4
Fuels and Chemicals from Biomass via Thermochemical Routes
“If you can provide inexpensive lipids, we can turn them into aviation fuel, diesel, and gasoline without too much trouble.”
Robert Brown
The two primary approaches to using thermochemical process to convert biomass into fuels and chemicals are gasification and pyrolysis. Gasification produces syngas, a mixture of carbon monoxide and hydrogen that is already used in the petrochemical industry. Pyrolysis produces bio-oil, a thick, corrosive mixture that in some respects resembles crude oil, and charcoal, which can be used as either an energy source or a carbon sequestration agent. Both approaches yield intermediates that are then used as feedstocks for further processing. An advantage of using thermochemical processing, as opposed to biological processing, is that it can more readily break down lignocellulosic materials in a controlled manner to produce high concentrations of desired intermediates. An important obstacle to thermochemical processing is the inorganic contaminants in biomass, which can foul the catalysts used to convert syngas or bio-oil into fuels and chemicals, said Robert Brown, founding director of the Bioeconomy Institute at Iowa State University.
THERMOCHEMICAL ROUTES TO FUELS AND CHEMICALS
The choice of whether to use a biochemical route, which uses enzymes and microorganisms to generate desired products, or a thermochemical route, which uses heat and catalyst to generate product, depends on the type of feedstock being processed, said Brown. The three major classes of biomass, he explained, are lipid-rich biomass, which historically has been soybeans but that would include algae in the future; lignocellulosic biomass; and waste biomass, which is a mixture of all different kinds of feedstocks.
The beauty of lipid feedstocks is that they are nearly hydrocarbons that are not difficult to turn into aviation fuel, diesel, and gasoline, Brown noted. In fact, petroleum companies have developed and proven large-scale processes for making fuels from lipid biomass, but all of these operations have been shut down for one reason, said Brown—the high cost of the feedstock. If ongoing research can successfully develop cheaper feedstocks, such as those that could grow on marginal land, thermochemical conversion of those feedstocks into fuels will be attractive. Algae may prove to be one of those feedstock sources, but algae also produce high levels of protein that will need to be dealt with in an economically viable manner, by turning it into either fuel, which will involve catalytic removal of nitrogen, or food.
Lignocellulose is naturally recalcitrant to degradation, but thermochemical approaches can break down lignocellulose in a controlled manner that produces high concentrations of desirable molecules. See Figure 4-1 for lignocellulose’s structure. The question that needs to be asked, said Brown, and one that he does not have an answer to, is whether efforts should focus on lignocellulosic or lipid feedstocks. In essence, this comes down to a decision as to what kind of plant should be used to deoxygenate carbohydrates—a petrochemical-type plant that produces carbon dioxide and water as the waste stream, or a green leafy plant that also deoxygenates and decarboxylates sugars in situ.
Gasification and Pyrolysis
Turning to the concept of thermochemical processing, Brown explained that the basic idea is that a feedstock is depolymerized into what DOE calls a feedstock intermediate and what Brown calls a thermolytic substrate. The thermolytic substrate then undergoes some type of upgrading, either through biological or chemical processes, to produce a biofuel. The two major types of thermochemical processes are gasification and pyrolysis.
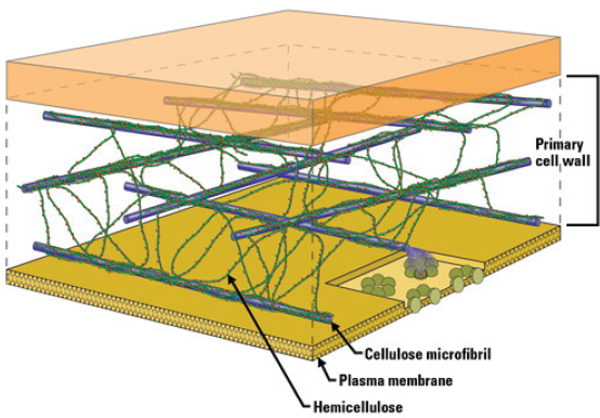
FIGURE 4-1 Lignocellulose structure.
SOURCE: Office of Biological and Environmental Research of the U.S. Department of Energy Office of Science.
