5
MANUFACTURING PROCESSES FOR ROTOR BLADES
CURRENT MANUFACTURING PROCESSES
This chapter focuses on those parameters that pertain to the manufacturing process for rotor blades. However, the design and the process are so interrelated that one can not be discussed without the other. Attention to local variations in resin content, fiber curvature, and very local matrix strains that result from the manufacturing process but that are inherent either in the design or the process are germane to understanding fatigue in composite structure.
Blade manufacturing methods have been discussed in depth in recent reports (Challis, 1987). Therefore, this discussion will only be sufficient to allow comparison with processes that are candidates for future blade manufacturing. Current processes will be discussed in two categories, manual and mechanized. The small and medium blades in the field now are largely made by manual processes, basically wet lay-up. Large blades have been made by filament and tape winding in glass-reinforced plastic, hand-laminated wood/epoxy, aluminum, and steel. The following is a brief discussion of the current methods for manufacturing composite blades in order that the relation of material choice, design, and manufacturing method to fatigue life may be presented.
Wet lay-up is a process where fiber, in the form of fabric, mat or roving, is placed in the tool and impregnated with the resin by hand. This process is labor intensive but offers considerable flexibility in placement and orientation of material. With respect to cost this can be the least-cost process available. Quality can vary from poor to excellent depending on the skill and interest of the worker. Resin content is difficult to control, which results in a weight and balance problem. Vacuum bag rub-out can improve laminate quality and aid in uniformity of resin distribution.
Prepreg lay-up, where the fiber form is impregnated by a supplier, is also a manual process but assures close control of resin content. Prepregs must have a reasonable shelf life even though the resin is already catalyzed, so cure is usually initiated by elevated temperature. Pressure is required for compaction, as air is easily trapped by the prepreg during lay-up. The cost of manufacturing is higher than for wet lay-up because of the need for heat and pressure in the curing process.
Blade skins made by manual processes are generally laid-up in one half shell and cured. Assembly bonding of the skins and spar, if required, is accomplished with paste adhesives. The two shells are sometimes cured as one piece in cavity-bonded tools using an internal bag to press the material out against the tool.
Filament and tape winding processes have been associated with a design approach utilizing a tubular spar for structural strength together with a lighter aerodynamic surface covering. The spar is wound first, leading and trailing edge mandrels are added, and winding is continued to completion. This process is relatively restricted in terms of fiber orientation and thickness in design, but it offers major reductions in labor with attendant uniformity in quality.
Tooling and facility requirements are minimal for the manual lay-up processes except where an autoclave is required for cure. Design options are maximized and costs are low. It is understandable then that manual processes
were chosen for start-up or developmental phases by wind turbine blade producers. However, there is a practical size limit imposed by either the risk of human error in a lengthy lay-up operation or just the risk of dealing with thickness and warpage variations that increase with scale. The greater tooling and facility costs of the mechanized winding process are justified when the processing risk is sufficiently great.
Wood/epoxy blades are made by laminating 0.10-inch-thick wood veneers together using an epoxy resin system. Sheets of length 2.4 m (8 feet) are coated with resin and placed in mold halves end on end from the root to tip. The outside plies are scarf jointed, but all inner plies are simply butt jointed in a staggered pattern. A fiberglass surface ply is used on both the inner and outer surfaces of the skin.
Once lay-up is completed, a vacuum bag system is installed and the laminate is formed to the mold by vacuum pressure. Cure is completed at room temperature in 2 to 8 hours depending on the size of the component. The blade skin is made in two halves, which are trimmed at the mating plane after the skins are cured. A root to tip shear web is made from quality plywood, and grooved blocks are bonded to both surfaces of the blade to receive the web on assembly. The two halves and the spar are bonded together with a filled epoxy.
The root end attachment is accomplished by bonding custom-designed studs into spanwise holes drilled in a thickened section of the spar. Bolts thread into these inserts for attachment of the blade to the hub. This process yields a lightweight, reliable quality blade at costs under $50/lb (Stoddard, 1989).
Blade Root End Concepts
The various types of root ends that were reviewed in Chapter 4 will be discussed here with relation to manufacturing considerations.
The Danish Hütter design turns a flange, in fiberglass, normal to the axis of the blade to allow attachment to a steel hub with tension bolts. The bolt holes are wrapped through 180° with roving that is brought from the shank
of the blade. This concept results in voids, resin pockets, and high resin stress. Metal collars trap this flange material as the attachment fasteners are tightened in an attempt to partially relieve the resin stresses, but field experience has shown this to be an unreliable concept.
