6—
Wetlands
OVERVIEW
Wetlands occupy a special position in restoration ecology, because they have been affected by so many disturbances and because they fall under regulations that require mitigation of future damages. Unlike lakes and streams, wetlands have not always had recognized value. In recent years, public attitudes have changed from a general disregard of wetlands to a widespread desire to protect and restore them. A major policy forum has recommended ''no-net-overall loss" and "net gain" in the quality and quantity of the nation's wetland resources (The Conservation Foundation, 1988). Thus, there have been numerous attempts to restore degraded wetlands, and there are many opinions about the status of wetland restoration.
The Bush administration has espoused the concept of no-net loss of wetland acreage and functioning. However, attempts to implement such a policy have proved difficult, because wetlands often stand in the way of development. Alaska wetlands were given special status (exemption) in the agreement to mitigate damages to wetlands (memo of agreement between EPA and COE, 1990). At present the area of protected wetlands may be reduced by modifying the delineation manual that is used to identify wetlands that are under the Clean Water Act of 1977 (P.L. 95-217), Section 404 jurisdiction. For example, seasonal wetlands would need to be wetter longer; peripheral areas would need to have vegetation classified as wetland
"obligates" (species confined to wetlands) not just "facultative" species (those that occur in both wetland and upland habitats). Obviously, if less of the wetland is under regulatory domain, development can continue without a net loss (in the legal sense).
Although the delineation of wetlands is outside the scope of this chapter, one question is central to the committee's charge: Can damaged wetlands be restored? If so, then restoring one wetland might compensate for damaging another. The answer often depends on how good the wetland science is. Determining whether a damaged wetland has been restored requires good information on wildlife, vegetation, soil, and hydrology.
This chapter discusses the functional values of wetlands and describes historic losses and damages. Current wetland restoration technology is summarized, along with constraints on achieving restoration goals, problems encountered during restoration, opportunities for major restoration projects, programs for wetland restoration, and reasons for varying opinions on the success of wetland restoration. Conclusions, recommendations, and research needs complete the chapter; however, recommendations on wetlands policy and institutional changes pertaining to wetlands are included in Chapter 8.
Definition of Wetlands
In the scientific view, wetlands are transitional areas between terrestrial and open-water systems. In the legal view, wetlands are discrete units subject to regulatory jurisdiction. The diversity of wetland types makes it difficult to have a single definition for a wetland.
According to the U.S. Fish and Wildlife Service (FWS), "wetlands are lands transitional between terrestrial and aquatic systems where the water table is usually at or near the surface or the land is covered by shallow water" (Cowardin et al., 1979). The FWS lists three attributes that help identify wetlands: the presence of hydrophytes, hydric soils, and saturated or inundated substrate. The temporal nature of some wetlands is acknowledged—hydrophytes and hydrologic indicators need only be present periodically. This definition is more inclusive than that used by the U.S. Environmental Protection Agency (EPA) and the U.S. Army Corps of Engineers (COE) (Clean Water Act, Section 404 (b)(1) guidelines) for regulatory purposes. The major federal agencies involved in wetland regulation have adopted a uniform manual for delineating wetland boundaries (Federal Inter-agency Committee for Wetland Delineation, 1989).
The diversity of wetland habitat types and the diversity of species they support are impressive. The classification system of Cowardin
et al. (1979) for U.S. wetlands includes 5 system types, 8 subsystems, 11 classes, 28 subclasses, and a large number of dominance types. Included within the category of wetlands are vegetation types that range from early colonizing (i.e., pioneer communities dominated by species such as cattails [Typha spp.]), to ancient, self-maintaining (i.e., old-growth, forested wetlands dominated by species such as bald cypress (Taxodium distichum) in the South and black spruce (Picea mariana) in the northern United States). The disturbances these systems have experienced likewise vary, as does the degree of restoration success.
The dynamic nature of wetlands also makes them ecologically complex. Along the edges of rivers, newly deposited sediments will be readily invaded by opportunistic plants and animals. Initial colonists are unlikely to be the same species as those of the floodplain forest that eventually develops. Along the edges of continents, mud flats are formed by alluvial outwash and are gradually colonized by salt marsh, grasses and succulents, which in turn trap sediments that raise the topography and attract additional plant and animal species. Along the edge of an acidic lake, sphagnum moss and herbaceous plants develop a mat that eventually supports bog shrubs and bog forest trees. In all these habitats, the nutrient content of the soil and the biomass of plants and animals increase through time, along with increases in species diversity and ecosystem complexity. The development of open substrates into persistent ecosystems is often called primary succession, a process that may occur over centuries or millennia. However, the process is not unidirectional, and Niering (1989) suggests that the term succession be replaced byvegetation development or biotic change to reflect the complex changes that ecosystems undergo in response to gradual and catastrophic events.
Historical Perspectives on Wetlands
Until the last two decades, wetlands were considered to be wastelands, having little productive use to society and no direct economic value to private landowners. They needed to be "reclaimed" through draining, ditching, diking, or filling to enhance their benefit to the public. Some federal, state, and local governmental policies actually provided incentives for destruction of wetlands. The purpose of the first "official" federal acts dealing with wetlands—the Swamp Lands Acts of 1849, 1850, 1860—was to convey to 15 states along the Mississippi River and to Oregon all swamp and overflow lands unfit for cultivation so that the states could reclaim the land for agriculture (adapted from Reitze, 1974). The drainage and destruction of wetlands
continued to be the accepted and often encouraged practice in the United States until the mid-1970s.
By the early to mid-1900s, negative impacts, such as declining waterfowl populations, were becoming apparent. The Fish and Wildlife Coordination Act of 1934, coupled with the U.S. Fish and Wildlife Wetlands Inventories of 1954 and 1973 (Reitze, 1974), prompted the realization that the loss of wetland habitat was causing a decline in fish and waterfowl populations. The public has begun to realize that wetlands are valuable systems providing many benefits to society. However, the conflict between private ownership of wetlands (and limited private benefits) and the desire to preserve social and economic values continues to contribute to the loss and degradation of wetlands.
Functional Value of Wetlands
Wetlands have properties of both aquatic and terrestrial ecosystems. Their most widely valued function is providing habitat for fish, birds, and other wildlife (Table 6.1), that is contributing to the maintenance of biodiversity (Table 6.2). In addition to this "food chain support" function, wetlands carry out hydrologic functions (e.g., flood-peak reduction, shoreline stabilization, ground water recharge) and water quality improvements (sediment accretion, nutrient uptake), all of which are recognized as valuable to society as a whole (Adamus and Stockwell, 1983). For individuals, wetlands provide recreational, educational, research, and aesthetic functions (see Table 6.1).
FOOD CHAIN SUPPORT
Although wetlands within the conterminous United States constitute only about 5 percent of the land surface (more than 40 million hectares, or about 104 million acres; Tiner, 1984; Dahl, 1990), many wetlands are among the most productive of natural ecosystems, exceeding the best agricultural lands and rivaling the production of tropical rain forests (Mitsch and Gosselink, 1986; Niering, 1986; The Conservation Foundation, 1988; CEQ, 1989). They provide habitat for a rich variety of native species. Riverine wetlands also serve as corridors for large, far-ranging species such as the Florida panther and black bear, as well as wetland-dependent species such as amphibians (Harris, 1988). More than one-third of the federally endangered and threatened plants and animals require wetland habitats during some portion of their life cycle (T. Muir, U.S. Fish and Wildlife Service, personal communication, June 1990).
TABLE 6.1 Wetland Functions
Flood conveyance—Riverine wetlands and adjacent floodplain lands often form natural floodways that convey floodwaters from upstream to downstream areas. |
Protection from storm waves and erosion—Coastal wetlands and inland wetlands adjoining larger lakes and rivers reduce the impact of storm tides and waves before they reach upland areas. |
Flood storage—Inland wetlands may store water during floods and slowly release it to downstream areas, lowering flood peaks. |
Sediment control—Wetlands reduce flood flows and the velocity of floodwaters, reducing erosion and causing floodwaters to release sediment. |
Habitat for fish and shellfish—Wetlands are important spawning and nursery areas and provide sources of nutrients for commercial and recreational fin and shellfish industries, particularly in coastal areas. |
Habitat for waterfowl and other wildlife—Both coastal and inland wetland provide essential breeding, nesting, feeding, and refuge habitats for many forms of waterfowl, other birds, mammals, and reptiles. |
Habitat for rare and endangered species—Almost 35 percent of all rare and endangered animal species either are located in wetland areas or are dependent on them, although wetlands constitute only about 5 percent of the nation's lands. |
Recreation—Wetlands serve as recreation sites for fishing, hunting, and observing wildlife. |
Source of water supply—Wetlands are becoming increasingly important as sources of ground and surface water with the growth of urban centers and dwindling ground and surface water supplies. |
Food production—Because of their high natural productivity, both tidal and inland wetlands have unrealized food production potential for harvesting of marsh vegetation and aquaculture. |
Timber production—Under proper management, forested wetlands are an important source of timber, despite the physical problems of timber removal. |
Preservation of historic, archaeological values—Some wetlands are of archaeological interest. Indian settlements were located in coastal and inland wetlands, which served as sources of fish and shellfish. |
Education and research—Tidal, coastal, and inland wetlands provide educational opportunities for nature observation and scientific study. |
Source of open space and contribution to aesthetic values—Both tidal and inland wetlands are areas of great diversity and beauty, and provide open space for recreational and visual enjoyment. |
Water quality improvement—Wetlands contribute to improving water quality by removing excess nutrients and many chemical contaminants. They are sometimes used in tertiary treatment of wastewater. |
SOURCE: Adapted from Kusler, 1983. |
TABLE 6.2 Wetland Attributes That Assist in the Maintenance of Biodiversity
• |
Persistence of habitat for mating, nesting, and protection from predators during extreme environmental conditions. |
• |
Resilience, the ability to recover from natural or human disturbances (e.g., environmental extremes, such as tidal closure and drought), often conferred through marsh soils. |
• |
Ability to maintain plant populations. Regions with high environmental variability need refuges for long-term maintenance of populations and to ensure resilience (ability to recover rapidly) following extreme events. |
• |
Resistance to invasive species (exotic to the region or alien to the habitat). The continual threats of disturbance to topography and hydrology lead to the need for constructed wetlands to resist invasive species. |
• |
Ability to support nutrient transformations (microbial and chemical processes controlling the concentrations of nutrients and other compounds and faciliting the biogeochemical cycling of nutrients and the flow of energy). Nutrient transformations are not well known for all wetland types. Plant productivity of freshwater marshes is often phosphorus limited, whereas that of coastal marshes is often nitrogen limited; thus, these elements have been the focus of most assesments of nutrient dynamics. In coastal wetlands, the nitrogen dynamics are very important; both fixation and denitrification rates are linked to availability of organic matter in the soil. |
Wetland production is important to both aquatic and terrestrial food webs, as summarized by the Council on Environmental Quality (CEQ, 1989):
Wetlands provide cover, freedom from disturbance, food, and other vital habitat factors. It is estimated that over one-half of all the saltwater fish and shellfish harvested annually in the United States, and most of the freshwater game fish, use wetlands for feeding areas, spawning grounds, and nurseries for young. About one-third of the North American bird species are wetland associates. In addition to supporting resident birds year-round, wetlands are important breeding grounds, overwintering areas, and feeding areas for migratory birds, particularly waterfowl. Of the 10 to 20 million waterfowl that nest in the conterminous 48 United States, 50 percent or more reproduce in the Prairie Pothole wetlands of the Midwest. Bald eagles, ospreys, hawks, egrets, herons, kingfishers, and a variety of shore, marsh, and passerine birds are other components of the wetland avifauna.
Wetland-dependent mammals include muskrats, beaver, marsh rice rats, and swamp rabbits, and otter, mink, raccoon, bobcat, meadow mouse, moose, and white-tailed deer use wetlands as feeding areas.
Our knowledge of how food webs are modified as wetland habitat diminishes is not extensive, nor is our understanding of how trophic
structure responds to declines in predatory species, such as the bald eagle and peregrine falcon. The native food web is no doubt essential to the maintenance of community structure. Power (1990) studied one stream system and demonstrated that communities with and without fish have contrasting structures: Where fish are absent, smaller predators increase in abundance and reduce the numbers of chironomids (midge larvae); thus algae are released from chironomid grazing and they develop a tall, thick turf. In the presence of fish, each trophic level reverses in abundance; the fish reduce numbers of smaller predators, so chironomids increase and in turn consume the algal turf, reducing it to a prostrate form. Elsewhere, introductions of exotic animals are known to have caused major changes to the wetland ecosystem (e.g., nutria alter plant successional processes and ecosystem structure in Louisiana coastal marshes; M. Rejmanek, University of California-Davis, personal communication, September 1990). The introduction of foreign plants can lead to vegetation growth that "swamps" native food chains (e.g., water hyacinths clog southern waterways). Until food chain functions are well understood, restoration projects will be jeopardized by the inability to ensure the reestablishment of critical links.
HYDROLOGIC FUNCTIONS
Their position in the landscape, whether as isolated wetlands or floodplains contiquous with rivers and streams, gives wetlands a major role in storage of floodwater and abatement of flooding. Wetlands intercept storm runoff and release floodwaters gradually to downstream systems. Because it is usually the peak flows that contribute to flood damage, wetlands reduce the impact of flooding (Novitzki, 1979). When wetlands are converted to systems that are intolerant of flooding (drained agricultural lands, filled developed land), their storage capacity decreases and downstream flooding occurs.
The cost of lost flood storage and abatement functions is substantial, and it is borne almost exclusively by taxpayers. Riverine wetlands along the Charles River in Massachusetts were deemed effective in protecting Boston from flooding, and purchasing them was less expensive than building flood control structures (U.S. Army Corps of Engineers, 1972). In what is now a classic study of wetland hydrologic values, the COE determined that losing 3,400 ha of wetlands in the Charles River basin would increase flood damage by $17 million per year (equivalent to $5,000 /ha per year, or about $2,000 per acre per year). That such flood protection values are real is supported by experiences where flood protective functions have been lost. Along
the Mississippi River, constructing levees and draining the floodplain have reduced floodwater storage from an estimated 60 days to 12 days (Gosselink et al., 1981) because waters can no longer spread out and be absorbed by the broad floodplain. The result has been annually recurring floods along the lower Mississippi River; the costs include flood damages and construction of extensive structures to abate flooding.
WATER QUALITY IMPROVEMENT FUNCTIONS
The value of wetlands for improving water quality is often overlooked, yet wetlands can remove and transform both organic and inorganic materials—including human waste, toxic compounds, and metals—from inflowing waters (Tuschall, 1981; Best et la., 1982; Best, 1987). Wetland attributes that make them effective in improving water quality include the following (adapted from Mitsch and Gosselink, 1986):
-
As water floods into wetlands from rivers and streams, its velocity decreases, causing an increase in sedimentation. Thus, chemicals sorbed to sediments are removed from the water and deposited in the wetlands.
-
A variety of anaerobic and aerobic processes function to precipitate or volatilize certain chemicals from the water column.
-
The accumulation of organic peat that is characteristic of many wetlands can ultimately lead to a permanent sink for many chemicals.
-
The high rate of productivity of many wetlands can lead to high rates of mineral uptake by, and accumulation in, plant material with subsequent burial in sediments.
-
Shallow water coupled with the presence of emergent vegetation leads to significant sediment-plant-water exchange.
HUMAN VALUES
As discussed above, wetlands play an active part in hydrologic functions, water quality improvement, and food chain support functions that serve human needs. Because of their importance in floodpeak reduction, shoreline stabilization, ground water recharge, sediment accretion, nutrient removal, toxic material removal, and support of commercially important fish, shellfish, ducks, and geese, wetlands have received special protection under federal and state laws and many local ordinances. Wetlands serve a number of purposes that
translate into economic values through reduction in flood and storm damage, conservation of water supplies, treatment of wastewater, and production of food.
Moreover, wetland recreation often leads to private profits. Consider, for example, anglers who buy special gear and clothing; the growing numbers of bird watchers who purchase books, cameras, and binoculars; the publication of sport and wildlife magazines; and the tourism that is generated by aquatic reserves and a new generation of visitor centers in places as unlikely as wetlands constructed to treat urban wastewater (e.g., Arcata, California). Habitats that provide opportunities for research and education contribute additional human values, with increasing numbers of programs for field experiences at the elementary and high school levels, as well as college and graduate course work.
Because wetlands are extremely valuable natural resources, their degradation or loss results in real costs to society. As Dahl (1990) concluded, "Environmental and even socioeconomic benefits (i.e., ground water supply and water quality, shoreline erosion, floodwater storage and trapping of sediments, and climatic changes) are now seriously threatened." However, these values are principally societal values, whereas private wetland owners receive few direct economic benefits from wetlands—and the ownership of wetlands is largely private. Of the acres of wetlands that remain in the United States, almost three-fourths (74 percent) are privately owned (CEQ, 1989).
Restoring damaged wetlands should be a high priority, now that wetlands are recognized as valuable environmental and socioeconomic systems. However, restoration is often very expensive—with estimates as high as $10 million to $50 million for a small (260-acre), urban wetland in Los Angeles, depending on the degree of restoration selected. Restoring farmlands to wetlands may be inexpensive and easier to accomplish. Whereas the costs of wetland draining and filling were borne largely by private owners seeking to achieve a direct personal increase in economic benefits, the restoration of wetlands will be borne almost entirely by the public. Exceptions are wetlands restored within the regulatory process: landowners who disturb or destroy existing wetlands often propose to mitigate the damages by restoring or creating degraded wetlands.
King (1990) has begun an analysis of the cost effectiveness relationship for wetland restoration projects. His approach is to model combinations of tasks that will speed wetland restoration (e.g., site contouring, vegetation planting, soil augmentation, control of exotic species) and the degree of functional equivalency achieved with each
additional task and cost. His intent is to determine the point at which it is no longer economical to spend more on a project (i.e., when the additional ecological benefits would be minimal). A major shortcoming of his analysis is, of course, lack of data on functional equivalency. For the San Diego Bay project (Box 6.1), the use of 11 measured values suggested that, at the age of 5 years, the constructed wetland had less than 60 percent of the functional equivalency of reference wetlands. A second data point (obtained at perhaps 10 years) is needed to determine if site development has leveled off or if the site can eventually achieve great similarity with natural wetland functioning.
A second major shortcoming of cost-benefit analyses in general is the inability to put dollar values on ecosystem attributes. Although one can estimate the cost of many of the human values described above, one can never predict all that might be derived from wetland restoration. An endangered plant that might be rescued by a marsh restoration project may some day be found to produce an important pharmacological chemical; restoration of a coastal wetland may prevent real estate damage should sea level rise at unexpected rates; wetland plants may become horticulturally or agriculturally important (Glenn et al., 1991); a habitat-dependent bird may be shown to be effective in controlling mosquito and malarial outbreaks; the presence of open space may be shown to be essential to mental health.
LOSS OF WETLANDS
Trends in historical losses of wetlands in the United States were recently summarized in a report to Congress (Dahl, 1990):
At the time of Colonial America, the area that now constitutes the 50 United States contained an estimated 392 million acres (about 160 million hectares) of wetlands…. Over a period of 200 years, the lower 48 states lost an estimated 53 percent of their original wetlands…. On average, this means that the lower 48 states have lost over 60 acres (about 25 hectares) of wetlands for every hour between the 1780's and 1980's [emphasis added].