Gasification, Brown explained, is the thermal decomposition of organic matter into flammable gases, using either a bubbling fluidized bed reactor or an entrained flow gasifier to produce syngas. These technologies have been commercialized for coal, but in some respects they work even better with biomass. Though gasification is in theory an equilibrium process at very high temperatures and long residence times, in practice equilibrium is obtained rarely and so the process generates tar, char, and small amounts of contaminants that agronomists would like to claim as nutrients. These contaminants, which include small amounts of alkali metals, sulfur, nitrogen, and chlorine that must be removed before upgrading in order to prevent poisoning of catalysts. Removal of each contaminant requires its own catalyst, adding substantial costs to any gasification process.
A significant advantage of the gasification process is that there is no question of what to do with lignin, as it is turned into syngas, too. Gasification can also handle virtually any feedstock, including waste streams, and produce a uniform intermediate product for upgrading. It can also be used to produce heat, power, fuels, or chemicals and allows for energy integration into biorefinery operations.
Technical challenges are particularly challenging in terms of developing technologies that can cost-effectively remove contaminants from the gas stream (Figure 4-2). Gasification operations must also be integrated with fuel synthesis operations, which is not a simple matter given that fuel synthesis occurs at high pressures and under exacting stoichiometries. From a commercial perspective, gasification only works economically at large scale, which translates into high capital costs that could be as high as $10 per gallon of annual plant capacity. Brown added that biomass gasification must also compete with steam reforming of natural gas, which while not a renewable resource is a domestic resource that would move the country away from imported petroleum and reduce greenhouse gas emissions compared to petroleum.
The other major thermochemical technology is fast pyrolysis, which rapidly heats biomass in the absence of oxygen to produce three products: syngas, charcoal, and a liquid mixture of organic compounds and water known as bio-oil that is recovered from pyrolysis vapors and aerosols (see Figure 4-3). Charcoal, also known as biochar, can be used as a carbon sequestration agent. In combination with the bio-oil, biochar presents an opportunity for producing carbon-negative fuel, said Brown.
Fast pyrolysis, he explained, is characterized by residence times of 0.5 to 2 seconds, a very high rate of heating at moderate temperatures of 400–500°C, and the production of a liquid that looks like petroleum but smells like barbecue sauce. Typically, 60–70 percent of the weight of biomass is converted to bio-oil (see Figure 4-4). The yield of biochar ranges from 13 to 15 percent and that of syngas is in the 13–25 percent range. The syngas can be used as an energy source to support this process.
Pyrolysis chemistry is poorly understood, and Brown stressed the need for chemists and chemical engineers to study this process. Studies at Iowa State have found that pyrolysis converts cellulose into products in a number of competing parallel pathways. This work has also shown that alkali present in biomass acts as a powerful catalyst that produces undesired light oxygenates, and so research is needed to understand how to control or suppress this process. Chemists can play a critical role in this research and help produce a more valuable product in the end. (To see a figure of pyrolsis chemistry, please see Figure 4-5.)
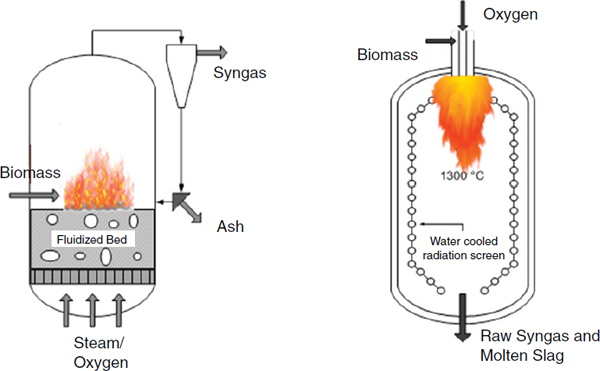
FIGURE 4-2 Gasification can be done in either a low-temperature fluidized bed system (left) or a high-temperature entrained-flow gasifier (right).
SOURCE: Office of Biological and Environmental Research of the U.S. Department of Energy Office of Science.
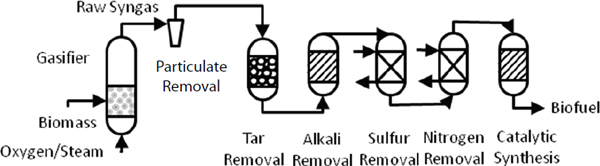
FIGURE 4-3 Removing impurities from biomass-generated syngas is a major challenge.
