2
New Opportunities in Catalytic Technology
SOCIETAL IMPACT OF CATALYTIC TECHNOLOGY
The chemical industry is one of the largest of all U.S. industries, with sales in 1990 of $292 billion and employment of 1.1 million.1 It is one of the nations's few industries that produces a favorable trade balance; the United States now exports chemical products amounting to almost twice the value of those that it imports (exports of roughly $37 billion compared to imports valued at about $21 billion).2 Between 1930 and the early 1980s, 63 major products and 34 major process innovations were introduced by the chemical industry. More than 60% of these products and 90% of these processes were based on catalysis. Catalysis also lies at the heart of the petroleum refining industry, which had sales in 1990 of $140 billion and employed 0.75 million workers.3 The very high degree of automation in the chemical and petroleum industries contributes to their lower labor intensity and to the fact that labor costs are much less important than material costs in the manufacture of petroleum refinery products and most chemicals. Clearly then, catalysis is critical to two of the largest industries in sales in the United States; catalysis is also a vital component of a number of the national critical technologies identified recently by the National Critical Technologies Panel.4
CATALYTIC CRACKING: MAJOR IMPACT ON THE U.S. BALANCE OF PAYMENTS During the refining of petroleum, large hydrocarbon molecules are broken down into smaller ones in a process known as cracking. The amount of gasoline that can ultimately be produced from a barrel of oil depends on how efficiently cracking is performed. If carried out incorrectly, cracking can lead to the formation of gases such as methane and ethane, and high-molecular-weight components called residue, which cannot be used to make gasoline or other transportation fuels. Today, through the use of highly optimized catalysts, more than 70% of the cracked products end up as transportation fuels. The story began in 1936 when acid-washed natural clays were first employed as catalysts. Subsequent research revealed that higher cracking efficiencies could be achieved by using amorphous silica-alumina. In the late 1950s and early 1960s, significantly greater efficiencies were found to be possible by using cracking catalysts based on zeolites. These materials are crystalline solids containing pores and cavities of molecular dimensions. The interior surfaces of the zeolite contain highly acidic centers that serve as the active sites for cracking petroleum (Figure 2.1). Mobil Corp. introduced the first zeolite-containing cracking catalyst in the early 1960s, and today virtually every refinery in the world uses catalysts containing Y-zeolite and, in some instances, ZSM-5. The use of zeolite catalysts has greatly benefited the U.S. balance of payments, because the improved efficiency in cracking has permitted a savings of more than 400 million barrels of oil per year, or more than $8 billion a year at $20 a barrel, and the story continues with new catalysts showing promise of even greater selectivity. For example, a mere 1% shift in product selectivity to gasoline allows a reduction in oil imports by more than 22 million barrels of crude per year or more than $400 million in the U.S. balance of payments. |
Looking into the future, one can see many exciting challenges and opportunities for developing totally new catalytic technologies and for further improving existing ones. Increasing public concern with the effects of chemicals and industrial emissions on the environment calls for the discovery and development of processes that eliminate, or at least minimize, the use and release of hazardous materials. Concern with the environment and the supply of raw materials is also focusing attention on the opportunities for recycling. Of particular interest for the chemical industry is the prospect of producing polymers that are readily recyclable. Although the world supply of petroleum is adequate for current demand, there is a need to continue the search for technologies that will permit the conversion of
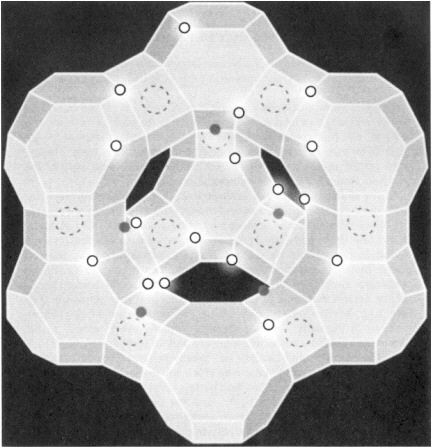
Figure 2.1 A representation of the molecular structure of HY zeolite. Solid circles represent the Brønsted acid sites responsible for cracking of petroleum. Open circles represent (AlO4)- sites, and dashed circles represent Me+ sites. (Figure courtesy of Union Carbide Corporation.)
methane, shale, and coal into liquid fuels at an acceptable cost. Also, to maintain economic competitiveness, it will be necessary to shift to lower-cost feedstocks for the production of commodity and fine chemicals. Taken together, these forces provide a strong incentive for increasing research efforts aimed at the discovery of novel catalysts and catalytic processes.
The markets for industrial catalysts are usually broken down into three sectors: chemicals, fuels, and environmental protection. The same classification is used in the sections that follow to discuss new opportunities in catalytic technology.
PRODUCTION OF CHEMICALS
Existing Products
In considering new routes to existing products, emphasis here is placed on major advances rather than on incremental improvements, even though the latter are often quite valuable and justified. With the increasing maturity of catalytic technology for most large-volume commodity chemicals, major advances in the future will require technical discontinuities. These discontinuities, as opposed to improvements in existing technologies, offer the real opportunities for catalysis to have an impact on the economy.
One can recognize and identify limits in the current technology for almost all major products made via catalytic processes. Furthermore, in most cases, at least one potential pathway to a major advance can be visualized. Each such advance constitutes a latent opportunity to shift to a lower-cost feedstock or to a simpler, less-capital-intensive route.
Lower-Cost Feedstocks
For typical commodity chemical processes, feedstock constitutes about 60-70% of the total manufacturing cost. Thus, a great financial impact can result from moving to a lower-cost feedstock. For example, the Monsanto process for making acetic acid (CH3COOH) via methanol (CH3OH) carbonylation involved a feedstock change—a shift from ethylene (C2H4), used in the previously dominant Wacker process, to methanol (CH3OH). Since its launching in 1970, the Monsanto process has captured most of the world's new capacity for making acetic acid. Feedstock price changes in recent years have further magnified the cost advantage of the methanol carbonylation route.

