4
Resources of the Solid Earth
ESSAY: NATURAL EXPLOITATION
All organisms exploit the surrounding environment to sustain their metabolism, and in the process they produce waste. Prokaryotes, single-celled bacteria, have colonized every niche on the Earth's surface, absorbing local chemical compounds and excreting unused or altered compounds. Cities with vast human populations do the same thing—they use natural resources and produce waste. Whether a single-celled organism or a complex society, the efficient location and exploitation of resources and the production and appropriate disposal of neutral or recyclable wastes determine success or failure, life or death.
The resources required for a complex civilization are more diverse than those needed by simpler organisms. Besides the obvious needs for primary materials—chemical and energy sources—shared by all living beings, humans depend on physical and biological systems that process primary resources. The ability to invade potentially hostile environments depends on the use of many of the Earth's subsystems. We require large amounts of water and landscapes that provide the means to feed and shelter us.
Water may well be the Earth's most vital resource. While regions subject to drought are usually concerned with the quantity of available water, all communities must now care for the quality of water. In the United States, irrigation methods and personal habits deplete ancient aquifers and drain mountain runoff. At the same time, toxic chemicals and dangerous pathogens can seep into water supplies. Only with conservation and careful planning will water become a renewable resource again; education programs are needed to deter both sophisticated cultures and simple communities from fouling their own supplies.
All organisms run on energy. In plants and most animals energy is supplied by proteins and sugars. Human societies require fuel to operate the mechanisms that supply food, warmth, and services. Less complex communities depend on wood for energy, but since the Industrial
Revolution energy sources have expanded to include gas, coal, oil, steam, and fissionable materials.
Mineral resources produce the tools that enable society to supply water, food, fuel, and manufactured goods to growing populations. Exploration and extraction respond quickly to market values of minerals, and market values vary according to technological innovation. Certain minerals will always be needed, but there is a point at which the price of supplying a product to market is more than the price of developing techniques that may render that product obsolete. Because technological developments cannot be predicted in the long run, the need for some minerals cannot be predicted.
Curiously enough, research into the formation of all these resources involves an understanding of fluid movement within solid materials. Separate investigations of the solid, fluid, and gaseous envelopes of the Earth are no longer possible. Researchers now are reorienting their goals to address an earth system that integrates geology, hydrology, and atmospheric sciences with biochemical elements. The breadth of the earth system allows for full appreciation of the consequences of concentration and redistribution of material by natural causes and human intervention.
Often the search for resources is frustrated, and even when it is successful their extraction can be difficult and dangerous. To warrant the effort there must be a degree of profit. As market values change, managers must decide whether a particular resource is economic. If it is economic, it is worth the effort to discover and extract deposits. There are unlimited amounts of all the compounds and elements that humankind needs, but they are not usually concentrated into deposits that are economically worthwhile. If a deposit is worth the effort, or if it can be reasonably assumed that it will become worthwhile, it is classified as a reserve. New discoveries and new extraction techniques contribute to the increasing quantity of what can be considered reserves.
But the rising population continually puts more demand on available resources. As accumulations of waste material grow and concentrations become more hazardous, a new factor affects assessments of a resource's economic value: the cost of landscape reclamation and by-product neutralization. Communities have come to understand that they can no longer dump sewage into streams and let solid wastes accumulate indefinitely. Mining enterprises have come to understand that they can no longer leave mountainsides gouged and stripped, with piles of tailings leaching toxic compounds into the watershed.
As understanding of the earth system grows, resource scientists can moderate the effects of society on nature. One exciting research area is the ability of wetlands to clean contaminated fluids. Wetlands and marshes were once considered useless wasted tracts. If not left to hunters and trappers, these regions were often drained. Researchers have now concluded that the chemical and biological processes that prevail in marshy environments can remove toxic compounds from contaminated water. Constructed wetlands are now being introduced as systems that cleanse wastewater and return acid levels to natural levels. Specific bacteria can clean up specific compounds through bioleaching, biosorption, and bioreduction.
Researchers have developed ways to reclaim mining sites by conservation methods and recycling. Techniques used for extraction of minerals from low-grade ore can be adapted to detoxify contaminated soils as well as water. And mining practices in use now, such as surface landscaping and sealing mine walls to reduce seepage through the mine into the water table, have successfully minimized environmental modification. Incorporating the traditional practice of backfilling spent caverns with tailings to prevent subsidence, modern reclamation efforts strive to recreate the original scene as closely as possible.
Recovery of petroleum resources also can threaten the environment. Oil spills come to mind—the Exxon Valdez spill and the oil spill released into the Persian Gulf during the fighting to reinstate the status quo of the Kuwaiti Emirate. But petroleum geologists are proud of their efforts to reduce the dangers of spillage during the production phase. Today, oil lost to the environment during production is much less than the amount released naturally through seepage. The greatest dangers from oil spillage are found in the transportation phase—and tanker transportation is the most dangerous of all.
The burning of coal produces emissions that accelerate global warming trends. Nuclear energy creates radioactive by-products that can threaten the environment in a variety of ways—as emission into the atmosphere or invasion into the groundwater. Even burning wood causes smog. But nature does have the ability to absorb some of this abuse—it has a carrying capacity. The carrying capacity is the upper limit of a system's ability to support all components within the bounds of available resources. When the carrying capacity is exceeded, a threshold is crossed, and new equilibria establish themselves. Often a new equilibrium spells disaster for the components supported by the former system. For living beings crossing such a threshold can mean extinction.
The carrying capacity of a natural system may be threatened by various means. Natural climatic changes can devastate landscapes and destroy soils just as efficiently as humans can in their roles of gatherer, farmer, or skyscraper builder. Thresholds of stress are crossed whenever a natural disaster hits—earthquakes, volcanoes, and floods all result from natural systems thrown into a different order. Seismologists, volcanologists, and hydrologists experiment and observe, in search of greater understanding of the natural systems, their carrying capacities, and their dangerous thresholds. That understanding should result in the ability to predict the potential for problems, and such predictions might permit mitigation of the intensity of an event—or at least provide time to warn populations to evacuate endangered regions and thus save lives.
The humans who occupy this planet are profoundly dependent on the ordered operation of its natural systems. Human populations exploit the minerals, fuels, soils, and waters, straining against capacities and adapting to limits. That straining and adapting is what characterize life: it's completely natural—as extinction will be, if the human species strains too hard or adapts too slowly.
ROCK-FLUID INTERACTIONS
Traditionally, the science of geology concerned rocks and minerals—the solid part of the natural environment. But over the past two centuries, geologists have become increasingly aware that divisions between solid, liquid, and gaseous environments can be unnecessarily restrictive. The phases are interactive and interdependent; they are not distinct. The nature of the crust cannot be understood separately from the atmospheric and oceanic systems. Most recently, earth scientists have been surprised to detect evidence of organic forms thriving in the depths of the crust and in the heights of the atmosphere. Rock, water, air, and biota interchange molecules throughout a layer of skin covering the planet. The list of interdependent processes lengthens every day as new discoveries are made, and it reflects much geological research—as it is currently defined.
Understanding of rock-fluid interactions began with the study of groundwater flow. Empirical laws were developed in the 1800s to define the rate and intensity of the underground flow feeding springs and wells. Further studies have revealed characteristics of fluid systems that are determined by permeability of the rock, composition and volume of the fluid, and pressure and temperature gradients within the system. These characteristics can be determined close to the surface through wells, but rock-fluid interaction occurring at great depth must be deduced from examination of outcropping rocks that formerly lay far below the surface. Outcrops generally provide evidence of the effects left by deeply circulating fluids, only rarely offering samples of the fluid itself.
Indications from wells and outcrops suggest that fluids may be present in significant amounts at most crustal levels, although they are locally sporadic, and that the surrounding rocks undergo chemical modification as a result of contact with migrating fluids. These conclusions are consistent with observations of the pivotal role played by fluids in the genesis of both mineral and fossil fuel concentrations. Recent geophysical studies have added to the insights about fluids within the crust. Electrical conductivity inferred for great depths suggests a persistent aqueous fluid phase, while seismic reflections from possible low-velocity zones imply abnormally high fluid pressures. This information supports the opinion that extensive fractures and large volumes of aqueous fluids permeate the deep crust.
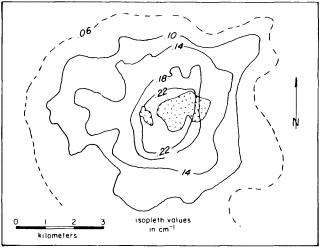
FIGURE 4.1 Vein/joint abundance map of the Sierrita system, Arizona. The total number of fractures per centimeter increase as the pluton (patterned area) is approached. Figure from S. R. Titley, NRC, 1990, The Role of Fluids in Crustal Processes.
Researchers testing the behavior of crustal rock have concluded that fluid proportion decreases with depth, but they do not understand the transition between the extremes of saturation and scant traces. At every level water acts to redistribute heat in hydrothermal systems. Because an increase in temperature raises fluid pressure, any substantial introduction of magma into the crust will initiate a convecting system of groundwater around the magma body (Figure 4.1). The convecting water transports heat from the magma, controlling its cooling rate, which affects the crystallization rate of the rock and thus some of its subsequent identifying characteristics as a solid.
The convecting water also redistributes chemical components from the intrusive body, and from the surrounding host rock, to more remote locations. Even if the surrounding rocks are initially impermeable, the heat generates enough pore-fluid pressure to open hydraulic fractures, creating permeability. Subsequent episodes repeat the whole process until the fractures fill with minerals, permeability decreases, and fluid flow abates. At the same time, the magma must release its influence along a different front to shut down the intricately integrated feedback system.
Horizontal and vertical movement—convection and advection—of fluid through rock disperses chemical components and supports chemical reactions between the minerals and fluids. Dissolution, precipitation, ion exchange, and sorption continue as the fluids migrate through the matrix. Material that does not dissolve may be forced into migration
along the front of the fluid plume; this is what happens to oil and gas. The pressure of the aqueous fluid drives hydrocarbons into reservoir rocks where they collect and remain, if a seal successfully traps them. Otherwise, the pressure of groundwater and their own buoyancy will force them to the surface.
Understanding the role of fluids in the crust necessitates analysis of the interacting thermal, chemical, mechanical, and hydrological processes. Researchers believe that role may extend to influencing, perhaps even initiating, tectonic events. Extremely high pore-fluid pressures, which are characteristic of actively tectonic regions, may facilitate major crustal movements. Frictional resistance to slippage and faulting becomes negligible in certain cases of high pore-fluid pressure.
While seismologists analyze the influence of fluids on fault susceptibility, research continues to reveal how aqueous fluids move in subduction zones and return to the surface through volcanic eruptions. Aqueous fluids also cycle through crustal material at ocean spreading centers, spewing from vents loaded with particulate matter as "black smokers." On continents such fluids bubble to the surface as geothermal springs and geysers.
Ores, those materials that contain valuable metals or other materials, can form by many concentration processes involving chemical reaction with water. Water can seep through soil horizons, leaching solutes away and leaving residual materials such as the bauxite deposits that form aluminum ore. When it reaches solid bedrock, water sustains weathering of minerals and carries away the residue. Deep within the crust, water percolates through the metamorphic zones where igneous intrusions shoulder into the native rocks and contributes to the process of change. And where hot igneous rock and cool saline water make contact along the 40,000-km length of the oceans' spreading centers, researchers can watch minerals precipitate. These observations support analogies that help describe processes characterizing other areas of igneous activity, such as volcanic arcs and continental rifts. Eventually, even the oldest water returns to the surface through uplift, exposure, and erosion; then it quickens again and churns through a shorter episode along the surface.
