Whether the NASA MMOD programs focus on protecting the space environment or the spacecraft, monitoring, reporting, and analysis of satellite anomalies are of vital importance. Particulate-induced anomalies could provide valuable validation of environment characterization of objects within critical size ranges (5 mm to 10 cm for debris and 10–11 g for meteoroids) and velocities (7 km/s for debris, up to 72 km/s for meteoroids if in bound solar orbit), as well as a better understanding of operational effects owing to particulate impacts.
Satellite anomalies are mission-degrading or mission-terminating events affecting on-orbit operational spacecraft. However, it is not normal procedure to provide information on these anomalies to the public or even to other offices within the same organization, for to a variety of reasons: limited staff for reporting and analysis, concerns about system reputation, desire to protect proprietary information, uncertainty in the meaning or cause of the events, national security, and so on. Depending on their severity, a program operations philosophy, and an available staff, anomalies are recorded and analyzed to some degree. Individual operational satellite programs, such as Iridium, Defense Meteorological Satellites Program, and others, use such information as a means to (1) assess system performance, (2) determine potential changes in operations, or (3) diagnose the cause of an event.
There is no standard nomenclature for describing system symptoms associated with anomalies or how they are recorded, shared, resolved, or stored. There is no standard approach to prioritizing steps in a process for addressing an anomaly, including recording, resolution, and/or determination of cause. Many system operators are much more concerned about getting their satellite back into operation than about determining the cause of a failure. Repeat failures often get examined much more rigorously.
Typically, the following causes of anomalies are considered: routine failures of parts, electrostatic discharge, single-event upset, command error, particulate impact, and unknown. Unfortunately, there is no standard resolution process to determine the cause of an anomaly. The process of determining a cause is unreliable, and the degree of confidence applied to any one cause is minimal. “Unknown” is attributed to the vast majority of anomaly cases, since it is so difficult to determine exactly what happens in space without dedicated instrumentation to provide insights from on-orbit encounters that adversely affect satellite operations. There may be times when an “unknown” is erroneously attributed to a meteoroid or orbital debris event. Or there may other times when additional data indicates a high probability that the failure was caused by an MMOD event (see Box 10.1).
From a flight safety perspective (i.e., protecting the spacecraft), determining the cause of anomalies in space is important to better assess how the system will continue to function and how future systems might perform. From an environment characterization perspective, accurate accounting of environment-based anomalies provides
BOX 10.1
Damage Analysis
After nearly 7 years of service providing power to the International Space Station (ISS), the P6 solar photovoltaic power module (P6) was moved from its original location on the Z1 truss to its permanent location on the port outboard truss of the ISS. When the crew of the STS-120 mission redeployed the solar array in its new location in 2007, the array started to tear in two places as shown in Figure 10.1.1, forcing the crew to stop deployment at 90 percent of completion.
If this had been a robotic spacecraft, the only clues available to ground controllers would have been those provided by telemetry to guide their analysis of what had caused the failure, with the result perhaps being a conclusion that the failure was only mechanical, or a design flaw.
However, the STS-120 astronauts were able to cut away the frayed guidewire that caused the tear in the panels and bring it back to Earth for analysis. NASA scientists examined the frayed end of the guidewire with a scanning electron microscope, discovering damage to it in the form of melting that was characteristic of fusing from the impact of a meteoroid or orbital debris. Further examination using a narrow-focus electron microprobe and energy dispersive x-ray spectrometer to analyze the chemical elements in the impact zone resulted in the conclusion that the damage was caused by orbital debris, not a meteoroid.