U.S. designs utilize a metal flange on a tube that fits inside the fiberglass spar with bonding as the attachment method. The tube and spar are bonded together although fasteners may be added for their fail-safe benefits. The wall of the tube is tapered to better distribute load transfer in the bond. The tapered portion may also vary in diameter along the length to mechanically trap the blade should the bond fail. High matrix strains, which can limit blade life, are introduced at the outboard end of the metal taper because the metal cannot practically be tapered to a knife edge.
A third method employs bonded inserts for bolts that are aligned with the axis of the blade and attach directly to the mating flange. This latter method is used on the laminated wood and glass-reinforced plastic (GRP) blades and seems to be fabricable and reliable.
Manufacturing Methods Influence Blade Life
Manufacturing procedures can introduce conditions in the composite that greatly influence fatigue life. These are local resin content variations, local fiber curvature, and local residual stress. Such conditions are variables in all composite manufacturing processes and should be considered in design. A brief description of each of these potential problem areas follows.
Prior to cure, the viscosity of the resin is low enough that it can move around in the assembly due to the forces of gravity or slight differentials in bag pressure or fiber tension. The result is large variations in resin content. An area of low resin content will be damage prone and subject to microcracking under compressive transverse or out-of-plane loadings. Areas of high resin content or resin pockets tend to be brittle and will crack under
low applied strain or even cure shrinkage stress. These cracks can propagate as delaminations in fatigue (Rogers et al., 1990).
Movement of the laminate prior to cure, such as occurs during compaction of thick sections, can change the fiber length required by the final geometry. Since the fiber will not stretch or compress elastically, because the actual fiber stress is low, the fiber will either bridge, causing a void, or buckle, yielding local fiber curvature. Either way, significant resin stresses are induced as the fiber tries to straighten out under tension loading or buckle under compression loading. The matrix will crack, and the fracture will propagate under fatigue loading as a delamination in tension or as fiber crimping in compression. The latter failure mode is catastrophic (Morse and Piggon, 1990).
Heat is produced by the chemical reaction of cross-linking for all plastic matrix systems. This phenomenon is called exotherm. The matrix gells and becomes rigid at a given location as a function of time at temperature. The exotherm can raise the internal temperature of a laminate well above the surface temperature, which causes gelling to occur at different locations and different times. The fiber and matrix generally have different thermal coefficients of expansion, which results in residual stresses on cool down after cure. Filament winding processes introduce residual fiber stresses in the fiber matrix assembly that are retained through cure. Each of these phenomena can result in local residual stresses of significance in the matrix. Procedures for computing these stresses were developed under U.S. government contract and reported by Rai and Brockman (1988); thus, the code is available on request.
The design phase is not complete until the effects of these conditions have been determined and their stress effects included in fatigue analysis.
Matrix Stress Versus Fatigue
Fatigue failure in GRP composite materials, especially carbon, is a matrix-dominated phenomenon (see, for example, Lagace, 1985). Matrix strength in the composite is low compared with the fiber strength. Matrix failure through the thickness of a ply is termed transverse cracking or splitting, depending on whether the primary laminate load is transverse to or aligned with the fiber direction of the ply. Matrix failure between plies is termed delamination. Delamination can induce unstable sublaminate buckling in compression (Chai et al., 1981). However, all of these matrix failure modes cause local points of strain concentration on the fiber (Rogers et al., 1990). The fiber can be described as brittle since its stress-strain response is linear to failure (Prandy and Hahn, 1990). Therefore, fiber failure will occur when these local strain concentrations, together with the global strain, exceed either the fiber tensile strength or compression instability strength (O'Brien, 1980). Fiber failure, once initiated, generally propagates catastrophically across the laminate (Reifsnider, 1980).
Transverse cracking in the matrix occurs in plies that are off the axis of the load-carrying plies due to their strain. But transverse cracking and delamination also occur as a result of loads that are out of the plane of the laminate (Chan et al., 1986; O'Brien, 1980). These failure modes both occur in areas of curved fibers. The fiber possesses linear strain response and excellent fatigue properties. The matrix is viscoelastic, thereby absorbing energy in each strain cycle and yielding very poor fatigue properties (Mandell et al., 1985).
Any attempt to design long-life blades must consider minimization of matrix strain. Any design that contains curved fibers or any process that cannot maintain fiber alignment will result in fatigue-limited characteristics that are not represented by the coupon-generated fatigue data unless the coupon contains the same defect in a controlled manner.
Examination of the failed blades presented to the committee revealed quality variations that exceed good practice. A portion of the disappointment in blade life must be attributed to lack of quality control.