By the 1980s, wetlands constituted only 5 percent of the landscape, down from an original 11 percent. The distribution and abundance of wetlands have also changed significantly since the 1780s (Figure 6.1). The midwestern farm belt states of Illinois, Indiana, Iowa, Michigan, Minnesota, Ohio, and Wisconsin lost more than 36 million acres (about 15 million hectares) of wetlands—roughly one-third of all wetlands lost in the history of our nation. All states, except for Alaska, Hawaii,
BOX 6.1 SWEETWATER MARSH NATIONAL WILDLIFE REFUGE, SAN DIEGO BAY, CALIFORNIA Southern California's best-studied wetland restoration site is in the Sweetwater Marsh National Wildlife Refuge, which includes 128 ha of wetlands (mostly intertidal salt marsh) and some uplands along the eastern side of San Diego Bay, California (32°38´N, 117°6´W). The site and the restoration project are both significant—the wetland provides habitat for endangered species and thus is critical for maintaining regional biodiversity; the project has exceptionally high criteria for judging success and thus serves as a model for future restorations. Protection of the site and strict standards for restoration came about only after a lengthy court battle. The new refuge was designated after a federal district court (Thompson, 1988) settled a lawsuit filed by the Sierra Club and the League for Coastal Protection against three federal agencies. Wetland habitat had been damaged by construction of a wider freeway, a new freeway interchange, and a flood control channel. Endangered species had been jeopardized, and mitigation measures had not been implemented. The lawsuit also led to reinitiation of consultations and a new biological opinion by the U.S. Fish and Wildlife Service (1988), which included strict criteria for successful mitigation. The requirements were expanded to include functional wetlands that would support persistent populations of three endangered species, the lightfooted clapper rail (Rallus longirostris levipes) , the California least tern (Sterna antillarum browni), and the salt marsh bird's beak (Cordylanthus maritimus ssp. maritimus). The current shoreline of San Diego Bay bears little resemblance to what was once the natural landscape. The bay entrance to Paradise Creek marsh has been filled, and tidal flushing has been rerouted through a channel dredged straight south to the Sweetwater River. A railroad and Interstate 5 cross the landward edge of the refuge and wetlands. The alterations preceding the restoration/mitigation project included widening of Interstate 5, construction of a new freeway interchange, and excavation of a new flood control channel through existing wetland. Restoration began in fall 1984 with the excavation of about 4.9 ha of disturbed upper intertidal marsh, including areas previously used as an urban dump. Eight lower intertidal islands and adjacent channels |
were constructed in fall 1984 and planted with cordgrass (Spartina foliosa) in winter 1985. The goal (DeWald and Rieger, 1982) was to create nesting habitat for the light-footed clapper rail, and foraging habitat for the California least tern, which commonly nests on the nearby dredge spoil. Cordgrass plants that would have been destroyed by construction were salvaged from Paradise Creek and placed in a small (0.1 ha or 0.23 acre) intertidal nursery that was constructed to hold and propagate cordgrass. Additional cordgrass plants and other species were moved to pots for propagation off-site. The California Department of Transportation (Caltrans) used the advice of salt marsh ecologists to design habitats with the appropriate intertidal elevations. The island configuration was Caltrans's attempt to accommodate a large area of channels and cordgrass marsh, as well as higher marsh refuges, all within a small, linear site (of approximately 5 ha). Cordgrass was transplanted at 6- and 3-ft intervals in early 1985, with survival somewhat reduced by rough handling of potted plants (Swift, 1988). Plants were fertilized with urea four times during 1985–1986 (H. Hunt, Caltrans, personal communication, June 1990). After settlement of the lawsuit, Caltrans began a monitoring program to assess plant cover and faunal use. They also funded a propagation research program to improve transplantation methods and to develop techniques for establishing the endangered salt marsh bird's beak, which is a hemiparasitic annual plant. Comparisons of constructed and natural wetland functioning were initiated in 1987 (after three growing seasons for the marsh transplants), with research funding from the California Sea Grant College and monitoring funds from Caltrans (Cantilli, 1989; Rutherford, 1989; Zalejko, 1989; Langis et al., 1991; Zedler, 1991; Zedler and Langis, 1991). Paradise Creek was selected as a reference system because part of that wetland had been filled, and it represented the lost habitat. In addition, it was hydrologically connected to the northern four islands of the restoration site. Three wetland functions were evaluated in the lower-marsh cordgrass habitat: 1. Food base for top carnivores. Rutherford (1989) found differences in epibenthic invertebrates based on quarterly sampling, |
mostly in year 4 of the marsh development. The constructed marsh averaged only one-third as many individuals as were present in Paradise Creek marsh. The seven most abundant invertebrate taxa were a fly larva of the genus Pericoma, capitellid polychaetes, the isopod Ligia , the amphipod Orchestia, a small snail Assiminea, a midge, and a Grapsid crab. A native anemone (Diadumene franciscana) was common in the natural marsh but rare in the constructed marsh. Its functional role is unknown. An exotic mussel (Musculista senhousia) was more common in the constructed marsh than in the natural marsh. (Sacco, 1989, also found similar species but lower densities of infaunal invertebrates (mostly oligochaete and polychaete worms) in cordgrass marshes of North Carolina. The presence of less soil organic matter was suggested as a reason for the low density.) 2. Plant growth. Clapper rails prefer dense cover of tall cordgrass (Jorgensen, 1975), which protects them and their nests from aerial predators. Although transplants expanded their cover between 1987 (after three growing seasons; Swift, 1988) and 1989 (year 5; Pacific Estuarine Research Laboratory [PERL], unpublished data), biomass and plant height were not equivalent in the constructed and natural marshes. Aboveground biomass was about one-half as great (Langis et al., 1991), and plants were about 20 cm shorter in the constructed marsh in July 1988 (PERL, unpublished data). Shorter cordgrass provides poorer cover and lacks the vertical refuge that many marsh insects require at high tide. Debris deposition, erosion and accretion, and poor handling of transplants explain the delayed vegetative expansion of cordgrass, but lower biomass and height are more likely due to differences in nitrogen (Covin and Zedler, 1988). Foliar nitrogen concentrations were 16 percent lower in the constructed marsh than in the natural marsh, whereas phosphorus concentrations were similar (Langis et al., 1991). 3. Nitrogen supply function. Nitrogen-fixation rates were lower on the soil surface and were limited by low concentrations of soil organic matter (Zalejko, 1989). Soil nitrogen concentrations were lower in the constructed marsh, with less |
than one-third as much in the sediment and about one-tenth as much in the pore water (Langis et al., 1991). (Others have also found lower concentrations of nutrients in constructed salt marsh soils in North Carolina [Craft et al., 1988] and New Jersey [Shisler and Charette, 1984].) At San Diego Bay, soil nitrogen concentrations did not increase during the 2-year study (Langis et al., 1991); thus it is hard to predict when or if the site will be functionally equivalent to the reference wetland. Reasons for low concentrations of organic matter in the soil and for low concentrations of nutrients are related to the site's history; the sandy substrate was part of the alluvial outwash of Sweetwater River. To suggest an overall comparison, Zedler and Langis (PERL, 1990) presented a ''functional equivalency index" based on 11 marsh attributes that indicated less than 60 percent equivalency when the marsh was 4 to 5 years of age. For each attribute, the mean value for the constructed marsh was expressed as a percentage of the mean value for the reference wetland (organic matter content, 51 percent; sediment inorganic nitrogen, 45 percent; sediment nitrogen total Kjeldahl nitrogen (TKN), 52 percent; pore-water inorganic nitrogen, 17 percent; nitrogen fixation (surface centimeters), 51 percent; nitrogen fixation (rhizosphere), 110 percent; biomass of vascular plants, 42 percent; foliar nitrogen concentration, 84 percent; height of vascular plants, 65 percent; epibenthic invertebrate numbers, 36 percent; epibenthic invertebrate species lists, 78 percent). Although cordgrass cover in the reconstructed marsh is expanding to fill in bare areas, there is no evidence that nutrient conditions are improving. It is not yet possible to predict if or when plant heights and biomass will be equivalent to those of the reference wetland. Because a disturbed high-marsh wetland was excavated to construct the cordgrass marsh, there was a net loss of acreage. Because the disturbed site was not studied, and because there are few data on the role of higher marsh habitats, those functional losses will go unrecorded. |
and New Hampshire, have lost more than 20 percent of their original wetland acreage. The highest percentage of loss, 91 percent, has taken place in California, where only 454,000 acres (ca. 183,700 ha) remain, while the highest acreage loss, approximately 9,286,713 acres (ca. 3,759,800 ha) has occurred in Florida (a 46 percent loss; Figure 6.1).
Damages to Wetlands
Alterations to wetlands fall in three sometimes overlapping categories—biological, chemical, and physical (Table 6.3). The biological alterations result from management to maximize one or a few specific wetland values, from harvesting or removal of natural vegetation or animals, and from introduction of nonnative plants and animals. Like lakes and streams, wetlands are also subject to chemical alterations through point and nonpoint nutrient runoff from surrounding uplands; discharge of toxic, hazardous, or other chemical waste; and increased nutrient loadings.
For wetlands, the most destructive alteration has been physical, often eliminating the topography and hydrology that supports the wetland ecosystem. The most significant historical loss of wetlands has resulted from agricultural practices (87 percent; Tiner, 1984), with most due to wetland drainage (Figure 6.2). The construction of dams and the dredging of river, stream, and coastal waterways eliminate wetlands at the project site and also affect downstream systems. The stabilization of water levels for rivers or lakes eliminates the vital pulsing function that flooding provides, thus interrupting nutrient and sediment delivery (Loucks, 1989). The natural maintenance and expansion of wetlands often depend on sedimentation events, which are interrupted by dams (long-term reduction in sediment load) and dredging projects (short-term increase in sediment release). In urban areas, filling has accounted for significant wetland loss. This continues to be the case where restoration of one wetland is proposed as mitigation for additional development of other wetlands (Fonseca et al., 1988; Zedler, 1988a). In most physical alterations of the habitat, the wetland ecosystem is obliterated, and there is little opportunity for restoration once the habitat has been flattened or replaced by urban development, reservoirs, ports, or marinas.
Biological, chemical, and physical alterations often occur together, and the result is a cumulative impact that may well exceed the "sum" of the individual disturbances. A wetland that has been bulldozed to remove vegetation would recover more rapidly and more completely if it were not also contaminated with toxic materials or affected by hydrologic alterations. In addition, the alteration of one wetland
TABLE 6.3 Types of Alterations to Wetlands
Biological |
|
• |
Grazing—consumption and compaction of vegetation by either domestic or wild animals |
• |
Disrupting natural populations |
Chemical |
|
• |
Changing nutrient levels—increasing or decreasing levels of nutrients within the local water or soil system; forcing changes in the wetland plant community |
• |
Introducing toxics—adding toxic compounds to a wetland either intentionally e.g., herbicide treatment to reduce vegetation) or unintentionally, adversely affecting wetland plants and animals |
Physical |
|
• |
Filling—adding any material to change the bottom level of a wetland or to replace the wetland with dry land |
• |
Draining—removing the water from a wetland by ditching, tiling, pumping, and so on |
• |
Excavating—dredging and removing soil and vegetation from a wetland |
• |
Diverting water away—preventing the flow of water into a wetland by removing water upstream, lowering lake levels, or lowering ground water tables |
• |
Clearing—removing vegetation by burning, digging, application of herbicides, scraping, mowing, or otherwise cutting |
• |
Flooding—raising water levels, either behind dams or by pumping or otherwise channeling water into a wetland. |
• |
Diverting or withholding sediment—trapping sediment through construction of dams, channelization, or other types of projects, thereby inhibiting the regeneration of wetlands in natural areas of deposition such as deltas |
• |
Shading—placing pile-supported platforms or bridges over wetlands, causing vegetation to die |
• |
Conducting activities in adjacent areas—disrupting the interactions between wetlands and adjacent land areas, or incidentally impacting wetlands through activities at adjoining sites |
SOURCE: Reprinted, by permission, from World Wildlife Fund and The Conservation Foundation, 1988. Copyright © by The Conservation Foundation, Washington, D.C. |
affects the condition of neighboring wetlands. If an exotic plant gains a foothold in one disturbed system, its seeds are more likely to disperse to nearby wetlands, increasing chances of spread into less disturbed systems. Changes in hydrologic regimes, such as those brought on by ground water depletion from wells or by dams, levees, or drainage, can effectively eliminate wetland characteristics and the values associated with those characteristics. The cumulative effect of both local and regional disturbances is reduced potential for wetland restoration.
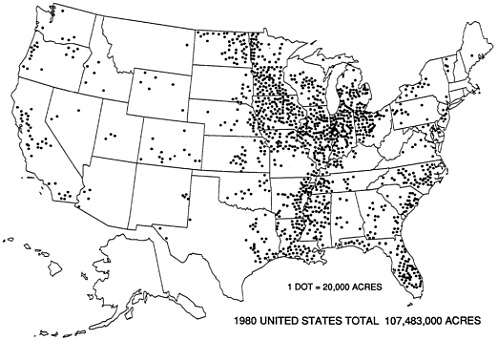
FIGURE 6.2 Extent and location of artificially drained agricultural land in the United States in 1985. Data courtesy of U.S. Department of Agriculture Economic Research Service. Source: Dahl, 1990.
Effects of Cumulative Impacts
Recently, it has become apparent that the cumulative impact of many individual actions, no single one of which is particularly alarming, threatens the integrity of entire wetland landscapes. Cumulative impact is defined in the Council on Environmental Quality regulations as "the impact on the environment which results from the incremental impact of the action when added to other past, present, and reasonably foreseeable future actions regardless of what agency (federal or nonfederal) or person undertakes such other actions. Cumulative impacts can result from individually minor but collectively significant actions taking place over a period of time" (40 C.F.R. 1508.7 and 1508.8; Gosselink et al., 1990a).
Although the Clean Water Act and regulations for implementation of its Section 404 by both EPA (44 C.F.R. 230) and COE (33 C.F.R. 320-30) require consideration of cumulative impacts, they are seldom evaluated in permit review processes (Gosselink et al., 1990a). The Section 404 permit process focuses on the impact of a proposed activity at an individual wetland permit site. In contrast, cumulative
impacts are landscape-level phenomena that result from decisions at many individual permit sites, as well as activities that are not regulated under Section 404 (Gosselink and Lee, 1989).
Conversion of a wetland forest to agricultural use results in a typical cumulative impact (Gosselink et al., 1990b). Historically, the incremental clearing of 10 ha to as much as 2,000 ha in an individual permit has been perceived to have no significant ecological impact on a total forest system of several million hectares, and the cumulative effect of many such permitted activities has been ignored (Gosselink et al., 1990b). Currently no methodology for cumulative impact assessment is generally accepted by scientists and managers (Gosselink et al., 1990a). Furthermore, wetland restoration has generally been undertaken on an ad hoc basis, and the potential role of restoration in a cumulative context or on a landscape scale is not usually considered.
Gosselink and Lee (1989) described a methodology for cumulative impact assessment and management that incorporates a process of ecological characterization, goal setting, and planning. The method also focuses attention on the landscape level and bases planning on landscape ecology principles. Researchers used the Tensas River basin, an area of approximately 1 million hectares in northeastern Louisiana, as a case study to test this general approach to cumulative impact assessment and management (Gosselink et al., 1990a).
Potential Impacts of Global Climate Change and Sea Level Rise
Most ecosystems will be affected by global warming over the next century, and planning for restoration of self-sustaining ecosystems must consider the potential impact of climate change. The problem is predicting how the climate will change at specific locations (will it get wetter, drier, warmer, or cooler?) and what measures might offset any negative impacts of changing weather and hydrology. For biodiversity reserves, Peters (1988) has suggested that a number of management actions may be needed to prevent species extinctions as climate changes. For example, much larger reserves may be necessary to perpetuate populations, so that heterogeneous topography and soils are included, thus protecting a wider range of habitat types (and presumably also ecotypes). We may need to plan to control environmental conditions in reserves (e.g., through irrigation or drainage). Control of predators and exotic species may become more critical. Peters (1988) also suggests that creating reserves outside the normal climatic regions of an ecosystem type may be needed. Finally, he mentions the possibility of moving reserves as the climate changes,
depending on the ability of restoration ecolgy to reconstruct communities in new locations.
For coastal wetlands, the future is more easily predicted, and management recommendations are clearer. Global warming will result in a rise in sea level, with the effect that existing coastal wetlands will be flooded, salt marshes will be lost and species will be unable to "migrate" inland because of urbanized or otherwise developed landscapes. Because the rate of rise will accelerate, not all species will be able to move inland fast enough to keep up with the rate of loss at lower elevations (Park et al., 1989) Thus, species diversity may decline along with the area of each wetland plant community. Park et al. (1989) have calculated that a 1-m rise in sea level would result in the loss of 65 percent (6,441 square miles) of the coastal marshes in the contiguous United States. Such an increase would result from an average temperature increases of 3 ° C and is probable by the year 2100 (Park et al., 1989). A global warming of 6 ° C could result in a 2.3-m rise and a 77 percent (7,423 square miles) loss of the coastal wetlands of the contiguous United States (Park et al., 1988). The specific predictions stemming from a 1-m rise in sea level are summarized by Park et al. (1989, p. 78):
Some sites, such as Charleston, South Carolina, are well buffered for sea level changes up to one meter, due to high tidal ranges and high sedimentation and accretion rates. Other sites, especially in the Gulf of Mexico, are quite vulnerable to small changes in sea level. Although the model is perhaps too simple for representing complex deltaic dynamics it projects a continuation of current trends for the Louisiana coast south of New Orleans, with the entire undiked area shown to be at risk without any acceleration in sea level rise. With protection of all existing residential and commercial developments, by the year 2100 salt marshes and freshwater swamps would gradually decline in area, mangrove swamps would gradually increase, and freshwater marshes would gradually decline until a sea level increase of 0.8 m is reached. Freshwater marshes would then disappear rapidly (reflecting a pattern seen in both the Gulf and Mid-Atlantic Coasts); 35 percent of vegetated wetlands would be lost with a 0.5-m rise, 49 percent with a 1-m rise, 56 percent with a 2-m rise, and 68 percent (almost 9,000 mi2) with a 3-m rise.
Several recommendations for restoration of coastal wetlands follow from the above predictions for a more rapidly rising sea level. The first is that a broad margin or buffer needs to be preserved adjacent to the restoration site, so that high marsh communities will have space to migrate inland. An additional consideration is that changes in water levels may occur at a rate too rapid for marsh flora and fauna to
colonize buffer areas, unless these areas are aided by restorationcreation activities. The second recommendation is that long-term management will need to include censuses of species composition across elevation gradients that take note of changing abundances and disappearance of species. Because restoration sites may lack some of the resilience of natural marsh communities (i.e., persistent seed banks, highly organic soils to ameliorate changes in soil moisture, ability to resist exotic plant invasions), constructed wetlands may be more susceptible to rising sea level than their model ecosystems. Comparative long-term censuses of species abundances are needed to ensure that biodiversity is conserved.
RESTORATION OPPORTUNITIES
Wetland Settings and Their Effects
Opportunities and requirements for restoring wetlands differ depending on whether the wetland occurs in a coastal-estuarine, riverine, depressional, or large-scale freshwater setting.
COASTAL-ESTUARINE WETLANDS
Coastal-estuarine wetlands are characterized by the rise and fall of the tides and resulting salinity gradients, which determine, in large measure, the distribution of plant and animal species. Coastal and estuarine wetlands have been diked, filled (in some areas), and ditched for mosquito control or other purposes. Most coastal wetland restoration efforts have involved either the breaching of dikes to allow the reentry of tides or the restoration of vegetation (mangroves, Spartina alterniflora) on denuded areas after development projects. In some instances, fills have been removed, primarily as the result of regulatory violations and subsequent enforcement actions. Canals have also been filled to restore coastal or estuarine wetlands, and sediment diversions are proposed for the lower Mississippi.
Most of the wetland restoration and creation literature concerns coastal and estuarine wetlands. Perhaps the greatest success in at least restoring look-alike vegetation is with coastal and estuarine marshes. This success is due not only to the breadth of experience to date but also to the relative ease of determining appropriate elevations by using tide records and elevations and adjacent reference sites. In general, fewer wetland plant species occur in these systems (due to the need for salt tolerance) than in comparable freshwater
systems. However, the habitat value of restored coastal wetlands is not fully documented.
STREAM AND RIVERINE WETLANDS
Stream and riverine wetlands are often severely altered. Not only have rivers been the depository for most liquid pollutants, but their hydrologic regimes have also been altered by dams, pumping, dikes, channelization, dredging, bank stabilization, and watershed development. Wetlands in headwater areas, in oxbows, and in low-velocity channels have not been extensively restored, although rather largescale floodplain forest restoration programs are proposed for areas in the lower Mississippi. Many small-scale restoration projects have been undertaken as part of local greenway and stream restoration programs such as the Urban Streams program in California.
Efforts to restore riverine wetlands are complicated by the hydrologic and sediment regime changes typical of most rivers, which make it impossible to return wetlands to their natural condition without massive removal of dams, channelization, and so on. Nevertheless, these wetlands are increasingly recognized as having great value for water quality protection, fish and wildlife habitat, flood control, and bank stabilization.