By far the strongest current thrust toward lower-cost feedstocks is the effort to substitute alkanes (ethane, propane, and butane) for the corresponding olefins and to convert methane to olefins or aromatics. The difference in price between these alkanes and their olefin counterparts can frequently be as much as 10¢ per pound, which is substantial. An excellent example is the production of maleic anhydride, a monomer for specialty plastics. Over the past 40 years, advances in catalytic technology have enabled the industry to switch from high-priced, toxic benzene to butenes and, more recently, to the lower-cost hydrocarbon butane. This latter development was possible only as a result of the discovery of the vanadyl phos-
phate catalyst by Chevron Research and Technology Company. A new process for producing maleic anhydride by using novel catalyst and reactor technologies is currently under development by Du Pont and is scheduled for commercialization in the mid-1990s.
An extensive worldwide effort is now under way to develop the catalytic oxidative coupling of methane to petrochemicals as well as liquid fuels. This effort encompasses oxidative coupling of methane to ethylene or aromatics, oxidative methylation of toluene to ethylbenzene and styrene, oxidative methylation of propylene to C4 olefins, and dehydrogenative coupling of methane to aromatics. This area of methane conversion captured exceptional interest and attention all over the world in the mid 1980s.

The relative abundance of LPG (liquefied petroleum gas, containing mostly propane and butane) and the strong demand for aromatics have prompted British Petroleum (BP) to develop a process for the catalytic conversion of LPG to aromatics. The BP process employs a zeolite-based catalyst developed by BP in conjunction with UOP's continuous catalyst regeneration system. LPG is converted to a mixture of aromatics, 95% of which are benzene, toluene, and xylenes. The aromatics yield is 65%. In another alkane utilization project, BP Chemicals is developing a process for the direct one-step ammoxidation of propane to acrylonitrile. Key to the process is a proprietary catalyst. Now at the pilot-plant stage, the process is targeted for commercialization in the mid-1990s.
A new commercial development in catalytic alkane dehydrogenation relates to the production of isobutylene and of propylene. The isobutylene requirement is for the production of gasoline octane enhancers (i.e., methyl tertiary-butyl ether, or MTBE), and the propylene need is driven by changes in the feedstock used to produce ethylene, which have resulted in less byproduct propylene production. Several companies have recently installed or are currently installing new plants for the production of isobutylene and propylene. In light of this remarkable development, there may also be opportunities for new catalysts that would be capable of promoting oxidative dehydrogenation of lower alkanes (i.e., ethane, propane, and isobutane) to their corresponding olefins. Direct functionalization of hydrocarbons remains a very significant approach (i.e., ethane to ethanol, propane to acrylonitrile, and butane to 1,4-butanediol).
Catalytic dehydrogenation of paraffins is also widely practiced commercially for the production of linear olefins in the C10-C17 range, used in the manufacture of biodegradable detergent intermediates. Typically, olefins in the C10-C14 range are used in the production of linear alkylbenzenes (LAB)
whereas heavier olefins in the C14-C17 range are used in the preparation of detergent alcohols via hydroformylation. The preparation of yet heavier olefins by catalytic dehydrogenation is also possible for specialized applications, including the manufacture of synthetic lubricating oils and oil additives. Worth noting at this point is the recent introduction of solid (heterogeneous) acid catalysts for the alkylation of benzene with heavy olefins in the production of LAB; this will allow the replacement of traditional catalysts, such as hydrogen fluoride (HF) or aluminum chloride (AlCl3) used for this purpose and will thus avoid the operational hazards associated with the handling and processing of corrosive catalysts and ameliorate the environmental characteristics of this alkylation process.
C1 chemistry (i.e., chemical processes based on carbon monoxide, carbon dioxide, or methanol as the starting material) now provides another interesting arena for feedstock-driven innovations in industrial catalysis. After the oil embargo of 1973, there was an extensive worldwide effort to pursue C1 chemistry for the production of chemicals as well as fuels. This effort eventually subsided when it appeared that the cost of carbon from C1 sources such as coal and natural gas could not really compete effectively with its cost from petroleum-based sources, even at the much inflated prices of the latter. However, it appears that some significant changes have occurred in the past decade (before Iraq invaded Kuwait) and that the opportunities for making chemicals via C1 chemistry should be revisited. In particular, methanol should be considered as a feedstock. Figure 2.2 illustrates the historical and forecasted trends between 1955 and 1998 for the ratio of methanol to ethylene prices. A substantial downward trend in favor of methanol can be observed.
It has recently been reported that rhodium-based homogeneous catalysts promote the reductive carbonylation of methanol to acetaldehyde at selectivities approaching 90% and at much lower pressure than required for prior-art catalysts. With the addition of ruthenium as co-catalyst, it is possible to achieve in situ reduction of acetaldehyde to ethanol, thus providing a new catalyst system for the homologation of methanol to ethanol.
One great challenge for catalysis has been the possibility of producing ethylene glycol via the oxidative coupling of methanol rather than the standard process based on ethylene as feedstock. Significant progress has been made recently in the catalytic oxidative dimerization of dimethyl ether to dimethoxyethane. Dimethoxyethane, in turn, should be hydrolyzable to ethylene glycol. Unlike methane coupling, which requires a temperature in excess of 600° C, the oxidative coupling of dimethyl ether proceeds at about 200° C with a mixed magnesium-tin oxide catalyst. By use of the ether rather than methanol, protection against side reactions has been achieved. These results are an extremely interesting lead which, coupled with the favorable trends in methanol pricing, could pave the way to another major
advance in industrial catalysis (i.e., the production of ethylene glycol from dimethyl ether). In addition to acetaldehyde, ethanol, and ethylene glycol, other large-volume chemicals currently made from ethylene or propylene may become attractive candidates for manufacture via C1 chemistry.
New Catalytic Oxidation Processes
Of the different classes of catalytic reactions, hydrocarbon oxidation (i.e., reaction with oxygen) is the one that generally has the lowest selectivity. In addition to the desired partial oxidation products, significant quantities of carbon monoxide, carbon dioxide, and water are often obtained. This results in complex and costly separations that, in turn, lead to processes with unusually high capital intensity. The annual capital expenditure for oxidation processes, per annual pound of product, is usually several times that for non-oxidative catalytic processes.