HELICOPTER ROTOR BLADE DESIGN AND PROCESSING
A brief discussion of the design and manufacturing process for helicopter blades is included here to complete the background on current manufacturing processes. A helicopter blade is a tension-dominated structure for which mass balance is critical and cost is a secondary consideration.
Thus, a good design for a helicopter blade may not be a good design for a wind turbine. However, since composite helicopter blades are considered to offer unlimited life, the knowledge gained in their development should have some value to the wind turbine designer.
Helicopter blades are made by semimechanized processes. Both Covington (1990) and Friedman (1988) describe the design and manufacturing process but with slightly different design concepts (Figure 5-1). Spar caps, which are unidirectional tape or tow, are wound by polar winding equipment around a root end pin fid and a tip pin. The tip end wrap is used to tension the cap material during cure to maintain fiber alignment. A compression-molded fiberglass filler called a fid is placed between the windings outboard of the root pin in order to lead the windings smoothly away from the pin. These unidirectional spar caps, generally four, are placed in a two-part cavity tool and wrapped with bias ply material. The caps react to bending and centrifugal loads and the bias plies react to shear. An internal rubber bag is placed inside the spar material. When it is inflated, the spar and torque wrap material is compacted against the insides of the cavity. The tool is then placed in an autoclave for cure.
The pin wrap at the root end of the blade is used for mechanical attachment of the blade to the hub. Although these fibers are curved, resin strains are low because the material is trapped between the flanges of a steel spool. Out-of-plane forces are minimum.
The trailing edge is honeycomb sandwich with very thin skins, and the leading edge is a thin stainless steel sheet, both of which are secondarily bonded to the spar. Balance and contour are maintained within 10 inch-pounds and ±0.010 on surface geometry, respectively.
Blade quality is excellent, but costs are high, greater than $100/lb, even though the bulk of the material is assembled by machine. The autoclave high-pressure cure entails an 8-hour cycle time, and both spar and assembly of the trailing and leading edges are separate autoclave cures. Current wind turbine blades are sold for under $10/lb.
MANUFACTURING PROCESSES APPLICABLE TO WIND TURBINE BLADES
Three processes are being evaluated for blade production that offer advantages and disadvantages relative to those already discussed. They are resin transfer molding, pultrusion, and fiber placement. Each is discussed in turn below.
Resin Transfer Molding (RTM)
Resin transferred by vacuum and pressure into a dry preform of aligned fibers that are constrained by matched inner and outer surface tools is termed RTM. Special, low-viscosity, delayed-reactivity resins have been developed, making this process attractive. Of course, these resins have not been fully characterized in fatigue. Many ways have been utilized to make the preforms. Weaving, braiding, and manual assembly with local area stitching are methods used today. The key to low-cost, quality construction is mechanization of the preform fabrication and handling process. The low pressures involved allow low-cost tooling materials and concepts to be employed. The process lends itself to high-rate production, as the part residence time in a tool is as low as one-half hour (Monroe, 1990).
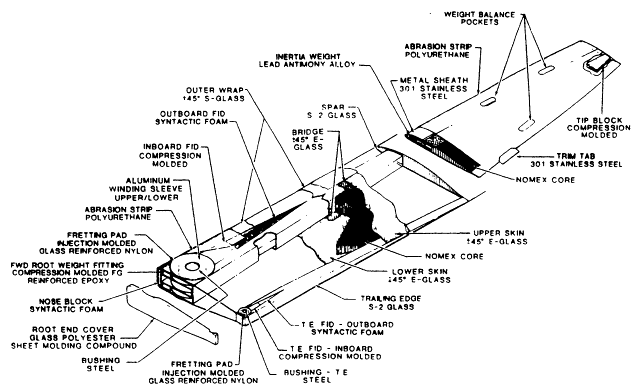
Figure 5-1
UH-1H composite main rotor blade. Source: Covington (1980).
The RTM process is utilized in the production of cooling tower fan blades up to 12.2 m (40 feet) in diameter. These blades look quite different from current wind turbine blades because of constraints of the process (Figure 5-2). The rigid inner mandrel must be removed from the cured blade; therefore, the root end must be the largest section of the blade. To live with this constraint, tower blades are made in two parts, an outer section extending from quarter span out to the tip and an inner portion containing a root end joint. These sections are joined by bonding, and both sections are at their largest cross section at the splice joint. Local fiber curvature can occur due to nonuniform flow of the resin through the preform. Cooling tower blades produced by this process sell for under $10/lb. (Monroe, 1990).