DEPRESSIONAL WETLANDS
Many small, depressional wetlands were formed by glaciers 9,000 to 12,000 years ago. Hundreds of thousands of these wetlands exist in the northern tier of states (prairie potholes). Other depressional wetlands were created by solution (karst topography), the wind (sand hills of Nebraska), or other processes (Carolina bays of the South-east). These wetlands have not, in most states, been modified as substantially as have riverine wetlands. However, many have been drained or are used during dry years for agriculture. Others have been used as landfills or filled for urban development.
Except for efforts to remove drainage tiles and restore natural drainages in the prairie pothole region, few efforts have been made to restore such depressional wetlands. Restoration efforts may be quite inexpensive if cessation of agriculture or blockage of drainage ditches is the primary activity. However, restoration is potentially expensive where fill must be removed or where extensive removal of drainage tiles is involved.
LARGE FRESHWATER WETLANDS WITH POOR DRAINAGE
Many of the larger, altered wetland complexes consist of relatively flat, poorly drained lands. Examples include former lake bottoms formed during glacial periods (e.g., lands adjacent to the Great Lakes), wide floodplains or deltas (lower Mississippi), coastal areas of low relief (e.g., pocosins and pine flatwoods of the Southeast), the Florida Everglades, and alluvium in front mountain ranges. Many of these lands have been at least partially drained for agricultural purposes. However, the wetland soils and hydrology are otherwise largely intact.
These lands constitute the largest area of potential wetland restoration sites, not only because of their large acreage but also because wetland restoration may be less expensive and may be achieved through the filling of drainage ditches or installation of minor water-control structures. Land values may also be relatively low, particularly where such lands are no longer being used for agriculture and there are no alternative uses.
RESTORATION OF FORMER WETLANDS IN AGRICULTURAL USE
More than 80 percent of historic wetland loss in the 48 conterminous states has been due to agricultural conversions. Such conversions have involved clearing of the natural forested or herbaceous wetland vegetation, introduction of drainage, and flood control. Federally funded agricultural flood control and drainage projects have facilitated private conversions. In arid parts of the country, massive federally funded irrigation projects have promoted conversions of wetlands to agriculture.
Any national program designed to restore millions of acres of former wetlands will have to focus primarily on wetlands converted to agricultural use. In many cases, the technical problems of reconverting such agricultural lands to functioning wetland systems are not as challenging as those encountered in the urban context, where the physical components of natural wetlands have been severely altered by chemical pollution, fill, barriers to water movement, and vast changes in the watershed. Former wetlands now in agricultural use can typically be reestablished by cessation of planting crops or domestic animal foraging, breaking drainage tiles, filling in drainage ditches, and if feasible, removing flood control structures. In the prairie pothole region of the country, particularly in Minnesota, the U.S. Fish and Wildlife Service, the Soil Conservation Service, and the state of Minnesota have achieved partial restoration of thousands of acres of prairie
potholes in this manner. Wetland vegetation has quickly reappeared, and ducks have returned in profusion (see Prairie Pothole case study, Appendix A). However, marsh vegetation may not replicate the historic community, and some animals have not returned. In small-scale restorations of the lower Mississippi valley, forested wetlands are being reestablished themselves on the alluvial soils that retain moisture for long periods following precipitation, even where federal flood control levees and channels block riverine overbank flooding. However, these small-scale wetland restorations are not often subject to rigorous scientific evaluation.
The Swampbuster program of the 1985 Food Security Act, as amended in the 1990 Farm Act, changes in the tax treatment of agricultural drainage in the Tax Reform Act 1986 (P.L. 99-514), and the Clean Water Act Section 404 program, as well as lower grain prices, have reduced significantly the rate of agricultural conversions of wetlands since the early 1980s. Yet the rate of restoration has been very slow. The major impediments are more often legal, institutional, and financial rather than technical. Former wetlands now in agricultural use are almost all in private ownership, and restoration can occur only when financial arrangements with landowners are available to promote reconversion. In some cases, federal flood control projects will have to be modified. The small-scale restorations described above have occured in the last 3 to 4 years because such programs have become available.
Small-scale wetland restorations have occured in the last few years as a result of foreclosures on former wetlands by the Farmers Home Administration (FmHA) and the Resolution Trust Corporation (RTC) in response to the bankruptcies of farms and savings and loan institutions, respectively. The U.S. Department of Agriculture (USDA) and the Resolution Trust Corporation have an arrangement with the U.S. Fish and Wildlife Service to assess these lands for their value as wetland or other wildlife habitat before they are auctioned, resold, or in the case of some FmHA foreclosures, returned to their original owners, typically at bargain prices. In some cases, the U.S. government through the FWS has retained title to these former wetlands that are now being restored.
In the 1990 Farm Act, Congress established, for the first time, a significant wetland restoration program. Known as the Agricultural Wetland Reserve Program (AWRP), it provides that, of the remaining 6 million acres of cropland eligible for the Conservation Reserve Program (CRP), up to 1 million acres may be wetlands for inclusion in the AWRP over the next 5 years. The AWRP has a limit of 200,000 acres per year. However, in contrast to the CRP, easements are to have terms of 30 years or longer. Although this program may cost
the taxpayers as much as $100 million annually, once 1 million acres are enrolled, this acreage will no longer be eligible for USDA grant and subsidy programs; its net cost may therefore be significantly less than the appropriation for the program suggests. The AWRP is the largest wetland restoration program in the nation's history, and it has the potential to play a significant role in the restoration of some aquatic ecosystems. For this to happen, environmental quality criteria must have at least as much weight in the selection of AWRP candidate parcels as production control and cost criteria do. Environmental quality criteria should include consideration of opportunities to restore natural vegetation corridors along streams and rivers, as well as corridors between isolated but large wetland tracts. Within large ecosystems, such as the prairie pothole region or the floodplains of the Mississippi River and its tributaries, for example, federal resource agencies with support from state agency and academic experts and the public should be able to identify high-priority wetland restoration sites. If the AWRP can be used to foster restoration of such sites, it will become a credible, powerful tool for aquatic ecosystem restoration that should be expanded when the Food Security Act next comes up for reauthorization in 1995.
Federal Water Projects
Federal agricultural and urban flood control, navigation, and irrigation projects have done extensive damage to wetland systems. One careful, economic study has shown that at least 30 percent of the loss of millions of acres of bottomland hardwood wetlands in the lower Mississippi valley from 1934 to 1984 was caused directly by federally funded COE and Soil Conservation Service flood control projects. The pace of these projects has slowed considerably in the last 10 years as a result of budget constraints, completion of many projects, and passage of the Water Resources Development Act (WRDA) of 1986 (P.L. 99-662) that, for the first time, imposed significant cost-sharing requirements on states or local interests for major water projects. However, the role of federal agencies in designing and implementing wetland restoration projects has been exceedingly limited to date. In coastal Louisiana, COE has used some dredged materials from maintenance of navigation channels to construct deltaic wetlands and has constructed the Carnaevon water diversion project, which is designed primarily to divert Mississippi River water and sediments to offset saltwater intrusion and build new wetlands.
At least four actions of Congress in 1990 suggest that federal water development agencies may begin to become actively involved in actual wetland restoration projects.
-
Section 306 of the 1990 WRDA provides that ''environmental protection" is now a central mission of COE, coequal with its traditional missions of navigation and flood control. Although environmental protection is not synonymous with aquatic system restoration, it is a step in the right direction. Section 307 of the 1990 WRDA also calls upon COE to develop a wetland plan "within one year." The COE water resource development program, has as an interim goal no overall net loss of the nation's remaining wetland base, as defined by acreage and function, and as a long-term goal increasing the quality and quantity of the nation's wetlands, as defined by acreage and function. The COE is also directed to carry out wetland restoration and creation demonstration projects.
-
The Congress appropriated $6 million for COE to help finance part of the restoration of the Kissimmee River and floodplain wetlands in Florida, to begin the process of undoing the channelization of the river that COE completed 20 years ago. In addition, Congress appropriated $500,000 to the National Park Service to conduct a hydrologic study of the Everglades system that could serve as a basis for a larger water redistribution program for South Florida, with the objective of restoring the Everglades ecosystem.
-
Through the Coastal Wetlands Planning, Protection and Restoration Act of 1990 (P.L. 101-646), Congress has directed COE, EPA, and other federal agencies to work with the state of Louisiana to identify and construct wetland restoration projects. These agencies will also assist Louisiana in devising and implementing a management plan to abate wetland loss based on the no-net-loss principle and to achieve wetland restoration in coastal Louisiana, an area that has 40 percent of the nation's coastal wetlands and 80 percent of its coastal wetland erosion. Congress has appropriated approximately $50 million per year toward this major initiative.
-
Finally, Congress enacted the Fallon Paiote Shoshone Truckee Carson Pyramid Lake Settlement Act, which includes an appropriation to buy 50,000 acre-feet of water rights from willing farmers to help to restore water flows to and in the Stillwater wetlands in Nevada.
PROGRAMS FOR WETLAND RESTORATION
Although the nation has programs under the Clean Water Act to restore the chemical quality of water in aquatic ecosystems by reducing point source pollution, it has not had any systematic programs to restore the physical characteristics of such ecosystems. Programs such as that provided by Section 404 of the Clean Water Act and the Swampbuster program are intended to retard loss of wetlands, not restore them. Various federal programs encourage small-scale,
nonsystematic restoration programs. These include the Section 404 program, the Conservation Reserve Program, FWS restoration projects, and a limited number of COE and Bureau of Reclamation projects.
Section 404, Clean Water Act
Clean Water Act regulations establish procedures for permitting many activities in wetlands. Consistent with Council on Environmental Quality (CEQ) regulations implementing the National Environmental Policy Act, Section 404 permit writers typically seek to avoid or minimize impacts on wetlands resulting from those activities. Where impacts are unavoidable, however, other forms of mitigation such as enhancement, restoration, or creation of replacement habitat are often employed to offset losses. In many cases, restoration should be the preferred method. Although many small-scale wetland restoration projects are being conducted as required by conditions of Section 404 permits. COE maintains no systematic record of these projects.
Conservation Reserve and Agricultural Wetland Reserve Programs
Congress established the Conservation Reserve Program in the 1985 Food Security Act to provide incentives to farmers to revegetate highly erodible lands. Under the program, farmers enter into 10-year contracts and receive annual payments as long as they remain in the program. Some drained wetlands have been restored through this program. Most of these are prairie pothole wetland restoration projects in north-central states; CRP has funded a smaller number of wetland restoration projects in the South. The USDA estimates that millions of acres of cropland have been taken out of production through this program.
As much as the CRP has achieved, it has severe limitations as a wetland restoration program. The USDA views the CRP primarily as a crop production control program. Wetlands were included almost as an afterthought. Although restoration of scattered wetlands in the prairie pothole region successfully reestablishes duck breeding, feeding, and nesting habitat, such opportunistic, small-scale restoration projects may have fewer benefits in other kinds of wetlands systems, such as broad floodplains of southern rivers. Furthermore, CRP contracts are for only 10 years, enough time for the establishment of herbaceous wetland species but not for the reestablishment of wetland forests. Also, farmers may withdraw the contracts after 4 years.
The Agricultural Wetland Reserve Program of the 1990 Farm Bill is directed at wetland systems and provides for conservation easements
of 30 years or longer. With these features, the AWRP has the potential to be used to promote restoration of large, forested wetland systems. The 1990 bill authorizes funding that could support the restoration of one million or more acres of former wetlands.
Farmers Home Administration and Resolution Trust Corporation Lands
The Farmers Home Administration and the Resolution Trust Corporation have been taking over and assuming title to hundreds of thousands of acres of land due to foreclosures on farmland and to the bankruptcies of savings and loan banks. Some of this acreage is former wetlands, cleared and drained for agricultural or other use.
In general, these small wetland restoration projects provide very useful opportunities to restore individual wetland parcels. By themselves, however, they are unlikely to restore larger wetland systems that have been seriously degraded or to restore wetlands throughout a large landscape. The federal agencies responsible for water resource development projects, in particular COE, the Tennessee Valley Authority, and the Bureau of Reclamation, have, until very recently, paid little heed to opportunities for aquatic ecosystem restoration. Even EPA's Office of Wetland Protection does not have a program for supporting active wetland restoration. Its policy emphasis is more on wetland creation than on wetland restoration (W. Sipple, U.S. Environmental Protection Agency, personal communication, October 1990).
In the absence of federal programs designed to support large-scale restoration of wetlands, such projects are thus left to a mixture of grassroots, local, and state initiatives.
STATUS OF WETLAND RESTORATION RESEARCH AND TECHNOLOGY
Research on wetland restoration has focused chiefly on techniques of species establishment and on development of species composition and wetland community structure. The functional values of wetlands, although widely recognized, are seldom evaluated. Mitigation efforts cannot yet claim to have duplicated lost wetland functional values. It has not been shown that restored wetlands maintain regional biodiversity and recreate functional ecosystems (Zedler and Weller, 1989). There is some evidence that created wetlands can look like natural ones; there are few data to show that they behave like natural ones. In many cases, scientific knowledge of how to restore degraded
wetland systems is limited, and wetland creation has been largely a matter of trial and error.
The largest single wetland restoration and creation research program has been the Dredged Materials Research Program of the U.S. Army Corps of Engineers (e.g., Woodhouse et al., 1974; Johnson and McGuinness, 1975; Parnell et al., 1978; Saucier et al., 1978; Newling and Landin, 1985). However, the construction of wetland habitat on dredge spoils is not considered restoration. Establishment of wetland biota and functions on spoils may involve unique problems, such as high erosion rates, extreme hypersalinity, or toxic contaminants that often go beyond those of on-site restoration. However, the research from dredge spoil sites has helped develop restoration ecology, as evidenced by the literature on individual species requirements, contaminant impacts, successional patterns, and wildlife establishment (e.g., Parnell et al., 1978).
Importance of Setting Goals for Functional Value
To set goals for the functional values of wetlands, we must understand how each type of natural wetland performs (i.e., in support of food chains, hydrologic functions, and water quality improvement). Success could then be measured by the degree to which those functions are achieved. Functional performance is much more difficult to assess than are structure or composition. It is also much more difficult to characterize in the permit process. Goals of wetland restoration have seldom included functional performance, and functional values have not been followed over time. An exception is a restoration site along the San Diego Bay (see Box 6.1), where functions such as providing persistent vegetative cover, self-maintaining populations, and nitrogen fixation were required as part of a legal settlement involving endangered species habitat.
Techniques for Restoring Wetlands
Techniques for restoring wetlands fall into three broad categories: (1) reestablishing or managing wetland hydrology, (2) eliminating or controlling chemical or other contaminants affecting wetlands, and (3) reestablishing and managing native biota (may include control of nuisance species). The restoration technique required depends on the type of disturbance (see Table 6.3). At the simplest level, restoration may involve elimination or control of overgrazing, allowing for ultimate reestablishment of native wetland biota. At a more complex level, restoration may require a combination of techniques ranging
from removing fill, reestablishing proper hydrology, reintroducing native flora and fauna, and controlling exotic species. Some of the more commonly used wetland restoration techniques follow:
REESTABLISHING RIVER FLOW
Numerous wetlands have been modified through diversion of water away from wetlands. The first step in restoration is reestablishing flow into the wetlands. The Kissimmee River and Marsh Restoration project in Florida is an excellent example of reestablishing the marsh hydrology (Loftin et al., 1990; see the Kissimmee Riverine-Floodplain case study, Appendix A). Initial restoration techniques consisted of installing structures to redivert water flow back into old river channels and adjacent marsh. Because some of the area still supports the original marsh communities, restoring the hydrology is allowing residual plant communities to reinvade the reflooded marsh.
RESTORING FLOOD REGIMES
Alteration of surface wetland hydrology occurs in several forms, ranging from excessive flooding to excessive draining. Excessive flooding of wetlands often occurs in dammed and diked rivers, streams, lakes, and wetlands. Providing control structures, or removing the structures that cause flooding, is the first step in restoration. If sedimentation in the flooded area has altered the original or "designed" elevation of the wetland, sediment removal may be required.
HALTING DRAINAGE
Loss of surface waters also commonly results from subsurface drainage, or lowering of ground water. General dewatering of the landscape through ditching even if not directly in streams or wetlands, ground water pumping, subsurface tile drainage systems, or other mechanisms can lead to surface dewatering of wetlands. As an example, installation of tile drainage systems to improve agricultural production in the prairie pothole region of the north-central United States (see case study, Appendix A) has generally resulted in the conversion of wetlands (in this case, seasonally wet systems) to agricultural lands. Restoration of the hydric condition and, ultimately, of the prairie pothole wetlands can be accomplished by destroying the drainage tile system.
The solution is more complicated when sediment loading to the depressions has raised the wetland's elevation relative to the landscape.
Where sediment loading is significant, it may be necessary to remove sediment and reestablish original wetland elevation, in addition to plugging tiles, or it may be necessary to create a low level berm to impound water in the wetland.
REESTABLISHING TOPOGRAPHY
When wetland landscapes have been altered through filling or substrate removal (dredging), reestablishing the proper landscape contour concomitant with surface and subsurface hydrology is an essential first step in wetland restoration. Needed are removal of materials from filled wetlands and replacement of materials in dredged wetlands. Finding a source of proper-quality fill material may be difficult.
CONTROLLING CONTAMINANT LOADINGS
The simplest technique for restoring chemically contaminated systems is removal of contaminant inflow. Depending on the type and concentration of contaminants, removal of already deposited material may be necessary to achieve restoration. If removal of material is necessary, it may be difficult to locate a proper disposal site for contaminated sediment.
REESTABLISHING BIOTA
The final step in restoration generally involves establishing the "proper" species or at least enhancing the process of ecological succession, especially of the native plant community or communities. The level of effort depends on the ability of surrounding wetlands to provide a source for natural invasion of flora and fauna, the size of the area being restored, and the potential for invasion of exotic species.
Although the ultimate goal of restoration should be a self-sustaining ecosystem, some management (or control) may be necessary in the initial phase of restoration (e.g., stabilizing hydrology to assist in plant community establishment). Introduction of fauna and replanting or additional planting may be necessary to achieve the desired ecosystem. The length of time dedicated to "initial management" depends in part on the degree of success achieved in reestablishing ecological complexity and wetland functions.
Most of the research related to wetland restoration has focused on planting vegetation, on the assumption that establishment of the fauna will then follow naturally. Research on introducing animal species to restored wetlands is extremely limited.
CONSTRAINTS ON ACHIEVING RESTORATION GOALS
The restoration of wetlands has the same general goal as that for all aquatic ecosystems: returning the system to a close approximation of the predisturbance ecosystem that is persistent and self-sustaining (although dynamic in its composition and functioning). The more degraded a site, the harder restoration becomes. A degraded wetland, surrounded by an industrial metropolis, may achieve its highest restoration state as an impaired system that requires active management to support native species. Even the smallest urban remnants, however, can serve an educational role, informing residents of their landscape heritage.
The sections below discuss several constraints on achieving 100 percent success in returning wetland ecosystems to their ideal predisturbance conditions. The most important of these appears to be the degree of disturbance to the site and its landscape, as illustrated by restoration projects visited by the committee or reviewed by others. Additional limiting factors include ecological, biological, and institutional constraints.
Degree of Disturbance to the Site and Its Landscape
In large urban settings (see Box 6.2), the entire hydrologic regime has been altered due to fills, drainage, ground water extractions, dams, dikes, levees, and other alterations. Water quality is often degraded by both point and nonpoint source pollutants. Sediment yields from the watershed are often great. Ecotones and buffers are often absent or degraded. Exotic plant and animal species are common.
Wetlands in rural contexts may be similarly affected. However, the number of impacts is often much lower for rural wetlands, where (because there is less development) there is often greater opportunity for restoring natural hydrology and functions. It may not be necessary to start with a comprehensive watershed and/or land use plan.
A simple model (Figure 6.3) shows the contrast between the restoration potential of a somewhat disturbed community (little damage, of short duration) that exists in a landscape with many other wetlands in good ecological condition and the restoration potential of a much-disturbed wetland in a highly disturbed (e.g., urban) landscape.