But once the fluids reach the surface, they do not cease their interaction with the rocks. Oceans continue to pound against the shores, as waves and rocks break each other. Rivers erode the mountains and carry them away. Rain pelts against outcrops, dislodging a grain at a time. Rainwater seeps into fractures and pores, expands on freezing, and thus weathers the rocks mechanically. Water flowing along the surface also dissolves rocks through chemical weathering, forming sinkholes, caverns, caves.
Geologists have always had an appreciation of the links between the solid-earth and its fluid envelopes, but they are now realizing that those envelopes permeate the patches of soil that clothe the continents and the ooze that shrouds the ocean floor. Hydrogen, oxygen, and carbon compose a major proportion of the elements that circulate through the air, ocean, and crust in a variety of fluid forms—coupling into compounds, migrating through pores, dissolving, and precipitating. That circulation of hydrous fluids, in different phases and forms, through the few kilometers between the mantle and space makes this planet what it is.
WATER RESOURCES
The Earth is the water planet. This claim is not based on a mere fluid veneer. The distinctive features that set the Earth apart from other solid planets—the deep, wide oceans; the abundance of living beings; even the buoyant, mutable, silica-rich continents—can be attributed to circulation, and concentration, of water. In the narrow view, water is the most critical resource required for human survival. In the wider view, it is a necessary component of many earth subsystems (Figure 4.2).
Most water exists, rich with salt, in the oceans, and many theorists agree that this has been the rule for nearly all of earth history. Theories proposing a hydrous mantle that slowly generated the ocean waters by gradual degassing do not resolve problems associated with retention of large amounts of water-bearing minerals within the host mantle. Water is continuously cycling through the shallow mantle (Figure 4.3). Lithospheric slabs plunge down into the mantle at subduction zones, notably along the deep oceanic trenches. These slabs, detected by instruments that sense density anomalies, contain water in the form of hydrous minerals—inorganic chemical compounds that incorporate the components of water. The volatile water returns to the surface in complex processes associated with subduction zones, resulting in volcanic arcs that arise where one ocean plate subducts beneath another ocean plate or in volcanic spines that run along the edge of a continent where an oceanic plate subducts beneath it. Certain ancient volcanoes, such as those found in the South African interior, generated material containing hydrous minerals that came from
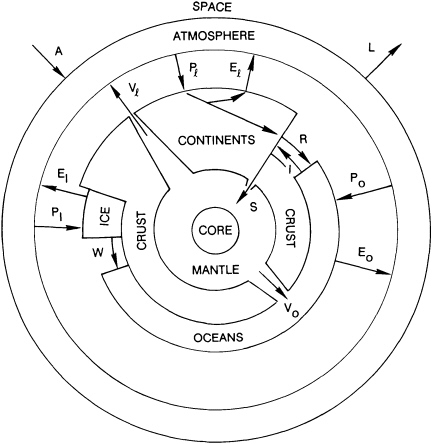
FIGURE 4.2 The hydrologic cycle as a global geophysical process. Enclosed areas represent storage reservoirs for the Earth's water, and the arrows designate the transfer fluxes between them. Figure from NRC, 1991, Opportunities in the Hydrological Sciences.
depths exceeding 150 km. These data suggest that some surface water has been recycled to great depths. Hydrous minerals have even been found imbedded in diamonds that form only at temperatures and pressures found at depths greater than 100 km.
All but a fraction of the water remains at or near the surface in six interacting reservoirs that can be listed according to decreasing volume (Figure 4.4). The largest volume of water—1.35 billion km3—circulates freely throughout the oceans. There is a substantial amount of water frozen in glacial ice sheets and ice caps, but it is still less than 2 percent of the oceans' volume. Permeating the pores and cracks of the crust, groundwater forms a reservoir with 0.7 percent of the oceanic volume. For all the vast reaches of fresh water we swim in and sail on, only 130,000 km3—not even 0.01 percent of all the oceans—fills the lakes and rivers. And only trace amounts, relative to the oceans' vast capacity, cycle through the atmosphere as vapor and through the crust as aqueous fluids.
Water circulates among these reservoirs in a system known as the hydrologic cycle, and it abides in the disparate reservoirs for varying times. In an average year about 60,000 km3 of water is carried over the United States in the atmosphere. This represents an amount sufficient to cover all the land areas to a depth of 30 cm. Of this amount, one-tenth falls as meteoric water—rain or snow. About two-thirds of that precipitation returns immediately as evaporation or moves up through plant roots, carrying nutrients from the soil, and enters the atmosphere as transpiration through leaves. The remaining one-third runs along the surface, accumulating in arroyos, brooks, and creeks. They flow together, gathering as streams and rivers, and pause to form lakes. Eventually, after carving or molding the land and sustaining or sapping the groundwater, rivers run down to sea level, building deltas and mixing with the salty ocean. Ocean, runoff, rain, and vapor in the atmosphere constitute the overwhelming bulk in the hydrologic cycle. The other stores of water involve much slower cycling, although the volumes they contain are substantial. Of particular significance—economically, scientifically, and socially—are the residual products resulting from reactions between subsurface water and the
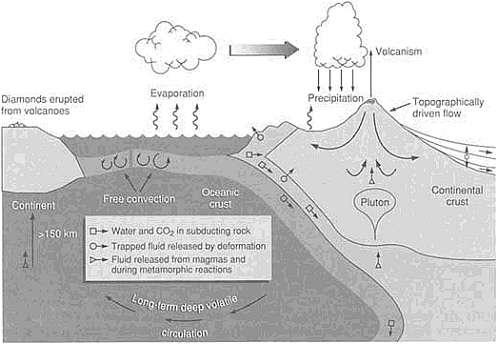
FIGURE 4.3 Circulation of water in a variety of reservoirs. Water and other volatiles return to the surface in complex processes associated with expulsion in subduction zones and related volcanism.
minerals it encounters. The small proportion of rainfall that enters the groundwater reservoir may return to the surface through natural springs or feed dwindling stream flows. Or it may move through rocks of various composition, dissolving minerals and carrying them away. Or it may displace lighter fluids, forcing them into other rocks.
Water Quality
Water circulates through the hydrologic cycle, dissolving elements, sometimes carrying them over great distances, and eventually depositing them into sinks. Earth systems depend on that circulation to constantly supply their moisture requirements. Wa

FIGURE 4.4 The volumes of water in the near-surface reservoirs of the Earth (in thousands of cubic kilometers). The total volume is about 1.5 billion km3; a comparable volume may be dispersed within the Earth's interior.
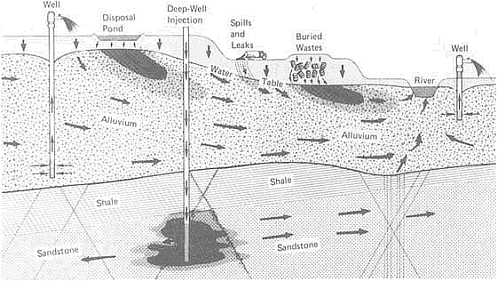
FIGURE 4.5 Schematic representation of contaminant plumes (arrows indicate flow direction) possibly associated with various types of waste disposal. From NRC, 1984, Groundwater Contamination.
ter dissolves salts from soil, carries them to the ocean, and then evaporates, leaving the collected residue to accumulate. Moving through the atmosphere, water arrives again over the land surface to replenish the puddles, rivulets, lakes with fresh water. But within the past few decades Americans have realized that increased concentrations of toxic residues are contaminating fresh water prematurely—long before it can naturally process the material—largely because of the actions of humans.
Traditionally, waste products have been allowed to flow into the nearest body of water. As a consequence, streams, estuaries, and large parts of the coastal environment have become contaminated. Coastal zones, including estuaries, are areas of active sedimentation, and deposited materials often contain toxic wastes. These wastes may seep to the surface or landward into aquifers. They may be exhumed—either naturally or through channel dredging and widening—and redistributed by erosion. Solid wastes placed in landfill sites and other terrestrial depositories are gradually dissolved by moving groundwater; in many cases they contaminate the groundwater supply (Figure 4.5). Because streamflow is often nourished by groundwater, dissolved contaminants can eventually make their way into streams and other water courses.
Groundwater contamination studies have produced many surprises. Volatile organic compounds are mobilized through the atmosphere and may become components of the soil, where they remain for long periods of time. Transport through the soil and groundwater is generally slow, and long periods are required to move contaminants significant distances. Hydrologists agree that once contaminated, groundwater will remain contaminated unless remedial action is taken. Much of the water contamination identified today is the result of waste disposal practices 20, 30, 40, or more years ago.
There is a significant difference between pollution by toxic compounds and pollution by toxic elements. Compounds may spontaneously decompose, through inherent instability, or may be decomposed by heat, biological action, or catalytic properties of earth materials. Either course may result in the loss of toxic character. Heavy metals are elements and remain toxic unless they are immobilized into an insoluble state or detoxified by chelation. Chelation constrains metal atoms within an innocuous but stable chemical species; the heavy metals are tamed or caged by an organic compound that establishes bonds with the metallic ions and eliminates the potential for the metal to react with any other compound.
It is possible that ill-conceived detoxification tactics might accidentally exacerbate the predicament. For example, the native element mercury is toxic but only very slightly soluble. Conversion by industry produces the compound methyl mercury (Hg(CH3)2), which is both soluble and toxic.
Methyl mercury accumulates in short-lived species throughout the food chain with no effect, but when contaminated fish provide the major source food for a human population, mercury concentrations reach malevolent levels. Mercury poisoning results in disorders of the nervous system, mental impairment, and irrational behavior; the mercury compounds used in the production of felt hats during the nineteenth century also produced the stereotypical Mad Hatter.
Plants acquire necessary nutrients by absorbing enriched water from the soil. They return almost pure water to the atmosphere through transpiration and leave unwanted minerals, such as residual salts, concentrated in the soil. Salt buildup is an agricultural problem as ancient as the practice of irrigation. On one hand, it is desirable to apply only the amount of water that the plants actually require. On the other hand, sufficient water must be applied to transport residual salts and minerals beyond the root zone. An effective conservation program in irrigated agriculture requires carefully calculated amounts of water to address both plant requirements and residue removal. Further complications arise when the residual salts or minerals collect in sinks before they reach the ocean. For example, in the Central Valley of California, selenium occurs as a trace element in soils and is mobilized by irrigation water. It moves into the drainage canals and to holding reservoirs where it is concentrated. Eventually, aquatic birds high in the food chain receive large doses of this selenium. Currently, there is no obvious solution to the problem other than to retire a substantial area from irrigation or to flush the reservoir continually into the ocean. Either alternative is costly and temporary.
One major deficiency in our hydrologic understanding and management has been a lack of systematic measurements of water quality. The National Water Quality Assessment (NAWQA) programs are beginning to address this problem. But for all the millions of cubic kilometers of flowing water, the U.S. Geological Survey (USGS) maintains only a small long-term network of approximately 500 gauging stations where water quality is routinely measured. Water samples from these stations are chemically analyzed for the major anions and cations, while some are tested irregularly for traces of metals and organic materials.
To assess the state of the nation's water quality, the Environmental Protection Agency (EPA) relies largely on reports from water quality agencies in the 50 states. The EPA has questioned the adequacy of this information because the states do not share a standardized data base. The agency has begun nationwide sampling to assess the concentrations of particular constituents in surface water, but the appraisal of groundwater is even more limited than that of surface water. Contaminated groundwater has been located at many sites, but there is uncertainty as to the extent of the problem. The contamination is detected in areas devoted to specific land-use patterns, leading to conclusions that are then extended nationwide. This line of reasoning estimates that 0.5 to 2.0 percent of the usable groundwater in the United States is contaminated. Even the higher percentage may be an underestimate: pollutants are generally produced in highly populated areas, while investigations providing the data base tend to concentrate in areas where groundwater use is important, and the two kinds of areas do not necessarily coincide. For instance, there is little information on groundwater contamination in the northeastern urban corridor of the United States, a region dependent on surface water supplies, but common sense suggests that groundwater in that area would be contaminated.
