Reduction and Analysis Software
Major instruments with wide public use on federally supported telescopes and facilities would benefit greatly from pipelines that deliver calibrated data and data products for storage in a public archive. General-purpose community analysis software packages like the Image Reduction and Analysis Facility and Astronomical Image Processing System currently used by optical and radio astronomers are more than 30 years old and will not be able to handle future needs. In addition, specialized programs for automated data-handling tasks across many areas of astronomy and astrophysics must be written. New packages capable of handling large data sets are urgently needed. These are likely to be created and employed within a common-use environment. Flexibility, openness, and platform independence, modularity, and public dissemination are essential to this effort. Focused investment in a series of small-scale initiatives for common tool development and the collection of those tools in a public portal may be the most cost-effective approach, although there are undoubtedly synergies with the pipeline development needed for the large-scale projects. Further, central data archives, in which the survey committee recommendeds all future major projects participate, could maintain current software versions and provide community access and documentation for general data reduction tools.
MEDIUM-SCALE ACTIVITIES
A major recommendation of this report, directed to both the ground and the space programs, is that more support should be directed toward activities of intermediate scale. For the space program, both NASA’s Explorer program and its Suborbital program are recommended in Chapter 7 for funding increments. For the ground-based program, the committee endorses the recommendations of several previous advisory groups to NSF that there be mid-scale funding opportunities, and it recommends in Chapter 7 a new competed program. Medium-scale programs and experiments offer excellent return for the investment and are essential to the capability for responding flexibly to new scientific opportunities, for demonstrating novel techniques and instruments, and for training the experimental scientists, engineers, and managers who will execute the major missions and observatories of tomorrow.
Technical Workforce Development
The designers of missions and telescopes, and those who implement instruments and understand their performance, are central to the astrophysics enterprise. One of the most important elements for reducing technical and schedule risk for any space mission or major ground-based project is a highly experienced team.
The current distribution of activities and grants funding poses particular challenges for maintaining a workforce skilled in instrument and project development. Although properly funded programs for space and ground facilities often provide significant support for the training of new data analysts, the opportunities for training students in instrumentation have declined precipitously over the past 20 years. Training for the next generation of instrumentalists is most efficient when there is a steady-state hierarchy of project sizes, so that people can progress from relatively smaller, simpler, and faster projects to responsibilities in larger and more complex activities.
Despite existing NASA and NSF funding mechanisms that can support technology training, the data gathered by the survey’s Infrastructure Study Groups show that fewer than 5 percent of students recently receiving Ph.D.s from astronomy departments classify themselves as belonging to “instrumentation and methods” subfields. If there are to be enough young instrumentalists to spearhead the ambitious new instruments and facilities of the coming decade, more must be done within graduate astronomy programs to educate and train them. The growth of astrophysics research within physics departments can help in this regard.
Some of the input Astro2010 received in white papers submitted by the community discussed the need for increased emphasis on instrumentation within U.S. astronomy and astrophysics Ph.D. programs. It is important that universities recognize the value of skilled instrumentalists, and that they continue to provide opportunities for early-career training. Further, the scientific community must value the intellectual contributions of instrumentalists as an integral part of the astrophysics endeavor.
NASA Explorer and Suborbital Programs
The Explorer program, which develops small and mid-size missions on timescales of a few years, is a crown jewel of NASA space science. Its tremendous scientific productivity results from the selection and implementation of focused scientific investigations enabling rapid response to new discoveries. For example, among the astrophysics Explorers, the WMAP Medium-scale Explorer (MIDEX) mission capitalized on the discovery made by a previous Explorer, COBE, that the microwave background has measurable fluctuations. Launched just 5 years after the COBE results were published, WMAP demonstrated that precise information about the early universe is imprinted on these minute fluctuations, leading to greater understanding of the age, geometry, and content of the universe; the papers based on WMAP data are the among the most highly cited in all of astrophysics. The Swift gamma-ray burst (GRB) MIDEX was launched just 7 years after the discovery that GRBs—bright, few-seconds-long, high-energy pulses from the cosmos—are accompanied by long-lived afterglows extending down to radio wavelengths. These
afterglows enable us to associate GRBs with the birth cries of black holes from across the universe. Swift’s success was rewarded when it was identified as the highest-ranked mission in the 2007 senior review—a process that compared its scientific returns to those of major flagship missions. The GALEX Small Explorer (SMEX) ultraviolet mission is changing our understanding of how stars formed and how galaxies evolved over the past 10 billion years of cosmic history, and it is now supporting an active guest investigator program. The recently launched WISE MIDEX is successfully conducting an all-sky mid-infrared survey with announced discoveries ranging from asteroids and comets to active galactic nuclei.