RELATIVELY UNDISTURBED SITE
The Kissimmee River site (see case study, Appendix A) is a relatively little-disturbed site in a landscape that retains many wetlands of a similar type. The flow of the river was diverted 20 years ago,
Box 6.2 San Francisco Bay, California The ecological condition of San Francisco Estuary has national relevance because much of what has happened there has also occurred in the world's other large urbanized estuaries. The Sacramento and San Joaquin rivers drain California's Central Valley—40 percent of the state's land area. They empty into a 3,000-km 2 marsh region, known as the "delta." The estuary has a surface area of 1,240 km 2(San Francisco Estuary Project, 1990). Since the mid-nineteenth century, one-third of the bay has been converted to dry land by filling, 90 percent of the bay's wetlands have been destroyed, and more than 60 percent of its freshwater inflow has been diverted, mainly for irrigation. The reduction in fresh water has been accompanied by a massive, although gradual, infusion of toxic chemicals into the bay from refineries and other industries, municipal sewage discharges, return irrigation water, accidental oil spills, and oil and grease washed by rain through storm drains into the bay. Records of commercial fish catches are the earliest systematic data on the wildlife of the bay region (Skinner, 1962). So productive were the bay's fisheries that in 1875, the bay region supplied 93 percent of the state's commercial fishery products. In 1892, the bay shrimp catch exceeded 5 million pounds; the salmon catch peaked at 10 million pounds for the year, and the oysters catch at 15 million. All fishing production in the bay peaked between 1870 and 1915 except for sealing and whaling, which had declined earlier. By 1950, however, the entire fishery was almost depleted. A similar story could be told of the waterfowl, shorebirds, and game that once abounded in the San Francisco Bay region. Prior to the Gold Rush of 1849, the 2,200-km 2 tidal marshes of the bay covered twice the area of the bay's open water. About 1,400 km 2 of these marshes were in the delta; the remaining 800 km 2 fringed the bay proper. Today only about 5 percent of the aboriginal marsh remains (Atwater, 1979) due to diking, filling, and anthropogenic sedimentation. Before gold mining and flood control came to the Sacramento River Valley, nearly 60 percent of the delta was flooded by ordinary tides, and high tides covered almost the entire Delta (Kahrl, 1979). The delta's 2,023 km 2 were also inundated by the Sacramento River's flood waters. The bay was contiguous with the open water of the delta, which was itself contiguous with an enormous expanse of tule marshes many kilometers wide, consisting of tule (Scirpus validus), common |
tule (Scirpus acutus) and other dominant and subdominant marsh species. The tule marshes followed the courses of the Sacramento River bottomland and extended along the San Joaquin River through the Central Valley. Extensive levees on the delta eventually excluded tides and floodwaters from 90 percent of the delta, vastly changing its physical character, vegetation, and fauna. Changes in the bay-delta are well documented in Nichols et al. (1986). Nineteenth-century hydraulic mining in the Sacramento-San Joaquin drainages deposited tens of millions of cubic meters of earth and rock in the bay, reducing its depth, and changing its shape and circulatory patterns. Combined with the loss of marshes and the reduction in freshwater inflow to less than 40 percent of historic totals, deleterious changes detected in planktonic abundances had repercussions throughout the aquatic food web. Dams above the delta have cut off anadromous fish from their spawning grounds, and alterations in freshwater flow regimes and salinity have contributed to the demise of bay fisheries. These problems have been compounded by massive discharges of agricultural wastewater, much of it containing toxic elements, such as selenium, leached from the soil, along with sulfate and nitrate from fertilizers and soil amendments. Untreated urban runoff, containing substantial quantities of oil and grease, and spills of industrial chemicals add further to the stresses being placed on the estuary. Damage to aquatic life from untreated discharges was first documented in the early 1950s, about the time municipalities around the bay began giving their sewage primary treatment (San Francisco Estuary Project, 1990). Secondary treatment of sewage began in the mid-1960s, along with the consolidation and relocation of discharges to deeper water (San Francisco Estuary Project, 1990). Effluent discharge standards were tightened as a result of the state's Porter-Cologne Water Quality Act of 1969 and the federal Clean Water Act of 1977. Today 37 percent of the publicly owned treatment works perform tertiary treatment on waste streams, and the remainder deliver secondary treatment (San Francisco Estuary Project, 1990). Over the past 30 years, more than $3 billion has been spent on improvements in wastewater treatment or discharge (Condit, cited in San Francisco Estuary Project, 1990). Numerous wetland restoration projects have been conducted on San Francisco Bay (Berger, 1990) and on the West Coast (Josselyn and Buchholz, 1984). For a comparison of natural and restored eastern coastal marshes with respect to fish and wildlife habitat value, see Roberts (1989). |
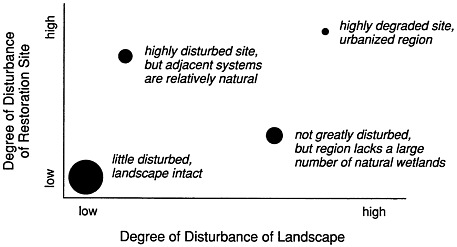
FIGURE 6.3 Hypothesized model of restoration potential for wetlands differing in degree of disturbance and landscape condition. Large dot indicates high potential for successful restoration; smaller dots indicate comparatively lower potentials.
but much of the meandering riverbed was left intact. Flows were restored to a portion of the natural river channel 5 years ago. The 15-year disturbance was sufficient to allow upland species to invade the old river shoreline to eliminate the food base for invertebrates, and to modify the substrate. However, after 5 years of restored flows, many of those damages are being repaired—predisturbance wetland vegetation is returning, the substrate and food base are recovering, and native invertebrates are reestablishing themselves. The abundant wetlands in and around the Kissimmee River retained seed banks and provided a reservoir of native invertebrate, fish, and bird species able to reinvade the restored river channel. The river flow is a hydrologic connection that serves as a wildlife corridor, allowing dispersal of wetland plant propagules and migration of aquatic animals.
HIGHLY DISTURBED SITES
Examples of highly degraded systems (Figure 6.3) are the urban salt marshes of Southern California (see Box 6.1) and New Jersey (see Box 6.3). In both these cases, the damage to individual sites has been severe, and large portions of the landscape have been modified, leaving few refuges for native species and thus reducing opportunities for reestablishment of the total plant and animal community.
In San Diego Bay, the historic wetlands have been subjected to both dredging and filling. The natural shoreline is no longer visible. Excavation for cordgrass restoration unearthed sandy, rather than
Box 6.3 Restoration in the Hackensack River Meadowlands: Summary The Hackensack Meadowlands is a 21,000-acre estuarine area of fresh-and saltwater marshes and meadows situated in the lower Hackensack River basin amidst the New York-northeastern New Jersey metropolitan area. Almost 18,000 acres of the Hackensack Meadowlands was originally wetland (M. Thiesing, Marine and Wetlands Protection Branch, U.S. Environmental Protection Agency, personal communication, 1991), but extensive development, drainage, diking, filling, garbage dumping, and sewage pumping have disturbed many of the Meadowlands' natural ecological processes. The Hackensack Meadowlands Development Commission (HMDC) was set up by an act of the New Jersey legislature in 1969 to improve the management of the Meadowlands by providing for the reclamation, planned development, and redevelopment of the Hackensack Meadowlands within Bergen and Hudson Counties (HMRDA, 1968). Since 1969, HMDC has overseen economic development worth $1.5 billion in the Meadowlands while helping to bring about major environmental improvements. Some of the new development, however, has impinged on natural areas. From 1969 to 1984, more than 863 acres of wetlands were filled in accordance with HMDC's masterplan; little filling has occurred since then (Smith, 1991), and habitat enhancement work on 190 acres of wetlands has been performed in mitigation. Detailed records have been reviewed on a recent significant mitigation conducted by Hartz Mountain Industries (see Hackensack Meadowlands case study, Appendix A). Ecological studies dating back to the late nineteenth century indicate that in the last phase of its natural succession, the Hartz Mountain project site may have been high brackish marsh dominated by salt hay (Spartina patens) and salt grass (Distichlis spicata), with an Atlantic white cedar (Chaemecyparis thyoides) bog at its southern upland edge before the whole area was ditched and then diked between 1914 and 1950 (HMDC, 1984; Kraus and Smith, n.d.; Smith 1991). The resulting altered hydrology quickly led to major changes in vegetation. With tidal flow excluded and water salinity reduced, the common reed (Phragmites australis) invaded the area and became the dominant vegetation. Construction of the Oradell Dam across the Hackensack River upstream from the project site in 1922 reduced the freshwater flow, further altering ecological conditions. |
The Hartz Mountain project, a mall and office complex, was allowed to proceed and fill 127 acres of wetlands, with the stipulation that the company would have to mitigate its impacts by construction of a 63-acre brackish marsh in Secaucus, New Jersey on Mill Creek. The mitigation goals were to enhance wildlife diversity and abundance by converting the site from a common reed-dominated high marsh community to a cordgrass (Spartina alterniflora) intertidal marsh. Between 1985 and 1987 the high marsh was sculpted into channels and open water; lower-elevation intertidal zones, and raised areas (berms) from +5.73 to +10.33 National Geodetic Vertical Datum (NGVD), built up of excavated materials. More than 80 percent of the site is now inundated during part of the mean tide cycle, and a vigorous growth of cordgrass has become established. The intertidal cordgrass marsh created out of high marsh at the mitigation site appears to have met its goals of enhancing habitat heterogeneity, vegetational diversity, and wildlife utilization, principally by birds. However, the project should be viewed as a habitat enhancement and conversion rather than an ecosystem restoration for the following reasons:
The regulated development of HMDC is far better than the indiscriminate dumping and haphazard development that preceded HMDC. Water quality in the Hackensack River appears to be far better than the sewerlike conditions reported 20 |
years ago. Certain aquatic organisms, such as grass shrimp and mummichog, are now thriving in vast numbers, and some species of waterfowl and fish have returned. However, as this committee has pointed out elsewhere, ecosystem restoration involves more than water quality improvement and increased wildlife use. |
native, marsh soils, as well as broken glass and other buried trash. Within San Diego Bay and in San Diego County as a whole, only about 10 percent of the natural salt marsh acreage has escaped urban development. The Hackensack Meadowlands site is a second example of restoration efforts undertaken in an extremely disturbed urban setting (see Box 6.3) characterized by widespread changes in tidal flow patterns, extensive deposits of toxic materials, human disturbances, and invasions of undesirable species (Phragmites) . In both these cases, the sites have experienced great damage both locally and to their biological support systems.
SITES WITH INTERMEDIATE DISTURBANCE
Systems with an intermediate degree of disturbance (Figure 6.3) also exist, where either the site or the landscape (but not both) is still intact. Several examples illustrate the variety of challenges facing restoration projects in such sites. Carolina Bay wetlands of the southeastern United States are abundant throughout the southeastern Atlantic Coastal Plain (Prouty, 1952). These isolated elliptical wetlands range in size from less than 50 m to more than 8 km in diameter and may be either only temporarily inundated or permanently flooded. Although they occupy a small areal portion of the landscape, their ecological importance to wetland and semiaquatic organisms is great (Sharitz and Gibbons, 1982). Most of these wetlands have been disturbed, chiefly through ditching and draining to support agricultural usage. Many have been repeatedly plowed and planted or continually grazed by livestock; permanent ponds have been dug in others. Most of these bays are surrounded by agricultural land or managed forests; very few are physically connected with other wetlands (Sharitz and Gibbons, 1982). Reestablishing the hydroperiod by closing ditches or filling artificial ponds and cessation of agricultural use may allow these bays to resume their wetland function (Schalles et al., 1989).
On the Savannah River site in South Carolina, characteristic biotic communities have recovered in the 40 years since agricultural usage ceased and natural hydroperiod was reestablished. The persistent seed bank allows rapid recovery of the vegetation of these wetlands if disturbance has not been excessively severe (e.g., soil removal) or long. Dispersal of wetland plant species to recovering bays may be facilitated by waterfowl that use these wetlands when they are inundated. Thus, functional recovery of these systems may occur relatively rapidly after restoration of a more natural hydrologic state.
The Salmon River salt marsh in Oregon (Frenkel and Morlan, 1990) is also an example of a site with intermediate restoration potential— the effects of disturbance are not easily reversible, yet the site exists within a region that has large reserves of natural habitat. In this case, a 21-ha salt marsh was diked in 1961 and used for grazing, as fresh water gradually diluted the salts and allowed pasture vegetation to become established. During the 17-year diked period, the topography subsided 30 to 40 cm due to compaction and loss of soil buoyancy. The U.S. Forest Service breached the dike in 1978 to restore the salt marsh to a functional ecosystem. However, the topographic subsidence was not readily reversible. Ten years after dike breaching, accretion had raised the topography only 2 to 5 cm throughout most of the site. Native salt marsh plants have now reestablished themselves at their appropriate intertidal elevations, but the area of low salt marsh is much larger than that present before diking. From a functional standpoint, aboveground biomass indicates a high level of similarity with productivity in reference wetlands. Thus, the restoration process is under way, but at the current rate of accretion, reestablishment of the predisturbance high salt marsh could take several decades. The case is an example of a site that would fall in the upper left portion of the model shown in Figure 6.3.
In the northern United States and Canada, prairie pothole wetlands were altered by European settlers to facilitate farming (see case study, Appendix A). The poorly drained depression soils were drained by elaborate and extensive tile fields, seasonal inundation was eliminated, and the potholes were converted to tillable land. Approximately one-half of the 20 million acres was drained, with dramatic effects on fish and wildlife habitat. Restoration efforts supported by federal, state, local, and private programs are now under way. Physical measures to restore the natural hydrology, such as breaking the tile fields and filling ditches, followed by natural recovery of wetland plant communities, have restored waterfowl habitat. The chief obstacle is development of a program to persuade individual landowners to take pothole areas out of agricultural production and allow
these restoration measures to take place. Many of these pothole sites could be described as falling in the lower right portion of Figure 6.3.
Bottomland hardwood forests of the southeastern United States have undergone rapid reductions in area and changes in composition (Box 6.4). The lower Mississippi River floodplain is an example of a large-scale disturbance in which the physical condition of the wetland area has been altered and cumulative impacts have occurred. Large areas of mature forested wetlands have been removed or totally disrupted through diking, draining, and clearing for conversion to agriculture, and through urban encroachment. Extensive federal water management and flood control programs in the Mississippi watershed have altered the basin hydrology on a large scale and have allowed major changes in land use to occur. Thus, within this landscape, only 23 percent of the area of floodplain forest remains (Tiner, 1984). The converted agricultural lands typically retain the natural alluvial soils even though the hydrology of these floodplain and backwater areas has been substantially altered. If crop cultivation ceased, wetland vegetation (including forests) would be reestablished eventually in much of the area because the poorly drained alluvial soils hold sufficient moisture. Blockage of small drainage ditches and canals and breaching of levees or dikes would enhance recovery of riverine overflow hydrologic conditions. These former forests could be characterized as falling somewhere in the middle of the model shown in Figure 6.3 and would require a longer time for regrowth than would marshes.
Reforestation with bottomland species is being undertaken in several large-scale efforts on federal lands, with wildlife habitat and increased timber values as the goal. Much of the converted wetland area in the lower Mississippi valley is privately owned, however. Furthermore, removal of large water-control structures is not politically or economically realistic. Thus, the major constraints on wetland restoration in the lower Mississippi valley are not so much physical or technical as institutional and economic.
If the model shown in Figure 6.3 is correct, then the two disturbance variables (for the site and the surrounding landscape) can be extremely useful in predicting the restoration potential of various wetland systems. Understanding the factors that limit restoration potential can lead to setting realistic goals for systems that will be most difficult to restore and to making better decisions when restoration is proposed as mitigation for further destruction of wetland habitat.
Because some landscapes have lost the majority of their wetland area to irreversible uses, restoration opportunities may be few in these areas. The remaining degraded systems may be highly stressed, yet
Box 6.4 Bottomland Hardwood Wetland Restoration In The Mississippi Drainage At the time of European settlement, approximately 80 million hectares of forested wetlands existed in the conterminous United States. By the mid-1970s, this area had been reduced to about 24.4 million hectares (Gosselink and Lee, 1989). Drainage, logging, and conversion to agriculture accounted for most of the losses, especially in the Mississippi floodplain, where 78 percent of the forested wetlands have been lost (MacDonald et al., 1979). Clearing for cotton fields was extensive in the early 1800s. In the 1920s, following major floods, congressional actions began to increase federal flood control project construction in the lower Mississippi River valley. River channelization and flood control structures altered the natural hydrologic regimes of vast floodplain areas. Further conversion for soybeans has had substantial impacts on the bottomland hardwood resource in recent decades, as have urbanization and industrial development. The drained and converted agricultural lands of the Mississippi floodplain retain the natural alluvial soils; it is the hydrology that has been greatly altered. In these cases, restoration of large areas could be initiated by halting row crop planting and other farmland activities and by closing drainage ditches and small canals. In many areas, the clay soils retard drainage and hold sufficient moisture, so that wetland vegetation would become reestablished. Planting of wetland forest species can accelerate the return to a bottomland forest. It is not realistic, however, to anticipate that true restoration to the original geologic, hydrologic, and biological conditions is possible except in very limited areas. Most bottomland forest restoration projects focus on techniques of planting and establishing forest species (i.e., reforestation). Restoration success is commonly judged, at least in the early phases, by the success of tree seedling establishment, and increased timber values and wildlife habitat are often the major goals. Typicalcosts of direct seeding in 1989 were about $40 to $60 per acre (Allen and Kennedy, 1989); planting seedlings costs two or three times as much. The most successful technique is to plant mixtures of species in blocks or rows. This approach enhances the establishment of species that grow more slowly and compete poorly. A small number of restoration projects have come under the Conservation Reserve Program. Most of these projects began during the late 1980s. Although some may appear promising in terms of species composition and structure, it is too soon to assess the recovery of other wetland functions. |
they can still perform critical functions associated with wetlands. Restoration measures must be carefully designed to retain any remaining values, including functional attributes, wetland soils, biota, and gene pools. In such landscapes, the creation of new wetlands should be explored as an interim measure, to provide habitats for mobile organisms, to determine how well restoration efforts might work in degraded areas, and to retain genetic diversity.
Ecological Constraints
Ecologists predict that early succession communities should be easiest to restore. Hartman (1988) calls the smooth cordgrass (Spartina alterniflora) a good colonizer species, with a ready ability to expand vegetatively. Seneca and Broome (in press) have evaluated a large number of restoration projects that were studied over a period of several years; for some, the vegetation, invertebrates, and birds are very similar to those found in natural cordgrass marshes. Broome (1989) reviewed wetland restoration projects in the southeastern United States and stated that "a smooth cordgrass stand established on sand in an area where natural marshes are relatively young will likely be comparable to the natural marsh for most measurements in a few years." In contrast, Broome predicts that it will take several years to restore more mature marshes that have accumulated peat and have highly organic and nutrient-rich soils.
Similarly, ecosystems dominated by short-lived plants might be more quickly restored than those dominated by long-lived perennials. Not many wetlands are dominated by annuals, although the vernal pools of California are, and these are persistent communities. Because one of these annual species is endangered (the mesa mint, Pogogyne abramsii; cf. Zedler, 1987), there are now attempts to restore its habitat and recover the population to levels that would allow it to persist. Restoration involves the scraping of shallow impoundments where soils already have a clay layer to retain rainwater. Four years after construction of several dozen pools at Del Mar Mesa (San Diego) in 1986, successful establishment of mesa mint and its other plant associates is still not at hand (P. Zedler, San Diego State University, personal communication, June 1990). A series of dry years (with less than the average 25-cm annual precipitation) has reduced mesa mint densities in natural pools, as well as in artificial ones, so it is not clear if declines would continue in wetter years while a seed bank is still present. The project might not fail; however, its success seems to depend on the weather—a most unfortunate circumstance in a region with a history of prolonged droughts.
Biological Constraints
The native biota may also set limits on the degree to which predisturbance conditions can be restored. On the one hand, cattail marshes are a pioneer community with a few plant species that spread rapidly into open areas, including disturbed areas. Such species are preadapted to colonize newly graded sites. A mature spruce bog, on the other hand, requires decades, if not centuries, to develop in nature. Reconstruction of a forest would take at least as many years as the age of the oldest trees, and replacement of the peat that had accumulated over centuries would take considerably longer.