Systematic collection of water quality information is difficult and expensive. The problem is one of complexity. There are several hundred, perhaps thousands, of potentially toxic chemicals for which every water sample could be analyzed. Lakes, rivers, and groundwater all occupy three spatial dimensions that make representative samples expensive to obtain. Water quality also varies through time, so that recurrent sampling is required. And, finally, sampling in an extensive geographic area will probably reveal unsuspected links between various reservoirs.
Three priorities have been established by the USGS: detecting major contaminated reaches of rivers; determining baselines in uncontaminated areas as benchmarks; and detecting groundwater contamination for those parts of the country where rivers or aquifers, or both, provide major water supplies. The cost for this minimal assessment phase is on the order of $100 million annually. This impressive sum is only 1 percent of the approximate $10 billion spent building new sewage treatment facilities each and every year.
Water Supply and Use
Every human being requires about 2 liters of fresh water every day to maintain the minimal physiological functions. But many people use more than that amount; the added quantity varies according to an individual's personal habits and standard of living. In North America every individual consumes or
uses material that requires an average of 1,500 liters of water per day. This total includes the water that cools the turbines of power plants and the water that irrigates cotton fields, as well as the moisture that plumps the artichokes and provides the bulk of milk or muscatel. It is the fluid that showers bodies and washes cars. It drips from faucets and drowns the roots of suburban lawns. When it rains, this fluid runs in silken sheets along the slopes of parking lots and collects in open foundations at construction sites. It gets pumped into the sewer system and mixed with organic waste and then runs out—to someplace downstream. As the population expands, and as material expectations rise, the need for water increases at an exponential rate.
Over the past 300 years, the amount of water removed from freshwater reservoirs by humans has increased more than 35-fold. Most of the water used in North America does return to the hydrologic cycle after only a short interruption. The problem is that it frequently returns in a very different state and often to a very different reservoir. If pure water is taken from a river and returns to the river contaminated by fecal matter or toxic materials, it may take long reaches of that river out of the supply side. And if vast quantities of water are mined from a 100,000-year-old aquifer and run through an irrigation system, resulting in extensive evapotranspiration, the aquifer may not be replenished for another few hundred thousand years.
Because the hydrologic cycle transfers water from one reservoir to another at various rates and because it does not always transfer to the location most convenient for the schemes of civilized minds, humans must use available water resources very carefully. Water cannot be manufactured economically from its component elements and ocean water cannot be desalinated without incurring large, usually unacceptable, expenses. So humans must adapt to the natural limitations on available fresh water imposed by the hydrologic cycle.
In the United States the opportunity exists to deliberately formulate water distribution and wastewater treatment policies according to principles of conservation, wisdom, and justice. The United States uses 770 km3 of fresh water every year. About 340 km3 is consumed—exposed for evapotranspiration or consigned to uses sequestered from the hydrologic cycle. The rest is recycled into the system as wastewater. Irrigation uses 330 km3—41 percent of the total—and accounts for 215 km3 of the consumption. Domestic uses amount to 66 km3, consuming 20 km3; and industry uses 294 km3, but consumes only 29 km3.
Most of the river and lake water becomes available in the spring and early summer when the snow melts, ice breaks up, and rain falls to flush out—perhaps to flood—systems that run low in late summer. Mitigation of droughts and prevention of floods traditionally require water control projects. Management of water supplies—circulating 740 km3 through U.S. cities, suburbs, forests, and fields—necessitates planning that modifies seasonal supply to match independently fluctuating demands.
In the half of the United States west of the 100th meridian—which slices through Texas, Oklahoma, Kansas, Nebraska, South and North Dakota—stream runoff is less than 1 inch in an average year. Throughout that region runoff comes in early spring, largely as snowmelt from mountains hundreds of kilometers away. The water flows through the region months before the optimal time for watering crops. Storage of water—either in artificial surface reservoirs, lakes, or aquifers—dampens the lag in timing between supply and demand. But no reservoirs create water; they only allow a delay of the transfer within the hydrologic cycle. Artificial reservoirs and lakes can be refilled when the runoff returns. Underground aquifers—the groundwater—serve as vast and wonderful reservoirs; but they often cannot be refilled in the next season.
Several western states now depend on mining underground aquifers—taking water out faster than the rate of recharge. In the past, official policy toward groundwater use endorsed the idea of safe yield. Safe yield was a concept accepted by hydrologists as a maxim—an aquifer should not be pumped faster than it is naturally recharged. In the early 1960s this idea was replaced by one that treated underground water as a nonrenewable resource: depletion of groundwater is justifiable if it creates an economy that can afford to buy more expensive water when the well runs dry. Adoption of this maxim reflects the development of irrigated agriculture in the High Plains as well as population migration to the Sunbelt. Now, urban and energy developments are coveting the water available to agriculture—especially in the Southwest.
This competition will undoubtedly intensify, posing two major issues for society: how local, state, and regional communities can manage increased competition for water and to what extent the country can wean itself from irrigated agriculture in the West. The present domestic and industrial water requirements can be effectively met without serious impact on agriculture. Diverting 10 percent of current agricultural water consumption
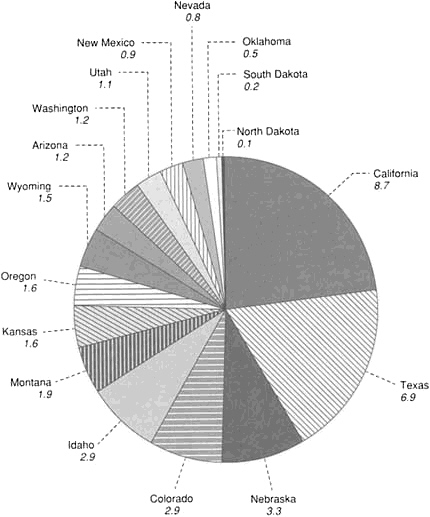
FIGURE 4.6 Amount of irrigated acreage (in millions of acres) in the 17 western states.
would permit a doubling of water use by others. But even without the competitive pressure from population centers, irrigated agriculture is in trouble.
In the western states, irrigation accounts for more than 90 percent of consumptive use, and the surge in groundwater withdrawal over the past 25 years—since the philosophy of safe yield was rejected—has been due almost exclusively to irrigation. In the conterminous United States, the 17 western states consume 84 percent of the country's fresh water, mostly for agriculture. The acreage irrigated in the 17 western states is shown in Figure 4.6. In Texas and California, which account for 42 percent of the total, almost one-half of this water is pumped from the ground. Irrigation is also being increased in the more humid areas of the country to raise crop yield
Hydrologists calculate the volume of freshwater supply in terms of relative depletion. Relative depletion is calculated from total consumption plus the total water exported from each drainage basin, divided by the total input of rain and snow. The ratio is expressed as a percentage. Groundwater is not considered part of the total supply because it is not replaced by precipitation. The exclusion of groundwater from the calculation results in relative depletion that can exceed 100 percent in some areas. Several regions in the United States are considered to be critically depleted; in most of the lower Colorado River basin, in Southern California, and in Nevada, depletion exceeds 100 percent. In south-central California, which includes the San Joaquin and Owens valleys, in the High Plains of Colorado and West Texas, and in most of New Mexico, depletion exceeds 75 percent.
These relative depletion figures are incomplete and probably low, because instream flow requirements are not included. The instream flow requirement represents the minimum streamflow necessary to preserve aquatic and associated ecosystems.
Maintenance of the instream flow is becoming an important constraint on water availability as the sensitivity of individual ecosystems is recognized. A familiar example is the case of Mono Lake in eastern California. Mono Lake is the last puddle left of an inland sea that has occupied the basin for more than 1 million years. During the most recent glaciation 12,000 years ago, the lake reached depths of 250 m and extended over 870 km2. In the course of postglacial warming, the lake level fell, but it has managed to maintain a rich variety of biotic systems thriving in its salty waters. In 1941 the city of Los Angeles—540 km to the south—began to divert the water that feeds Mono Lake. The lake's area decreased nearly 25 percent and its surface fell almost 20 m. If the trend continues, there will be no ecosystem left in the lake: its instream flow requirement will not be met, and the lake will be too saline and too alkaline to support any life. A 1991 court decision has forced Los Angeles to stop diverting much of the water that normally would flow to Mono Lake, which may be sufficient to save the lake. If not, it will share the fate of Owens Lake, which once glistened 150 km to the south. All Los Angeles has left is the lake bed—250 km2 of alkaline salt flats.
The withdrawals from Owens and Mono lakes are only a fraction of the water exported to urban centers. In 1990 approximately 50 percent of the U.S. population depended on groundwater for domestic use. Efforts to eliminate the overdrafts of groundwater in Arizona have led to increasing dependence on surface supplies from remote locations. The Central Arizona Project provides surface water to the Phoenix area from the Colorado River, over 200 km to the west. Arizona has also implemented a new law to eliminate groundwater mining by the year 2020.
California has eliminated relative depletion in the Central Valley by increasing surface water supplies. Southern California continues to import 6.0 billion m3 of water every year. The city of Los Angeles alone extends its water delivery system over hundreds of miles throughout the Southwest and draws the power for the system from projects reaching across six states. It has been involved in disputes over water with the state of Arizona and most recently with Mexico. But in California the cities use only 15 percent of all the water consumed. The rest goes to irrigation. In 1990, 16 percent of the state's water supply irrigated fields of alfalfa, which is used to feed horses and cattle. That adds up to more water than is needed to supply 30 million people for a year. Difficult choices lie ahead for urban and rural Californians as droughts continue.
The southern High Plains represent an area of the United States that will return, sooner or later, to dry-land farming. The transition will come sooner and with fewer ecological and economic crises if the agricultural industry is weaned gradually from its dependence on groundwater irrigation. If nothing is done until all the accessible water in the Ogallala aquifer has been removed, the transition will be ecologically dangerous and economically dreadful. Approximately two-thirds of this water is in Nebraska, which has an enormous reserve of groundwater and is nearest to the most productive recharge area. In Texas and New Mexico more than 10 percent of the water initially in storage has been depleted. However, the depletion statistics for an aquifer such as the Ogallala are deceptively optimistic simply because it has not been economically feasible to remove all the water in underground storage because of the high cost of pumping from great depths. It is estimated that 50 to 60 percent of the water might be removed under favorable economic conditions. Some areas in Texas and New Mexico have already reached the point at which irrigation from the Ogallala aquifer is no longer practical. In such areas of severe groundwater depletion, episodic surface runoff might serve for aquifer recharge if appropriate capture strategies were devised.
Influencing the Water Cycle
Humanity plays an integral role in the hydrologic cycle. While consuming less than 3 percent of the rainfall and returning large portions of that small percentage to the system, humans still profoundly disrupt the natural cycle. Human influence on our water resources is disproportionate to actual use because of the tendency to concentrate efforts in areas where the natural water supply is sparse. The earliest irrigation was practiced in the deserts of the Middle East and was characterized by the same aims as today: to redistribute water geographically and seasonally—in space and time. Mesopotamians built canals and dams that would divert and delay the water flowing through the Tigris and Euphrates rivers. Early Egyptians worked to maintain the ponds and sluices that would trap the flood waters of the Nile and its rich silt. Both the water and the silt would be redistributed over fields at the proper time.
Water may have been a truly renewable resource in early history. Although monsoons and even the annual Nile flood might occasionally fail to take place, they always returned in a year or in seven.