SOURCE: Office of Biological and Environmental Research of the U.S. Department of Energy Office of Science.
From a technical standpoint, the advantages of fast pyrolysis are that it occurs rapidly and at atmospheric pressure. It is a pathway to drop-in fuels or hydrocarbons, and it can produce multiple products. Commercially, fast pyrolysis offers the lowest-cost option for drop-in biofuels today, and bio-oil can be economically produced on a scale as small as 200 tons per day, which offers opportunities for distributed processing. Small facilities, located near the source of biomass, could produce bio-oil that would then be transported to a centralized facility just as is done with petroleum today.
The primary technical challenges facing fast pyrolysis, according to Brown, are that bio-oil is unstable, corrosive, and contains high levels of oxygen and water. Also, as he already mentioned, the fundamentals of pyrolysis are poorly understood. Commercially, there have been no demonstrations of bio-oil production and upgrading. Also, the pathway to finished fuels is still uncertain, though he remarked that the fact that there are many possibilities that have not yet been explored is what excites him as a researcher.
Other Pyrolysis Routes
Brown then briefly discussed two other types of pyrolysis—catalytic pyrolysis and solvolysis. Catalytic pyrolysis employs catalysts in the pyrolysis reactor or immediately downstream before bio-oil recovery to produce
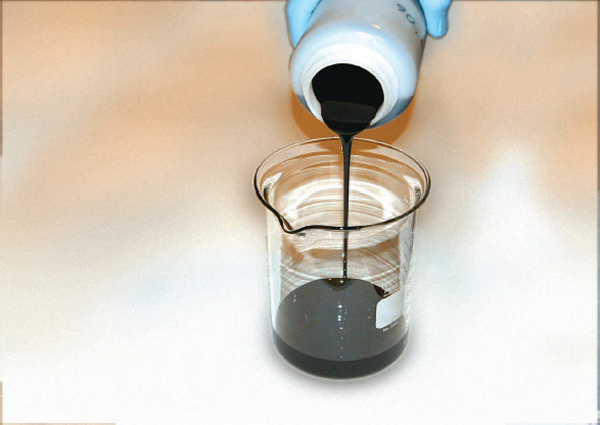
FIGURE 4-4 Bio-oil produced by fast pyrolysis of biomass.
SOURCE: Office of Biological and Environmental Research of the U.S. Department of Energy Office of Science.
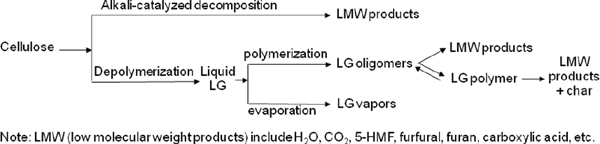
FIGURE 4-5 Pyrolysis chemistry.
SOURCE: Office of Biological and Environmental Research of the U.S. Department of Energy Office of Science.
highly reduced molecules that are more stable and more easily turned into fuels. Some researchers, he noted, have claimed that they have produced diesel fuel directly via catalytic pyrolysis, and while this represents a big advance, the product still needs some upgrading before it can be used as a fuel. The main challenge here is that yields are relatively low because of coking. Nonetheless, a large number of companies are exploring this approach even though the fundamental chemistry is not well understood.
Solvolysis is pyrolysis in a solvent, and it has two major manifestations. One involves direct liquefaction to produce a bio-crude that is similar to bio-oil but more deoxygenated. The other approach uses hydrothermal processing to produce sugar and a lignin-like product. The advantages and challenges with solvolysis are similar to fast pyrolysis with the added challenge of operating at high pressures. Brown noted that two start-up companies are working on commercial prototypes using solvolysis, one using direct liquefaction, the other hydrothermal processing.
Brown concluded his talk by briefly discussing various approaches for upgrading thermolytic substrates, and focused his remarks on the solubilized carbohydrates that can be produced. These are essentially highly concentrated solutions of sugars, typically greater than 20 weight-percent, which would have to be diluted to use for fermentation.
Discussion
Paul Bryan asked about the possibility of producing methanol as a pyrolysis product and using it as a fermentable intermediate. Brown replied that this is a good possibility, among probably a dozen others, that the market will need to assess. A participant asked if the high aromatic content of fast pyrolysis is a problem given that the fuel industry is moving
away from aromatics. Brown responded that aromatics are expected to be an important component of fuels for some time to come. They are particularly produced by catalytic pyrolysis using zeolites, but through further research alkanes might instead be produced.
