Since the beginning of armed conflict, armor has played a significant role in the protection of warriors. In present-day conflicts, armor has inarguably saved countless lives. Over the course of history—and especially in modern times—the introduction of new materials and improvements in the materials already used to construct armor have led to better protection and a reduction in the weight of the armor. Body armor, for example, has progressed from the leather skins of antiquity, through the flak jackets of World War II to today’s highly sophisticated designs that exploit ceramic plates and polymeric fibers to protect a person against direct strikes from armor-piercing projectiles (Figure 1-1). The advances in vehicle armor capabilities have similarly been driven by new materials, as shown in Figures 1-2 and 1-3.
But even with such advances in materials, the weight of the armor required to manage threats of ever-increasing destructive capability presents a huge challenge. For example, body armor, which presently constitutes almost 30 percent of a soldier’s fighting load,1 is the single largest weight carried by an Army rifle squad. For vehicles, up-armored Humvees have reached the limit beyond which armor cannot be added without “compromising essential vehicle capabilities.”2
The challenge for protective material developers, made clear by current military engagements, is twofold: (1) to ensure the rapid (re)design and manufacture of armor systems optimized against specific threats and (2) at the same time, ensure that these systems are as lightweight as possible. As described above, many of the advances in the performance of lightweight armor have historically come from the introduction of new or improved materials. However, it has become increasingly difficult to produce new materials with properties that allow the design of complex new armor systems or the rapid iterations of such designs. Not only must a material be quickly identified, but it must also be reliably produced,
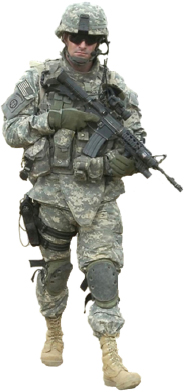
FIGURE 1-1 A soldier wearing protective equipment. SOURCE: Adapted from Gaston Bathalon, Commander, U.S. Army Research Institute of Environmental Medicine, “The Soldier as a Decisive Weapon: USAMRMC soldier focused research,” presentation to the Board on Army Science and Technology on February 15, 2011.
______________
1Dean, C. 2008. The modern warrior’s combat load: Dismounted operations in Afghanistan. 2003. Medicine and Science in Sports & Exercise 40(5): 60.
2Inspector General, U.S. Department of Defense. 2009. Procurement and delivery of joint service armor protected vehicles. Report No. D-2009-046. Available online at http://www.dodig.mil/audit/reports/fy09/09-046.pdf. Accessed April 7, 2001.
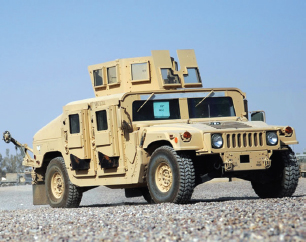
FIGURE 1-2 Up-armored high-mobility multipurpose wheeled vehicle (HMMWV, or Humvee). SOURCE: Available at http://www.militaryfactory.com/armor/imgs/hmmwv-m1114uah.jpg. Courtesy of U.S. DoD.
which is not currently possible with the extensive, costly, and time-consuming practice that is perhaps best described as “build it, shoot it, and then look at it.” This problem, including specific recommendations for areas of investigation, will be addressed further at the end of Chapter 3.
This seeming technological inability to keep up with evolving needs is not exclusive to protection materials. A recent National Research Council (NRC) study, Integrated Computational Materials Engineering: A Transformational Discipline for Improved Competitiveness and National Security,3 describes how, like advances in armor, the “vast majority of disruptive technologies since the start of the industrial revolution” have been due to materials innovations, but that “the insertion of new materials technologies has become much more difficult and less frequent” as materials development fails to keep pace with the rapid design process. This describes exactly the problems experienced with development of the new protection materials that are the focus of this study. The Integrated Computational Materials Engineering (ICME) report cites many advances and several examples of successful implementation. It advocates pushing the large body of existing computational materials science to the next step. Unfortunately, while “the optimization of the materials, manufacturing processes, and component design” is well described in the ICME report, the path forward for protection materials is far more complicated, since designs must deal with highly nonlinear and large deformations typically not encountered in commercial products, where applied stresses are kept well below the elastic limit in the linear regime. Simply put, the key materials properties—for example, tensile strength and toughness—that inform the design of commercial structures and devices are well established and extensively measured. Such is not the case for armor.