But today hydrologic practices in some areas use water resources faster than they can be replaced. A lake impounded behind a dam may fill with sediment, although the chains of impoundment on many major rivers effectively reduce this risk. And ecological systems downstream of a dam may depend on the natural flood to flush pathogens. Rivers that flow into arctic waters maintain a cap of fresh warm water over the cold saline water that sinks to the bottom. If stream flow were sapped from these systems, it could affect global ocean-atmosphereclimate links.
Awareness of these and similar influences modifying the environment is growing at an astonishing rate, not just among scientists and engineers but in the world at large and especially among politicians. Global change is a concept that has captured attention. Interest has focused on the changes that are likely to follow most directly from the burning of fossil fuel and the related increase in atmospheric carbon dioxide.
Human involvement in modifying the hydrologic cycle has received limited attention as an aspect of global change, but the obvious can no longer be ignored. In the past 100 years human endeavor has destroyed natural streamflow patterns by damming up most of the major rivers of the Northern Hemisphere. Aquifers in arid and semiarid areas have been mined to dangerous levels. Water quality has deteriorated because of the disposal of wastes in streams, lakes, and aquifers, and water salinity has increased in critical areas as a result of irrigation practices. The dissolved salt and the organism components of groundwater are receiving more attention with the recent recognition that irreversible changes are occurring in important aquifers. They are being contaminated by sewage and also by seawater. When fresh water is removed too quickly from groundwater reservoirs along coastal plains, the spaces fill with saltwater. Because of such saltwater intrusions, the cities of Miami, Pensacola, Daytona Beach, Brooklyn, and Los Angeles have had to abandon important aquifers.
Impoundment of a major reservoir behind an artificial dam illustrates how human-induced change affects the hydrologic environment which in turn propagates through the earth system. Impoundment of Lake Nasser by the Aswan Dam was expected to change agricultural practices downstream that had responded to the annual Nile flood for thousands of years. However, the generation of earthquakes caused by the load of the lake on the lithosphere was not considered, nor was the higher elevation of water levels in the Nubian sandstone beneath the desert on either side of the lake. Neither did anyone expect an epidemic spread by snails that had formerly been flushed from the marshy river banks with the annual flood or depletion of the soil and incursion of the Mediterranean (and loss of fisheries) into the delta region because sediment supply had been cut off. On the other hand, rising water levels in the Nubian sandstone have made water wells more productive over a large area. The drought and famine in Ethiopia and neighboring countries upstream from Aswan have not affected Egypt because of the availability of irrigation water from Aswan. The dam has also prevented several dangerous floods.
Humanity can learn from its mistakes. If so, one of its major goals will be that of understanding the complex earth systems well enough to predict the possible consequences of ongoing changes. In the field of hydrogeology, a variety of crises have stimulated accelerated studies and actions, especially an improved understanding of groundwater flow. But many aspects of our water resources remain undefined or even unrecognized.
The geoscientist's role is to understand and synthesize the multiple factors involved in the maintenance of adequate water resources so that policy makers—regionally, nationally, and internationally—can reach informed decisions. The demands of that role represent a remarkable opportunity for newly integrated studies of the hydrologic cycle and particularly for the application of new observational, analytical, and modeling approaches to understanding the groundwater system.
MINERAL RESOURCES
The word mineral can be defined in a variety of ways. One definition includes any naturally occurring substance that is not a vegetable or an animal. The geoscientist defines minerals as all the naturally occurring solids—plus a few liquids—that display distinctive chemical composition and crystalline structure and that are the components of rocks. To the economist, minerals are materials extracted from the Earth that have current or potential economic worth, and any site from which such material can be recovered is considered a mineral deposit.
For this section the most appropriate definition includes economically valuable material that is not considered mineral in the strict geological sense, such as the synthetic open-framework silicates—the types of zeolites that are used as molecular sieves. Mineral resources include the naturally occurring combinations of elements, the ores that contain
metals such as gold and iron. Understanding the location of metallic ores and how to mine them is one of the most ancient fields of geoscientific endeavor. Research involving mineral deposits is similar in some ways to research in oil and gas exploration. Both are strongly affected by economic fluctuations and international politics. When the industries thrive, the larger companies manage extensive research programs that foster close links between exploration projects and scientists from government and universities. At the same time, smaller enterprises can justify ventures that would be considered too risky in a sluggish market. Advances in geophysics, geochemistry, and data-gathering and data-handling capabilities have revolutionized mineral exploration and development, as they have the petroleum industry. Both industries are especially stimulated by applications of new theoretical concepts.
Mineral resources are generally concentrations, sometimes exceptionally high concentrations, of one or more of the materials that constitute the solid-earth. Ninety-nine percent of the crust is made up of only nine chemical elements: oxygen, silicon, aluminum, magnesium, iron, calcium, sodium, potassium, and titanium. It is the other 1 percent of the crust that captures the interest of the mineral exploration geoscientist who wants to know how, when, and especially where concentrations of minerals occur. Interest centers on understanding the processes that form the mineral deposits, the environments in which those processes operate, and the distribution of deposits through space and time. From this understanding, new deposits can be predicted, discovered, and developed, and existing ones can be exploited efficiently.
Mineral deposits form through a wide range of geological processes that may operate at uncommon levels of effectiveness or in unusual associations. In some situations, rocks such as sandstone and limestone qualify as mineral deposits because they are of economic value in a particular place. Sands and gravel deposited in the bed of a mountain stream provide a simple example of such a situation. Where a stream erodes crustal rocks that contain gold, the downstream gravels may contain anomalously high concentrations of the precious metal. This is because the weathered gold particles are denser than the gravels and sands. The stream deposits the gold particles when it loses the capacity to carry particles of that density and size, so the gold accumulates within a particular reach of the stream. These are the placer deposits that have been the sites of gold mining throughout history, from the mythical deposits of Colchis where Jason found the Golden Fleece to those of Sutter's Mill in California that inspired the forty-niners of a century and a half ago.
Less than one part in 10,000 of the metals present in the upper kilometer of the continental crust is concentrated in known mineral deposits. The remainder is widely dispersed at low concentration and, for that reason, is unsuited to economical recovery. Ore deposits are rare indeed! A simple illustrative plot for the metal lead shows how concentration of a typical element varies in the crust (Figure 4.7). The range of concentrations is so large that it is necessary to represent it logarithmically. Common crustal rocks contain about one part in 100,000 of lead by weight; this is represented by the crest of the bell-shaped curve. There are virtually no rocks in which the amount of lead is too small to be measured. The smooth curve represents the gradual increase in lead abundance, with the maximum representing the most probable value for a randomly selected sample. The fall in abundance from this value is not symmetrical. Economically workable lead concentrations—rocks with a lead context of more than a few percent—occupy a place at the far right end of the curve.
Deposits with lower lead content are expected to become economically profitable at some time in the future when all larger deposits are exhausted. A threshold identified as the mineralogical limit separates a deposit in which rocks contain lead-dominant minerals from a deposit of lead so evenly distributed through the rock that it does not form discrete mineral concentrations. Although by definition all lead ores fall within the first category, there is a far greater total amount of lead distributed thinly throughout crustal rocks.
Lead is just one example of crustal element occurrence that can generate a bell-shaped distribution curve. Such distribution patterns indicate the challenge of mineral exploration, which is to discover the small amounts of concentrated materials constituting ore bodies. These concentrations not only are rare but can be extraordinarily localized. For example, seven gold fields covering an area of about 5,000 km 2—no larger than the county of Los Angeles—within the Witwatersrand basin of South Africa have produced more gold than has been discovered over the remainder of the surface. The mercury deposit at Almaden, Spain, has yielded more mercury than any other source and still retains the bulk of the world's reserves. The Bushveld intrusion in South Africa contains 98 percent of the world's chromium reserves, most of them in a single layer.
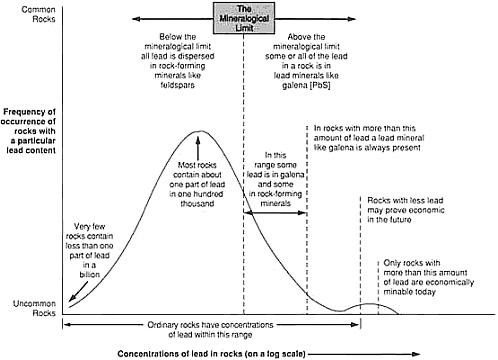
FIGURE 4.7 Schematic grade-tonnage curve for scarce elements; although this curve is for lead, similarly shaped curves also apply to copper, tungsten, chromium, and other metallic elements.
These are unusual concentrations of gold, mercury, and chromium, but researchers question whether they are unique. Such rich deposits represent only minuscule proportions of otherwise ordinary parts of the crust. The possibility exists that similar deposits are hidden in the unexposed basement rocks of the North American mid-continent, beneath the jungles of South America or Southeast Asia, or under thin cloaks of glacial drift in Canada. While exploration has the potential to be a very prosperous undertaking, success in a venture characterized by cryptic clues and expensive tests requires steadily increasing levels of understanding about deposits and their environments.
To illustrate the fact that new sources of strategic minerals continue to be discovered, consider two commodity sources that once were thought to be unique but have proven otherwise: the Climax molybdenum deposit and Southeast Asian tin. The Climax molybdenum deposit at Fremont Pass, Colorado, was for half a century the overwhelmingly predominant source of molybdenum—used in the steel industry—although by-product molybdenum was also recovered in significant amounts from some copper deposits. Despite a ready market, other major molybdenum deposits were not identified until the 1960s, when the essential geological characteristics of the Climax deposit were recognized in numerous other localities. The result was that a series of major molybdenum deposits was discovered and brought into production. Similarly, the marine placer deposits of Southeast Asia dominated the world tin market for years, because they could provide tin more cheaply than any other source. However, in the 1980s the world tin market collapsed, and many Southeast Asian operations were sharply curtailed when abundant low-cost tin emerged from the Rondonia district of Brazil. Thus, molybdenum and tin are examples of mineral resources in which suppliers with no rivals suddenly found themselves downgraded or displaced by more economically competitive sources.
But new deposits are not the only reasons for change. Other factors may include price fluctuations in response to demands or cartels; environmental and other concerns that restrict mining, milling, or smelting; or national policies that encourage or discourage mineral exploration and development. Changes also result from new or improved technologies. Within the past two decades the steel industry
has developed a new technological approach called the minimill. Rather than producing bulk standardized steel, with profit gained through tonnage alone, minimills produce customized types of steel in smaller batches. This means that the steel product does not have to be milled again by the final manufacturer. Minimills are even more profitable when they can be located in regions where the iron and alloy minerals necessary for a specific product occur together or in proximity to each other. Predicting suitable locations for particular minimill sites is a new task assigned to the mineral geologist.
The locations of undiscovered major mineral deposits are among nature's best-kept secrets. Except for bulk materials such as sand or limestone, most deposits constitute minute targets in complex but valueless rocks. Ore concentrations are rare and difficult to find, but their values can be immense. An example is the recently developed Red Dog zinc-lead-silver deposit in the arctic expanse of northwest Alaska, which contains metals worth $15 billion to $20 billion within 1 square mile. The Red Dog deposit is remarkable because high-grade zinc ore was exposed at the surface—and visible to a curious pilot who talked a geologist into flying over some strange rocks. Such obvious occurrences are rare. Most exposures of significant concentrations appearing directly at the surface have long since been discovered by prospectors throughout the world. The tops of shallow deposits commonly are worn by weathering, and their bulk is concealed by soils, gravels, and lavas or is submerged beneath swamps, lakes, and rivers. Unexposed deposits usually indicate their presence only by meager evidence. Thus, earth scientists have the challenging task of detecting mineral caches to maintain society with its basic resource requirements. Just as necessary is the identification of deposits with economic potential, to select optimum strategies for land use. This latter task could help to avoid future conflict involving exploration and exploitation in territory that has been set aside for preservation.