Currently, most robotic spacecraft do not have systems sophisticated enough to provide the kind of detailed information necessary to say conclusively that an MMOD impact caused a failure of some part or system. However, clues that MMOD may be a cause are found by correlating a failure with data on the environment at the time (e.g., the spacecraft passed through a meteor shower or an orbit known for large amounts of debris), or an unexplained change in momentum. In this case, NASA’s astronauts, and scientists, were fortunate to retrieve a piece of hardware directly involved in damage to the spacecraft and to be able to thoroughly examine that hardware in the lab. Without it, they would not have had all of the pieces of the puzzle to solve this mystery.1
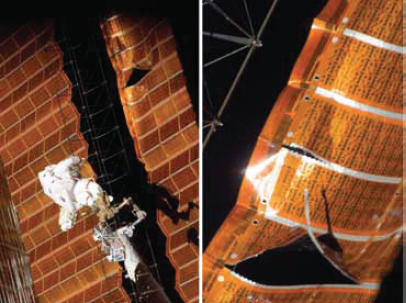
FIGURE 10.1.1 Two tears approximately 30 cm and 90 cm long in the P6 solar array wing 4B after an attempt to redeploy the array during the STS-120 mission. SOURCE: Courtesy of NASA, from D. Ross, E. Christiansen, and D. Lear, MMOD damage to the ISS solar array guidewire, Orbital Debris Quarterly News 15(1):4, January 2011.
___________________
1 D. Ross, E. Christiansen, and D. Lear, MMOD damage to the ISS solar array guidewire, Orbital Debris Quarterly News 15(1):4, January 2011.
another means to help characterize the environment and validate environment models. It is possible that impacts from debris and meteoroids of sizes as small as 100 microns and as large as several centimeters might adversely affect operational systems without the satellite owner being able to determine that an impact had occurred, other than by evaluating how the system functioned after the impact.
Figure 10.1 depicts generally how the orbital debris population presents a challenge from the perspective of collision risk and environment characterization. Basically, the smallest debris objects are only characterized by returned samples; their impact is not normally a concern since the hazard can be largely managed by shielding and inherent design; yet the flux (impacts per exposed area over time or probability of collision) of debris of this size is the highest.
In the debris population, the largest objects are the least numerous; however, the potential consequence, if an impact were to occur, is catastrophic. These objects are cataloged and so potentially can be avoided. The medium size range, approximately 5 mm to 10 cm for orbital debris, is the most difficult to characterize, with only episodic radar measurement campaigns contributing to knowledge of the environment, yet the risk (probability of collision times consequence) may be the greatest in this size range.
Undoubtedly, impacts with debris in the medium size range should be occurring much more often than collisions between trackable objects. It is this “residual risk” that may be better characterized by clearer, consistent recording, analysis, reporting, and sharing of satellite anomalies, since such orbital encounters cannot be observed from the ground. However, even the lower end of the range of sizes at which particles might be causing spacecraft anomalies is highly uncertain because of the wide variety of space system designs encountered, varying impact speeds and directions, and a wide range of particle characteristics. These uncertainties hold for both meteoroids and orbital debris.
For orbital debris, the size threshold for particles likely involved in anomaly-inducing impacts in LEO is significantly different from the threshold in GEO, because impact velocities are much greater in LEO and the smallest object regularly cataloged in GEO is 1 m, compared with but 10 cm in LEO. As a result, the anomaly resolution process will be slightly different in the two orbits, adding yet another complexity to the analysis of satellite anomalies.
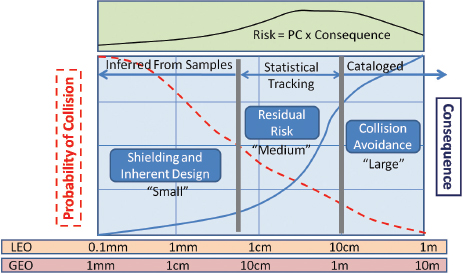
FIGURE 10.1 Risk versus debris impactor size. The debris sizes of concern in these categories are roughly offset by a factor of 10 between low Earth orbit (LEO) and geosynchronous Earth orbit (GEO). A compilation of satellite anomalies would provide insight into the environment and subsequent effects that might potentially pose the greatest overall risk to current spacecraft.
For the natural particulate environment, it is critical to determine just how smaller, faster meteoroids might create mission-degrading and mission-terminating failures. Significant debate and ongoing research are focused on the possibility that particulate-catalyzed electrical failures may contribute measurably to the compendium of satellite anomalies. Strong data from experimentation show that plasma is produced during a hypervelocity impact event. At meteoroid velocities such free charges near a spacecraft might be expected to cause electrostatic discharge (ESD) and electromagnetic pulse (EMP) events (for additional detail on these phenomena, see Chapter 4, “The Meteoroid Environment and Its Effects on Spacecraft”). In addition, ground-based experiments have shown that significant plasma is produced when a particle impacts a satellite even at speeds typically associated with orbital debris (around 7 km/s). The electrical anomalies resulting from either a debris or a meteoroid impact remain poorly characterized.1
Although it will be difficult to determine the contribution to anomalies from meteoroids versus orbital debris, it is important to gain an understanding of that balance to provide a forcing function for future research and operational procedures. This issue is also discussed in Chapter 3, “Orbital Debris Modeling and Simulation.”