CONSTRAINTS ON THREATENED SPECIES
Large numbers of wetland species have received special attention because their populations have dwindled to levels that mark them as endangered. Careful study of endangered species indicates that they have complex requirements and narrow ecological limits. One particular plant, the salt marsh bird's beak (Cordylanthus maritimus ssp. maritimus), is a good example of how difficult it can be to restore such populations (cf. Fink and Zedler, 1990). This hemiparasitic annual lives only along the upper margin of salt marshes. Although it can live independently in the greenhouse (with water and nutrients added), it grows best when its roots can attach to those of grass species hosts. The plant is not very salt tolerant during germination or growth, nor are the seedlings very tolerant of inundation. It needs partial shade because plants grow poorly under either open conditions or a dense canopy. Attempts to reestablish populations of this species to restored salt marsh habitats have not yet succeeded (B. Fink, San Diego State University, personal communication, June 1990). In addition, field trials suggest that pollinators are limiting and that the small-scale disturbances that create openings in the marsh canopy may be lacking. Small burrowing rodents may have been the natural factor that maintained open patches in the salt marsh.
We cannot yet rely on our ability to restore wetlands that support remnant populations of endangered species, desirable as that may be. Therefore it is not an acceptable mitigation policy to allow further damage to ecosystems that cannot be fully restored to compensate for further losses. Until restoration and creation activities can guarantee full replacement of wetland functions, no further modifications of endangered species habitat should be allowed, because the risk to biodiversity is too great. Where restoration is intended to
compensate for further damages to wetlands, the activity should be restricted to systems that clearly can be replaced.
CONSTRAINTS IMPOSED BY EXOTIC SPECIES
The susceptibility of a site to invasion by exotic or undesirable species is of primary concern because aggressive plant species such as Phragmites australis, Lythrum salicaria, or Typha species may dominate sites intended for other vegetation (Larson, 1988; Odum, 1988). Of recent and urgent concern is the spread of three Spartina species (S. alterniflora, S. patens, and S. townsendii/anglica) along the West Coast of North America. All three species occur in Washington, Oregon, and California's San Francisco Bay. It is ironic that at least some of these introductions were made deliberately for the purpose of marsh "restoration" (Spicher and Josselyn, 1985). The exotic plant problem is now considered urgent in the Pacific Northwest. The state of Washington formed a multiagency working group in 1989 to address the problems of habitat alteration and impacts on fisheries and wildlife. They funded a literature review (Aberle, 1990) and sponsored a scientific workshop in November 1990 (T. Mumford, Department of Ecology, Seattle, personal communication, 1990) to develop a research, management, and control program.
Exotic animal species are of equal concern, although their ecology and control mechanisms are little understood. The zebra mussel invasions of the Great Lakes (see Chapter 4) show how quickly a lake system can become dominated by an exotic brought in with ballast water. The recent invasion of San Francisco Bay by an Asian clam, Potamocorbula amurensis, and its current dominance of subtidal habitats (F. Nichols, U.S. Geological Survey, personal communication, July 1990), verify the need for concern. Estuaries are particularly susceptible because of their "seascape linkage" to other coastal areas that are used as ports and where ships disperse foreign organisms and larvae as they discharge ballast water. Carlton (1989) indicates the magnitude of the problem by calling attention to 32 known exotic species that have become established at South Slough, a National Estuarine Research Reserve (NERR) in Coos Bay, Oregon. The exotics include two plant species (Zostera japonica and Sargassum muticum), two fish species, four bryozoans, nine crustaceans, three molluscs, several polychaete species, two coelenterates, and one sponge. Further south, Nordby and Zedler (1991) reported the occurrence of two exotic fish species in Tijuana Estuary, another NERR, and Rutherford (1989) documented the occurrence of a Japanese mussel, Musculista senhousia, in a San Diego Bay salt marsh restoration site. In the lower
Mississippi drainage area, the introduced nutria (Myocastor coypu) has spread throughout swamps and marshes and has retarded reforestation of bald cypress wetlands by feeding on planted cypress seedlings (Conner and Toliver, 1990).
Although some efforts are under way to control invasive plant species (e.g., Spartina species; T. Mumford, Washington State Department of Ecology, personal communication, July 1990), there is little possibility of controlling invasive animals, other than by preventing their introduction. As Carlton (1989, p. 271) said of their impact,
These have been so fundamental and pervasive that we may never fully know what the biota of the continental margins looked like before ships and before the movements of commercial fishery products. But with increased awareness of the scale and rate at which introduced species are being transported and released today, we may be able to develop more specific and enforceable controls on the movement and release of species for mariculture, for scientific research, or by ballast water.
Such invaders may pose much greater problems for restoration sites than for natural systems, because disturbed substrates have few defenses against germinating seeds or settling larvae.
Institutional Constraints
Legal, political, and economic constraints often govern where restoration can be done and how effective it will be. Legal issues concern land ownership and regulatory processes, discussed below. Political decisions may determine whether funds are available. Federal land management, water resource development, agricultural, and environmental agencies lack a clear mandate for wetland restoration. Although the lengthy list of restoration strategies posed by the U.S. Fish and Wildlife Service (1990) is encouraging, funding for restoration is not high on the nation's environmental priority list.
Wetlands are sensitive to small changes in water supply, hydroperiod, flood cycles, and sediment regimes because they are shallow-water or wet-soil systems. A persistent change in water depth of 0.3 m can greatly affect the functioning and species composition (e.g., marsh versus shrub versus forest) of a wetland. Wetlands are thus very susceptible to hydrologic manipulations caused by dams and dikes that reduce inflows, and by irrigation runoff, urban drainage, and wastewater discharges that increase inflows. Thus, restoration of wetland hydrology must be integrated institutionally, as well as technically, with manipulation of water level and management of hydroperiod. Manipulation of water levels is often the task of one agency
(e.g., U.S. Army Corps of Engineers), whereas restoration efforts are carried out by another (e.g., U.S. Fish and Wildlife Service or a state's natural resources department). Integrated water and land use planning is needed to restore the essential surface water elevations, flood cycles, water velocities, and (for coastal systems) salinity regimes so that the desired wetland ecosystem can be achieved.
Implementing the president's goal of no net loss of wetland acreage and function will not be cheap. Economic constraints will always limit the location, number, and types of projects that can be implemented. The techniques used to restore wetlands vary widely in cost. Wetlands that are near urban centers are the most expensive to restore because of the cost of land. For example, the city of San Diego recently paid $3.5 million for a 20-acre semitidal wetland that will still need hydrological restoration and topographic modifications. Restoring the 250-acre Ballona wetland (near Los Angeles airport) may cost $50 million, in part because a major roadway through the marsh would have to be elevated so that it would not be inundated by the restored tidal flows. At state and local levels, other demands for land use and funds usually take precedence. Thus, in general, the potential for major restoration projects is currently lower in urban than in rural areas.
REGULATORY VERSUS NONREGULATORY CONTEXT
Wetlands have been restored in several nonregulatory contexts such as the creation of waterfowl impoundments using water-control structures, the removal of dikes from coastal and estuarine marshes, the blockage of drainage on partially drained agricultural lands no longer used for agricultural purposes, and the grading of gravel pits and other strip-mined lands.
The majority of wetland restoration efforts, however, have occurred as a result of federal, state, or local regulatory actions. In these contexts, private or public landowners seeking permits for various types of development are required either to create, to enhance, or to restore wetlands on-site after damage or to restore wetlands at other sites to compensate for wetland damage at a development site. Few of the Clean Water Act, Section 404 mitigation projects have constituted wetland restoration as defined in this report, and COE and EPA—the two agencies responsible for implementation of the Section 404 program—do not have systematic information about the number of acres of wetlands restored or the effectiveness of particular restoration projects. In the few states for which Section 404 permit records have been surveyed by EPA, the general finding is that mitigation was not
accomplished. The site had not been examined to see if restoration had been done, was not monitored, or was shown to differ substantially from what was required in the permit (U.S. EPA Environmental Research Laboratory, Corvallis, Oregon, manuscripts in preparation).
For several reasons, the requirements for successful restoration are often unfulfilled in regulatory (mitigation) contexts:
-
Wetland restoration projects undertaken as mitigation are, quite often, poorly designed by individuals lacking multidisciplinary expertise. Hydrology, sediment regimes, control of exotics, and protection of buffers or ecotones are often inadequately addressed.
-
Landowners often prepare the least expensive and least time-consuming restoration plan acceptable to the regulatory agency. The owners and managers of mitigation wetlands are rarely motivated to complete the restoration or make corrections except those mandated by regulatory requirements. This often means half-hearted attempts to restore or, in some instances, failure to complete a restoration. In contrast, the owners and managers of wetlands in nonregulatory contexts usually have long-term wetland protection and management goals.
-
Wetlands restored in regulatory contexts are often small in size, widely separated from other wetlands, and threatened by adjacent land uses. Wetlands in nonregulatory contexts are often larger, more closely tied in with existing wetland and aquatic systems, and at least partially buffered from adjacent activities.
-
Wetlands restored in regulatory contexts often receive little management after initial restoration because private and public landowners, who are not motivated to provide such management, may move on or have no legal obligation for such management. Similarly, the responsible federal agencies do not have staff to assess the adequacy of restoration projects and do not monitor or require permittee monitoring of permit mitigation conditions for sufficient time periods (10 years or longer). As a result, such wetlands may be overrun by exotic species, quickly filled by sediment, polluted, or otherwise misused.
For these reasons, wetlands restored in regulatory contexts are much less likely to achieve the restoration goals, and the risk of failure is much greater. Many things can be done to reduce the risk of failure in a wetland restoration project (see Box 6.5). This is particularly true where landowners are allowed to destroy or damage an existing wetland based on a ''promise" of future restoration. However, in many instances the goals and success criteria for such restoration are not clearly articulated; mechanisms are not incorporated in the regulatory permit to ensure compliance with restoration plans
Box 6.5 Ways to Reduce Risks Of Failure In Wetland Restoration Projects
|
and to provide for mid-course corrections if plans fail to achieve their intended results; and no attempt is made to relate individual, piecemeal restoration efforts to broader hydrologic and ecosystem management goals.
The standards for "worthwhile efforts" will differ in nonregulatory and regulatory contexts. In rural, agricultural areas, the cessation of agriculture in floodplains and potholes will be worthwhile, even if preagricultural plant and animal communities are not restored. An attempt to achieve 100 percent success in a restoration is a desirable, but not essential, criterion for undertaking projects. Such projects would be considered more successful if measures were taken to recreate native ecosystems. The important decision is to begin the highly worthwhile process of restoring wetlands.
In the regulatory context, and particularly in highly disturbed urban settings, what is worthwhile depends entirely on the functions retained by degraded sites and on the likelihood that a more desirable system can be provided. It must be ensured that the restored system will provide more functions than were carried out before
restoration and that any functions retained by the degraded wetland will not be lost. Otherwise, the requirements of no net loss will be violated. Careful and detailed assessment of functions before and after restoration will be needed to justify restoration as mitigation for additional wetland loss.
Problems Encountered In Restoration Projects
A recent EPA publication (Kusler and Kentula, 1989) on the status of wetland creation and restoration efforts includes a wide range of experiences, opinions, case descriptions, and date that are useful to the committee's evaluation of restoration science efforts. Sixteen chapters, contributed by authors from around the nation, review the state of the art of restoring wetlands along the eastern, southern, and western coasts of the United States; in marsh, pothole, and wooded wetlands of the interior; and in riparian systems along the streams of the Midwest, West, and Southwest.
In reviewing wetland restoration projects in the coastal plain of Florida, Lewis (1989) listed several problems encountered during the early years following restoration of salt marshes, mangrove forests, salt barrens, and brackish marshes. These problems included incorrect elevations for target plant species, improper drainage (ponding and transplant mortality), wave damage, inappropriate transplanting material, human disturbance, and insufficient monitoring and reporting. He also indicated the need for buffers, control of exotic species, and long-term management of restored sites. Because mangrove ecosystems are dominated by trees, it is clear that it will take many years to restore their forest character. Attempts to plant older (>1 year) seedlings were considered risky due to lower rates of survival and higher cost.
Broome's (1989) review of tidal wetland restoration in the southeastern United States echoed many of Lewis's concerns, covering in addition experiences with sites that were too saline (hypersalinity restricts plant growth); or had the wrong soil physical properties (wrong texture) or soil chemistry (low nutrients); that had improperly timed construction (too late for planting); that lacked maintenance (e.g., replanting, fertilization, wrack removal); and that had potential problems (loss of genetic integrity) if transplanted materials were not obtained from areas close by.
For restoration projects carried out in the northeastern United States, Shisler (1989) identified additional problems associated with the colder climate. He noted that ice rafting can damage marsh vegetation, that thick peaty soils cannot be restored easily (sand is a
poor replacement), and that migrating Canada geese and snow geese can damage wetlands. He called attention to invasive species (Phragmites australis, Lithrum salicaria) and native Typha species that may need to be controlled to facilitate restoration of postpioneer plant communities. He also noted that in restoration projects, "endangered species" needs might not be met, because so few of their requirements are known.
Reviews of seagrass ecosystems (Fonseca et al., 1988; Fonseca, 1989) added three important problems to the growing list of challenges encountered in restoration projects: the difficulty of replacing climax species (e.g., turtlegrass, Thalassia testudinum; Williams, 1990), the inability of some restored sites to attract animal communities equivalent in abundance and composition to the communities of predisturbance sites, and the possibility of major transplant failure (200 acres in Biscayne Bay). On the Pacific Coast, eelgrass restoration is in a similar state, with little assurance that this wetland type can be replaced, due to poor quality of the overlying water and the underlying substrates, especially in urban areas (S. Williams, San Diego State University, personal communication, June 1990). Fonseca (1989) concludes that every eelgrass mitigation project results in a reduction in habitat. Problems that may be unique to this intertidal and subtidal habitat include damage from smothering by macroalgae, grazing by fish, clouding of water by decapods, and disturbance by motorboat propellers.
Attempts to restore forested wetlands of the Southeast (e.g., bottomland hardwoods and cypress swamps) have encountered difficulties related to the time required to replace mature trees, the lack of material to transplant, the lack of knowledge of how and when to carry out seeding or transplantation (Clewell and Lea, 1989), and altered hydrology (drainage for conversion to agriculture) of the wetland area. Natural forested wetlands may support hundreds of plant species, many of which thrive in the understory (91 percent of 409 species in one riverine forest were understory species). Old-growth forests are dominated by trees that gradually achieve a dominant role in the canopy and that are self-sustaining through their ability to reproduce in their own shade. It is not clear that such climax species can be successfully established in open sites, or whether their introduction must await development of seral (intermediate successional stage) plant communities. Clewell and Lea (1989) noted the need for intensive site preparation to reduce competition between weeds and transplanted tree seedlings. Their review was the first to mention insect herbivory and fire as potential problems. In many cases, restoration of suitable hydrologic conditions will be necessary. The short
time period within which forest restoration attempts have been monitored precludes an evaluation of their functional equivalency with natural reference systems.
In the arid and semiarid Southwest, restoration of riparian habitat has the unique problem that transplants need to be irrigated (Carothers et al., 1989). Because riparian forests became established during times of flooding, the hydrology necessary to recreate them cannot be produced at will. In many cases, dams prevent the restoration of the hydrology necessary to sustain riparian woodlands. Thus, although the tree canopy can be recreated through transplantation and irrigation, the system cannot maintain itself unless flooding regimes are also restored. In addition, invasion of exotics such as salt cedar (Tamarisk), damage to plants from grazing practices and from beaver, and permanent lowering of water tables due to ground water depletion by wells used for irrigation are other obstacles to the restoration of western riparian sites.
Holland's (1989) discussion of pothole wetlands included problems that may be most frequent in wetlands that lack an outflow. These systems have highly variable water levels, and plant distributions can shift up or down the slopes within a year as establishment and mortality occur. In transplanting such sites, it may be difficult to predict the elevation that will have the appropriate hydrology in the following growing season. A swamp restoration project in Massachusetts experienced 80 percent mortality of planted shrubs due to a year of unusual flooding.
Wetlands along the fringes of lakes and rivers experience similar water-level fluctuations, as well as erosion due to wave action. Levine and Willard (1989) documented an Ohio case of dike undermining during a year of high water and transplant failure due to drought. Either water-level extreme can restrict restoration success.
Additional problems were noted for a highly urbanized wetland in San Diego Bay, where a combined freeway widening, new freeway interchange, and flood control project has been under way for six years. Such large, complicated projects are likely to experience problems with contaminated substrates and construction errors (see Box 6.6). The most recent error (July 1990) was the accidental drainage of an important lagoon used by birds.
Summary
The problems encountered during restoration include every aspect of construction—site selection, topographic contouring, transplantation, inadequate nutrient supplies for plant growth, and pest invasion.
Box 6.6 Unforeseen Problems The problems encountered while restoring salt marsh habitat at San Diego Bay (see Box 6.1) were numerous. They indicate the need for constant surveillance during project construction as well as a mechanism to repair damage and errors as they develop. Problems will occur, and project managers will need to be on call to solve and correct them. Toxic Materials During the excavation of Connector Marsh, bulldozers unearthed an old landfill that contained toxic materials (discarded lead paint). The sediment was sufficiently contaminated with lead that large quantities had to be trucked to a toxic waste dump at a large and unanticipated cost. Project Vegetation Salvage An attempt was made to preserve the gene pool of cordgrass plants to be covered by the highway detour road. A 10,000 square feet intertidal nursery was excavated to receive the salvaged cordgrass. During excavation of the nursery site, just south of the Connector Marsh, an old city dump was uncovered, and the substrate was full of broken glass—an unforeseen hazard for personnel who did the transplantation work. Then, the initial transplant failed, apparently due to insect herbivory (transplants carried the larvae and pupae of the Dipteran, Incertella sp., to the nursery). A second transplantation effort worked well, and the nursery plants thrived for about 5 years (1985-1990). Then, during a second restoration phase, bulldozers excavating channels adjacent to the nursery also bulldozed the nursery. The nursery had not been mapped as an ecologically sensitive area, and biologists were not on-site the day it happened. Removal of Temporary Fill In constructing the temporary detour road, contractors laid down a fabric layer so that the integrity of marsh soils could be preserved. Five years later, when the material was to be removed, some sections had buckled and sunk below the planned excavation grade. While the construction crew was on hold, |
biologists had little time to decide whether the buried fabric could be left or the excavation plans altered. Plans were immediately revised, so that all fabric could be excavated, and habitat intended for mid-upper marsh was replaced by lower marsh and tidal pond habitats. Tidal Modifications Excavation of two marshes caused major and sudden changes in the tidal flushing regime of the reference wetland at Paradise Creek. Early during construction, a tide gate was erroneously installed midway along the Connector Marsh channel. The gate was in place for more than a year, and it muted tidal flows to the northern four marsh islands and the Paradise Creek Marsh. Its removal, following the advice of researchers, led to increased growth of cordgrass in the northern four islands during 1988. Early in 1990, three culverts were placed in the same channel, and a temporary access road was built with fill, in order to excavate an adjacent 17-acre marsh, known as Marisma de Naci<n. Tidal flows to Paradise Creek and the north islands were again muted. A hydrologist (Haltiner, 1990) advised that six culverts would be needed to provide adequate tidal flushing to the marshes upstream. Constractors suggested a cheaper alternative, the partial excavation of a planned flood control channel to San Diego Bay. This alternative was accepted, and the Connector Marsh restoration site was then subdivided hydrologically, with the northern half connected directly to San Diego Bay (providing strong tidal circulation) and the southern half connected through Sweetwater River. The access road was in place for several months until Marisma de Nacion was completed. Removal of the access road led to a third major change in tidal flushing, with connection of the southern islands to the bay as well as to Sweetwater Marsh. Most recently, in November 1990, the direct tidal flows to San Diego Bay were terminated because the flood control channel was again under construction. None of the physical impacts of these tidal modifications has been assessed. Hydrologists predicted that the new tidal regime would alter the sedimentation dynamics and, as a result, gradually modify the topography of the channels marsh habitats (Haltiner, 1990). Both the constructed marsh and the natural reference wetland were affected by muted |
tidal flows and sudden shifts in tidal regimes. In late 1990, after the cordgrass marsh had experienced greater tidal flushing for about one growing season, the above-ground biomass of plants had increased significantly in both the natural and the constructed marsh sites (J. Zedler and R. Langis, San Diego State University, unpublished data). This finding supports the argument that impaired tidal flushing has been detrimental throughout the multiyear construction process. The lesson is that large-scale, long-term restoration projects will not provide "stable" hydrology until well after construction has been completed. |
The result is that many projects fall short of the goal of returning ecosystems to the predisturbance condition, and there is indeed considerable controversy over whether or not wetlands can actually be restored. The arguments are particularly important when wetland restoration is undertaken within the mitigation context, and the promise of full restoration of a degraded site allows a natural wetland to be destroyed.