Large sums of money are invested annually in locating and evaluating mineral deposits. The successes are neither frequent nor spectacular, and mining does not rank high in capital return rate. Various exploration approaches can be used in the search for economically viable deposits. Some techniques examine the crust for geophysical or geochemical signatures that suggest the presence of mineralization by identifying fundamental distribution controls. Others define targets within a broad geological context, including direct observations of unmineralized rocks that can serve as outer rings around an unseen bull's-eye of ore. The effectiveness of today's technology depends on the application of tools and the nature of the target. In all cases the greater the understanding about the nature of the crust, the better the chance of discovering worthwhile mineral deposits.
At the present time, an oversupply of many nonfuel commodities exists despite the fact that minerals are being consumed globally at an unprecedented rate. This disparity is partially due to demands that fluctuate more rapidly than supplies produced by the mining industry. Inevitably, the rate of discovery will decline, and shortfalls will emerge once more. While each new technique or concept revives portions of the Earth as prospecting targets, the remaining virgin territories are disappearing.
Understanding Mineral Deposits
Ore deposits can be subdivided into two major classes, epigenetic and syngenetic. The former involve addition of minerals to existing rock; the latter are mineral concentrations that form when the host rock forms. Epigenetic deposits precipitate from migrating aqueous solutions when mineral solubility changes through variations in pressure, temperature, or chemistry. These ores tend to form at contacts between rock types or near the margins of intrusive rocks, generally within a few kilometers of the surface, where metal-rich solutions can mix with cooler surface waters. Epigenetic deposits are associated with near-surface environments. Their host rocks are extremely variable and may lack genetic affiliation with the epigenetic minerals, and they are less likely to provide clues about the mineral deposit.
In contrast, syngenetic deposits, which are generated along with their host rocks, are spread throughout the crust. Any attempt to inventory the crust's mineral wealth must recognize that although surface samples may be valid representations of the abundance of syngenetic mineralization in the crust, such samples are likely to underrepresent epigenetic mineral accumulations.
Recent major advances in understanding the genesis and nature of mineral deposits fit into four general groupings. The first is acceptance of the fact that ore deposits are fundamental parts of the crust. Theoretically, this concept is neither novel nor complex, but its application to ore exploration has been acknowledged only recently. The second advance is the exploration of less hospitable environments—sea-bottom thermal vents, the subsurface of the crust below active hot springs, and lava lakes and flows—that have revealed active ore-forming
processes. Third, a less adventurous but equally significant frontier involves the rapid manipulation of masses of data that can identify intricate correlations and analyze complex processes—leading to improved ore deposit models. Finally, the host of modern tools and techniques, such as stable isotope measurements, ion microprobes, fluid inclusion studies, and age dating systems, allow geoscientists to analyze and select interpretations from the myriad of heretofore untestable hypotheses regarding the formation and distribution of mineral deposits. The application of these four kinds of advances to problems in mineral exploration and exploitation has provided a number of deposit models, which represent application of new ideas to a wealth of new data.
Crustal Processes and Ore Deposits
Plate tectonic concepts have been applied to mineral exploration as associations between tectonic environment and certain types of mineral deposits have become clear. For example, general links between porphyry copper deposits and volcanic arc systems are well established. However, recognition of this relationship does not indicate why one 1,000-km2 tract is more favorable to mineral concentrations than an adjacent area. Often the only effective method for local exploration is a detailed study in the immediate vicinity of a mine or prospect. Combining plate tectonic concepts on a large-scale with detailed geological, geophysical, and geochemical data on the local scale represents a constructive advance in understanding mineral deposits.
As the Earth evolved, the abundances of various types of mineral deposits also changed (Figure 4.8). Until about 2.8-billion-years ago, magnesium-rich lavas known as komatiites erupted on the seafloor and, as they crystallized, precipitated rich deposits of nickel that are now preserved in Canada, Australia, and South Africa. During the intervening eons, komatiites have been essentially absent from the numerous varieties of rocks forming on our planet.
Between 1.8 billion and 2.8-billion-years ago, huge sedimentary banded-iron deposits formed in what are now areas of Australia, Brazil, the former Soviet Union, and the Lake Superior region of the United States and Canada. At the same time, gold and uranium were accumulating as placer deposits. These iron deposits contain the bulk of the world's
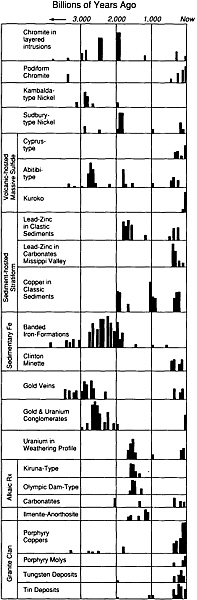
FIGURE 4.8 Distribution through geologic time of several types of ore deposits. The width of each vertical bar represents approximately 50-million-years; the length is a measure of the relative quantity of that type of ore formed during that time period.
economic iron resources, and the paleoplacers include more than one-half of the total known gold resources. No accumulations rivaling in size those banded-iron formations and gold-bearing paleo-placers have been discovered in rocks that have formed since that ancient period.
These cases of mineral formation—distinguished by time of genesis—are relatively straightforward, and there is little likelihood of statistical aberration. An explanation of their formation could be complex, however. For example, it is agreed that the mantle of the younger Earth was hotter than it is today. The very high-temperatures, approaching 1600°C, required to keep komatiites molten could have occurred close enough to the surface to permit eruption of the rock magmas. Formation of the large iron deposits and the gold-uranium-rich placers has been attributed to a different composition of the early atmosphere.
Examples of other time-dependent processes are little more than as yet unexplained correlations. Some well-documented associations between deposit type and geological time may result from a higher probability of exposure and weathering of deposits formed near the surface in continental environments. Erosional surfaces can be recognized, but there is no way of knowing what has been eroded. And because some deposits can be found only in rocks that have formed recently does not prove that the conditions necessary for their formation have occurred only recently. Deposits such as the Mississippi Valley type appear only in relatively young formations, but similar deposits formed in the past may have been subsequently removed from the geological record.
The ores of the Mississippi Valley type, as exemplified in southeast Missouri and the Joplin region of Oklahoma, Kansas, and western Missouri, are prime sources of zinc and lead within the conterminous United States (Figure 4.9). The ores result from the filling of solution cavities in carbonate rocks with metal-rich material at relatively low-temperatures (90° to 125°C). Recent developments that have contributed to understanding this process include the discovery of brines rich with zinc and lead in deep petroleum exploration wells drilled throughout the region, recognition that metal-bearing brines have permeated carbonate rocks extensively through the midcontinent regions, and advanced modeling of fluid flow in compacted sedimentary basins. These scientific developments have produced a refined model of mineral deposition based on the expulsion of metal-bearing brines from a sedimentary basin that concentrates mineralization around the margins of the host basin. The more eastern Mississippi Valley-type mineralization was probably emplaced as a discrete pulse during the collision of an island arc with North America about 450-million-years ago. The larger western episode of mineralization was related to the collision of Gondwana with Laurentia, during the assemblage of Pangea about 300-million-years ago. In both dramatic events, mountains depressed the North American continental margin and drove subsurface fluids toward the interior of the continent.
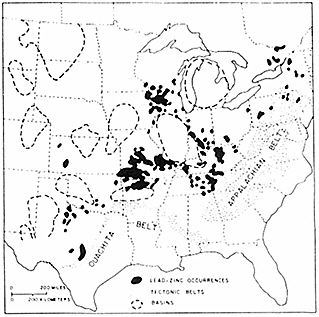
FIGURE 4.9 Map showing the relationship between Mississippi Valley-type lead-zinc deposits and tectonic belts and basins. After J. E. Oliver, NRC, 1990, The Role of Fluids in Crustal Processes.
Researchers suspect that the cessation of komatiite eruptions resulted from the long-term cooling of the Earth; other changes, such as the closing of ocean basins during continental assemblage, are cyclic. Another example of cyclic change that affects mineral deposition is the global warming that dissipates polar ice caps. Such a warm period occurred 100-million-years ago. Sea level was high; circulation of cold, well-oxygenated polar water toward the equator was diminished; and the deep oceans were warmer than the near-freezing temperatures of today. This caused stagnation in the deep basins; with a continual supply of organic matter from dying organisms near the surface, the deep waters became chemically reduced. This permitted the reduction of manganese oxides on the seafloor to form the relatively soluble divalent manganese. Solution progressed and the manganese content of
the deep seawater increased. As upwelling manganese-rich water crossed shoals near the continents, it mixed with oxygenated surface water and reprecipitated the manganese, yielding the giant manganese deposits of Chiatura and Nikopol in the former Soviet Union; Groot Island, Australia; and Malango, Mexico.
At convergent plate boundaries, a plate passes beneath another overlying plate and descends into the mantle. The lower plate heats enough to drive off water—water that lowers the melting temperature of the rocks above the site of dehydration, which produces a series of volcanoes parallel to the plate boundary. The volcanoes ringing the Pacific Ocean form an excellent example of this type of pattern. In a terrestrial setting the roots of the volcanoes can produce a complex of porphyry copper, skarn, and replacement deposits of both precious and base metals. These deposits are part of the crust that is elevated, so they tend to become eroded and destroyed in a geologically short time. Thus, even though such deposits may have continually formed over much of earth history, the preserved record is almost entirely young.
Genetic Studies of Ore Deposits
The record of the geological past is cryptically retained in the rocks of today. The geologist's challenge in interpreting mineral deposits is to determine what happened, when it happened, how it happened, why it happened, and where else it might have happened. Investigations aimed at answering these questions are termed genetic studies.
Genetic studies attempt to decipher distinguishing characteristics that will provide details about the processes involved in ore deposit formation. Over the past 30 years one of the great accomplishments in the area of genetic studies has been in the field of geochemistry. The original temperatures, pressures, and chemical compositions of the fluids that deposited ores can be determined by detailed interpretation of existing mineral assemblages, particularly from fluid inclusions, by the use of isotopic and other chemical tracers, and by the analysis of organic interactions.
Fluid inclusions are tiny amounts of fluids or gas trapped within a mineral. They are very common; jewelers distinguish between natural and synthetic gems on the basis of the presence or absence of fluid inclusions. Primary inclusions are those trapped as the host crystals grow. Secondary inclusions form as scars after a crystal cracks and is healed by subsequent crystallization. The hot liquid from which the crystals formed cools, shrinks in volume, and releases gas—generally steam—to form a bubble. When crystals are heated on a microscope stage, the liquid within the fluid inclusion expands. When the fluid fills the cavity and the bubble disappears, it indicates the temperature of mineral formation. Crystals form within the briny fluids. Sodium chloride and other salts precipitate as the crystal cools and are present in the inclusions as daughter minerals. If the crystal is chilled and the liquid frozen, the freezing temperature reveals the total salt content of the liquid. Microchemical and electronic instruments have been devised to analyze the fluids chemically and isotopically. The results of such analyses may indicate the physical and chemical nature of the host rock's genetic environment and thus possible sources of the fluids.
The isotopic and trace chemical signatures of mineral deposits are complex, and that chemical complexity is used by geoscientists to identify possible sources of deposits. Stable and radiogenic isotopes are the most useful tracers, although other indicators such as rare earths and the platinum-group metals are also used to constrain models of mineral genesis.
Stable isotopes exhibit patterns that are readily distinguished in terms of various processes and sources, thereby providing fingerprints that enable geoscientists to decipher processes. As an example, water escaping from magmas is depleted in deuterium relative to the amount in seawater, and meteoric water—rain—of the American cordillera is depleted by comparison to the deuterium amounts from magmatic water. Thus, by measuring the deuterium fraction of hydrous mineral fluid inclusions of cordilleran mineral deposits, it can be determined whether the original fluid was seawater, magmatic water, or rain.