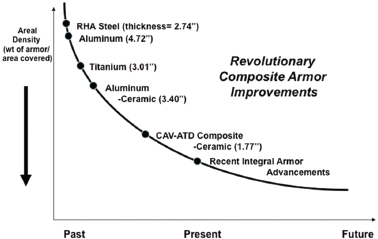
FIGURE 1-3 Areal density of armor versus time, demonstrating that new lightweight materials such as titanium, aluminum, and ceramics have provided increased protection at a lower weight per unit area over time. The flattening curve illustrates that the challenge for the future is to be able to continue to decrease the areal density of the armor despite increased threats. SOURCE: Adapted from Fink, B.K. 2000. Performance Metrics for Composite Integral Armor. ARL-RP-8. Aberdeen Proving Ground, Md.: Army Research Laboratory.
The armor that protects U.S. fighting forces is seldom a single, homogeneous material. More often than not, what is called “armor” is actually a complex system constructed of several, often quite different, materials arranged in a very specific configuration designed to protect against a particular threat. As will be discussed extensively in this study, the properties and behavior of a protection material must be considered in the specific context of how it will be used in the construction of a particular armor system. Further, there is often little understanding of how to link specific material properties to the actual behavior of the materials and armor systems during the many types of ballistic and blast events. It is often the case that new protection materials have not been well characterized with respect to strain rates, pressures, and the like under appropriate conditions, either alone or as part of an armor system, and databases for materials’ performance and constitutive relationships are often not available. This is especially true at the high strains and very high strain rates relevant to ballistic and blast threats. This gap in knowledge greatly limits the ability of simulation codes to play a significant role in guiding the development of new materials. Moreover, the design philosophy is completely dependent on how the armor system is to be used.
In this study, the committee was guided by military applications that necessitate lightweight armor, with particular emphasis on (1) personnel protection, which includes body armor and helmets, (2) vehicle armor, and (3) transparent
______________
3NRC. 2008. Integrated computational systems engineering: A transformational discipline for improved competitiveness and national security. Washington, D.C.: The National Academies Press.
armor for face shields, vehicle windows, and other applications requiring transparency. For each of these applications, system-level constraints affect armor design and, ultimately, the design and choice of protection materials. The committee viewed the need for strong coupling between armor system designers and protection materials developers as the most difficult challenge to be addressed.
The Assistant Secretary of the Army (Acquisition, Logistics, and Technology) requested that the NRC’s Board on Army Science and Technology and its National Materials Advisory Board collaborate to form an ad hoc study committee to investigate opportunities in protection materials science and technology for the Army.
The committee was given the following statement of task:
An ad hoc committee will conduct a study and prepare a report on protection materials for the Army to explore the possibility of a path forward for these materials. Specifically, the committee will:
1. Review and assess the current theoretical and experimental understanding of the major issues surrounding protection materials.
2. Determine the major challenges and technical gaps for developing the future generation of light weight protection materials for the Army, with the goal of valid multi-scale predictive simulation tools for performance and, conversely, protection materials by design
3. Suggest a path forward, including approach, organizational structure and teaming, including processing, material characterization (composition and microstructure), quasi-static and dynamic mechanical testing and model development and simulation and likely timeframes for the Army to deliver the next generation protection materials.
The sponsor requested that in considering the questions posed by the task statement, the committee should consider the following:
- Shock wave energy dissipative (elastic, inelastic and failure) and management mechanisms throughout the full materials properties spectrum (nano through macro).
- Experimental approaches and facilities to visualize and characterize the response at nano and mesoscales over short time scales.
- Multi-scale modeling techniques to predict energy dissipative mechanisms (twinning, stacking faults, etc.) from the atomic scales and bulk material response.
- Materials and material systems issues including processing and characterization techniques focusing on intrinsic (single crystal) properties and processing controlled extrinsic characteristics (phases, microstructure, interfaces).
The sponsor further requested that the study not include restricted material so as to permit wide dissemination of study results to the research and development communities.
The study consisted of six full two- or three-day committee meetings held mostly in Washington, D.C., but also included a three-day meeting held near Aberdeen Proving Ground, in Maryland, one day of which was devoted to visiting the U.S. Army Research Laboratory and observing some of the relevant experimental testing facilities. The committee received briefings from academic, industrial, military, and government presenters covering lightweight materials for warfighter protection as well as vehicle protection. Topics ranged from ballistic threats to blast threats and from very hard to relatively soft armor materials and included a brief from the National Aeronautics and Space Administration on protection of space vehicles against hypervelocity impacts from meteors. The committee met in closed sessions to develop conclusions and recommendations responsive to the study task, drawing upon the materials presented in open sessions and additional published materials cited throughout the report.
The report contains 6 chapters and 10 appendixes. This first chapter provides the introduction and background to the study and defines the overall perspective of the report. Chapter 2 introduces the reader to some armor systems and gives examples relating to the key concept of reducing the areal density of the protection materials while improving the performance of armor against ever-increasing threats. Chapter 2 also makes the important distinction between armor systems and material systems.