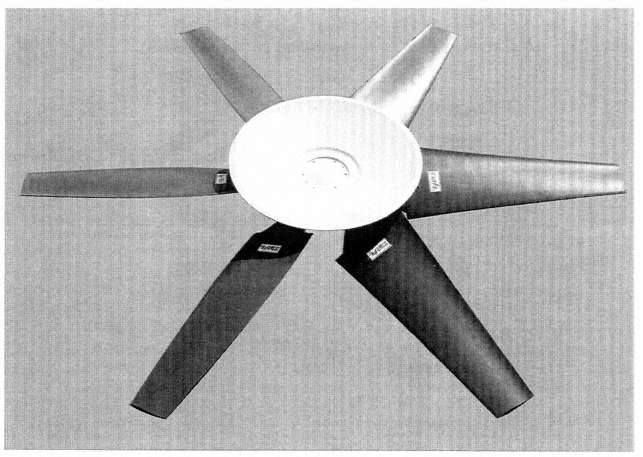
Figure 5-2
RTM cooling tower blade. (Courtesy, Hudson Products Corp.)
Pultrusion
Pultrusion is a process in which a continuous dry preform is pulled through a matched die while resin is injected under high pressure. It is first heated for cure and then cooled in the die to a temperature where it possesses sufficient strength to support the pulling clamp pressure. Once in operation, the process is almost labor free, yielding a product at just above the material cost (Goldsworthy, 1990). Die cost is reasonable if any volume is required. The most obvious restriction is that the part must be constant in cross section and laminate design. The quality of the laminate is excellent.
Fiber Placement
Fiber placement is similar to filament winding in the sense that the raw material is tow rather than tape. It is also similar to tape laying machinery, where a roller is used to stick the ply to the tool or prior ply. The merger of these two ideas yields a machine that can place fiber on any tool surface in any direction and produce a quality laminate. This process offers all the design freedom of manual lay-up together with the uniform quality of machine fabrication. Although quality is high and design freedom great, production costs are not comparable to either RTM or pultrusion processing. Lay-down rates of 10 pounds per man-hour have been quoted (Policelli, 1990).
Thermoplastics are touted as the low-cost composite material of the future largely because the requirement for cure is eliminated. Simply melt the resin while the preimpregnated plies are held together and then let it solidify. Currently, high processing temperatures and pressures keep tooling costs high. If the raw form of the material is a precompacted sheet, design freedom in fiber orientation is restricted. If prepreg tow could be located on the tool by a fiber placement machine that had heated rollers and could fuse and compact the added ply to the laminate already laid, perhaps thermoplastics could then replace thermosets. Efforts in this direction have not been successful to date.
ROOT END DESIGN FOR PRODUCIBILITY
The helicopter industry has come to appreciate composite materials for their inherent fatigue resistance, provided that the criticality of the resin matrix is understood. As a result, not only the blade but also the hub can be made of composite materials (Hause, 1989) (Figure 5-3). The ability of composite material to flex and retain fatigue strength has allowed movement toward bearingless designs. These hubs tend to be thick laminates of essentially rectangular cross section. An understanding of free edge and residual stress coupled with the effects of fiber curvature is required (Chan et al., 1986).
A fundamental difference between the aerospace industry's approach to design of the blade to root attachment and that of the wind turbine industry is noted here. The aerospace industry uses a lap joint with bolts in shear, while the wind turbine industry largely uses a flange with tension bolts. Aerospace emphasis is in keeping the load in the fiber and minimizing the load in the matrix and avoiding use of metal except for bolts and bushings. The helicopter industry is committed to composite blades and hubs because of their excellent fatigue life and benign failure modes. These reasons are just as applicable to the wind turbine industry.
MANUFACTURING RECOMMENDATIONS
To meet economic realities, the fatigue life and reliability of the blades must be improved. Fatigue life can be significantly improved by advancements in design. Reliability will be improved through carefully controlled mechanized manufacturing processes. This all must be accomplished with lower labor content.
Pultrusion offers an immediate solution to the economic issues but at reduced aerodynamic and structural efficiencies. RTM offers slightly longer-term opportunities while retaining aerodynamic efficiency. Fiber placement offers optimum aerodynamic and structural efficiency but will require further development to meet the necessary cost objectives. Fiber placement may be the best match for the emerging family of thermoplastic matrices. It is not clear which of these processes is most suitable for wind turbine blade production in this decade, but it is clear that the next generation of blades can meet their performance goals, and it is likely that one or more of these processes will achieve the cost objectives.