In addition to these stand-alone experiments, the Explorer program supports Missions of Opportunity (MoOs)—contributions of instruments or investigations to space programs led by other countries. MoOs provide highly leveraged mechanisms to broaden the astrophysics program, deploy new technologies, and return significant science for relatively modest investments. In addition, suborbital science experiments can be proposed as MoOs.14
NASA’s suborbital (balloon and rocket) programs enable scientific experiments with equipment ranging from particle detectors to X-ray, gamma-ray, infrared, and microwave instruments. They support substantive scientific investigations in areas such as CMB and particle astrophysics, fulfill essential needs in technology development, and provide invaluable hands-on training. Notably, key positions in mission development across NASA are occupied by people who received their training through participation in suborbital missions. This group is aging, however, and replacements are few (Figure 5.8). Although NASA maintains a technical workforce within its stably funded centers, the groups in universities that train students to renew NASA’s talent are subject to large variations in funding associated with individual missions. As a result of diminishing astrophysics budgets combined with full-cost accounting, the NASA centers are also now competing for the smaller training projects that used to be located across multiple universities.15 In light of identified exceptional science opportunities now and ahead, there is a clear need to renew the talent pool of experienced instrumentalists, and the committee makes a number of related recommendations in this report. In Chapter 7, the committee recommends increased support for the Suborbital program, as well as an augmentation to the Explorer program that will double the number of opportunities for stand-alone missions and vastly increase the number of Missions of Opportunity. Historically, Explorer missions and suborbital experiments have
14 |
National Research Council, Revitalizing NASA’s Suborbital Program: Advancing Science, Driving Innovation, and Developing a Workforce, The National Academies Press, Washington, D.C., 2010. Available at http://www.nap.edu/catalog.php?record_id=12862. Accessed May 2010. |
15 |
See the 2010 National Research Council report Capabilities for the Future: An Assessment of NASA Laboratories for Basic Research, The National Academies Press, Washington, D.C. Available at http://www.nap.edu/catalog.php?record_id=12903. |
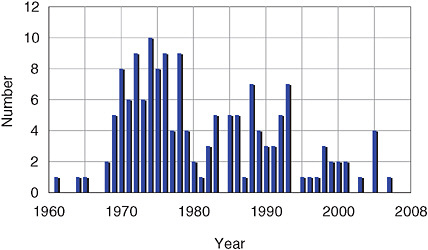
FIGURE 5.8 Number of Ph.D.s awarded per year to individuals trained in sounding rocket programs, 1961 to 2008. SOURCE: NASA Astrophysics Sounding Rocket Assessment Team.
included significant instrumentation efforts centered at universities, and their development timescales are suitably short, compared with those of flagship missions, so as to match graduate student and postdoctoral fellow terms of study.
NSF Mid-Scale Innovation Program
The cost of major new instruments and facilities has increased significantly over the past 20 years, to some extent reflecting increasing scale and complexity. For example, instruments for 8- to 10-meter-class ground-based telescopes are necessarily larger and hence more costly than those for smaller telescopes. Similarly, large focal plane arrays for radio telescopes are more costly than the previous generation of non-multiplexed receivers. In addition, providing a significant enhancement over current capabilities requires applying new and more expensive technology such as adaptive optics systems in the optical or complex correlator systems in the radio. Finally, as data output increases to many terabytes, data management and software complexity add to instrument and facility costs.