Just as the Orbital Debris Program Office works to significantly increase measurements of the sub-5-mm population through returned samples and of the 1- to 10-cm population through Haystack measurement campaigns, developing standard processes for recording, analyzing, reporting, and sharing satellite anomalies will provide a rich source of information not only on the environment flux but also on the effects on satellite systems of impacts by particles in the 5 mm to 10 cm size range.
An examination performed on a limited set of records for the past 20 years for satellite anomalies identified discrepancies in the available data that may make any post facto analysis of limited use, and it is essential that NASA now prepare for the future and establish a means to change this situation going forward. Many physical phenomena are reported indirectly but are crucial for determining the impactor that caused an anomaly: sudden pointing direction change, sudden drop in current from solar arrays, discrete voltage changes on instruments or power system, and so on can all be used to diagnose an anomaly.
Previous analyses have focused on the probability of impact equating to a probability of physical damage, yet it is possible that an impact itself may not be the only causative mechanism for failure. The impact may directly create an EMP or be the catalyst for an electrostatic discharge, as hypothesized above for fast meteoroids.
The difficult chore of writing and promulgating a standard process for satellite anomaly recording, analyzing, reporting, and sharing could leverage the successful migration of debris mitigation guidelines into international standards. This new effort can also leverage the current outreach and international dialog in which ODPO participates at the Inter-Agency Space Debris Coordination Committee, the United Nations, and other such organizations.
Databases already exist that contain spacecraft anomalies. An example is the Aerospace Corporation’s Space System Engineering Database (SSED).2 The SSED provides valuable data related to satellite failures and anomalies; however, it does not provide sufficient coverage or fidelity to permit the development of causative relationships with MMOD particulate environments. Attempts to use existing databases have produced results suggesting that a statistical relationship might exist between certain types of failures and the MMOD environment, but its insufficiency makes the information in the databases conclusive.3
Development of standard processes for characterizing satellite anomalies will also provide a database that will complement the continued measurement campaign in this size range, which in turn will support the continued refinement of MEM, ORDEM, and BUMPER. Predicted flux levels of orbital debris in the 5 mm to 10 cm size range and for meteoroids (10–11 g) in the 100 µm to 1 cm size range can be correlated with the actual number of satellite anomalies, creating a tighter linkage between environment definition and satellite operations.
___________________
1 See D.A. Crawford, and P.H. Schultz, Electromagnetic properties of impact-generated plasma, vapor and debris, International Journal of Impact Engineering 23:169-180, 1999; and Burchell et al., 1996.
2 See J.F Binkley, Aerospace Corporation, “The Space System Engineering Database (SSED),” demonstration, available at http://klabs.org/mapld04/tutorials/mishaps/intro_aerospace.htm.
3 D. McKnight, W. Riley, I. Shukry, and A.Shukry, Correlation of spacecraft anomalies to the debris environment, Proceedings of SPIE 2813:185-196, 1996.
Finding: Spacecraft anomalies are a direct measurement of both the state of the particulate environment in space and the adequacy of a spacecraft design. However, no formal recording, analyzing, sharing, and reporting procedures exist to take advantage of data on spacecraft anomalies despite that data’s potential as valuable information about particulates in a critical size range that is typically not sampled continuously.
Recommendation: NASA should initiate a new effort to record, analyze, report, and share data on spacecraft anomalies in order to better quantify the risk posed by particulates too small to be cataloged yet large enough to disrupt spacecraft operations. The results of this effort would provide general insights into the effects of meteoroids and orbital debris on operational space systems. Eventually, this effort could provide data to upgrade current MMOD models—the Meteoroid Environment Model, Orbital Debris Environment Model, and BUMPER.