Experience with wetland restoration varies with region and wetland type. Many coastal and estuarine mitigation projects have been constructed along the Atlantic Coast and have been monitored and evaluated in the scientific literature. Fewer projects have been followed along the Gulf of Mexico and Pacific coasts, and there is less information in the literature about the success of restoration in these regions. Less is known about how to restore inland as opposed to coastal wetlands. The most common types of freshwater wetland restoration projects are impoundments to create waterfowl and wildlife habitat and the establishment of marshes on dredged spoils along major rivers. Although there is much literature describing waterfowl abundances, there are few critical studies of the success of these restoration efforts as persistent, naturally functioning ecosystems. The problems encountered encompass every aspect of wetland restoration. If all were to coincide, the resulting worst-case scenario (see Box 6.7) would clearly be a total failure.
Worst-case restoration projects have happened, but the lack of reports makes documentation difficult, and describing the failures of projects that powerful entities consider successful is not without risk. Even with evaluation and reports of projects, there are often large differences in opinion about how well efforts succeeded.
Box 6.7 Characteristics Of A Worst-Case Wetland Restoration Project Most restoration projects include at least one major short-coming, and the worst cases have all the following attributes:
|
CONTROVERSIES ABOUT THE SUCCESS OF RESTORATION PROJECTSCONTROVERSIES ABOUT THE SUCCESS OF RESTORATION PROJECTS
Whether we can succeed in restoring wetlands is a conterversial subject. The authors of the EPA review papers (Kusler and Kentula, 1989) were asked to evaluate the projects they described. Owing to a lack of standard criteria for measuring success and inadequate statements
as to the goals of restoration, the degree of success achieved by many projects could not be characterized. The range of opinions on those that were judged covered the spectrum from success to total failure. At conferences dealing with restoration, creation, and mitigation, a similar range of opinions can be heard, at times with controversy over a single project that has been evaluated by different individuals (J. Zedler, personal observation). Many ecologists believe that it is a major challenge to recreate in a short period of time what nature has produced over centuries (Bradshaw, 1988).
Vested Interests
Some individuals insist that wetlands can be restored to fully functional ecosystems. They point to greenbelts, parks, and mitigation sites and to lovely pictures of wetland vegetation. Because so much wetland restoration is done within the regulatory context (i.e., as a mitigation requirement for damage done elsewhere), the stakes are incredibly high, and the controversy is a heated one, as indicated in the following example.
A San Diego developer hoped to convince resource agencies that he could build 416 multifamily units on 10 acres of a 20-acre urban wetland (Famosa Slough) without having a negative impact, even though he would need to fill intertidal flats and marsh habitats. He promised to restore an equal area of city-owned wetland and to increase the value of the remaining lagoon. Wetland biologists rebutted his claims, and the city decided to buy the property as a wildlife reserve. The developer then asked $15 million for the site, claiming that the project could obtain a Section 404 permit from COE. Although there were many legal and political factors, the issue of whether or not lost wetland functions could be replaced played a role in determining the property value. In September 1990, after years of controversy and negotiation, the city of San Diego purchased Famosa Slough for $3.5 million. The difference in sale price more than $0.5 million per acre—is some measure of the value of being able to replace lost wetlands through compensatory restoration.
Within the mitigation context, proponents of new developments are extremely eager to believe that habitat functions can be moved about at will. It is not surprising that entrepreneurs promise the desired success and that considerable effort is made to promote completed projects as successful. At the same time, there is little incentive, in the form of funding, to evaluate projects independently, thoroughly, or scientifically. In particular, the funding needed to examine how mitigations sites function( not just what they look like) is nearly
always lacking. Project proponents do not want to know, and regulatory agencies cannot afford to find out.
Different Standards of Evaluation
For projects that EPA review authors (Kusler and Kentula, 1989) labeled as successful, the overwhelming majority were judged on the basis of vegetation establishment. Neither the wildlife present nor the functional capacity (i.e., for hydrologic functions, water quality improvement, food chain support) of the ecosystems played and important role in evaluation for the simple reason that reason that data were not available.
For restoration projects in general, examination of a few characteristics may indicate success, whereas detailed studies would reveal shortcomings. Although restoration sites that develop dense plant cover or support native plant species may be considered a success by some evaluators, others would require data on fish, invertebrate, bird, and mammal uses and would want to examine the site's long-term persistence before providing a stamp of approval.
Evaluations of the food chain support function are rare, although several EPA review authors (Kusler and Kentula, 1989) indicated that animal components would be established on their own. Determining whether or not the presence of several animal species constitutes equivalence of food chain support functions requires detailed studies of the kind not usually funded by project proponents or required by regulatory agencies. For an indication of standards to be applied in assessing the functional equivalency of animal communities, some well-studied Atlantic coastal marsh restoration sites provide some insights.
Cammen (1976a,b) reported significant differences in the invertebrate infauna of constructed wetlands along coastal North Carolina. At a 1-year-old marsh, the dominant taxa were insect larvae, whereas polychaetes had dominated the natural wetland. Total densities and calculated secondary production also were markedly lower than in the natural system. Similar observations were made at a 2-year-old site. However, Sacco et al. (1987) revisited the latter site when it was approximately 15 years old and reported a 10-fold increase in densities and high similarity with the natural indigenous fauna. Moy (1989) and Sacco (1989) found that the fauna of a site graded to support Spartina alterniflora had fewer nematodes, ostracods, copepods, and oligochaetes than did natural marshes.
Species composition in natural and man-made wetlands does not
always become more similar through time. Moy (1989) demonstrated a convergence of species lists for one pair of adjacent marshes but a divergence in composition over a 2-year period in another pair receiving the same source water. Sacco (1989) evaluated the composition, density, and trophic structure of infauna in coastal North Carolina marshes of age 1 to 17 years. Although variable, the data showed that the six constructed marshes had similar faunal components and trophic groupings (deposit feeders, suspension feeders, and carnivores) but that densities were always lower than in natural marshes.
Duration of Evaluation Period
A judgment of success shortly after site preparation and transplantation may not tell the whole story. Long-term success may increase or decrease, although so few examples of long-term evaluation are available that it is difficult to know why some restoration projects improve and others disintegrate. One factor might be the presence of pest species. Clewell and Lea (1989) call attention to weed species, which are abundant in the early stages of a restored forested wetland. Problems with weeds should diminish in time, with development of an overstory canopy and with the use of control measures, but other problem species may become more prevalent as restoration sites age. In San Diego Bay, a transplanted cordgrass marsh that grew well for 3 years developed an outbreak of native scale insects in its fourth year (Zedler, 1988c). The once-lush cordgrass has declined to a level that would now be considered a failure. The reason for the scale population explosion appears to be the lack of native predatory beetles, which owe their rarity at the restoration site to its isolated location (away from natural marshes) and the lack of tall cordgrass stems to provide a refuge from inundation by high tides. The reason for the short plants is not entirely clear, but low concentrations of soil nutrients are a probable cause.
The principle in both these examples is that the total ecosystem was not transplanted—projects always start with something less (e.g., fewer canopy strata, fewer trophic levels), expecting that the rest will follow in time. There is no guarantee that it will, and there is not enough experience to predict when or why it might not. Some species are keystones of the communities, and we do not yet know which ones they are. One scientifically useful aspect of restoration failures is the information they provide about the role of species that are absent.
NEEDS
Need for Wetland Restoration
Despite the constraints on achieving 100 percent success, despite our inadequate knowledge of how to restore wetlands, and despite the problems that will occur during implementation, there is an urgent need to restore large areas of wetland throughout the nation. This need derives from the severe losses of acreage and function that have occurred over the past two centuries (Dahl, 1990), as well as from the potential for substantial human benefits from restored wetlands.
Where wetlands have been drained for agriculture or silvicultural purposes, wetland restoration must be balanced against both the need for agricultural commodities and the landowners' property rights. Because landscape drainage affects both surface and subsurface waters, the halting of drainage may increase crop yields in surrounding lands, while only moderately reducing crop loss in the restored wetland. If restoration of wetland hydrology is shown to cause unreasonable losses in production, then programs to offset personal losses (e.g., CRP, AWRP crop subsidy programs) may be needed.
Perhaps the greatest short-term return can be realized by initially restoring isolated wetlands, wetlands within minimally developed watersheds, and wetlands in the upper reaches of watersheds. Elsewhere, the higher priority may be acquisition of property and/or flood easements in critical parts of the landscape to control hydrology in downstream portions of the landscape before restoration is undertaken. For example, the Russell Sage National Wildlife Refuge System near Monroe, Louisiana, is located in the midreaches of its watershed. Although reforestation of some original bottomland hardwood forest is possible, off-site liabilities related to watershed drainage and flood ''management" limit the potential for restoration of a functional bottomland hardwood forest.
Setting a Wetland Restoration Goal
Setting an acreage goal for wetland restoration in the United States requires several assumptions about the extent and condition of damaged wetlands. If only the wetlands along rivers and streams are considered, and if a 100-ft-wide wetland along each side of the waterway is restored, one estimates a potential wetland area of 77,578,758 acres (3.2 million miles, 0.038 mile wide, 640 acres per square mile).
If 30 percent of these waterways are within agricultural areas (and thus are damaged), there should be approximately 23,272,727 acres of potential buffer. Also, if as much as 50 percent of the area is undamaged, there is still the potential for more than 10 million acres of wetland restoration along streams and rivers alone.
Because more than 100 million acres of wetland habitat have been lost in the past 200 years (Dahl, 1990), the restoration of 10 million acres would constitute less than one-tenth of the lost habitat. (See Chapter 8 for recommended goals.)
Need for Adaptive Management
Wetland ecosystems are complex and incompletely understood. The shortcomings of restored and constructed wetlands are only beginning to be known. Whether or not restored wetlands will be successful depends in large part on their hydrology, which controls their structure and functioning (Larson, 1988). Nutrient supply functions are especially important. Microbial and chemical processes, which are determined by hydrologic conditions, control the concentrations of nutrients and other compounds, and facilitate the biogeochemical cycling of nutrients and the flow of energy. Restored coastal wetlands need to be able to support nitrogen dynamics, including the processes of nitrogen fixation and denitrification, both of which are influenced by soil organic matter. Freshwater wetlands need to have long-term capability for phosphorus retention by sediments, which is related to the amount of extractable aluminum in the wetland soil (Richardson, 1985).
Because our limited knowledge of how to restore ecosystems makes it impossible to guarantee that objectives can be fulfilled, projects should include an initial experimental phase for the more risky aspects. The restoration plan should be adaptive, supporting the acquisition of new information and its use in project implementation and long-term management. A plan that has flexibility and relies oninformed decision making should best be able to accommodate difficulties during construction and follow-up monitoring. As restoration proceeds, managers can reevaluate whether the degree of restoration achieved is acceptable or if new techniques should be introduced. For those situations in which unexpected benefits develop (e.g., nesting of the endangered California least tern on part of a San Diego Bay site intended for salt marsh; see Box 6.1), an adaptive management program is essential in order to capitalize on those events and to expand and revise restoration objectives appropriately.
Need for Improved Assessment
The recognized importance of natural wetland, together with the development of a policy for no net loss in wetland acreage and function, places a large burden of proof on wetland restoration projects. The emphasis in evaluation techniques should thus be on the functioning of restored systems. Ewel (1987) listed five criteria for successful ecosystem restoration: sustainability, low susceptibility to invasion by exotics, productivity similar to that of a natural counterpart, ability to retain nutrients, and biotic interactions similar to those of reference systems. It is also important to take into account the ability of restored wetlands to resume their unique role in global hydrologic and nutrient cycles.
Wetland assessment protocols have been developed for extant wetlands (e.g., the U.S. Fish and Wildlife Service's Habitat Evaluation Procedure (HEP) and Wetland Evaluation Technique (WET); Adamus and Stockwell, 1983), but these procedures can have a very narrow focus (HEP) or assume existing knowledge of the system (WET). Neither was developed to compare the functioning of restored and natural wetlands. Based on what is known of restored salt marsh systems, suggestions have been made for southern California coastal wetlands (Zedler et al., 1988; PERL, 1990). Here the committee provides general recommendations for functional assessment.
Determining whether a restoration site has developed the natural functions requires a long-term assessment. Judging its success or failure after the first year or two is inappropriate. As Odum (1988, p. 67) points out, "Unfortunately, dramatic unanticipated changes may occur over the ensuing years ... it is not uncommon for the plant community to become invaded and dominated by aggressive 'disturbance species'.... The long-term result may be a wetland environment which has limited functional value for wildlife habitat support or nutrient processing and which lacks aesthetic attractiveness to the degree originally planned." Likewise, Broome et al. (1987, p. 197) conclude that monitoring a constructed wetland for four growing seasons was insufficient to determine "if the created marsh reaches equivalent levels of production for all plant species and remains self-sustaining."
The committee recommends periodic evaluations, to determine the rate and extent of restoration, with the frequency of comparison declining through time. Perhaps after several long-term assessments have been done, that information can be used to select critical assessment time periods. Until then, evaluations at 1, 2, 3, 5, 10, 15, and 20 years are recommended. These will provide valuable information
on the differential rates of development for various functions. For example, the establishment of full canopy coverage by vegetation may take a year or two, but development of the appropriate canopy architecture (necessary height profiles) may take much longer. Nitrogen fixation may begin immediately, but nutrient concentrations (e.g., pore water (NH(4)] comparable to that of natural marshes) may take decades to build up. A constructed marsh that is 60 percent "equivalent" at 5 years of age (cf. San Diego Bay; see Box 6.1) may stagnate, or it may continue to gain functional values.
The committee believes that the standards for assessing restoration projects should be high and that field sampling protocols should be able to withstand scientific peer review. Sound evaluations should have two beneficial outcomes—results will indicate (1) the rate of the development of ecosystem structure and function, and (2) causal factors responsible for functions that are lacking or impaired. If, for example, plants are growing poorly, data on nutrient concentrations and soil redox potential can help identify corrective measures for the site (e.g., fertilization, drainage improvements) as well as help prevent similar problems in the future (Broome et al., 1987).
The absence of sufficient funding of long-term monitoring efforts is a major obstacle to evaluating the success of restoration sites. Providing financial incentives, such as bonding of restored sites in a fashion similar to that specified in current regulations for restoration of mined lands, is one possible approach. Bond monies are returned to the project developer after standards for restoration have been achieved over a period of time. Milestones for restoration success can be established, and a percentage of the bond could be returned when each milestone is reached.
Need for High Standards in Assessing Functional Equivalency (Restored Versus Natural Wetlands)
Structural and functional attributes should form the basis for evaluating the degree of restoration achieved. The committee suggests that restoration projects be evaluated against a range of specific objectives (e.g., Table 6.4), resulting in a judgment that could be as negative as total failure, where no progress is made in replacing lost values, or as positive as relatively successful in returning lost structural and functional attributes. No project should be considered a failure just because it did not reset the clock. Instead of resulting in a yes or no judgment, evaluation procedures should provide a graded scale of achievement.
Two factors will thus influence the rating of a restoration project: (1) the specific criteria used to evaluate achievements and (2) the
reference data or sites used for comparison. From the ecological perspective,
-
Assessment criteria should include both structural and functional attributes of the ecosystem.
-
Criteria should be established well before the assessment takes place and should be linked to the specific objectives of the restoration project.
-
Several criteria should be included in the evaluation; the more information, the less chance there will be for an error in judgment.
-
Criteria may need to be regionalized to reflect special concerns (e.g., in regions where wetland species are threatened with extinction, more strict standards may be required if the restoration goal is to retain biodiversity).
-
A reasonable range of reference systems and long-term data sets should be available with which to compare the restored system's attributes.
-
Assessment measurements should take into account both temporal variation and spatial heterogeneity. Attributes that are known to be patchy in either time or space would need widespread and long-term characterization.
-
There should be an a priori indication of how similar the restored system could ever be to the reference system(s). We know that 100 percent similarity to presettlement conditions is impossible to achieve. If multiple samples are taken within a community type, they may average around 85 percent similarity with one another; such an approach indicates a reasonable target for the similarity of restored and natural sites. Two parts of the same pristine aquatic ecosystem would never be 100 percent similar in either structure or function. Furthermore, two samples of the same ecosystem would not produce identical data sets.
-
There should be an a priori time frame within which the system is expected to achieve the required similarity to reference systems. For regions in which environmental conditions are highly variable from year to year, the time frame will probably be long. At least one project in San Francisco Bay has a 20-year monitoring requirement; this is appropriate for a wetland subject to highly variable inflows of fresh water.
-
Criteria and methods used to assess the restoration site should be able to stand up to peer review.
Detailed standards for assessing successful restoration have not been developed. The need is most urgent where restoration is carried out as mitigation — that is where functional equivalency must be
TABLE 6.4 Ecosystem Attributes to Consider in Assessing the Functional Equivalency of Constructed and Natural Wetlands Based on Experience in Coastal Salt Marshes
Function |
Suggested Measures |
Hydrologic function |
• Ground water recharge: Monitor water level in nearby wells. |
• Shoreline stabilization: Map shorelines from aerial photographs or install and monitor markers. |
|
• Flood-peak reduction: Monitor water levels in relation to flow velocity. |
|
• Restoration tidal flows: Monitor water levels over tide cycles; determine amplitude; lags; monitor salinity of water and soil. |
|
• Development of hydrologic equilibria: Measure erosion and accretion of channels and marsh. |
|
• Sample inflowing waters for nutrient concentrations (N, P) and flow rates. |
|
Nutrient supply functions and their limiting factors |
• Analyze soil texture and organic matter content. |
• Determine nutrient concentrations (N, P) in soil and pore water. |
|
• Survey for toxic substances (heavy metals, selenium, and others.) |
|
Persistence of the plant community |
• Determine cover of dominant species and map using aerial photographs and ground truthing. |
• Survey populations of sensitive species quantitatively. |
|
• Determine the life history characteristics of sensitive plant populations to predict their ability to persist in the restored wetland (e.g., numbers, flowering, seed production, seed germination potential, seedling establishment, and successful recruitment). |
|
Plant growth a and its limiting factors |
• Measure end-of-season live standing crop (EOSL); estimate biomass by measuring total stem length (meters per square meter) of species such as cordgrass. |
• Measure redox potential in soil profiles, and measure pH. |
|
• Assess/monitor organic matter decomposition. |
|
• Assess cover of floating or epibenthic algae by dominant type. |
|
• Determine nutrient content of inflowing waters. |
met to comply with the emerging policy of no net loss in wetland acreage and function. Recommendations have been put forth for southern California (PERL, 1990), where mitigation is driving many restoration projects and where several species are endangered due to past losses of habitat.
Need to Accelerate the Restoration Process
Wherever wetlands are restored to mitigate damage to mature wetlands, there will likely be a net loss in functioning while the younger system develops biomass and complexity. There should be ways to speed the development of ecosystem functions (often called ecological
succession) in restoration sites, thus shortening the time required to attain maturity. Mature vegetated wetlands have fine-textured soil that is rich in organic matter and nutrients, a well-developed rhizosphere and dense canopy of native vegetation, and a wide range of native arthropods and larger animals. In contrast, a newly developing marsh, such as would be found on a sandbar or an alluvial outwash, would have mineral soil, low plant cover, and few species of animals. The quality of the substrate in graded sites (i.e., soils that are coarse, low in nutrients, and lacking in organic matter) may slow succession.
Most transplantation attempts have concentrated on dominant species of plants. Interestingly, data from the Corvallis EPA Environmental Research Laboratory (M. Brown, University of Florida, and S. Gwin, U.S. Environmental Protection Agency Corvallis Laboratory, personal communications, June 1990) show that almost all of the vegetation present on created freshwater marshes in Oregon and Florida did not include species on the transplant lists. Although it is not clear whether the listed species were ever planted, it is clear that planners did not know what species would succeed on the sites. Note that plants acquired from the wild to accelerate restoration may degrade the site they were taken from, resulting in a net loss of wetland quality.