Within NSF, grants programs such as Astronomy and Astrophysics Research Grants, Advanced Technologies and Instrumentation, and Major Research Instrumentation provide funding for training young people in instrumentation and telescope design, data analysis, and data interpretation, and such training has been funded relatively steadily over the past decade (Table 5.3). NSF grants typically involve a graduate student or postdoctoral fellow who is learning about instrumen-
TABLE 5.3 Detailed NSF-AST Non-facilities Budgets (in millions of FY2010 dollars)
|
1999 |
2000 |
2001 |
2002 |
2003 |
2004 |
2005 |
2006 |
2007 |
2008 |
R&E |
34.21 |
42.19 |
44.77 |
57.68 |
62.04 |
61.02 |
60.60 |
61.92 |
63.91 |
64.53 |
AAG |
24.09 |
26.11 |
27.73 |
35.36 |
38.11 |
35.23 |
35.55 |
41.80 |
43.61 |
43.03 |
ESP |
6.19 |
6.05 |
6.20 |
6.55 |
6.25 |
6.15 |
5.81 |
5.94 |
5.70 |
6.87 |
AAPF |
0.00 |
0.00 |
0.73 |
1.44 |
1.69 |
1.78 |
1.79 |
1.63 |
1.58 |
1.41 |
ESM |
0.13 |
0.26 |
0.20 |
0.13 |
0.21 |
0.30 |
0.22 |
0.22 |
0.21 |
0.19 |
STC |
0.88 |
8.21 |
2.10 |
4.84 |
4.71 |
4.51 |
4.44 |
4.30 |
4.21 |
3.39 |
Projects |
0.00 |
0.00 |
0.00 |
0.89 |
0.47 |
1.32 |
4.01 |
1.30 |
1.49 |
2.59 |
Initiatives |
2.11 |
1.25 |
7.08 |
7.69 |
9.92 |
11.13 |
7.97 |
5.68 |
5.79 |
5.31 |
Panels/IPAs |
0.81 |
0.32 |
0.72 |
0.77 |
0.68 |
0.60 |
0.80 |
1.05 |
1.32 |
1.74 |
Inst/Tech |
8.92 |
9.36 |
10.23 |
16.29 |
22.36 |
22.42 |
23.79 |
21.63 |
31.57 |
30.01 |
ATIa |
7.99 |
8.22 |
8.01 |
9.75 |
12.18 |
10.79 |
10.51 |
8.44 |
10.28 |
9.22 |
CIP |
0.00 |
0.00 |
0.61 |
4.81 |
8.17 |
9.11 |
4.66 |
5.23 |
6.85 |
4.09 |
Tech/MSP |
0.93 |
1.14 |
1.61 |
1.73 |
2.01 |
2.52 |
8.62 |
7.96 |
14.43 |
16.70 |
TOTAL |
43.13 |
51.55 |
55.00 |
73.97 |
84.41 |
83.44 |
84.38 |
83.55 |
95.48 |
94.54 |
NOTE: AAG, Astronomy and Astrophysics Research Grants; AAPF, Astronomy and Astrophysics Postdoctoral Fellows; ATI, Advanced Tezchnologies and Instrumentation; CIP, Community Instrumentation Programs (TSIP, AODP, PREST); ESM, Electromagnetic Spectrum Management; ESP, Education and Special Programs; Initiatives, AST funding to NSF- or MPS-wide programs; Inst/Tech, Instrumentation and Technology Development programs; Panels/IP As, Review panels and Intergovernmental Personnel Act appointments; Projects, Special Projects; R&E, Research and Education programs; STC, Science and Technology Centers; Tech/MSP, Technology devel-opment and mid-scale projects. a NSF’s ATI program fluctuated by only about 15 percent in FY2010 dollars over the decade 1999 to 2008. SOURCE: NSF Division of Astronomical Sciences. |
tation, and the ~3-year grant duration is long enough to cover much, but frequently not all, of a graduate student’s Ph.D.-thesis years. The Telescope System Instrument Program and the University Radio Observatory program, described in Chapter 6, help provide the facilities for students to learn observing procedures and develop new instrumentation. In Chapter 7 the committee recommends augmentations for several of these programs. However, some of the most compelling science opportunities and instrumentation frontiers—and therefore the areas of highest interest among young people—are beyond the scales of even the largest of these mid-scale programs.