Well-recognized examples of major opportunities include the one-step
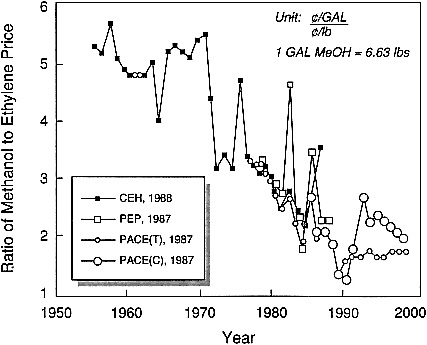
Figure 2.2 Ratio of methanol to ethylene prices, historical and forecasted trends, 1955 to 1998. (Reprinted, by permission, from J. Roth, 1991, p. 8 in Catalytic Science and Technology, Vol. 1, S. Yoshida, N. Takegawa, and T. Ono, eds., Kodansha Tokyo. Copyright 1991 by Kodansha Ltd.)
oxidation of methane to methanol, of higher alkanes to alcohols, of propylene to propylene oxide, and of benzene to phenol. Recent reports of success in these areas are tantalizing and suggest that catalytic oxidation should be one of the most important and fruitful areas for innovation in industrial catalysis. We seem to be at the threshold of several discontinuous advances.
New Products
New developments in catalysis can and will be the enabling technology that gives rise to new products in many sectors of the chemical industry. The potential impact of catalysis on new products is illustrated in the areas of polymers, pharmaceuticals, and biologically derived products.
Polymers
The production of raw polymers (e.g., pellets) in the United States approached 60 billion pounds per year in 1990, which corresponds to a $30 billion business. In terms of fabrication into end-use articles (e.g., synthetic fibers, films, containers, structural parts), the polymer business is significantly larger. Catalysis contributes to both monomer and polymer synthesis for a major part of this industry.
Today, the United States has a clear advantage in polymer science. This position now yields a positive balance of trade but is undergoing significant competition from developments in Western Europe and Japan. To ensure a continued prominent position, rather substantial advances in catalyst technology for both monomers and polymers will be required.
Every polymer scientist involved with synthesis or structure-property studies has a ''wish list'' for new monomers or polymers that have not yet been able to be synthesized via clear-cut economic routes for commercial practice. Almost every family of polymeric materials can utilize advances in catalysis, in monomer production or in the polymerization process. Changes in material requirements, environmental issues, feedstock availability and economics, and worldwide competitive pressures make future catalytic advances extremely important.
The primary area of intense catalytic activity involves the synthesis of new or improved polyolefins. This industry evolved out of the original Ziegler-Natta catalyst discovery in the 1950s, leading to tens of billions of pounds per year of polyolefins worldwide. New catalyst breakthroughs could lead to new markets of significant volume—including diversified products such as syndiotactic polypropylene, true thermoplastic elastomers (e.g., propylene-elastomeric polypropylene resin (EPR)-propylene block co-
A STRONG POINT FOR THE FUTURE Skyscrapers and bridges make our cities what they are. Airplanes, boats, and automobiles carry us anywhere in the world. Appliances fill our homes. Steel is the prime structural material in all these things. Steel has become as fundamental to our world as stone was to the caveman's. Going from stone tools to iron ones was a technological shift that must have taken considerable adjustment. Just as our ancestors made the leap from stone to metals, so are we moving from metals to polymers (plastics). Although our heightened expectations of technology have made this transition much less traumatic, it is every bit as far-reaching. In the past 50 years, polymers have become ubiquitous; we wear them (polyester permanent-press fabrics), we walk on them (polypropylene shoe soles), we ride on them (polyisoprene tires), we sit on them (polyurethane sofa cushion stuffing, plastic furniture), we package our food in them (polyethylene plastic bags), and we even feed them to our computers (poly(vinyl acetate) floppy disks). Yet, who would have thought that polymers—which we tend to think of as soft, stretchy, and pliable—would ever challenge steel as a structural material? When certain polymers are spun into fibers, the resulting materials are truly amazing. All polymers are long, chainlike molecules made up of many smaller molecules linked together. In flexible polymer fibers, such as polyester, the chains are partially relaxed—they are aligned along the direction of the fiber, but also loosely interwined like spaghetti dangling from a fork. The chains in these new rigid polymers are fully extended and lie parallel to each other, like a fistful of uncooked spaghetti. These fibers are unexpectedly strong for their weight. One such fiber, poly(para-phenyleneterephthalamide), was found to have a tensile strength higher than that of a steel fiber of the same dimensions, yet it weighed one-fifth as much. The commercial development of this product, Kevlar, by Du Pont, was a long and very expensive process. More than twelve capital-intensive steps are required to convert the basic aromatic feedstocks into a strong polymer. A combination of acid catalysts, hydrogenation catalysts, and oxidation catalysts makes up the eight catalytic steps in this complex process, which required extensive engineering beyond the initial laboratory work to develop the chemistry into a viable commercial process. Kevlar, this new kid on the structural materials block, has already made a dent in the automotive industry, replacing the steel belts in radial tires. Its strength, impact resistance, and light weight mean that cars and planes made largely of Kevlar may be in our future. Also, in terms of impact resistance, bullet-resistant vests worn by soldiers and policemen today are made of Kevlar (Figure 2.3). A thin vest of Kevlar can be worn comfortably inside one's shirt, yet it can stop slugs as effectively as a steel plate. This light, yet strong, material is only beginning to be used for the myriad applications that await it. |
polymers), and incorporation of polar monomers into various polyolefin classes. For example, if acrylates, vinyl esters, acrylonitrile, and the like could be incorporated into the present low-pressure polyolefin synthesis, the result would be a new family of olefin-based polymers that are likely to have major commercial significance. Of course, improvements in the present catalytic systems will have a pronounced effect on the polyolefins that are commercially available. Improvements in molecular weight distribution control (e.g., narrow molecular weight distribution), the ability to synthesize EPRs in gas-phase reactors, and the control of catalyst decay (e.g., improved efficiency) are advances that will surely occur.