Radiogenic isotopes, principally lead and strontium, are important in geochronological methods, but they also possess distinctive signatures that record their heritage. The rare earths share similar chemical properties and tend to remain closely associated through most geological processes. Thus, the ratios of rare earths to each other can be measures of processes related to mineralization. Ratios between platinum-group elements constitute a similar sort of signature.
The role of organic compounds in the formation of ore deposits serves as an outstanding example of how studies of mineral deposits are enhanced by other sciences. Organic matter can mobilize or fix metals directly; it can also control the oxidation or hydrolysis state of the system. Organic matter may
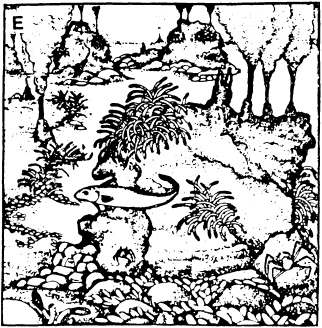
FIGURE 4.10 Processes active at the scale of individual vent fields. Hydrothermal venting (plumes) and low-temperature, diffuse venting (at tube worm/clam beds) release significant heat to the deep ocean. Massive sulfide-sulfate precipitation can result in the growth of complex vent structures or simple chimneys when high-temperature fluids mix rapidly with seawater.
supply sulfur that is subsequently incorporated into sulfides. Thus, organic matter has a powerful control over the mobility of metals in the crust, especially under near-surface conditions. One of the most exciting research frontiers in organic geochemistry is the development of engineered bacterial species that leach metals from ores—dubbed biometallurgists—or that precipitate and isolate metals from waste—biotrashmen, of course. The challenges, opportunities, and rewards in this field are great and are being vigorously pursued.
Organic matter also has a passive role in the search for and recovery of minerals, as a guide to conditions instead of as an active agent. For example, the thermal maturation of organic matter can provide a guide to related ore-forming thermal events. In addition, living or fossil species provide clues to the environment prevailing at sites of ore deposition. One of the best guides to active deep-sea smoker sites is the distinctive biota of giant white clams, tube worms, and deep-sea crabs. Fossils of that distinctive biota provide telltale evidence that mineral deposits formed at ancient ocean spreading centers may lie nearby (Figure 4.10).
Finally, geochemists have refined laboratory experiments that attempt to duplicate natural processes. Experiments in genetic studies use two broad but contrasting approaches. The first is to design and reconstruct a natural process and then use the reconstruction as a model. The second is to assemble the assumed necessary thermodynamic and chemical data and then calculate just how nature might perform under those conditions. With the second method, developing a model is a process of trial and error. The first method is most effective when the natural system is well established and reactions are rapid. Unfortunately, nature presents major obstacles to the study of certain natural processes: unrestricted time spans and complex environments. Nature has taken millions of years to weather a rock or to crystallize a glass, while experiments requiring more than a few months are difficult to maintain, and studies lasting years are rarely funded. Natural environments simultaneously involve high-temperatures, high pressures, and corrosive chemistries; only some of these combinations that alter and dissolve rocks can be duplicated in the laboratory.
The solution to this problem has been to combine several experimental approaches in the laboratory. Artificial systems can be simplified to eliminate extraneous factors for short periods. For instance, experiments can be performed at high-temperatures, where the reaction rates provide definitive results in relatively short time frames. Thermodynamic data can also be acquired that permit extrapolation of high-temperature results from experiments performed at lower temperatures or to a range of pressures and chemical reactivities.
Models in the Study of Mineral Deposits
Humankind has searched for minerals throughout recorded history and undoubtedly developed prospecting theories very early. Perhaps the approach was to search for another gold nugget in a stream in which one nugget had already been found. Then came the idea that other streams might have similar nuggets—the first germs of an analog model. Intensive prospecting in and around a stream might have led to an outcrop of gold-bearing quartz vein or perhaps to finding a nugget still attached to a piece of vein quartz, and eventually to the idea that there might be more than one place to look for gold. In this way the idea of alternative descriptive models would develop, one for stream placers and another for veins. Such models continue to develop today, and just as the first gold-bearing vein was a new kind of deposit to ancient miners, modern research-
ers continue to discover and develop new deposits. An ore body is defined as a new class of deposit if, in the absence of knowledge of its mineral content, an experienced exploration geologist would not recognize its economic potential. Even with current supposedly sophisticated knowledge of the Earth, new classes of deposits continue to be discovered. Classes recognized for the first time during the past 30 years include unconformity-related uranium, Olympic Dam type of ore, Carlin-type gold, salt-dome sulfides, and sediment-hosted tungsten.
Unconformity-related uranium deposits contain the largest known concentrations of uranium. The world's richest and largest known deposit is in Canada at Cigar Lake, Saskatchewan. It contains 150,000 metric tons of uranium metal in the form of high-grade ore—8 percent U3O8. This type of deposit also occurs in Africa and Australia but was not recognized until 1968.
The Olympic Dam deposit, an iron-rich breccia in South Australia, contains more than 1 billion tons of ore rich in copper, gold, uranium, and rare earths. No portion of the deposit was exposed, and it was discovered by drilling located on subsurface regional lineaments and magnetic signatures.
The Carlin-type gold deposits of Nevada are low-grade, large-volume deposits containing very fine-grained gold. Now the source of most of the current U.S. gold production, they were recognized only recently, although a few high-grade zones had been mined in the region during the past century. A major contributor to the successful production from such low-grade resources has been the advent of heap or pad leaching, which extracts the gold from the ore at minimal cost.
The cap rock of salt domes in the Texas Gulf Coast has been found to contain lead, zinc, and silver in minerals whose quantities and grades approach the profitable. The existence of similar sulfide concentrations in other salt-dome cap rocks is anticipated.
The largest known tungsten deposit in Europe, at Felbertal, Austria, was discovered relatively recently in metamorphosed sedimentary and volcanic rocks. This deposit of sediment-hosted tungsten is remote from the igneous rocks with which other tungsten mineralization is closely associated.
Thus, nonconventional ore deposits continue to be found, and prospects continue for discovering additional unique mineral concentrations. Such ore bodies provide a stimulus for continually revising the conceptual models of mineral deposits. Revisions either validate hypotheses embodied in existing models or generate completely new models and hypotheses to be tested.
Studies that build models for mineral exploration have several similarities with petroleum studies—questions about source, migration, and traps. However, mineral deposits are generally more complex because there is not a single source material, as there is in petroleum formation. Minerals may originate in the mantle or in the crust and may represent concentrations in a particular environment, such as dissolved salts in the ocean, which are concentrated by evaporation until they form salt deposits called evaporites. Transport processes are equally varied, as are controls on the deposition of mineral accumulations.
The variety of possible formation environments for copper deposits can illustrate the complexity of mineralization processes in contrast to the relative simplicity of petroleum development. Major copper deposits occur in primary or altered sedimentary environments, in veins within rocks of all types, in products of seafloor hot-spring vents, in segregates from mafic lavas as native copper, and in other distinct geological settings. This multiplicity of host environments suggests a variety of deposition and transport processes, which complicates the task of inventorying copper resources. Deposit types for other metals are just as diverse, requiring the design of various models and consideration of many alternatives. The source, transport, and accumulation processes that concentrate minerals in valuable deposits are seldom fully understood. New ideas that seem to answer outstanding questions form the bases for new models.
Transport, or migration, involves two basic steps. The first is mobilization of materials by means of a carrier, such as the solution of metals from source rocks into aqueous fluids or igneous melts. The second step involves movement of the carrier to the site of deposition. Research into transport by aqueous fluid is based on the recognition that the common ore minerals are extremely insoluble in pure water and that complex ions are required to increase solubility. Most of the ore minerals in vein deposits are sulfides, so sulfur chemistry is particularly important. Aqueous sulfur chemistry is strongly influenced by an environment's oxidation-reduction status and by its acidity. Reactions with wallrock minerals, water from sources including groundwaters, and loss of boiling gases are important factors in metal transport and eventual deposition. At temperatures above 400°C, ionic links tend to be replaced by molecular links, and solution chemistry becomes simpler in concept but experimentally very challenging.
Transport by means of igneous melt is even more
complicated. Magmas consist of partly molten rock, and they transport a variety of elements, including tin, molybdenum, iron, nickel, copper, the rare earths, and the platinum group. Such metals may then either precipitate directly from the magmas into magmatic deposits or be incorporated into aqueous fluids as the magmas solidify.
Whether aqueous fluid or igneous melt acts as the carrier in transport, the mineral must reach a trap and accumulate in significant concentrations before a deposit can be considered economical. These traps are the targets of mineral exploration. A great deal of progress has been made in quantitatively modeling individual accumulation processes, such as boiling, cooling, mixing with dilute waters, reactions with wallrock, adiabatic expansion, and sulfurization of metal-bearing magmas. These individual process models require considerable additional data before they can be integrated into a comprehensive form that can handle all the known variables together.
Computers, using data bases on both fluid and mineral characteristics, have enabled modeling the complex geochemical and hydrologic processes related to ore formation. For example, the flow paths, flow rates, and residence times for fluids can be predicted, given a geological starting point such as a sedimentation interval within a basin or the intrusion of a granite magma into a sequence of rocks. The anticipated temperatures, pressures, and minerals can be calculated for every point within a flow system. This exercise is analogous to watching a mineral deposit form. It is also an experiment ideally suited to predicting the behavior of chemical wastes injected into rocks and of fluids used for in situ mining.
Mineral Exploration and Exploitation
Mineral deposits display great diversity in material; grade; size; and style of localization, accessibility, and minability. These largely independent variables complicate the search for profitable mineral concentrations. The ideas developed by early geoscientists about the origin of mineral deposits were based on surmise because only a few cases of ore formation could be observed directly. These few included placers forming in streams, volcanic fumaroles yielding sulfur and metallic sublimates, and dried playa lakes and marine lagoons depositing salt. Additional examples of direct observation of ore formation have been recognized in recent years as exploration of our planet has reached into even the most hostile environments (see Figure 4.10).
Since 1979 spectacular discoveries of huge thermal springs have been made at spreading centers along the mid-oceanic ridges in the Pacific and Atlantic oceans. Heat from subseafloor basalt creates a massive circulation of hot seawater, and reactions between the water and the basalt form acidic solutions. Leached metals and sulfur from the rock are deposited from vents on the seafloor above the spreading center to form cones containing iron, copper, zinc, gold, and other metals. Active vents, which range in temperature up to 350°C, are characterized by rising plumes of rapidly precipitating sulfides, graphically called black smokers. These precipitates resemble the deposits hosting the copper ores that gave Cyprus its name as well as other minerals valued since ancient times. These long-familiar mineral deposits are now recognized as ocean-floor rocks formed at extinct spreading centers and then incorporated into the continents at arc and continental collision sites. Variants occur in Mexico's Gulf of California and off the continental shelf west of Washington and Oregon. Close to continents, active sedimentation buries the spreading center, resulting in mineralization that is disseminated throughout the sediments as well as around the black smokers on the seafloor.
Of even greater economic interest are the vents associated with rifts along the crests of island arcs, such as that in the China Sea west of Okinawa; they are associated with magmas that are richer in silica than ocean basalts. These magmatic heat engines are apparently larger and longer lived than those immediately above oceanic crust and could produce deposits similar to the Kuroko-type massive sulfides, rich in copper, zinc, lead, silver, and gold. The Kuroko deposits in northern Honshu, Japan, formed about 13-million-years ago when a rifting episode split the axis of the island arc. Oceanic waters flooded the rift to a depth of more than a kilometer, and the massive sulfides were deposited where seawater and magma interacted.