A National Science Board report16 and a National Research Council report17 both emphasized that NSF should address the need for mid-size infrastructure, as have the NSF Division of Astronomical Sciences (NSF-AST) senior review,18 several AAAC annual reports,19 and multiple Committees of Visitors to NSF-AST, NSF-PHY, and the NSF Division of Materials Research of its Directorate for Mathematical and Physical Sciences (MPS) between 2003 and 2009. All of these reports stated that NSF needs a better mechanism to fund projects with costs between the top of the MRI funding bracket ($4 million to $6 million varying over the decade) and the bottom of the MREFC funding bracket (~$135 million).
Since at least FY2007, mid-scale instrumentation has been identified as a priority of NSF’s MPS directorate. In FY2009 and FY2010 mid-scale instrumentation was called out as a priority for NSF-AST, with increases resulting in expenditures of $32 million in FY2010. Beyond spending on GSMT, LSST, and SKA technology, design, and development, the projects funded include SDSS-II and SDSS-III, VERITAS, the Murchison Widefield Array, the Atacama Cosmology Telescope, PolarBeaR, and QUIET. Some of these were co-funded by NSF-PHY.
In Chapter 7, the committee recommends the establishment of a formally competed mid-scale instrumentation and facilities line within NSF-AST with additional funding beyond that currently being provided. The program would be focused specifically on the construction costs of instruments and facilities that fall between the top of the MRI and the bottom of the MREFC funding ranges. This survey received 29 proposals that would be eligible for such a competition, many of which were highly rated by the survey’s Program Prioritization Panels (PPPs) because they address directly the frontier science questions identified by the SFPs.
16 |
National Science Board, Science and Engineering Infrastructure for the 21st Century: The Role of the National Science Foundation, NSB 02190, National Science Foundation, Arlington, Va., 2003. |
17 |
National Research Council, Advanced Research Instrumentation and Facilities, The National Academies Press, Washington, D.C., 2006. |
18 |
National Science Foundation, From the Ground Up: Balancing the NSF Astronomy Program, Report of the NSF Division of Astronomical Sciences Senior Review Committee, National Science Foundation, Arlington, Va., 2006. |
19 |
Astronomy and Astrophysics Advisory Committee, Annual Report 2007 and Annual Report 2008, available at http://www.nsf.gov/mps/ast/aaac.jsp. |
TECHNOLOGY DEVELOPMENT
Technology development is the engine powering advances in astronomy and astrophysics, from vastly extending the scientific reach of existing facilities to opening up new windows on the universe. All of the Astro2010 PPPs emphasized the critical importance of technology development, and each stressed the urgent need to augment existing funding levels to realize important programs essential to reducing the technical, cost, and schedule risk of planned missions. Mission- or project-specific technology development must reach an acceptable level before accurate costs can be determined, priorities set, and construction scheduled. Failure to develop adequately mature technology prior to a program start also leads to cost and schedule overruns.
NASA-Funded Space-Based Astrophysics Technology Development
Technology development in three categories is discussed in this section:
-
Near-term mission-specific technology development is directed toward the particular requirements of a specific mission.
-
Mid-term or “general” technology development is aimed at maturing the technical building blocks (detectors, optics, and so on) that will enable high-priority science to be done on future missions with low risk and predictable cost.
-
Long-term or “blue sky” development supports novel ideas and approaches that could lead to transformational improvements in capability and enable missions not yet dreamed of—a level of technology development that is crucial to the future vitality of NASA.