Novel metathesis catalysts for the synthesis of cyclic olefins have resulted in a host of new polymeric structures. Several of these have reached commercial status (e.g., trans-poly(octenamer)). The few catalysts that are effective are often expensive and require unattractive precautions during industrial scale-up. Further advances could make this a promising area for industrial exploitation.
In the field of functionalized monomers, the trend will likely revolve around new or existing monomers via biomass, coal, or C1 conversion, as the carbon source availability changes together with economics. Improved catalysts for creating monomers from agricultural commodities or waste materials offer increasingly important opportunities. This field could benefit from the genetic engineering of specific enzyme "catalysts." Oxidative coupling of methanol to ethylene glycol and ethanol to 1,4-butanediol could open new routes to these important monomers. A new process (in progress) involving the one-step ammoxidation of propane to acrylonitrile could change the commercial position. Other possibilities—for example, utilizing shape-selective catalysts such as zeolites—could yield lower-cost routes to 4,4'-diphenol via phenol coupling. Such a molecule is of interest for liquid crystalline and engineering polymers. Improved non-phosgene routes to diisocyanates are desired. Functionalized oligomers such as hydroxyl (OH)-, amino (NH2)-, and carboxyl (COOH)-terminated polyolefins could yield important blocks for step-growth polymers (e.g., urethanes, polyesters). Functionalized fluoroolefin oligomers for inclusion in step-growth polymers could likewise offer a new variety of polymers. A non-chlorine route to siloxane polymer precursors is also desired.
In the field of polymers, a number of prior failures have been well documented in the literature, primarily resulting from the unavailability of appropriate catalysts. The reaction of acetaldehyde to yield polyvinyl alcohol is one such example. Because phosgene is highly toxic, a non-phosgene route to polycarbonate is desirable. The polymerization of phenol to a highly linear unsubstituted poly(1,4-phenylene oxide) of high molecular weight would be of interest, as would the polymerization of polyphenylene
by benzene coupling. There are many other examples of novel polymers waiting to be polymerized from available monomers.
Cationic polymerization continues to be an area of increasing research made possible by improved Lewis or Brønsted acid catalysis. Continued improvement is desired to yield higher molecular weights and industrially acceptable process conditions. Extension to other monomers (e.g., vinyl acetate) would be of future interest. Anionic polymerization is of interest primarily for unique block copolymers. Extension to additional monomers and the resultant block structures deserves more attention.
Pharmaceuticals
There can be no question that the 1990s will be the "decade of chirality." Many of the opportunities and challenges in this explosively evolving field stem from the pharmaceutical area and the growing recognition that the "wrong" enantiomer of a racemic drug represents a "medical pollutant" whose toxic side effects can far outweigh the therapeutic value of the pharmaceutically active enantiomer. The classic example in this area is that of thalidomide (Figure 2.4). The R-isomer of thalidomide is an effective sedative; tragically, the drug was sold as the racemate, and it was subsequently discovered that the S-isomer is a powerful teratogen. More recently, Eli Lilly was forced to withdraw its Oraflex anti-inflammatory because of liver damage caused by the ''inactive" R-isomer. Although recent regulatory changes by the Food and Drug Administration stopped short of requiring that all drugs be sold as a single enantiomer, there is an obvious trend in this direction by drug companies.
Among the available strategies for the manufacture of optically pure substances, asymmetric catalysis provides powerful and unique advantages. Perhaps the foremost is the "multiplication of chirality"—the stereoselective production of a large quantity of chiral product by using a catalytic amount of a chiral source. Unlike fermentation, asymmetric catalysis is characterized by generality: processes are not limited to "biological"-type substrates, and the R- and S-isomers are made with equal ease. Asymmetric catalysis also circumvents the disposal of large amounts of spent nutrient media that are generated during fermentation. By comparison, optical resolution (i.e., diastereomeric crystallization) is extremely labor-intensive and necessarily produces 50% of the "wrong" isomer, which must be destroyed or racemized in a separate step.
Given the increasing importance of enantioselective synthesis, it is important that the United States place greater emphasis on this area. At present, Japan and the European Community are the leaders in basic research discoveries and applications.
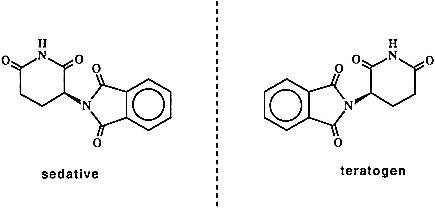
Figure 2.4 Enantiomeric pairs of thalidomide.
Biologically Derived Products
The rapidly growing field of biotechnology brings with it opportunities in the field of enzyme-catalyzed reactions. The role of genetically engineered microorganisms in synthesizing rare and valuable peptides used in human therapeutics is now well established. The same techniques of molecular biology can also be used to enhance the properties of enzymes as catalysts for industrial processes that are very similar to classic catalytic technology.
This approach can potentially revolutionize the applications of biological systems in catalysis. Enzymes and other biological systems work well in dilute aqueous solution at moderate temperature, pressure, and pH. The reactions catalyzed by these systems are typically environmentally friendly in that few by-products or waste products are generated. The catalysts and the materials that they synthesize are, as a rule, biodegradable and therefore do not persist in the environment. The reactions are typically selective with extremely high yields, and enzymes can be used to catalyze a whole sequence of reactions in a single reactor, resulting in vastly improved overall yields with high positional specificity and 100% chiral synthesis in most cases. The improved use of enzyme catalyst technology with whole-cell catalysis, reactions catalyzed by single enzymes, and mixed enzymatic and chemical syntheses are all important for the development of new catalyst technology.