Chapters 3, 4, and 5 provide the technical details of the committee’s assessment of current knowledge and discuss the gaps and opportunities meriting high priority in future research. In order to appreciate the task for designing materials for armor, Chapter 3 covers the complex interacting mechanisms and processes that take place during deformation and failure when a material is impacted by a high-velocity penetrator.
Chapter 4 addresses the computational and experimental approaches to armor material design and the need to better couple and integrate these activities to create materials by design and armor systems by design. Multiscale modeling and simulation are reviewed for a few key scenarios for threat-protection materials, illustrating the considerable challenge of accurately capturing the extreme deformations involved in penetration. The goal is to enable much more rapid advances in both materials and systems and, accordingly, a much faster and better response to changing threats.
Chapter 5 provides a broad perspective on the structure and composition of exemplary protection materials including ceramics, polymers, metals, and composites. It highlights the most exciting opportunities in materials research—opportunities that may lead to revolutionary advances in protection and a significant reduction in areal density. This chapter is extensively appended with descriptions and processing for specific materials.
Chapter 6 suggests a path forward and recommends future research tied to the conclusions of the earlier chapters. To realize all the potential gains for protection materials noted in the report, an important new paradigm is proposed, along with an organizational plan for its implementation.
Collectively, these chapters provide technical recommendations and a proposed way forward for long-term research directed at the development of the following:
- A fundamental understanding of how a ballistic object or a blast interacts with a material—in other words, the material’s performance. This would include an understanding of which time and length scales are important and how controlling the material’s composition and microstructure, and hence its mechanical behavior, contributes to altering the deformation mechanisms and improving performance;
- Experimental approaches to identify and quantitatively characterize the mechanisms and processes that lead to damage during these dynamic events;
- Quantitative relationships for the evolution of the damage during a high-deformation event and extending these relationships to account for multiple events, termed multi-hit relationships;
- Computational approaches—coupled with synergistic experiments that inform and validate—to predict the performance of specific protection materials in an integrated armor configuration;
- Model-driven methods to design new materials or improve existing ones to meet the behavior criteria for successful protection;
- Model-driven synthesis, processing, and manufacturing capabilities to produce affordable materials in quantities needed for defense applications; and
- An environment that allows successful interplay and collaboration among the DoD, government labs, industry, and academe while at the same time addressing security and organizational matters.
Appendix A includes the Statement of Task, Appendix B provides biographical sketches of the committee members, and Appendix C lists committee meetings and speaker topics. Appendixes D through J contain much additional detailed information on protection materials, supplementing the points made in Chapters 3, 4, and 5.
The sponsor asked that the committee suggest both an organizational structure and a teaming approach as part of the path forward. In considering the sponsor’s request that the study report not include restricted material so as to enable wide dissemination to the research and development communities, the committee recognized a broader issue—namely, that restricted information is a barrier to research collaborations.4Chapter 2 addresses armor system design at the unrestricted level but closes with a comment on the extensive regime of security and export control restrictions that affects research on protection materials. Several speakers from industry, government, and academic organizations told the committee that these restrictions make it extremely difficult for fundamental research in protection materials to be usefully communicated among the various organizations and to be connected to the development of armor systems, which entails restricted information. It notes that a review of classification guidelines and export control restrictions would facilitate clearer, more up-to-date boundaries for the necessary control of information. Chapter 6 proposes an organizational structure to bridge this gap.
The committee’s key recommendations are presented in Chapter 6, with ancillary recommendations found in Chapters 3 and 4. The overall thrust of this report, however, is evident in the following overarching recommendation:
Overarching Recommendation. Given the long-term importance of lightweight protection materials to the Department of Defense (DoD) mission, DoD should establish the defense initiative protection materials by design (PMD), with associated funding lines for basic and applied research. Responsibility for this new initiative should be assigned to one of the Services, with participation by other DoD components whose missions also require advances in protection materials. The PMD initiative should include a combination of computational, experimental, and materials testing, characterization, and processing research conducted by government, industry, and academia. The program director should be given the authority and resources to collaborate with the national laboratories and other institutions in the use of unique facilities and capabilities and to invest in DoD infrastructure where needed.
This overarching recommendation requires actions in four important elements of the PMD initiative:
______________
4A detailed discussion of the effects on research of classification guidelines, security, and export controls is beyond the scope of this study.
- Element 1. Fundamental understanding of mechanisms of deformation and failure due to ballistic and blast threats.
- Element 2. Advanced computational and experimental methods.
- Element 3. Development of new materials and material systems.
- Element 4. Organizational approach.
The chapters that follow develop the rationale and conclusions that underpin the detailed recommendations in Chapter 6 and identify actions that are needed to address the four elements of the initiative. The committee is unanimous in its support of these recommendations.