Near-Term Mission-Specific Technology Development Needs
Ensuring adequate funding up front for mission-specific technology development is critical to predicting and managing mission costs and schedules.20 It has been reported that “in the mid-1980s, NASA’s budget office found that during the first 30 years of the civil space program, no project enjoyed less than a 40 percent cost overrun unless it was preceded by an investment in studies and technology of at least 5 to 10 percent of the actual project budget that eventually occurred.”21
20 |
Such technology development was also recommended in a 2009 NRC report, America’s Future in Space: Aligning the Civil Space Program with National Needs, The National Academies Press, Washington, D.C. Available at http://www.nap.edu/catalog.php?record_id=12701. |
21 |
John C. Mankins, The critical role of advanced technology investments in preventing spaceflight program cost overrun, The Space Review, December 1, 2008. Available at http://www.thespacereview.com/article/1262/1. Accessed May 2010. |
Mission-specific technology development funding has suffered substantial cuts over the past decade, cuts reflected in the immature state of a number of missions the survey committee has ranked as having very high scientific priority. The Astro2010 Panel on Particle Astrophysics and Gravitation found that further investment is needed in systems engineering and life-testing of components for the LISA Pathfinder mission, which is designed to demonstrate a number of LISA’s critical technologies. The Panel on Electromagnetic Observations from Space identified significant technology development needs for IXO, primary among them being the selection and demonstration of the critical X-ray optics. The survey committee also found IXO technologies to be too immature at present for accurate cost and risk assessment, and therefore recommends (in Chapter 7) significant investment in technology development during this decade so that IXO can be considered ready for a mission start early in the next decade. Instrumentation for the SPICA mission is a third area where specific technology development funds are needed during this decade. Determining the optimum funding levels is difficult, but NASA should collect and analyze the appropriate statistical data and apply sufficient funds for technology maturation for LISA, IXO, and SPICA.
Mid-Term Technology Development
Mid-term technology development enables defining, maturing, and ultimately selecting approaches to realize future scientific goals. In mid-term technology development it is usually necessary to pursue multiple paths to the same end, since both the detailed science requirements and the success of particular technologies remain uncertain. In addition, it is essential to pursue a broad range of technologies spanning the electromagnetic spectrum to ensure the vitality of competed mission lines and to pave the way for next-decade missions. The later stages of mid-term development are typically more costly than early-stage concept demonstration, because they may involve expensive prototypes or significant engineering efforts to design systems that can withstand testing in relevant environments.
The committee identified a number of high-priority science areas for which mid-term investments are needed beginning early in the decade, including development of a variety of technologies for exoplanet imaging, such as coronagraphs, interferometers, and star shades, leading to possible late-decade down-selecting. In addition, mid-term investment is needed for systems aimed at detecting the polarization of the CMB, and for optics and detectors for a future space UV space telescope. Broad-based mid-term technology development is also crucial to the Explorer program, which selects missions that can be implemented on short timescales.
Mid-term technology development is funded primarily through NASA’s Astronomy and Physics Research and Analysis (APRA) program, which was cut
considerably in the middle of the past decade. Although APRA funding has been restored to approximately 80 percent of its 2004 level (in FY2010 dollars), specific science priorities identified by the committee and its Program Prioritization Panels led the committee to recommend establishing these mid-term technology development programs and restoring APRA funding to a level matched to the needs of long-term technology development as described below. In Chapter 7 the committee recommends specific technology development programs in exoplanet, CMB, and UV instrumentation, as well as an augmentation to general mid-term development efforts that would ramp up by the end of the decade. Suborbital programs (balloons and rockets),which demonstrate scientific potential and test technologies in a space environment, are also critical in mid-term technology development and also are recommended in Chapter 7 for an augmentation.
Long-Term Technology Development
Long-term technology development builds the future of the space astrophysics program. It has become standard to achieve order-of-magnitude or greater increases in capability with each generation of missions, and exciting science breakthroughs have been achieved as a result. The only way to advance to the next capability without an exponential increase in mission costs is to find transformational new technological solutions. Some of the breakthroughs and advances have come from outside (such as microelectronics and near-IR detector arrays), but many of the technologies required in astrophysics are unique to the field, and their development must be supported from within. Examples of truly revolutionary technologies, essential to existing and upcoming astrophysics missions, that have been largely or entirely supported by NASA are X-ray imaging mirrors, X-ray microcalorimeters, and large arrays of submillimeter detectors. Future needs might include atomic laser gyros for pointing an X-ray interferometer, lightweight active mirror surfaces, new grating geometries, and novel techniques for nulling interferometry.