Whole cells of various microorganisms are being used more frequently in the catalytic synthesis of complex molecules from simple starting materials. The use of whole microbioal cells as biosynthetic catalysts takes advantage
of one of the unique properties of enzymes: they were designed by nature to function together in complex synthetic or degradative pathways. Because of this property, whole cells and microorganisms can be used as catalytic entities that carry out multiple reactions for the complete synthesis of complex chiral molecules. A patent was recently issued for a genetically engineered Escherichia coli that synthesizes the molecule D-biotin directly from glucose. Biotin has three chiral centers, and the current chemical synthesis requires 13-14 steps with low yields. Similarly, researchers are constructing a microorganism that directly catalyzes the synthesis of a vitamin C precursor from glucose. Combining genes from various organisms results in a process that uses a microbially synthesized intermediate with a final chemical conversion to vitamin C. Whole cells of microorganisms are also used in the synthesis of antibiotics from carbohydrate starting materials, and whole cells are used in the biocatalysis of certain steroids. A number of specialty chemicals with complex synthetic schemes can be produced most efficiently by intact microorganisms utilizing a series of enzyme-catalyzed reactions designed by nature to work together.
The biotechnology field also has a growing number of examples of reactions of industrial significance catalyzed by isolated enzymes. The conversion of cornstarch into corn syrup by the enzymes alpha- and gluco-amylase and glucose isomerase is a large industrial process, generating corn sweetener for soft drinks and other uses. The enzymatic conversion of acrylonitrile to acrylamide has recently been commercialized in Japan. Japanese companies and researchers have been very diligent in developing enzymatic processes for the synthesis of fine chemicals. Enzyme-catalyzed reactions are used by the Japanese for the synthesis of monosodium glutamate, L-tryptophan, and phenylalanine.
The stereospecificity of enzyme-catalyzed reactions has been used to advantage in polymer synthesis as well. Workers at ICI have developed a combined enzymatic and chemical process for the synthesis of polyphenylene from benzene. Benzene is oxidized to a cis-dihydrodiol by an enzyme-catalyzed oxygenation. The diol is derivatized, polymerized, and rearomatized to give polyphenylene in a reaction that cannot be carried out by classic chemical methods because of solubility problems. This new route to polyphenylene is an excellent example of combined enzyme and classic chemical synthesis to make a product that is otherwise too expensive for practical use. Other biological polymers are also finding their way into the catalyst field in various applications. Microorganisms are used to synthesize materials such as poly(beta-hydroxybutyrate), a biodegradable plastic, and researchers are exploring a series of synthetic silklike materials that may have uses in high-tensile-strength applications.
PRODUCTION OF FUELS
Existing Fuels
Although the current understanding of how the individual components of gasoline affect various environmental issues is very limited, several components in gasoline are now considered harmful to the environment if released to the atmosphere in high concentration as either spills, vaporization losses, or the result of incomplete combustion. They include aromatics, notably benzene, a carcinogenic reagent; high-vapor-pressure hydrocarbons such as butane; reactive hydrocarbons such as olefins; and sulfur compounds, which could promote the formation of smog and acids. The petroleum industry is responding to these concerns by directing its research toward the reformulation of gasolines. As discussed below, gasoline reformulation will require a number of advances in catalytic technology.
Advanced Fluidized Catalytic Cracking Catalysts for the Production of Environmentally Acceptable Gasoline
Innovations in catalytic cracking catalysts over the past 30 years have improved the conversion of the heavier components of crude oil to gasoline and diesel oil, allowing a reduction in crude imports of more than 400 million barrels a year. In the future, new types of cracking catalysts will be required to produce motor fuels that are environmentally more acceptable. To reduce the aromatics content of gasoline while at the same time providing higher-octane paraffinic components will require that fluidized catalytic cracking (FCC) catalysts produce more olefins. The highly reactive olefins can be isomerized, oligomerized, or alkylated with paraffins as well as reacted with methanol to produce a variety of high-octane ethers. For example, the zeolite ZSM-5 has been shown to be active in producing olefins when mixed with conventional faujasite-type cracking catalysts. ZSM-5 and other molecular sieves have also been proved active in isomerizing and oligomerizing olefins to a variety of liquid fuels.
Cracking catalysts will also have to become more rugged to endure the higher temperatures required to produce more olefins for subsequent processing into high-octane gasoline components. The catalytic cracking process has been the workhorse of modern refineries for 30 years and, with improved catalysts, will continue to be the main process for converting the heavier end of crude oil into more environmentally acceptable components for gasoline and diesel fuels.
Oxygenates for Octane Boosting
The need to remove aromatics from gasoline has created a need for organic oxygenates as replacement octane enhancers. Today the two predominant
oxygenates used as octane enhancers are methyl tertiary-butyl ether (MTBE) and ethanol (EtOH). However, other oxygenates (e.g., alcohols, ethers, acetates, and carbonates) are known octane boosters. Some of these compounds have been evaluated only partially for their performance characteristics and may represent major growth opportunities in oxygenated fuels. Currently, approximately 150,000 barrels per day of oxygenated compounds (MTBE, EtOH) is added to gasoline in the United States. By the year 2000 it is projected that 750,000 barrels per day (the equivalent of roughly 10% of current U.S. production of petroleum) of oxygenates will be required for the gasoline pool. In the United States, the 1990 MTBE production capacity was 117,200 barrels per day. By 1993 it is targeted at 256,400 barrels per day. Iso-olefins (e.g., isobutylene) are now produced as a by-product of fluidized catalytic crackers in petroleum refineries. These quantities will support production of ether at a rate of only 200,000-300,000 barrels per day.
The process technology leading to the majority of oxygenates now in use has been made very efficient through catalyst modifications and engineering design. However, new processes leading to the same oxygenates may have cost advantages if different building blocks and feedstock sources (crude oil versus coal versus natural gas) are utilized.
The precursors of established octane enhancers—alcohols and ethers—rely heavily on natural gas and crude oil as their feedstock supply. With the United States importing approximately 55% of its crude oil, the price of oxygenates will track that of crude oil, which is currently approximately $20 per barrel. Industry estimates suggest that when crude oil prices pass approximately $30 per barrel, coal gasification to syngas and the conversion of syngas to hydrocarbons and oxygenates become cost competitive. Therefore, indirect liquefaction of coal offers promise for oxygenated fuels for the transportation industry.