Another mineral deposition environment occurs in deep depressions along the axis of the Red Sea. The water descending to the heat source along that axis dissolves large amounts of rock salt from deposits buried along the margins of the sea. The resulting brine forms dense pools at the bottom of the Red Sea. Large amounts of zinc and iron are precipitating in the brine pools, demonstrating that a deep stratified brine environment may have been a progenitor of the ancient strata-bound ore deposits exposed on the continents.
Active thermal springs and solutions encountered in the course of drilling for geothermal power
sources locally contain erratic quantities of ore-grade gold and silver mineralization similar to the deposits of the Comstock silver and gold lode in Nevada and the McLaughlin gold-bearing region of California. Chemical and physical observations from these geothermal operations have shown how water is heated by igneous bodies, alters host rocks, mixes with surface water, and precipitates minerals. We are provided with a peek at an ore-forming environment originating tens to hundreds of meters below geysers and bubbling springs. Gold has been discovered, in concentrations equivalent to relatively high-grade ores, in muds at the bottom of hot springs in Japan, New Zealand, and Yellowstone Park.
In particular types of ore bodies the concentrations of metal grades—the relative quantities of mineral in the ore deposit—are likely to extend over a limited range. The total tonnage of an ore body is also characteristic of particular classes of deposits. Both grade and tonnage can vary considerably, but if statistically sufficient examples of a particular type of ore body are available, the likely grade and tonnage limits for a yet-to-be discovered mineral deposit can be estimated. This is an extremely useful capability for the mining industry because it can supply guidance prior to the application of standard procedures for determining grade and tonnage of an ore body such as drilling, trenching, and extensive sampling. For instance, more than 400 Kuroko-type ore bodies have been recognized in rocks ranging widely in age in many parts of the world. Data plotted from the known Kuroko ore bodies show that the median size of a Kuroko ore body is 1.5 million tons, at an average grade of 1.3 percent copper (Figure 4.11). The tabulation also predicts that few Kuroko ore bodies can be expected to exceed 18 million tons or to contain grades of more than 3.5 percent copper.
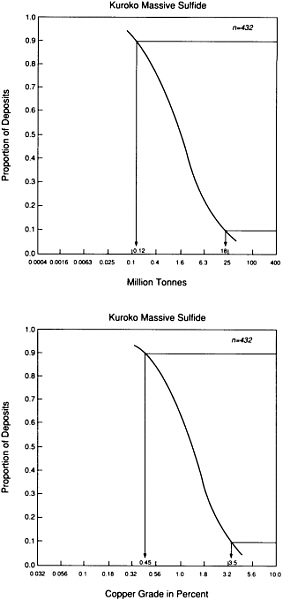
FIGURE 4.11 Ninety percent of Kuroko-type deposits have between 0.12 million and 18 million tonnes of ore (upper) and have ore grades (lower) between 0.45% and 3.5% of copper.
Grade and tonnage models can also be applied to ore bodies whose origin is not as well understood as the Kuroko-type deposits. This is illustrated (Figure 4.12) for the Carlin-type ore bodies, which consist of mineralization emplaced in carbonate rocks. The population considered in this model is virtually all from Nevada and is considerably smaller than that used for the Kuroko estimates.
Exploration is a risky business, and those who minimize the risks are generally the most successful. Traditionally, exploration methods have emphasized empirical field-documented analogies with known deposits, supplemented by diagnostic geophysical and geochemical signatures. These methods have offered the lowest feasible exploration risks and have proven very successful, as evidenced by continuing discoveries in recent years. However, exploration has been literally skimming off the top of the crust, discovering and developing the deposits that are most easily detected and exploited. Few prospecting programs have succeeded in extrapolat-
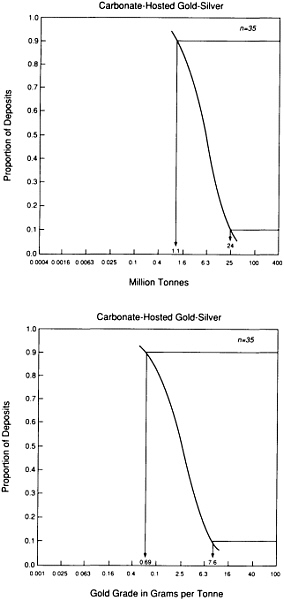
FIGURE 4.12 Eighty percent of Carlin-type gold deposits in Nevada are between 1.1 million and 24 million tonnes of ore (upper) and have ore grades (lower) between 0.69 and 7.6 grams of gold/tonne.
ing concepts into uncharted ground, applying more sophisticated models to predict unseen targets, and following up with persistent exploration.
One approach utilized in exploration for concealed deposits is shared by both the fuels and metals industries: the search for paleothermal events. Petroleum is formed from organic matter in sediments by gentle thermal maturation, so the parts of basins that have been simmered offer the best prospects for finding petroleum. In a similar way, metal deposits are often characterized by the superimposition of thermal events on rocks that, both before and after the mineralization, were relatively cool. An examination of rocks for evidence of a former thermal event may prove useful in locating buried mineralizing systems.
In mineral exploration, genetic concepts have been augmented by empirical methods that observe what has been overlooked at the surface, seek clues about deposits that are only thinly veiled, and attempt to detect the presence of deeply buried deposits having no surface expression. Geophysical methods are foremost among the subsurface sensing techniques, particularly where the geology is well known and the nature of the target can be identified by surface examination.
Geological observations of the surface serve as the foundation for the science of mineral deposits. Geophysical techniques can sense subsurface characteristics of the crust through various methods. Ground and airborne geophysics have their most dramatic successes in the direct discovery of ore deposits through detection of particular physical properties. Magnetic surveys have discovered numerous deposits bearing magnetite (Fe3O4) and pyrrhotite (Fe1_xS), and negative anomalies have identified magnetite-destructive alteration in igneous intrusions associated with other types of deposits. In the search for massive sulfide and gold-bearing deposits, increased use has been made of deeply penetrating pulsed electromagnetic surveys and of airborne surveys using a combination of airborne geophysical methods. Electrically conductive bodies have been found using induced polarization, audiomagnetotelluric, and controlled-source audiomagnetotelluric methods. There is an amazing variety of instrumentation and signal and detector array configurations.
In areas that are concealed beneath thick sedimentary deposits—such as the basement of the North American midcontinent—geophysical characterization of the region is an important adjunct to direct detection surveys. Both techniques can identify features related to mineralization. Even where the geological picture is well known, the geophysical characterization often provides provocative new information to upgrade the geological interpretations. High-speed computers permit the processing and graphic display of geophysical data, making geological interpretation and ore search easier. The
increased resolution and penetration of geophysical methods will enhance the search for, and evaluation of, mineral resources.
Remote sensing from either space platforms or aircraft offers another tool to consider for both reconnaissance and detailed examination of terrains. Techniques include side-looking radar and synthetic aperture radar, which reveal information about the geological structure in poorly exposed terrains, and a range of optical and infrared detection systems. A variety of multispectral instruments, including Landsat, can detect differences in vegetation that reveal nearby mineral concentrations and are capable of distinguishing the mineralogy of some altered rocks.
The examination of natural materials such as soils, stream sediments, and stream waters for traces of economically valuable metals or, more commonly, for pathfinder elements associated with the sought-for metals, is a widely used exploration technique. The method identifies primary anomalous patterns generated by the mineralization, as well as secondary patterns resulting from dispersion due to weathering. Soil-gas geochemistry applicable to mercury, sulfur gases, and radon is a developing area of mineral exploration.
The actual physical mining of ore bodies is certainly one of civilization's oldest primary industries. Surprisingly, the techniques used from earliest times barely changed until after the Industrial Revolution, well into the nineteenth century. Since the advent of the Iron Age, miners have descended into shafts that invade the crust. Working in closed spaces with poor ventilation and high-temperatures, miners have borne conditions that threaten lesser souls on a primal level. The traditions of miners and smelters are still with us in names such as nickel, taken from Old Nick, and cobalt from Kobold, a goblin, and in the only recently violated superstition that forbade women to enter mines. Many cultures relate tales of mining activities—that of the seven dwarves in one famous story or that of searching for ores with special power to work into swords, rings, or other talismans.
At first miners dug the ore from the walls with picks and shovels. They piled the rock in baskets and carried it out on their backs, sometimes up ladders of dizzying heights. Then they dug the holes a bit larger and trained draft animals to carry the piles of rock to central shafts where pulleys and ropes were used to haul the ore up to the surface. Picks and shovels were used to dig all the rock until the late nineteenth century, when hydraulic methods, engine-powered haulers and lifters, railroad cars, and elevators were developed. But even today there are many tight spots underground that can be reached only by a miner with a tool in hand. It can be a dangerous job. Dangers can include the collapse of roofs, bucking of floors, and seepage of gas, which could explode at the slightest spark, into the caverns. Because of such dangers, subsurface hand mining has become limited today to situations where no other method can be applied.
The discoveries and developments of the past quarter century have transformed modern mines into a different type of operation. Now surface mining produces a large proportion of society's mineral material. Heap leaching is a typical approach. Whole chunks of the surface are torn loose by a machine-driven shovel that fills a dump truck or railroad car in one scoop. The rock is transported to a crusher where it may be sieved and mixed with chemical catalysts. The crushed material is dumped onto a pad and treated with a spray of another chemical that will bond with the desired mineral, leach through the entire heap, and drain along the impermeable pad, leaving the unwanted material behind. The altered but separated mineral then collects in another receptacle where further treatment, either chemical or more commonly electrolytic, isolates the valuable metal.
Surface mining and separation methods such as heap leaching produce large amounts of minerals with negligible risk to the on-the-job welfare of miners. But this method could leave tracts of scarred landscapes, vast piles of rock debris, reservoirs of toxic liquid, and drastically altered environments as its by-products. As these are unacceptable, the mining industry has developed approaches that can ameliorate the effects. The industry's efforts to repair damage resulting from its activities have led to research forming the core of environmental engineering. One of the goals of such environmental engineering and related subjects is not limited just to amelioration. With enough foresight in planning complete mining operations and with dedication to prevention of hazardous and wasteful by-products, surface mining—when necessary—can be a benign activity within a reasonable time scale.
At the close of the twentieth century subsurface and surface mining are providing the bulk of needed mineral resources, but researchers are looking for breakthroughs with in situ mining techniques in the future. In situ mining is analogous to the hydrothermal mineralization process but works in reverse. It uses fluids to extract minerals without actually destroying the host rock. Strictly interpreted, this method is not new, because steam has been used to
melt and extract native sulfur, and chemically tailored extractive solutions have been used to dissolve rock salt, potash, copper, and uranium. In situ mining of other deposit types has been characterized by poor recovery, but the minimal environmental impact as well as the ability to mine under extreme temperature and pressure conditions make in situ techniques attractive for some deposits. The two major problems are reaching the sought-after mineral grains, which may be widely dispersed or chemically inactive within a rock, and finding the appropriate solvent to release ore minerals while not adversely affecting the environment. Successful applications of in situ mining have been limited so far to naturally permeable rocks, because attempts to create fracture permeability have generally failed, and to disseminated rather than massive mineralization. Cultivating bacteria to facilitate metal extraction represents a current and challenging opportunity for applications to in situ mining, heap leaching, and by-product recycling.
ENERGY RESOURCES
The human species depends on energy. Advanced societies consume fuel on a scale that represents one of the greatest single changes on Earth over the past century. Modern consumption habits have developed because geoscientists have been able to discover and exploit energy resources with remarkable success. However, this success entails some adverse consequences. Few resource developments have affected the environment more than the burning of coal, gas, and oil.