The appropriate level of investment in technology of long-term benefit is difficult to determine. A recent NRC report provides an excellent discussion of the metrics that should be used to establish and maintain a balanced technology development program but does not attempt to specify appropriate funding levels.22 It points out the clear importance of the long-range, high-risk, high-payoff component of technology development, noting that industry typically devotes 5 to 10 percent of R&D budgets to this component. Another report, which concluded that about 8 percent of a government entity’s research budget should be set aside for
high-risk research,23 also emphasized the difficulty of managing this type of development: when resources are limited, the temptation is always to cut long-range work in order to satisfy the more immediate demands of near-term technology requirements. Keeping the funding steady and healthy for promising long-term work while carefully evaluating it to avoid waste requires considerable attention from long-term program managers. An NRC recommendation to NASA was that the agency increase the number of scientifically and technically capable program officers, so that they could devote an appropriate level of attention to the tasks of actively managing the portfolio of research and technology development that enables a world-class space science program.24
Long-term technology development is funded at small levels from the APRA program. In the past, the Research and Engineering Directorate funded long-term and cross-cutting technologies (i.e., technologies with broad application within NASA), but this program was discontinued in the past decade. The committee was pleased to learn that NASA is planning to re-invigorate technology development across the enterprise, and it hopes that this effort will be managed in a way that provides an increased variety of opportunities for far-sighted work toward the future needs of astrophysics.
To address the issues raised above concerning support of mid-range technology development for future astrophysics missions, the committee recommends in Chapter 7 increases in the funding levels of NASA’s APRA and Suborbital programs. The adequate support of technology development for specific high-priority missions is also recommended in Chapter 7.
NSF-Funded Ground-Based Astrophysics Technology Development
The above discussion of the categories and benefits of technology development for the space program apply equally well to ground-based efforts, but the funding patterns are different.
At NSF, relatively near-term technology development is carried out in the course of instrument construction, for example at the national observatories and by the larger community funded by competitive grants from the MRI and ATI programs. The critical advancement of promising new technologies that are not yet ready for implementation, including next-generation and blue-sky technologies, is funded primarily by the ATI program. This aspect of NSF-AST
technology development will be crucial for meeting the needs for the program outlined in this report, including achieving the level of technology development and demonstration required before MREFC funding can be obtained for new major projects.
The types of high-risk, high-payoff technologies that can be transformative frequently take a large fraction of a decade to bring to the point of a convincing demonstration. An example of the kind of technological breakthrough that NSF is capable of enabling is adaptive optics with laser guide star technologies, which today improve the spatial resolution of ground-based images by factors of 20 to 50. The current outstanding performance of adaptive optics on 8- to 10-meter telescopes took more than a decade to achieve.
In view of the higher risk of potentially transformative technology development, one would expect ATI to have a substantial pipeline of projects under way with the realization that many will fail, but a few will succeed in dramatic fashion. In the decade from 1998 to 2008, ATI proposals had a somewhat higher rate of approval for funding than the average for NSF-AST, and the committee thinks that this is appropriate, given the great potential of new technologies for astronomy. The committee received community input in the form of white papers on the funding needs for technology development in areas such as adaptive optics, optical and infrared interferometry, millimeter and submillimeter detector arrays, and high-speed, large-N correlators. The Astro2010 Panel on Optical and Infrared Astronomy from the Ground and Panel on Radio, Millimeter, and Submillimeter Astronomy from the Ground made a convincing case that the current level of ATI funding should be augmented to enable successful pursuit of these highly ranked technology development programs and roadmaps. In Chapter 7 the committee recommends increased funding of the ATI program to meet the technology development needs of the future astronomy and astrophysics program.