New Fuels—Methanol Dissociation to Carbon Monoxide and Hydrogen
Methanol dissociation to carbon monoxide (CO) and hydrogen (H2) on-board a vehicle would provide a fuel that is even cleaner and more fuel efficient than undissociated methanol. The heat required for endothermic dissociation of methanol can be supplied readily by engine exhaust gas. This recovers waste heat and increases the heating value of the fuel. Fuel efficiency is further enhanced because an internal combustion engine running on dissociated methanol can be operated with excess air (i.e., lean combustion). Lean and complete combustion would ensure low CO and hydrocarbon emissions. The problems associated with formaldehyde emission would be significantly reduced. Reduction of nitrogen oxide (NOx) emissions by an order of magnitude has been demonstrated because of lower combustion temperatures.
Dissociated methanol can also be used as an efficient peaking gas turbine fuel utilizing the heat recovery from exhaust gas. The endothermic methanol dissociation reaction is also promising for cooling critical systems such as engine cooling for hypersonic jets when dissociated methanol is used as a fuel. Methanol can be produced on a large scale in a remote location for shipping. The methanol dissociation reaction can be modified to yield a wide range of H2 to CO ratios. It would provide an economically competitive and convenient source of CO or H2, especially on a small scale, and could be used in chemical plants, materials processing plants, and fuel cells on-board a vehicle.
Because of these emerging applications, there is renewed interest in developing methanol dissociation technology. Insufficient catalyst activity at low temperature and catalyst deactivation have been reported. These are two major challenges for catalysis research for on board applications. Generally, copper-based catalysts are used at 250-300° C. Zinc-chromium and precious-metal-based catalysts are utilized at 350° C or higher. Improvements in catalyst performance are being sought. For example, the activity of copper-based catalysts can be increased by adding appropriate promoters and improving pretreatment environments. Catalyst stability depends on reaction temperatures. Active catalysts would allow reaction at lower temperature and would improve catalyst stability. Methanol dissociation could operate at near atmospheric pressure for passenger cars. Extension to about 15-20 atm is needed for turbine applications and to more than 100 atm for diesel engines.
ENVIRONMENTAL PROTECTION
Public interest in protecting the environment has increased and expanded greatly. These public concerns manifest themselves in many different ways. The challenge is to preserve the benefits of modern technology without seriously contaminating the natural world.
Three strategies are available for reducing the impact of chemicals on the environment: waste minimization, emission abatement, and remediation. Waste minimization calls for the design and development of products and processes that are inherently low-polluting or non-polluting. The abatement of emissions can often be achieved by trapping harmful effluents or converting them to harmless substances (e.g., conversion of nitric oxide to nitrogen). Where an environmental insult has occurred, effective means of remediation are needed to restore the environment to its "green" state. As shown by the examples presented below, catalysis can contribute to these three approaches.
CATALYSIS FOR ENERGY INDEPENDENCE The long gas lines and soaring prices during the Arab oil embargo of 1973 and the turmoil in Iran in 1979 made the U.S. public painfully aware of the nation's growing dependence on foreign oil. As the crises dissipated, however, so did public concern. Few lessons appear to have been learned. Today, the United States imports more oil than it did a decade ago—a fact brought home to the public once again by Iraq's takeover of Kuwait in 1990. A few people did take a hint from the oil shocks of the 1970s. One oil company has developed a process to convert methanol (methyl alcohol, also called wood alcohol) to gasoline that stands as the first significant advance in synthetic fuel technology since the German program during World War II. The process converts natural gas, coal, or any carbon-rich raw material to premium gasoline. The carbon source is first ''gasified," converted to a mixture of carbon monoxide and hydrogen gas by heating in the presence of steam and a catalyst. Another catalytic procedure converts the carbon monoxide and hydrogen to methanol—a standard industrial process. Methanol is converted directly to 95-octane gasoline through the action of a synthetic catalyst called ZSM-5. ZSM-5 is a molecular sieve, which is to say that its three-dimensional crystal structure has an open, lacy quality to it—more hole than crystal. These pores are approximately 5 angstroms (about one-hundred billionth of an inch) in diameter, big enough to accommodate a methanol molecule. ZSM-5 catalytically breaks down the methanol molecule, whose chemical formula is CH3OH, to create a molecule of water and a hydrocarbon fragment (CH2). These fragments assemble themselves into hydrocarbon molecules—the stuff that gasoline is made of. (Gasoline is actually a rich stew of assorted hydrocarbon molecules, ranging from 6 to 12 carbon atoms per molecule.) All zeolites are molecular sieves, and many of them also catalyze this reaction, but the other zeolites keep adding the hydrocarbon fragments on to the gasoline-sized molecules, turning them into heavier and heavier liquids, and eventually into a solid. The pores in ZSM-5, however, are just the right size to construct gasoline-sized molecules, but too small to let larger hydrocarbons form. (Some of the intermediate products formed en route to gasoline-sized hydrocarbons are important chemicals in their own right. These chemicals can be intercepted as they form, and diverted for other uses. These uses include making high-quality synthetic lubricating oils and diesel fuels.) The process was commercialized in 1985, when a plant was built on New Zealand's North Island. This facility converts offshore natural gas, mostly from the extensive Maui Gas Field in the Tasman Sea, into 14,500 barrels of gasoline per day. This gas-to-gasoline plant is designed to supply one-third of New Zealand's total liquid fuel demand and is a key to increasing its energy self-sufficiency. |
Alkylation Catalysts
The alkylation of paraffins with olefins is one of the major refinery operations. The process reacts a paraffin, usually isobutane, with olefins, generally propylene and butenes, to produce highly branched C7 and C8 paraffins, respectively. They constitute a premium high-octane gasoline component (95-98 research octane number for C4 alkylate). The alkylation process is assuming increasing importance with increased olefin production from modern fluid catalytic cracking units and with recent emphasis on clean fuels of lower aromatics content.
Both of the currently used liquid acid catalysts, sulfuric acid and hydrogen fluoride, are very corrosive. Acid waste disposal in the sulfuric acid-catalyzed process is of increasing environmental concern, and liquid hydrogen fluoride is a potential health hazard. With increasing concerns and possible legislative action addressing environmental and safety issues, current alkylation processes may face critical scrutiny.