Various techniques for accelerating the rate of ecosystem development are currently emerging:
-
sculpturing the site to add topographic heterogeneity and provide microsites for enhanced microbial, arthropod, fish, and bird activities, at least in tidal marshes;
-
mulching the site with litter, detritus, seed, and root materials from native wetlands to provide organic matter and propagules (cf. Clewell and Lea, 1989); and
-
augmenting the soil (tilling in organic matter or mineral nutrients) to stimulate plant growth and shorten the time needed to provide dense cover. This should also enhance microbial processes (e.g., nitrogen fixation) and nutrient recycling rates. Potential pitfalls are high foliar nitrogen concentrations that may stimulate excessive herbivory. Research is proceeding through the California Sea Grant Program and the National Oceanic and Atmospheric Administration's Coastal Ocean Program to develop this ecotechnology for coastal wetlands.
Need for Research
Several regional reviews and conferences have considered the status of restoration knowledge (Josselyn, 1982; Lewis, 1982; Strickland, 1986; Larson and Niell, 1987; Kusler et al., 1988; Zelazny and Feierabend,
1988; Kusler and Kentula, 1989; Conners et al., 1990). This committee's review of research needs highlights the broad areas requiring further study and indicates the opportunities afforded by restoration projects. A more detailed statement would require substantial regionalization and consideration of the diversity of wetland types. For example, the review carried out by Conners et al. (1990) is a 60-page book that deals with only one wetland type in one part of the nation for one purpose—research needed to mitigate salt marsh that will be lost as highways are improved. The authors provide lists of research topics in each of the following areas: hydrodynamic processes, soil development, vegetation establishment, monitoring, spatial requirements, construction and equipment, and storm water treatment.
APPLIED RESEARCH
The practice of restoration must move from a trial-and-error process to a science—it needs to become predictive (Zedler and Weller, 1989). We need to know what ecosystem functions can be restored under various conditions (i.e., constraints) and how rapidly restoration can proceed. The curve for functional development over time cannot be plotted, because there are not enough data to indicate either its slope or its asymptote. A variety of approaches can work together to bring restoration into the area of science, given access to restoration (and reference) sites as well as funding opportunities:
-
The requirement for careful long-term monitoring of selected restoration projects will fill gaps in information on how ecosystem functions develop. An understanding of how early and late restoration conditions compare will lead to predictive power that will help in future projects.
-
A major need is to develop scientifically defensible standards for assessing the degree of restoration achieved. Not only is it important to have appropriate comparison data sets (reference wetlands), it is also essential that suitable sampling protocols be developed. Restoration success is usually interpreted as the establishment of vegetation that covers a specified percentage of the site for a defined period of time. Such measures do not evaluate whether a project is functioning properly or if it will persist. A mandate to develop standard assessment methods will stimulate comparisons of different sampling and measurement techniques, with the result that science will gain deeper understanding of how methods affect data, as well as how data are influenced by the type of ecosystem under study.
-
The gradual accumulation of baseline studies of wetland ecosystem
-
functioning will provide opportunities for comparison of different system types among various regions, and at different ages of development. Regional and national data bases would facilitate such comparisons. Syntheses of existing knowledge, especially papers in the "gray" literature (permit files, records of managers), are also needed.
-
The availability of restoration sites for experimental work would have great potential for answering research questions (Gross, 1987). Determining the relationship between various plant and animal species is very approachable on sites where single-species vegetative cover can be planted with or without animal associates. Testing the development of ecosystems with different hydrologic, substrate, and biologic features is feasible where these attributes can be part of the restoration design. The potential is limited only by one's scientific creativity.
-
The insistence on an adaptive management approach would facilitate studies of how management alternatives reduce or compensate for the impacts of sedimentation, erosion, eutrophication, contamination, and human disturbance.
CONCLUSIONS AND RECOMMENDATIONS
Planning
The piecemeal approach to restoration has not been efficient (economically or ecologically) or effective in achieving broader goals for restoring aquatic systems. The specific goals and objectives for a restoration site are often established in a vacuum. The following recommendations are directed to any person, state, or federal agency involved in a wetland restoration effort.
-
Use a landscape-level approach (at the biogeographic region or watershed scale), with advance identification of sites that will provide the largest gains after restoration.
-
Inventory biogeographic regions and establish sites with restoration potential. Develop priorities for restoration within each region.
-
Integrate the restoration project with the rest of the landscape; use regional and watershed approaches in setting restoration objectives.
-
Incorporate long-term studies, new findings, and local expertise into an adaptive management program for the restoration site within its regional context. Expect problems and be prepared to
-
provide rapid responses and solutions (e.g., for trash removal, exotic species invasions, sedimentation and erosion events).
-
Funding priority should be given to programs for restoration of damaged wetlands over wetlands creation because of the superior chances of success. An exception would be where restoration is part of a mitigation agreement that would result in a net loss of acreage.
-
Give whole-ecosystem restoration and restoration for nongame species priority over restoration to support game species. The goal should not espouse single-species management.
-
Retain habitat remnants (e.g., small, fragmented wetlands) in urban areas, for use in education, research, recreation, and aesthetic enjoyment.
-
An appropriate federal agency should prepare periodic ''state of the wetlands" reports.
Research Needs and Techniques
Traditional research on wetlands and ecosystem development should also be continued, using both natural and restored wetlands. Examples of this traditional research include the following topics mentioned by several EPA authors (modified from Kusler and Kentula, 1989):
-
the hydrologic needs and requirements of wetland plants and animals, including minimum water depths, hydroperiod, velocity, dissolved nutrients, the role of large-scale but infrequent events such as floods, and the effects of long-term fluctuations in water levels;
-
the importance and functional significance of substrate to wetland plants and animals, and to chemical and biological functions;
-
characteristics of development rates for natural successional vegetation;
-
recolonization of restored sites by invertebrate and vertebrate fauna;
-
functions of wetlands, with special emphasis on habitat values for a broad range of species, food chain support, and water quality enhancement;
-
evaluation of the stability and persistence of wetland ecosystems; and
-
evaluation of the impact of sediment deposition or erosion, nutrient loading or removal, toxic runoff, pedestrian and off-road vehicle use, grazing, and other impacts on wetland structure and function.
Additional research needs concern characterizing the role of microbes. Bacteria and fungi are important to nutrient cycles, acting as both facilitators and competitors for plant nutrient uptake. Cooke and Lefor (1990) sampled planted and natural salt marsh soils at Indian River marsh in Connecticut and observed that vesicular-arbuscular mycorrhizae (fungi that help plants take up nutrients) were absent in the transplanted site but present in nearly every natural marsh sample. The role of mycorrhizae in wetland soils is not at all clear, and restoration sites offer opportunities to carry out field experiments in this area. The potential for improving transplant growth by manipulating soil microbial communities needs to be explored. Other bacteria perform important "bioremediation" functions. By decomposing contaminants, they may reduce or eliminate the effects of toxic waste spills, such as gasoline seepage from aging underground tanks (R. Gersberg, San Diego State University, personal communication, 1990). Finally, the microbes of wetlands are important to global carbon, sulfur, and nitrogen cycles, and their ability to perform these roles in restored wetlands has barely been explored (Cantilli, 1989).
We also need to know what ecosystem functions can be restored under various constraints and how rapidly restoration can proceed. A variety of specific techniques may be used to restore physical features and ecological functions of wetlands, depending on wetland type, disturbance condition, and other constraints.
-
Develop innovative methods of accelerating the restoration process (e.g., better propagation techniques for native plant species), surveys and protocols for obtaining adequate genetic diversity in the transplant material, soil augmentation procedures to shorten time to obtain the desired vegetative cover, ways to use microbes to detoxify contaminants (bioremediation) and enhance nutrient availability, and methods for controlling exotic species.
-
Design and conduct experimental research programs to examine restoration techniques and functional development over time in different system types.
-
Use restoration sites for scientific experiments that are designed to accelerate the restoration process.
-
Support baseline studies of wetland ecosystem functioning to provide comparisons of different system types among various regions and at different stages of development. Establish regional and national data bases.
REFERENCES AND RECOMMENDED READING
Aberle, B. 1990. The Biology, Control, and Eradication of Introduced Spartina (Cordgrass) Worldwide and Recommendations for Its Control in Washington. Draft report. Washington State Department of Natural Resources, Seattle. 88 pp.
Adamus, P. R., and L. T. Stockwell. 1983. A Method for Wetland Functional Assessment. Vol. 1. Federal Highway Administration Report No. FHWA-IP-82-23. U.S. Department of Transportation, Washington, D.C.
Allen, J. A., and H. E. Kennedy, Jr. 1989. Bottomland Hardwood Reforestation in the Lower Mississippi Valley. U.S. Fish and Wildlife Service, Slidell, La. and U.S. Forest Service, Southern Forest Experiment Station, Stoneville, Miss. 28 pp.
Atwater, B. F. 1979. History, landforms, and vegetation of the estuary's tidal marshes. In T. J. Conomos, ed., San Francisco Bay, The Urbanized Estuary. American Association for the Advancement of Science, Pacific Division, San Francisco, Calif.
Belt, C. B., Jr. 1975. The 1973 flood and man's constriction of the Mississippi River. Science 189:681-684.
Berger, J., ed. 1990. Ecological Restoration in the San Francisco Bay Area: A Descriptive Directory and Source Book. Restoring the Earth, Inc., Berkeley, Calif.
Best, G. R. 1987. Natural wetlands-southern environment: Wastewater to wetlands, Where do we go from here? Pp. 99–120 in K. R. Reddy and W. H. Smith, eds., Aquatic Plants for Water Treatment and Resource Recovery. Magnolia Publishing, Orlando, Fla.
Best, G. R., J. R. Tuschall, P. L. Brezonik, J. R. Butner, W. F. DeBusk, K. C. Ewel, A. Hernandez, and H. T. Odum. 1982. The Fate of Selected Heavy Metals in a Forested Wetland Ecosystem. Report to U.S. Environmental Protection Agency. Center for Wetlands, University of Florida, Gainesville, Fla.
Bradshaw, A. D. 1988. Alternative endpoints for reclamation. Pp. 69–85 in J. Cairns, Jr., ed., Rehabilitating Damaged Ecosystems. Vol. II. CRC Press, Boca Raton, Fla.
Broome, S. W. 1989. Creation and restoration of tidal wetlands of the southeastern United States. Pp. 37-72 in J. A. Kusler and M. E. Kentula, eds., Wetland Creation and Restoration: The Status of the Science. Vol. I. U.S. EPA/7600/3-89/038. U.S. Environmental Protection Agency, Environmental Research Laboratory, Corvallis, Ore.
Broome, S. W., C. B. Craft, and E. D. Seneca. 1987. Creation and development of brackish-water marsh habitat. Pp. 197-205 in J. Zelanzny and J. S. Feierabend, eds., Increasing Our Wetland Resources. Conference Proceedings, National Wildlife Federation Corporate Conservation Council, October 4–7, 1987. National Wildlife Federation, Washington, D.C.
Cairns, J., ed. 1988. Rehabilitating Damaged Ecosystems. Vol. 1, 192 pp.; Vol. 2, 222 pp. CRC Press, Boca Raton, Fla.
Cammen, L. M. 1976a. Abundance and production of macroinvertebrates from natural and artificially established salt marshes in North Carolina. Am. Midl. Nat. 96:244–-253.
Cammen, L. M. 1976b. Macroinvertebrate colonization of Spartina marshes artificially established on dredge spoil. Coastal Mar. Sci. 4:357–372.
Cantilli, J. F. 1989. Sulfide Phytotoxicity in Tidal Salt Marshes. M.S. thesis, San Diego State University.
Carlton, J. T. 1989. Man's role in changing the face of the ocean: Biological invasions and implications for conservation of near-shore environments. Conserv. Biol. 3:265–273.
Carothers, S. W., G. S. Mills, and R. R. Johnson. 1989. The creation and restoration of
riparian habitat in southwestern arid and semi-arid regions. Pp. 359–376 in J. A. Kusler and M. E. Kentula, eds., Wetland Creation and Restoration: The Status of the Science . Vol. I. U.S. EPA/7600/3089/038. U.S. Environmental Protection Agency, Environmental Research Laboratory, Corvallis, Ore.
Chabreck, R. H. 1989. Creation, restoration, and enhancement of marshes of the northcentral Gulf Coast. Pp. 127-144 in J. A. Kusler and M. E. Kentula, eds., Wetland Creation and Restoration: The Status of the Science. Vol. I. U.S. EPA/7600/3089/038. U.S. Environmental Protection Agency, Environmental Research Laboratory, Corvallis, Ore.
Clean Water Act of 1977. P.L. 95–217, Dec. 27, 1977, 91 Stat. 1566.
Clewell, A. F., and R. Lea. 1989. Creation and restoration of forested wetland vegetation in the southeastern United States. Pp. 199–238 in J. A. Kusler and M. E. Kentula, eds., Wetland Creation and Restoration: The Status of the Science. Vol. I. U.S. EPA/7600/3089/038. U.S. Environmental Protection Agency, Environmental Research Laboratory, Corvallis, Ore.
Coastal Wetlands Planning, Protection and Restoration Act of 1990. P.L. 101–646, Nov. 29, 1990.
Conner, W. H., and J. R. Toliver. 1990. Observations on the regeneration of baldcypress (Taxodium distichum( L.) Rich.) in Louisiana swamps. South. J. Appl. For. 14:115–118.
Conners, D. H., F. Riesenberg, R. D. Charney, M. A. McEwen, R. B. Krone, and G. Tchobanoglous. 1990. Research Needs: Salt Marsh Restoration, Rehabilitation, and Creation Technique for Caltrans Construction Projects. Department of Civil Engineering, University of California, Davis. 60 pp.
Cooke, J. C., and M. W. Lefor. 1990. Comparison of vesicular-arbuscular mycorrhizae in plants from disturbed and adjacent undisturbed regions of a coastal salt marsh in Clinton, Connecticut, USA. Environ. Manage. 14:131–137.
Council on Environmental Quality (CEQ). 1989. Environmental Trends—Chapter 5: Wetlands and wildlife. Office of the President, Council on Environmental Quality, Washington, D.C. 152 pp.
Covin, J. D., and J. B. Zedler. 1988. Nitrogen effects on Spartina foliosa and Salicornia virginica in the salt marsh at Tijuana Estuary, Calif. Wetlands 8:51–65.
Cowardin, L. M., V. Carter, F. C. Golet, and E. T. LaRoe. 1979. Classification of Wetlands and Deepwater Habitats of the United States. Office of Biological Services, Fish and Wildlife Service, U.S. Department of the Interior, Washington, D.C.
Craft, C. B., S. W. Broome, and E. D. Seneca. 1988. Nitrogen, phosphorus and organic carbon pools in natural and transplanted marsh soils. Estuaries 11:272–280.
Dahl, T. E. 1990. Wetland Losses in the United States: 1780's to 1980's. U.S. Department of the Interior, Fish and Wildlife Service, Washington, D.C. 21 pp.
DeWald, J. M., and J. P. Rieger. 1982. Restoration of a Degraded Salt Marsh: Objectives and Techniques. Unpublished report. California Department of Transportation, Caltrans District 11, San Diego. 10 pp.
Erwin, K. L. 1989. Freshwater marsh creation and restoration in the southeast. Pp. 239-272 in J. A. Kusler and M. E. Kentula, eds., Wetland Creation and Restoration: The Status of the Science. Vol. I. U.S. EPA/7600/3-89/038. U.S. Environmental Protection Agency, Environmental Research Laboratory, Corvallis, Ore.
Ewel, J. 1987. Restoration is the ultimate test of ecological theory. Pp. 31–33 in W. Jordan, M. Gilpin, and J. Aber, eds., Restoration Ecology: A Synthetic Approach to Ecological Research. Cambridge University Press, New York.
Federal Interagency Committee for Wetland Delineation. 1989. Federal Manual for Identifying and Delineating Jurisdictional Wetlands. Cooperative technical publication. U.S. Army Corps of Engineers, U.S. Environmental Protection Agency, U.S.
Fish and Wildlife Service, and U.S. Department of Agriculture. Soil Conservation Service, Washington, D.C. 76 pp. plus appendices.
Fink, B., and J. Zedler. 1990. Endangered plant recovery: Experimental approaches with Cordylanthus maritimus ssp. maritimus. Pp. 460–468 in H. G. Hughes and T. M. Bonnicksenm, eds., Restoration '89: The New Management Challenge. Society for Ecological Restoration, Madison, Wis.
Fonseca, M. S. 1989. Regional analysis of the creation and restoration of seagrass systems. Pp. 175–198 in J. A. Kusler and M. E. Kentula, eds., Wetland Creation and Restoration: The Status of the Science. Vol. I. U.S. EPA/7600/3-89/038. U.S. Environmental Protection Agency, Environmental Research Laboratory, Corvallis, Ore.
Fonseca, M. S., W. J. Kenworthy, and G. W. Thayer. 1988. Restoration and management of seagrass systems: A Review. Pp. 353–368 in D. D. Hook, W. H. McKee, Jr., H. K. Smith, J. Gregory, V. G. Burrell, Jr., M. R. DeVoe, R. E. Sojka, S. Gilbert, R. Banks, L. H. Stolzy, C. Brooks, T. D. Matthews, and T. H. Shear, eds., The Ecology and Management of Wetlands. Vol. 2. Timber Press, Portland, Ore.
Frenkel, R. E., and J. C. Morlan. 1990. Restoration of the Salmon River Salt Marshes: Retrospect and Prospect. Final Report to the U.S. Environmental Protection Agency. Seattle, Wash.
Glenn, E. P., J. W. O'Leary, M. C. Watson, T. L. Thompson, and R. O. Kuehl. 1991. Salicornia bigelovii Torr.: An oilseed halophyte for seawater irrigation. Science 251(4997):1065–1067.
Gosselink, J. G., and L. C. Lee. 1989. Cumulative impact assessment in bottomland hardwood forests. Wetlands 9:83–174.
Gosselink, J. G., S. E. Bayley, W. H. Conner, R. E. Turner. 1981. Ecological factors in the determination of riparian wetland boundaries. Pp. 197–219 in J. B. Clark and J. Benforado, eds., Wetlands of Bottomland Hardwood Forest. Elsevier Science Publishing Co., New York.
Gosselink, J. G., W. H. Conner, J. W. Day, Jr., and R. E. Turner. 1989. Classification of wetland resources: Land, Timber, and Ecology. Pp. 28–48 in D. Jackson and J. L. Chambers, eds., Timber Harvesting in Wetlands. Division of Continuing Education, Louisiana State University, Baton Rouge, La.
Gosselink, J. G., L. C. Lee, and T. A. Muir. 1990a. Ecological Processes and Cumulative Impacts. Lewis Publishers, Chelsea, Mich. 708 pp.
Gosselink, J. G., G. P. Shaffer, L. C. Lee, D. M. Burdick, D. L. Childers, N. C. Leibowitz, S. C. Hamilton, R. Boumans, D. Cushman, S. Fields, M. Koch, and J. M. Visser. 1990b. Landscape conservation in a forested wetland watershed. BioScience 40(8): 588–600.
Greeson, P. B., J. R. Clark, and J. E. Clark, eds. 1979. Wetland Functions and Values: The State of Our Understanding. Proceedings of the National Symposium on Wetlands. American Water Resources Association, Minneapolis, Minn. 674 pp.
Gross, K. L. 1987. Mechanisms of colonization and species persistence in plant communities. Pp. 173–188 in W. Jordan, M. Gilpin, and J. Aber, eds., Restoration Ecology: A Synthetic Approach to Ecological Research. Cambridge University Press, New York.
Hackensack Meadowland Reclamation and Development Act (HMRDA). 1968. State of New Jersey Statutes. Chapter 17, Section 13:17-1 to 13-17-86.
Haltiner, J. 1990. Sweetwater Marsh: Morphology and tidal circulation. Philip Williams & Assoc., Ltd. Report Prepared for Caltrans District 11, San Diego, and Pacific Estuarine Research Laboratory, San Diego State University, San Diego, Calif.
Harris, L. D. 1988. The nature of cumulative impacts on biotic diversity of wetland vertebrates. Environ. Manage. 12:675–693.
Hartman, J. M. 1988. Recolonization of small disturbance patches in a New England salt marsh. Am. J. Bot. 75:1625–1631.