DOE-Funded Technology Development
DOE-supported laboratories offer capabilities for technology development that are frequently not accessible at universities. As a result, unique technologies that could be key for astronomical advances are developed at DOE laboratories in support of primary DOE missions, and later adapted for astronomical applications. Examples include (1) the very-large-format detectors that are now being applied to wide-area astronomical imaging in the Dark Energy Camera, and potentially in LSST and WFIRST; (2) the dye lasers developed for the Atomic Vapor Laser Isotope Separation Program that were later modified and adapted for use in laser guide star adaptive optics systems; (3) the electron beam ion traps that were used to measure atomic physics processes for DOE’s nuclear weapons mission and subsequently used to measure cross sections relevant to astronomical X-ray spectroscopy; and
(4) the technologies from high-energy physics that are being used very successfully in the Fermi Gamma-ray Space Telescope.
DOE has been supporting specific technology development activities for JDEM and LSST, as well as more general technology development for TeV experiments and cosmic microwave background polarization experiments. Continuation of these activities is of great importance to the committee’s recommended program.
LABORATORY ASTROPHYSICS
The Scope and Needs of Laboratory Astrophysics
Laboratory astrophysics plays an important role in ensuring the success of current and future missions and observatories, as highlighted in four of the five Science Frontiers Panel reports. The field of laboratory astrophysics comprises experimental and theoretical studies of the underlying physics that produces observed astrophysical processes. Astronomy is primarily an observational science, detecting light generated by atomic, molecular, and solid state processes, many of which can be studied in the laboratory (see Figure 5.9). Our understanding of the universe also relies on knowledge of the evolution of matter (nuclear and particle physics) and of the dynamical processes shaping it (plasma physics), substantial parts of which can be studied in the laboratory.25 As telescope capabilities expand in wavelength coverage and precision, laboratory astrophysics plays an increasingly important role in the interpretation of data. At the same time, support for laboratory astrophysics has eroded, and a more robust system of funding to support personnel, equipment, and databases is needed to ensure efficient use and interpretation of hard-won astronomical data.
Traditionally research in astronomy has required atomic and molecular transition data for use in understanding spectra at wavelengths ranging from radio through X-ray wavelengths and for nuclear interaction cross sections. These topics were also at the forefront of research in their respective areas of physics, with the generation of such data heavily supported by NSF-PHY and DOE. There have always been some efforts focused entirely on astrophysics, but the bulk of the data came “for free” from the physics community and especially the national laboratories. The frontiers of physics have evolved, particularly in the field of atomic, molecular, and optical science, and little work of this type is now done
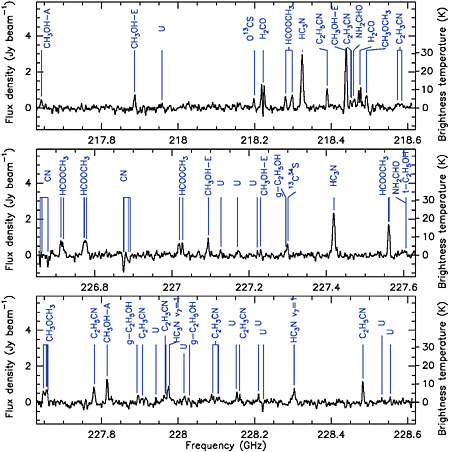
FIGURE 5.9 The richness of the submillimeter spectrum for probing molecular chemistry in regions where stars are born, illustrated with SMA data. Note the number of lines that are at present unidentified (“U”). The promise of SMA, ALMA, and CCAT will be enhanced with additional laboratory astrophysics work. SOURCE: C.L. Brogan, T.R. Hunter, C.J. Cyganowski, R. Indebetouw, H. Beuther, K.M. Menten, and S. Thorwirth, Digging into NGC 6334 I(N): Multiwavelength imaging of a massive protostellar cluster, Astrophysical Journal 707:1-23, 2009. Reproduced by permission of AAS.
in physics departments. At the same time, astronomy’s needs have expanded with the progression into new wavelength regimes and the rapid increase in measurement capabilities. For example, precision experiments on magnetized plasmas under astrophysical conditions are becoming available, as are high-energy-density experiments that make use of giant lasers and magnetic pinches to create relevant conditions for heating and shock propagation. In addition, it is possible to use these experiments to advance understanding of magnetic reconnection, which is of vital
importance in solar physics. The combination of these factors leads to a need for an increase in the level of support for laboratory astrophysics.