Exploratory studies have shown that new catalysts can be developed that are cleaner and safer than those presently used. However, it is also apparent that powerful acid catalysts are required for alkylation. It is a formidable challenge to produce a novel catalyst system that makes a new process economically feasible: high yield of alkylate, selectivity to produce high-octane gasoline, long life cycle, regenerability, and greatly reduced environmental and safety risks. This is a challenge that would benefit from broad-based fundamental studies of acid catalysis and from increased exploratory research. At the present time, no satisfactory solid alkylation catalyst exists.
Replacements for Chlorofluorocarbons
Chlorofluorocarbons (CFCs) are now believed to contribute to the seasonal ozone depletion over the Antarctic continent. However, because they are crucial to many aspects of modern society and have no available replacements, it is not practical to cease their production immediately. By 1988, total CFC consumption worldwide had grown to 2.5 billion pounds per year. The three major uses in the United States are as refrigerants (30%), foam blowing agents for polystyrene and polyurethane (28%), and industrial solvents and cleaning agents (19%).
Ironically, it is the high stability and inertness of CFCs, which make them so valuable, that has led to their downfall. Once released at ground level into the atmosphere, they rise slowly into the stratosphere where they are degraded by high-energy radiation from the sun, to release chlorine-containing free radicals that trigger a catalytic ozone depletion cycle. Following detailed though not definitive studies, agreement was reached on a
major global environmental treaty, the Montreal Protocol, to phase out CFC production by the turn of the century. A race by all CFC producers then began to find suitable and environmentally acceptable substitutes. The strategy is to reduce its atmospheric lifetime by introducing hydrogen into the molecule so that it is removed from the atmosphere by reaction with hydroxyl radicals in the troposphere. The commercially viable synthesis of these new compounds is a major challenge for catalysis, because catalysts used for the production of CFCs lack the required selectivity and activity to be acceptable for the production of hydrogen-containing substitutes.
The projected costs for these molecules, hydrogenated chlorofluorocarbons (HCFCs), are approximately 2-5 times those of the CFCs they are replacing, because of the complexity of the new manufacturing processes. Although rapid progress is being made toward the production of HCFCs, the latter are not entirely satisfactory and may have to be phased out in turn. Consequently, major advances in catalytic science and technology will be required to develop more acceptable substitutes before the turn of the century.
Emission Abatement
Catalytic technology is playing an ever-increasing role in environmental protection. In 1989, for the first time, the U.S. market for emission control catalysts (largely for automotive emissions) exceeded the market for petroleum refining catalysts. However, the area of stationary emission control (e.g., from power plants) has been flagged as one that will experience very large (20% per year) growth in the years ahead. There is also a need to reduce emissions from many chemical production plants. Thus, novel catalysis will in many cases be the critical technology that enables us to retain most of the benefits created by the chemical and petroleum industries, but with improved preservation of the environment.
Catalysts for automotive emission control are now well developed in the United States and, in general, meet mandated standards for removal of hydrocarbons, CO, and NOx. Recent Clean Air Act revisions will require significantly greater reductions in 1993 in the emissions of hydrocarbons, CO, and NOx, than those now mandated. In addition, very stringent local automobile emission standards (e.g., in California and Vermont) will require up to a 10-fold reduction in emissions by the late 1990s. This will necessitate more active catalysts, new catalyst supports (e.g., metallic supports), and new reactor designs that enhance low-temperature performance. A first step in this direction is the electrically heated converter, which offers a severalfold reduction in emissions over currently available technology. Because of the rapidly escalating price of rhodium (which promotes
AUTO EXHAUST CATALYSTS Automobile exhaust is a well-known pollution source. It contains products of incomplete combustion—carbon monoxide and assorted light hydrocarbons—as well as combustion by-products such as oxides of nitrogen and sulfur. Catalytic converters that transform incomplete combustion products to carbon dioxide and water, and oxides of nitrogen back to nitrogen and oxygen, are required equipment on all automobiles sold today in most industrialized countries. Manufacturing these catalytic converters is a worldwide business worth several hundred million dollars per year. The oxidizing converters that finish off the incomplete combustion products were first commercialized in the United States in the fall of 1974, and "three-way" converters that also handle nitrogen oxides became available, again in the United States, in the fall of 1980. Recent tests of a new catalytic converter design show that substantial further reductions in hydrocarbon and carbon monoxide emissions may well be possible to meet the stringent requirements of the 1991 Clean Air Act. Hydrocarbon and carbon monoxide emissions are at their highest levels during the first 10 minutes after the engine has been started. The cold engine burns fuel less efficiently, passing more incomplete combustion products on to the cold catalytic converter, which is itself less efficient. Heating the catalyst would make it more efficient faster, and the new design does just that. The converter is made of catalyst-coated stainless steel, which can be heated by passing an electric current through it (Figure 2.5). Tests of a prototype under the federal test procedure specified for catalytic converters have already shown substantial emission reductions in comparison to conventional technology. The electrically heated catalytic converter is being developed to meet the ultralow emission regulations enacted by California in late 1990 to help highly polluted areas such as the Los Angeles Basin. |
NOx reduction), there is a need for the development of lower-cost NOx reduction catalysts or the development of practical catalysts for NOx decomposition. With cleaner gasolines, there may also be opportunities to develop lower-cost catalysts containing a smaller amount of the expensive noble metals. Finally, with the very high cost of rhodium, there is need for an improved technology for recovering it.
The control of power plant emissions is another major area of opportunity for catalysis. In particular, there is a need for removal of NOx emissions either via selective catalytic reduction (SCR) or, if achievable, via NOx dissociation. The abatement of NOx from power plants is important in efforts to control acid rain and photochemical smog, the latter being linked
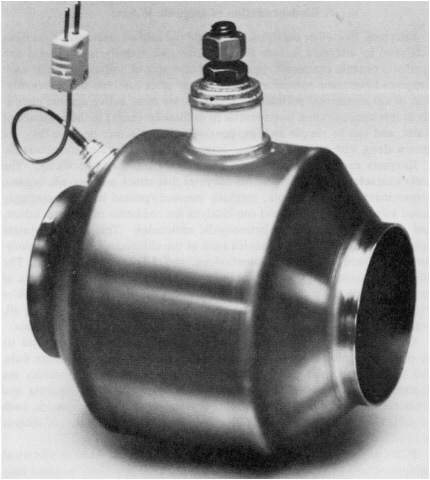
Figure 2.5 Electrically heated automobile catalytic converter. (Figure courtesy of W. R. Grace & Company.)
with harmful ozone production. SCR removes NOx in flue gas by reacting oxides of nitrogen with ammonia to form nitrogen and water. SCR was first commercialized in Japan and is now being used extensively there. It has also been commercialized in Germany. The catalyst is the heart of SCR technology, and it must afford both high activity and high selectivity (toward nitrogen formation). Major improvements in SCR catalyst performance can be achieved through strategic design of the catalyst pore structure.