Mark Barteau, from the University of Delaware, asked if co-processing of biomass with natural gas might be a way of shifting the carbon-hydrogen-oxygen ratio without using expensive hydrogen. Brown replied that with natural gas at the $2 level, this idea makes perfect sense and could provide a bridge to advanced drop-in biofuels. Helena Chum, from NREL, asked about the optimal scale for biomass processing, and Brown said that the issue of scale has to balance the fact that larger plants are probably offering economies of scale as far as process and capital costs are concerned, but that larger plants also have increased costs to transport biomass. He guessed that the optimal size for a plant that converted thermolytic substrates into transportation fuels would be somewhere in the 2000–3000 tons per day range although distributed processing facilities might be substantially smaller.
This breakout session was led by Douglass Elliott, of the Pacific Northwest National Laboratory, and the discussions began with the participants noting that there are a number of large-scale (greater than 500 tons per day of biomass) and pilot-scale (under 50 tons per day of biomass) demonstration projects, for both gasification and pyrolysis, either under construction or in the planning stage. The group acknowledged that gasification technology, while well proven with other feedstocks such as coal, may to struggle to compete with cheap, abundant, long-term supplies of natural gas. Having said that, many breakout group participants concurred that gasification is likely to be “omnivorous”; that is, it is likely to be adaptable for use with a wide variety of feedstocks. It was suggested, in fact, that while natural gas is the feedstock of choice today, it could serve as a bridge technology to biomass feedstocks in the future. Another suggestion was to integrate a corn stover gasifier into corn ethanol plants. Lignin and unconverted sugars could also be mixed into the corn stover for gasification. It was noted that such a plant is on the drawing board.
The breakout group discussed the use of gasification or natural gas to upgrade the bio-oil produced by pyrolysis and thought this was worth exploring. It was noted that Finland has been adding small pyrolysis chambers to existing fluidized bed combustion boilers. The pyrolysis unit uses some of the heat from the boiler system and in return feeds char and other byproducts of pyrolysis back into the boiler’s combustion chamber.
Turning to the subject of technological and commercial barriers, the breakout group noted that economic analyses and life cycle analyses are missing from most thermochemical conversion studies, particularly for combined systems. Some participants suggested that such studies be funded because there are many interesting concepts being developed today, but there is little thought being given to cost analysis. In the same vein, the breakout group described the need for better coupling of basic, translational, and applied research studies and noted that DOE could help fill that gap.
Another area of research that needs to be bolstered, some group participants noted, concerned the development of technologies to efficiently handle biomass solids of different characteristics and to determine how to best mix different biomass sources to produce bio-oil with more consistent properties. Some members of the breakout group also identified the need to develop methods for feeding biomass feedstocks into reactors at pressure, to design catalysts that are more tolerant of the poisons in biomass and of water and steam, and to perform separations at lower energy intensity. A basic research question that still needs answering, according to many breakout group members, was whether it might be best to process biomass as fractionated components rather than as the natural biocomposite that is lignocellulosic biomass.
The breakout group briefly discussed the belief that most efforts today focus too much on market “push” and not enough on market “pull.” In other words, the many members of the group noted, research was needed to identify products that could be made in an economically competitive manner from bio-oil or syngas. One approach to developing market pull that is being followed to some extent, the group noted, is to demonstrate how bio-oil or syngas would integrate seamlessly into existing petrochemical streams. Such efforts to develop market pull could help address the problem of attracting capital to this area.
In terms of skills needed, the breakout group said the main deficit in training in its opinion was the lack of interdisciplinary coursework and collaboration among engineering, chemistry, biology, and plant science investigators. Some breakout group members also noted they would like to see a greater emphasis in chemical engineering education on using carbon from biomass as opposed to just from petroleum.
Addressing the transportation infrastructure issue, the breakout group noted that there are far more questions than answers concerning how to best move biomass into a processing system and then move the direct products of biomass conversion into the secondary processing stream. Many members of the breakout group concurred that they would like to see the emphasis on using existing infrastructure and trying to make products that can be fit into today’s infrastructure at as early a point as possible.
This page intentionally left blank.