There is also an increased interest in non-traditional areas of laboratory astrophysics such as high-energy-density phenomena. Realization of the importance of magnetized plasmas in interstellar and intergalactic space has generated a need for basic information on the behavior of such plasmas, often in physical regimes far from those currently being studied for their application to magnetic fusion reactors. Laboratory measurements will allow us to understand the formation of molecules in interstellar space and stellar atmospheres, both critical for studies of star formation, for example by studying the complex chemical reactions on the surface of dust grains. DOE’s high-energy-density facilities26 will be able to host laboratory astrophysics experiments relevant to outstanding questions in radiative hydrodynamics, equation-of-state measurements relevant to planetary interiors, and turbulent flow. Those facilities are also performing experiments important to high-energy astrophysics, specifically involving the behavior of hot plasmas and dynamical magnetic field configurations.
The Science Frontiers Panel reports call out specific needs for research in laboratory astrophysics in order to accomplish the proposed research objectives for the next decade. New capabilities require expanded laboratory astrophysics research in the X-ray, UV, millimeter and submillimeter, and IR regimes as missions such as Herschel, JWST, and ALMA go forward. The SFP reports highlight the need for tabulation of spectral features for ions, molecules, and clusters of atoms. Additionally, measurements of gas-phase cross sections, for example of the polycyclic aromatic hydrocarbon molecules found in star-forming regions, are needed to understand the absorption features seen in the spectra of galactic objects. A better understanding of dust and ice absorption spectra and the chemistry of molecule formation is also needed.
The Funding Challenge
NSF-PHY support for laboratory astrophysics has declined to about one-third of that provided two decades ago. Despite an increase in the number of NSF-AST laboratory astrophysics awards in atomic and molecular physics, the combined NSF-PHY plus NSF-AST laboratory astrophysics support has fallen to about half of what it was 20 years ago.
Short-term funding for laboratory astrophysics, such as that tied to observing cycles, is inadequate for the health of stable laboratory astrophysics programs, and
some source of stable base funding is needed to support experimental facilities. National laboratories, especially those under the Department of Energy’s Office of Science and the National Nuclear Security Administration, may be the most dependable long-term reservoir of capability, given that most of these topics are no longer central to the interests of basic physics at universities. The work of compiling the data into useful catalogs and databases is probably still best done by astronomers, and it is vital to maintain databases of important astrophysical results. Such work might be done at national laboratories or at major data centers but has to be coordinated among all investigators.
CONCLUSION: DOE national laboratories, including those funded by the Office of Science and the National Nuclear Security Administration, have many unique facilities that can provide basic astrophysical data.
In summary, the need for laboratory astrophysics has increased because of new and highly capable observing modes that require investigation and because of the relevance of laboratory astrophysics to other physics and engineering problems. Thus a systematic, long-term, and robust funding strategy is required in order to ensure successful scientific returns from missions and programs. Support requires people, instrumentation, and maintenance of databases. NSF-AST support has been increasing, but at far from a sufficient rate to compensate for the loss of input from the atomic physics community and the increased needs of modern astronomical observations.
RECOMMENDATION: NASA and NSF support for laboratory astrophysics under the Astronomy and Physics Research and Analysis program and the Astronomy and Astrophysics Research Grants program, respectively, should continue at current or higher levels over the coming decade because laboratory astrophysics is vital for optimizing the science return from current and planned facilities. Missions and facilities, including DOE projects, that will require significant amounts of new laboratory research results to reach their science goals should include within their program budgets adequate funding for the necessary experimental and theoretical investigations.