Biodegradation of Organic Waste
Enzymes, like other catalysts, accelerate the rate of reactions. Reactions catalyzed by enzymes include the oxidation and hydrolysis of natural and synthetic organic chemicals regarded as pollutants of soil and groundwater. Enzymes have some natural advantages over other catalysts in the degradation of environmental pollutants. Enzymes are most active against materials at low concentration (micromolar to millimolar range) in the presence of water, and can be simple and inexpensive to manufacture because they are grown along with microorganisms. Enzymes themselves are biodegradable.
Enzymes can exhibit either narrow or broad substrate specificity. The latter characteristic is desirable for enzymes that attack and degrade organic contaminants. For example, methane monooxygenase has an amazingly broad substrate specificity and can catalyze the oxidation of alkenes, ethers, and alicyclic, aromatic, or heterocyclic molecules. This enzyme system can also degrade synthetic organics such as the chlorinated solvents chloroform, dichloroethylene, trichloroethylene, and 1,1,1-trichloroethane. The ability of enzymes to degrade natural organics such as the components of gasoline, crude oil, and most solvents, as well as synthetic organics such as trichloroethylene or polychlorinated biphenyls, means that most, if not all, organic contaminants can be degraded in reactions catalyzed by enzymes.
Chlorinated organics such as dichlorodiphenyl/trichloroethane and its by-product dichlorodiphenyl ethylene, pentachlorophenol, chlorocatechols, and other chlorinated aromatics used as preservatives and pesticides are degraded in oxidation reactions catalyzed by enzymes from bacteria and other microorganisms. Even the most complex halogenated organics, such as polychlorinated biphenyls (PCBs) and chlorinated solvents, are subject to catalytic attack by certain microorganisms.
PCBs were developed earlier in the century as oils for use in electrical equipment and as lubricating fluids in industrial applications because they gave good insulating and lubricating properties without being explosive or flammable. PCBs were later discovered to bioaccumulate and are now classed as environmental hazards. Recently, enzymes have been found in microbes that will reductively dechlorinate PCBs and oxidize them in the presence of molecular oxygen. Even though these enzymes were not evolved to degrade PCBs, they have a broad-enough substrate specificity to catalyze the initial degradation of PCB molecules in the environment. The use of broad substrate specificity oxygenases in bacteria may be the only practical method of treating PCB-contaminated soil and water because of their low cost and adaptability in the environment.
Similarly, chlorinated solvents have been widely adopted because of their excellent solvent properties and lack of flammability. Trichloroethylene
DINING ON POLLUTION Bacteria and other microorganisms have been digesting other creatures' waste products for millions of years. As a result, there are biological processes to break down most organic compounds, including the amazing variety of complex chemicals synthesized by plants. Some of these processes are now being exploited to break down the complex chemicals made by man. ''Bioremediation" is the name given to the use of biological processes to clean up contaminated soil or water. Almost every organic compound synthesized by man can be broken down, albeit slowly, by microorganisms if their other needs for growth are met. Microorganisms need water, certain inorganic nutrients, and sometimes air to live, but once furnished with those staples, they will obligingly break down many of the synthetic organic chemicals mankind has created, used, and discarded. In fact, scientists have discovered that many toxic chemicals—such as drain cleaners, paint thinners, and used crankcase oil—are routinely broken down by microorganisms that live in wastewater treatment plants downstream. Enzyme catalysts were used to clean up some of the beaches in Prince William Sound that were contaminated by oil in 1989. Cleanup crews added fertilizer to the beaches, stimulating the growth of their natural populations of microorganisms, which soaked up oil along with the fertilizer and catalyzed its degradation. Without fertilizer to help things along, the microbes would still have digested the oil, but at a very slow rate. The slow rates at which biological processes degrade pollutants such as spilled oil are one reason that bioremediation is not in more widespread use. However, bioremediation is potentially the most cost-effective approach for cleaning up a contaminated site. Most sites contain a complex mixture of various chemicals. The array of native microorganisms, bolstered when necessary by laboratory cultures of specialized bugs for particularly intractable substances, would simultaneously catalyze the breakdown of the whole assortment of substances present at a site—an approach that could be far cheaper than the current practice of applying a sequence of physical and chemical treatments, each one tailored to particular contaminants. Bioremediation should be less invasive, leaving the soil in place during treatment rather than digging it up, and thus the site should recover more quickly afterward. The process is also self-perpetuating—the bugs will eat the pollutant indefinitely as long as the fertilizer holds out. Thus stimulating natural microorganisms and introducing laboratory-bred or even rationally designed organisms could result in the safe and effective remediation of a large number of contaminated sites across the country. |
(TCE), perchloroethylene, and trichloroethane have been used widely in the past as dry cleaning solvents and chemical degreasers for metal finishing and electronic applications. Surprising numbers of different oxidative enzyme systems have been identified that will attack TCE or other chlorinated aliphatics.
The technique of enzyme recruitment offers the prospect of producing single organisms that contain a spectrum of genetically engineered enzymes capable of degrading hazardous waste in the environment. Enzyme recruitment permits microorganisms to degrade new molecules and broadens the ability of microorganisms to attack synthetic organic chemicals. The idea of using enzymes that can reproduce themselves, can be made very cheaply, and can work under conditions that are often found in the environment may be one of the most effective means modern science can devise for treating and degrading hazardous waste, including organic chemicals synthesized by man to be stable under harsh conditions. The broad substrate specificity of certain enzymes offers the opportunity to use enzyme catalysis for improving and protecting the environment.