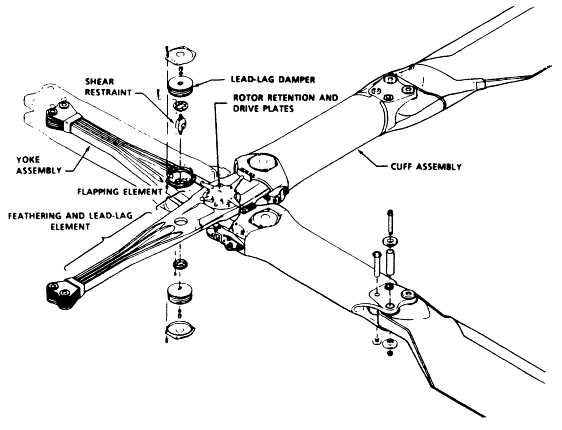
Figure 5-3
4BW hingeless bearingless rotor. Source: Friedman (1988).
The technology to design and manufacture wind turbine blades with the desired life and reliability is emerging. Loads must be determined. Matrix strains must be computed and fatigue allowables not exceeded. Quality in fiber alignment, resin content and weight, balance, and contour must be maintained and costs kept low. To achieve these requirements, the design must be developed with state-of-the-art and emerging analytical procedures. It is far more economical to examine design alternatives on the computer than in the field.
It is clear that aggressive use of composites to reduce the weight of the rotor is of benefit to the entire wind turbine system.
REFERENCES AND BIBLIOGRAPHY
Braswell, J. L., and B. F. Robertson. 1985. New Wings for the Huey. American Helicopter Society National Specialists' Meeting on Composites Manufacturing.
Chai, H., C. D. Babcock, and W. G. Knauss. 1981. One Dimensional Modeling of Failure in Laminated Plates by Delamination Buckling. International Journal of Solids and Structures, Vol. 17, Nov. 11.
Challis, A. L. 1987. Use of Composite Materials for Wind Turbines. Proceedings of BWEA/DEN Workshop, Harwell.
Chan, W. S., C. W. Rogers, J. D., Cronkite, and J. Martin. 1986. Delamination Control of Composite Rotor Hubs. Journal of the American Helicopter Society, August.
Covington, C. E. 1990. Composite Structural Applications in Helicopter Rotors. World Aerospace Technology 90, March.
Friedman, H. W. 1988. Design and Fabrication of an Advanced Light Rotor. 44th Annual Forum and Technology Display, AHS.
Goldsworthy, B. 1990. VA Wind Turbine Blades Made by the Pultrusion Process . NRC Workshop on Assessment of Research Needs for Wind Turbine Rotors, Washington, D.C., January 22-23.
Hause, J. H. 1989. The Four-Bladed Main Rotor System for the AH-1W Helicopter. 45th Annual Forum of the AHS.
Lagace, P. A. 1985. The Effect of Ply Thickness on Longitudinal Splitting and Delamination in Graphite Epoxy Under Compressive Load. ASTM Fatigue and Fracture.
Mandell, J. F., F. J. McGarry, A.J. Hsieh, and C. G. Li. 1985. Polymer Composites.
Monroe, R. C. 1990. Cooling Tower Fan Blades Made by the RTM Process. NRC Workshop on Assessment of Research Needs for Wind Turbine Rotors, Washington, D.C., January 22-23.
Morse, A., and M. R. Piggon. 1990. Relation Between Fiber Divagation and Compressive Properties of Fiber Composites. 35th International SAMPE Symposium.
O'Brien, T. K. 1980. Characterization of Delamination Onset and Growth in a Composite Laminate. Damage in Composite Materials, ASTM STP 775.
O'Brien, T. K. 1988. Towards a Damage Tolerance Philosophy for Composite Materials and Structures. NASA TM 100548.
Policelli, F. 1990. Filament Winding and Fiber Placement Techniques Applied to Wind Turbine Blades. NRC Workshop on Assessment of Research Needs for Wind Turbine Rotors, Washington, D.C., January 22-23.
Prandy, J. M., and H. T. Hahn. 1990. Compressive Strength of Carbon Fibers. 35th International SAMPE Symposium.
Rai, H. G., and R. A. Brockman. 1988. Cure Induced Microcracking of Composite Materials. Proceedings of 4th Japan-U.S. Conference on Composite Materials, pp. 732.
Reifsnider, K. L., ed. 1980. Damage in Composite Materials, ASTM STP 775.
Rogers, C. W., E. W. Lee, and D. A. Crane. 1990. The Effect of the Fracture Process on the Strength of Composite Laminates, 46th American Helicopter Society Forum, May 22.
Stoddard, F. S. 1989. Field Problems with Wind Turbine Rotors. Presented to Committee on Assessment of Research Needs for Wind Turbine Rotor Materials Technology, Washington, D.C., November 7.