Hollands, G. G. 1989. Regional analysis of the creation and restoration of kettle and pothole wetlands. Pp. 287–304 in J. A. Kusler and M. E. Kentula, eds., Wetland Creation and Restoration: The Status of the Science. Vol. I. U.S. EPA/7600/3-89/038. U.S. Environmental Protection Agency, Environmental Research Laboratory, Corvallis, Ore.
Hook, D., D., W. H. McKee, Jr., H. K. Smith, J. Gregory, V. G. Burrell, Jr. , M. R. DeVoe, R. E. Sojka, S. Gilbert, R. Banks, L. H. Stolzy, C. Brooks, T. D. Matthews, and T. H. Shear, eds. 1988. The Ecology and Management of Wetlands. Vol. 1, 592 pp.; Vol. 2, 394 pp. Timber Press, Portland, Ore.
Hunt, C. E. 1988. Down by the River: The Impacts of Federal Water Projects and Policies on Biological Diversity. Island Press, Washington, D.C.
Johnson, L. E., and W. V. McGuinness, Jr. 1975. Guidelines for Material Placement in Marsh Creation. Contract Report D-75-2 for Environmental Effects Laboratory, U.S. Army Corps of Engineers Waterways Experiment Station, Vicksburg, Miss. 189 pp.
Jorgensen, P. D. 1975. Habitat preference of the light-footed clapper rail in Tijuana Estuary Marsh, Calif. M.S. thesis, San Diego State University.
Josselyn, M., ed. 1982. Wetland Restoration and Enhancement in California. Sea Grant Technical Report No. T-CSGCP-007. Tiburon Center for Environmental Studies, Tiburon, Calif.
Josselyn, M. N., and J. W. Buchholz. 1984. Marsh Restoration in San Francisco Bay—A Guide to Design and Planning, Tech. Rept. #3, Tiburon Center for Environmental Studies, San Francisco State University, Tiburon, Calif.
Kahrl, W. L. 1979. The California Water Atlas. Governor's Office of Planning and Research and California Department of Water Resources, Sacramento, Calif.
King, D. M. 1990. A Framework for Evaluating Wetland Restoration Projects. April 1990 Draft report to Office of Policy, Planning and Evaluation, U.S. Environmental Protection Agency, Washington, D.C.
Kraus, M. L., and D. J. Smith. n.d. Competition and Succession in a Perturbed Urban Estuary: The Effects of Hydrology. Monograph. Hackensack Meadowlands Development Commission, Lyndhurst, N.J.
Kusler, J. A. 1983. Our National Wetland Heritage: A Protection Guidebook. Environmental Law Institute, Washington, D.C.
Kusler, J. A., and M. E. Kentula, eds. 1989. Wetland Creation and Restoration: The Status of the Science. Vol. I-II. U.S. EPA/7600/3-89/038. U.S. Environmental Protection Agency, Environmental Research Laboratory, Corvallis, Ore.
Kusler, J. A., M. L. Quammen, and G. Brooks, eds. 1988. Proceedings of the National Wetland Symposium: Mitigation of Impacts and Losses. Association of State Wetland Managers, Berne, N.Y.
Langis, R., M. Zalejko, and J. B. Zedler. 1991. Nitrogen assessments in a constructed and a natural salt marsh of San Diego Bay, California. Ecol. Appl. 1:40–51.
Larson, J. S. 1988. Wetland creation and restoration: An outline of the scientific perspective. Pp. 73-79 in J. Zelazny and J. S. Feierabend, eds., Increasing Our Wetland Resources. Conference Proceedings, National Wildlife Federation Corporate Conservation Council, October 4–7, 1987. National Wildlife Federation, Washington, D.C.
Larson, J. S., and C. Niell, eds. 1987. Mitigating Freshwater Wetland Alterations in the Glaciated Northeastern United States: An Assessment of the Science Base. Publ. 87-1. Environmental Institute, University of Massachusetts, Amherst.
Levine, D. A., and D. E. Willard. 1989. Regional analysis of fringe wetlands in the Midwest: creation and restoration. Pp. 305-332 in J. A. Kusler and M. E. Kentula,
eds., Wetland Creation and Restoration: The Status of the Science. Vol. I. U.S. EPA/7600/3-89/038. U.S. Environmental Protection Agency, Environmental Research Laboratory, Corvallis, Ore.
Lewis, R. R. III. 1982. Creation and Restoration of Coastal Plant Communities. CRC Press, Boca Raton, Fla.
Lewis, R. R. III. 1989. Creation and restoration of coastal plain wetlands in Florida. Pp. 73–102 in J. A. Kusler and M. E. Kentula, eds., Wetland Creation and Restoration: The Status of the Science. Vol. I. U.S. EPA/7600/3-89/038. U.S. Environmental Protection Agency, Environmental Research Laboratory, Corvallis, Ore.
Loftin, K. A., L. A. Toth, and J. T. B. Obeysekera. 1990. Kissimmee River Restoration: Alternative Plan Evaluation and Preliminary Design Report. South Florida Water Management District, West Palm Beach, Fla. June.
Loucks, O. L. 1989. Restoration of the pulse control function of wetlands and its relationship to water quality objectives. Pp. 55–65 in J. A. Kusler and M. E. Kentula, eds., Wetland Creation and Restoration: The Status of the Science, Vol. II. U.S. EPA/7600/3-89/038. U.S. Environmental Protection Agency, Environmental Research Laboratory, Corvallis, Ore.
Lowry, D. J. 1989. Restoration and creation of palustrine wetlands associated with riverine systems of the glaciated Northeast. Pp. 273–286 in J. A. Kusler and M. E. Kentula, eds., Wetland Creation and Restoration: The Status of the Science, Vol. I. U.S. EPA/7600/3-89/038. U.S. Environmental Protection Agency, Environmental Research Laboratory, Corvallis, Ore.
MacDonald, P. O., W. E. Frayer, and J. K. Clauser. 1979. Documentation, Chronology, and Future Projections of Bottomland Hardwood Habitat Losses in the Lower Mississippi Alluvial Plain. U.S. Department of the Interior, Fish and Wildlife Service , Washington, D.C., 2 vols.
Mitsch, W. J., and J. G. Gosselink. 1986. Wetlands. Van Nostrand Reinhold, New York. 539 pp.
Moy, L. D. 1989. Are Spartina Marshes Renewable Resources? A Faunal Comparison of a Man-Made Marsh and Two Adjacent Natural Marshes. M. S. thesis, North Carolina State University, Raleigh.
Newling, C. J., and M. C. Landin. 1985. Long-Term Monitoring of Habitat Development at Upland and Wetland Dredge Material Disposal Sites, 1974–1982. Tech. Rept. D-85-5. U.S. Army Corps of Engineers Waterway Experiment Station, Vicksburg, Miss.
Nichols, F. H., J. E. Cloern, S. N. Luoma, and D. H. Paterson. 1986. The Modification of an Estuary. Science 231:567–573.
Niering, W. A. 1986. Wetlands. The Audubon Society. Alfred A. Knopf, New York. 538 pp.
Niering, W. A. 1989. Vegetation dynamics in relation to wetland creation. Pp. 67–74 in J. A. Kusler and M. E. Kentula, eds., Wetland Creation and Restoration: The Status of the Science. Vol. II. U.S. EPA/7600/3-89/038. U.S. Environmental Protection Agency, Environmental Research Laboratory, Corvallis, Ore.
Nordby, C. S., and J. B. Zedler. 1991. Responses of fish and marcobenthic assemblages to hydrologic disturbances in Tijuana Estuary and Los Pe:asquitos Lagoon, California. Estuaries 14:80–93.
Novitzki, R. P. 1979. Hydrologic characteristics of Wisconsin's wetlands and their influence on floods, stream flow, and sediment. In P. E. Greeson, J. R. Clark, and J. E. Clark, eds., Wetland Functions and Values: The State of Our Understanding. American Water Resources Association, Minneapolis, Minn. 377 pp.
Odum, W. E. 1988. Predicting ecosystem development following creation and restoration of wetlands. Pp 67–70 in J. Zelazny and J. S. Feierabend, eds., Increasing
Our Wetland Resources. Conference Proceedings, National Wildlife Federation Corporate Conservation Council, October 4–7, 1987. National Wildlife Federation, Washington, D.C.
Onuf, C. P., and M. L. Quammen. 1985. Coastal and riparian wetlands of the Pacific region: The state of knowledge about the food chain support. Pp. 98–173 in Proceedings of National Wetland Technical Council Workshop, April 14–16, Mill Valley, Calif.
Onuf, C. P., M. L. Quammen, G. P. Shaffer, C. H. Peterson, J. W. Chapman, J. Cermak, and R. W. Holmes. 1978. An analysis of the values of central and southern California coastal wetlands. Pp. 186–199 in P. E. Greeson, J. R. Clark, and J. E. Clark, eds., Wetland Functions and Values: The State of Our Understanding. American Water Resources Association, Minneapolis, Minn.
Pacific Estuarine Research Laboratory (PERL). 1990. A Manual for Assessing Restored and Natural Coastal Wetlands with Examples from Southern California. California Sea Grant Report No. T-CSGCP-021. California Sea Grant Program, La Jolla, Calif. 105 pp.
Park, R. A., M. S. Trehan, P. W. Mausel, and R. C. Howe. 1988. The Effects of Sea Level Rise on U.S. Coastal Wetlands. HRI Report No. 142. Holcomb Research Institute, Butler University Indianapolis. 60 pp.
Park, R. A., M. S. Trehan, P. W. Mausel, and R. C. Howe. 1989. Coastal wetlands in the twenty-first century: Profound alterations due to rising sea level. Pp. 71–80 in Wetlands: Concerns and Successes. Proceedings of the American Water Resources Association, September, Tampa, Fla.
Parnell, J. F., D. M. DuMond, and R. N. Needham. 1978. A Comparison of Plant Succession and Bird Utilization on Diked and Undiked Dredged Material Islands in North Carolina Estuaries. U. S. Army Corps of Engineers Waterways Experiment Station, Vicksburg, Miss.
Peters, R. L., II. 1988. The effect of global climatic change on natural communities. Pp. 450–461 in E. O. Wilson, ed., Biodiversity. National Academy Press, Washington, D.C.
Pontius, F. W. 1990. Federal laws protecting wetlands. J. AWWA 82:12–16.
Power, M. E. 1990. Effects of fish in river food webs. Science 250:811–814.
Prouty, W. F. 1952. Carolina bays and their origin. Bull. Geol. Soc. Am. 63:167–224.
Quammen, M. L. 1986. Measuring the success of wetlands mitigation. Natl. Wetl. Newsl. 8:6–8.
Reitze, A. W., Jr. 1974. Wetlands, special problems in land use control. Chapter 2 in Environmental Planning: Law of Land and Resources. North American International, Washington, D.C. 941 pp.
Richardson, C. J. 1985. Mechanisms controlling phosphorus retention capacity in freshwater wetlands. Science 228:1424–1427.
Roberts, T. H. 1989. Habitat value of man-made coastal marshes in Florida. Pp. 154–179 in F. J. Webb, ed., Proceedings of the 16th Annual Conference on Wetlands Restoration and Creation. Hillsborough Community College, Tampa. Fla.
Rutherford, S. E. 1989. Detritus Production and Epibenthic Communities of Natural Versus Constructed Salt Marshes. M.S. thesis, San Diego State University.
Sacco, J. N. 1989. Infaunal Community Development of Artificially Established Salt marshes in North Carolina. M.S. thesis, North Carolina State University, Raleigh, N.C.
Sacco, J. N., F. L. Booker, and E. D. Seneca. 1987. Comparison of the macrofaunal communities of a human-initiated salt marsh at two and fifteen years of age. Pp. 282–285 in J. Zelazny and S. Feierabend, eds., Increasing Our Wetland Resources. Conference Proceedings, National Wildlife Federation Corporate Conservation Council, October 4-7, 1987. National Wildlife Federation, Washington, D.C.
San Francisco Estuary Project. 1990. Status and Trends Report on Pollutants in the San Francisco Estuary. Prepared under EPA Cooperative Agreement CE-009496-01 by the San Francisco Bay-Delta Aquatic Habitat Institute, 180 Richmond Field Station, 1301 South 46th Street, Richmond, CA 94804.
Saucier, R. T., C. C. Calhoun, Jr., R. M. Engier, T. R. Patin, and H. K. Smith. 1978. Executive Overview and Detailed Summary: Dredged Material Research Program. Tech. Rept. DS-78-212. U.S. Army Corps of Engineers Waterway Experiment Station, Vicksburg, Miss.
Schalles, J. F., R. R. Sharitz, J. W. Gibbons, G. J. Leversee, and J. N. Knox. 1989. Carolina Bays of the Savannah River Plant. SRO-NERP-18.U.S. Department of Energy, Washington, D.C. 70 pp.
Seneca, E. D., and S. W. Broome. In press. Proceedings of a NOAA Restoration Symposium. Invited Talk. September 1990.
Sharitz, R. R., and J. W. Gibbons. 1982. The Ecology of Southeastern Shrub Bogs (Pocosins) and Carolina Bays: A Community Profile. FWS/OBS-82/04. Division of Biological Services, U.S. Fish and Wildlife Service, Washington, D.C. 93 pp.
Shisler, J. K. 1989. Creation and restoration of the coastal wetlands of the northeastern United States. Pp. 145–174 in J. A. Kusler and M. E. Kentula, eds., Wetland Creation and Restoration: The Status of the Science. Vol. I. U.S. EPA/7600/3-89/038. U.S. Environmental Protection Agency, Environmental Research Laboratory, Corvallis, Ore.
Shisler, J. K., and D. J. Charette. 1984. Evaluation of artificial salt marshes in New Jersey. New Jersey Agricultural Experiment Station Publ. No. P-40502-01-84. New Brunswick, N.J. 160 pp.
Skinner, J. 1962. A Historical Review of the Fish and Wildlife Resources of the San Francisco Bay Area. Report 1. Water Projects Bureau, California Department of Fish and Game, Sacramento, Calif.
Smith, R. L. 1980. Ecology and Field Biology. 3rd ed. Harper and Row, New York. 835 pp.
Spicher, D., and M. Josselyn. 1985. Spartina (Gramineae) in northern California: Distribution and taxonomic notes. Madroño 32:158-167.
Stanley, J. T. 1989. Riparian wetland creation and restoration in the far west: A compilation of information. Pp. 417–464 in J. A. Kusler and M. E. Kentula, eds., Wetland Creation and Restoration: The Status of the Science, Vol. I. U.S. EPA/7600/3-89/038. U.S. Environmental Protection Agency, Environmental Research Laboratory, Corvallis, Ore.
Strickland, R., ed. 1986. Wetland Functions, Rehabilitation, and Creation in the Pacific Northwest. Washington State Department of Ecology, Olympia, Wash.
Swift, K. L. 1988. Salt marsh restoration: Assessing a southern California example. M.S. thesis, San Diego State University. 84 pp.
Tax Reform Act of 1986. P.L. 99–514, 100 Stat. 2085 (1986), 49 U.S.C. § 16 et seq.
The Conservation Foundation. 1988. Protecting America's Wetlands: An Action Agenda . Final Report of the National Wetlands Policy Forum. The Conservation Foundation, Washington, D.C. 69 pp.
Thompson, Justice Gordon, Jr. 1988. Memorandum Decision and Order. Sierra Club and League for Coastal Protection v. John O. Marsh, Secretary of the Army, et al. U.S. District Court, Southern District of California. Civil No. 86-I942-GT(IEG), May 13, San Diego, Calif.
Tiner, R. W., Jr. 1984. Wetlands of the United States: Current Status and Recent Trends. National Wetlands Inventory. U.S. Department of the Interior, Fish and Wildlife Service, Washington, D.C. 59 pp.
Tripp, J. T. B., and M. Herz. 1988. Wetland preservation and restoration: Changing federal priorities. Va. J. Nat. Resour. Law 7:221–275.
Tuschall, J. R., Jr. 1981. Heavy Metal Complexation with Naturally Occurring Organic Ligands in Wetland Ecosystems. Ph.D. dissertation, University of Florida, Gainesville, Fla. 212 pp.
U.S. Army Corps of Engineers. 1972. Charles River Watershed, Massachusetts. New England Division, Waltham, Mass. 65 pp.
U.S. Army Corps of Engineers and U.S. Environmental Protection Agency. 1990. Memorandum of Agreement Regarding Mitigation for Dredged or Fill Material Disposal in Wetlands. February 6.
U.S. Fish and Wildlife Service (FWS). 1988. Biological Opinion 1-1-78-F-14-R2. The combined Sweetwater River food control and highway project, San Diego Country, California. Letter from Walley Steuke, Acting Regional Director. U.S. FWS, Portland, Oregon, to Colonel Tadahiko Ono, District Engineer, Los Angeles District. U.S. Army Corps of Engineers, March 30. 28 pp. plus appendices.
U.S. Fish and Wildlife Service (FWS). 1990. Wetlands: Meeting the President's Challenge. 1990 Wetland Action Plan. U.S. FWS, Washington, D.C. 64 pp.
Walters, C. 1986. Adaptive Management of Renewable Resources. Macmillan, New York. 374 p.
Water Resources Development Act of 1986. P.L. 99–662, Nov. 17, 1985, 100 Stat. 4082.
Willard, D. E., V. M. Finn, D. A. Levine, and J. E. Klarquist 1989. Creation and restoration of riparian wetlands in the agricultural midwest. Pp. 333–358 in J. A. Kusler and M. E. Kentula, eds., Wetland Creation and Restoration: The Status of the Science. Vol. I. U.S. EPA/7600/3-89/038. U.S. Environmental Protection Agency, Environmental Research Laboratory, Corvallis, Ore.
Williams. S. L. 1990. Experimental studies of Caribbean seagrass bed development. Ecol. Monogr. 60:449–469.
Woodhouse, W. W., Jr., E. D. Seneca, and S. W. Broome. 1974. Propagation of Spartina alterniflora for Substrate Stabilization and Salt Marsh Development. Coastal Engineering Research Center, U.S. Army Corps of Engineers, Fort Belvoir, Va.
Zalejko, M. K. 1989. Nitrogen Fixation in a Natural and a Constructed Southern California Salt Marsh. M.S. thesis, San Diego State University.
Zedler, J. B. 1988a. Restoring diversity in salt marshes: Can we do it? Pp. 317–325 in E. O. Wilson, ed., Biodiversity. National Academy Press, Washington, D.C.
Zedler, J. B. 1988b. Salt marsh restoration: Lessons from California. Pp. 123–138 in J. Cairns, ed., Rehabilitating Damaged Ecosystems. CRC Press, Boca Raton, Fla.
Zedler, J. B. 1988c. Why it's so difficult to replace lost wetland functions. Pp. 121–123 in J. Zelazny and J. S. Feierabend, eds., Increasing Our Wetland Resources. Conference Proceedings, National Wildlife Federation Corporate Conservation Council, October 4–7, 1987. National Wildlife Federation, Washington, D.C.
Zedler, J. B. 1991. The challenge of protecting endangered species habitat along the southern California coast. Coast. Manage. 19:35–53.
Zedler, J. B., and R. Langis. 1991. Urban wetland restoration: A San Diego Bay example. Proceedings: Third Annual "Country in the City" Symposium, Portland, Ore., April 1990. Audubon Society of Portland.
Zedler, J. B., and M. W. Weller. 1989. Overview and future directions. Pp 465-473 in J. A. Kusler and M. E. Kentula, eds., Wetland Creation and Restoration: The Status of the Science. Vol. I. U.S. EPA/7600/3-89/038. U.S. Environmental Protection Agency, Environmental Research Laboratory, Corvallis, Ore.
Zedler, J. B., R. Langis, J. Cantilli, M. Zalejko, K. Swift, and S. Rutherford. 1988. Assessing the functions of mitigation marshes in southern California. Pp. 323–330 in J.
A. Kusler, S. Daly, and G. Brooks, eds., Proceedings of the National Wetland Symposium, Urban, Wetlands Oakland, Calif. Association of State Wetland Managers, Berne, N.Y.
Zedler, P. H. 1987. The Ecology of Southern California Vernal Pools: A Community Profile. Biological Report 85 (7.11). U.S. Fish and Wildlife Service, Washington, D.C. 136 pp.
Zelazny, J., and J. S. Feierabend, eds. 1988. Increasing Our Wetland Resources. Conference Proceedings, National Wildlife Federation Corporate Conservation Council, October 4–7, 1987. National Wildlife Federation, Washington, D.C.