The two main classes of energy sources are related to the external and internal engines of the Earth. A combination of external and internal engine components produces hydroelectric power. The topographical relief caused by cycling of the Earth's internal engine collects and channels river systems, which are elements of the externally driven hydrologic cycle. Dams are built to interrupt the downward river flow and harness the ensuing energy potential. The Sun, the Moon, and the Earth's spin—components of the external engine—generate solar energy, tidal energy, and wind energy. Wind power and solar power have recently come of age. Large wind farms can produce electricity at a profit. They are especially suited to flat open spaces such as western North America, northern Europe, North Africa, India, and the former Soviet Union. Similar environments, with the added characteristic of negligible cloud cover, also can support large thermal solar collection installations. Small-scale solar collectors are becoming more common, especially in other parts of the world—India reports the use of solar energy systems in some 6,000 villages. Tidal energy is still in the planning stage, with trial installations testing the suitability of various designs.
These energy sources are renewable and are generally harmless in a global environmental sense, although the concentration of collection facilities does alter the local landscape. However, in most situations, they are not yet economically competitive with fossil fuels. Fossil fuels are our main energy source. They are produced primarily by the external engine of the Sun, but they are also affected by the slow consistent heat of the internal engine. Oil, gas, and coal are all derived from organisms that relied on solar energy millions of years ago. Accumulations of such organisms provide the most economical source of energy today, but the burning of fossil fuels releases abundant amounts of carbon dioxide into the atmosphere. The quantity of carbon dioxide produced by industrialized society may cause significant global warming, which in turn could produce undesired environmental effects.
The interior of the Earth is hotter than the surface, although the rate at which heat reaches the surface from the Sun is at least 10,000 times greater than the rate at which heat emerges from inside our planet. The source of the internal heat is largely the slow decay of naturally radioactive nuclides. Gaining access to this natural decay of radioactive nuclides, with the goal of harnessing the resultant excess energy, remains a great challenge of the future.
Uranium is the most abundant natural source of available radioactive nuclides. The controlled fission of 235U in nuclear power plants has been used as a source of energy for more than 30 years. The most vexing problem involving nuclear power is long-term disposal of the radioactive waste. Although there are several proposed methods for the treatment and isolation of such waste, none have been licensed in the United States, both because of technical uncertainties and social and political resistance. Without an appropriate solution to the problem, public utilities are not investing in developing new nuclear power plants nor are operational licenses being granted.
The heat emanating from the Earth's interior is an attractive energy source. This geothermal energy can be used for electrical generation or direct space heating. It is a relatively clean source of energy, and large amounts are potentially available. Present use of this source is limited to areas where partly
molten, or recently crystallized (in a geological sense), rock heats groundwater that carries heat toward the surface where it can be harnessed to generate electricity. Few locations can claim the coincidence of both hot rocks and groundwater; consequently, geothermal resources have been developed only locally. Research in geothermal energy includes the use of magma as a direct energy source and the extraction of energy from hot dry rock by injecting water and recovering it to extract the heat.
Earth scientists will continue to address the nation's energy needs through the discovery of energy resources, their assessment, and their economic development in an environmentally sound manner. Fulfilling these responsibilities calls for an adequate scientific understanding of the environment in which the resource occurs. An appreciation of the past—how that environment and the resource have evolved over geological time—complements the anticipation of the future—how resource development will affect the environment.
Growth of the worlds population and rising per capita energy use will lead to increased energy demands in the next century. Despite concerns about the environmental effects of increasing carbon dioxide levels, the world simply cannot withdraw from its dependence on fossil fuels in less than several decades. Substitution, the development of new technologies, and conservation can reduce this reliance.
The development of solar, tidal, and wind energy requires continued study, as does increased use of geothermal sources. The continued use of nuclear energy is dependent on the efficient and responsible handling of radioactive wastes. Future energy resources may include forms that seem exotic today: hydrogen fuels and nuclear fusion are subjects of ongoing research. Regardless of the mix of eventual sources, society has become more conscious of the problems of integrated development, consumption, and waste disposal—the activities constituting the use of any energy or mineral resource.
Petroleum Resources
Significant accumulations of oil and gas are geological anomalies, and they constitute only a minute fraction of the total material within the sedimentary basins where they are found. These two factors create the scientific and technical complexity of the geoscientist's role in petroleum recovery and of the industry itself. Even in relatively well-understood sedimentary basins, such as the Gulf of Mexico, the success rate for exploration drilling is only about one in six. In the true frontier areas that risk might be increased by a factor of 10 or more. The cost of a single exploration well can be in the tens of millions of dollars. Appropriately, great effort and expense are invested in geoscientific research that increases the probability of successful strikes and effective extraction.
The historical development of petroleum resources has reached a critical point. In the conterminous United States, additional discoveries are sought within the framework of developed resources. Recent increases in reserves have resulted predominantly from enhanced recovery methods in known fields and from in-fill drilling between successful strikes. Some frontier contributions can be expected from the deep-water Gulf of Mexico and from Alaska. Worldwide, however, new and undeveloped resources are sought. The two searches are complementary, and they present a remarkable opportunity for geoscientists. Researchers can apply a full spectrum of approaches and technologies to the search for, and the exploitation of, petroleum deposits. These range from the use of traditional interpretations of oil genesis to the application of innovative quantitative and genetic models of producing reservoirs. Vast quantities of new data from both satellite imagery and seismic surveys offer unmatched opportunities for gaining insights that were unimaginable only a few decades ago. These new methods are being applied all over the world but especially in the Middle East.
According to the most recent estimates, the countries of the Middle East overlie more than one-half of the world's undeveloped petroleum resources. This accumulation came about as part of the cyclical process involving the opening and closing of the ocean basins, which is the consequence of plate tectonics.
About 100-million-years ago the area that is now Arabia was located along the north coast of the supercontinent of Gondwana, forming an Atlantic-type continental margin at a tropical latitude (Figure 4.13). Anoxic episodes, characterized by the absence of free oxygen, encouraged accumulation of organic debris in sediments deposited at water depths of a few hundred meters along the margin of the continent. In shallower water close to sea level, abundant limestone reefs grew; with oscillating sea levels, evaporite salt deposits precipitated among the limestones. Uplift to the west, in what is now Africa, resulted in erosion that episodically carried sand into the offshore area. These related events provided the carbon-rich source rocks in which petroleum would
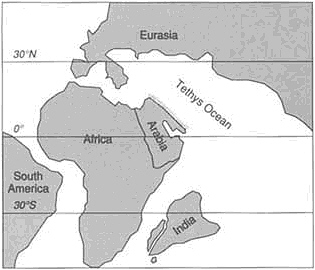
FIGURE 4.13 Organic-rich source rocks formed along the north coast of Gondwana. As the Tethys Ocean closed and with the collision of Arabia and Eurasia to form the Zagros Mountains, the oil migrated and was trapped in structures that now form the huge oil fields of the Middle East.
later form, the porous coarse limestones and sands that would act as future reservoirs, and the evaporites and fine-grained limestones that would trap the petroleum beneath their seal.
Approximately 15-million-years ago, this well-prepared Arabian shore collided with Asia and thrust up the Zagros Mountains. Today, the Zagros range continues to build over the Arabian plate. The now deeply buried oil source rocks are being heated to generate oil that migrates upward into huge retentive structures sealed by the fine-grained limestone and evaporites. Close to the Zagros, these structures are long anticlinal arches parallel to the mountain ranges in Iran, Iraq, Syria, and Turkey. Farther south, these structures are the huge domes underlying the Persian Gulf, Kuwait, Saudi Arabia, and the United Arab Emirates.
The exceptional petroleum resources of the Middle East result from a set of conditions excellent for forming oil and trapping it within structures ideal for its accumulation. Because this process has occurred within the geologically recent past—during the past 15-million-years—little of the oil has had time to escape from the traps, and it has not been degraded to gas by thermal cracking—the breakup of chemical compounds into simpler forms.
The petroleum resources of the Persian Gulf area have been discovered and exploited within the past 80 years. This same time span has witnessed the expansion of society's dependence on fossil fuel, the understanding of plate tectonics as an explanation for genesis and location of resources, and the development of vast data-processing abilities within the geological research community. Progressive understanding of the Persian Gulf oil fields has engendered an invaluable model that provides a basis for future exploration efforts and more efficient extraction techniques.
Origin of Petroleum Within Sedimentary Basins
Significant petroleum resources result from series of events that fall into four categories: genesis of oil and gas from preserved organic debris within a source rock, primary and secondary migration of the generated hydrocarbons over distance, entry of the hydrocarbons into a properly prepared reservoir, and finally entrapment of the hydrocarbons (Figure 4.14). All of these events occur within the confines of a specific basin. During the past century, geoscientists have studied the evolution of such basins closely, producing models such as that of the
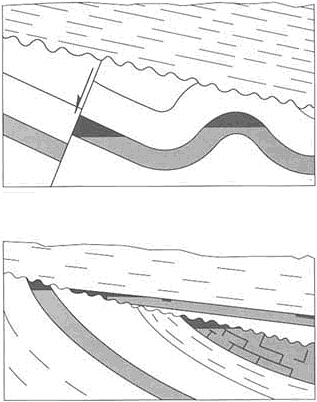
FIGURE 4.14 Idealized section (upper) shows characteristic pre-unconformity traps that are obscured by post-unconformity formations. A diagrammatic section (lower) shows traps commonly associated with surfaces of unconformities.
Persian Gulf. Each of the four developmental categories, while overlapping in time and location within a basin, has been studied separately, and in the final assessment all are combined into an integrated approach for exploration.
Organic material is oxidized at the surface by processes such as bacterial decay. Under some conditions the bacterial decay is incomplete, and burial of the remains segregates carbon-rich material within sedimentary rocks. It is this sequestered organic matter that, upon further burial, is capable of generating fossil fuels. Coal consists of solid plant matter preserved where it was originally buried, while oil and gas are fluids formed from buried organic matter that subsequently migrates from its source area.
Researchers try to establish exactly how distinct fluids are generated and what kinds of oil and gas relate to what source materials. This knowledge can help direct the search for new oil deposits. For example, although 50 years ago it became obvious that lake beds, such as the Green River Formation of Utah, may contain significant quantities of oil and gas source materials, it is only in the past decade that lake beds worldwide have been emphasized in oil and gas exploration.
Sedimentary basins are depressions on the surface where loose particles accumulate and eventually turn into solid rock. These depressions result from the effects of thermal convection on the lithosphere and from the application of loads generated by a variety of processes, including mountain building and rifting. The process of building a mountain belt puts a heavy load at the surface and forms depressions on either side; rifting thins the lithosphere and forms a depression where dense material, usually consigned to a deeper position, is brought closer to the surface. Lighter or buoyant loads are locally associated with rifts in volcanic areas where hot or light rock is abundant near the surface. These buoyant loads, which correspond to elevations on the crust, are distributed sporadically along the lengths of active rift systems like that in East Africa. An environment in which basins are particularly well developed is the rifted Atlantic-type margins of continents where juxtaposition of dense oceanic lithosphere against less dense continent generates an elevation difference of several kilometers. This is the environment in which most active sedimentation on Earth is concentrated, much of it building the world's great deltas.
The thickness of material accumulating in sedimentary basins may become greatly amplified in comparison with the depth of the initial depression. For every unit of sedimentary load deposited at the surface, a corresponding mass of material, with a somewhat higher density, flows away in the underlying asthenosphere. In this way further subsidence is induced; the fill in sedimentary basins may become as thick as 15 km.
Petroleum source rocks are accumulations that are rich in organic matter. Most are shales, but siltstones, limestones, evaporites, algal mats, and even coals can be important. The essential characteristic likely to create petroleum resources is rapid accumulation of organic material—fast enough to exceed the oxidation of carbon due to bacterial decay. Typical environments are primarily aquatic—such high biological activity takes place in lakes, swamps, lagoons, bays, and estuaries. These anoxic, or nonoxidizing, conditions have developed episodically in deep ocean basins—at times and places of unusually high nutrient flux and limited bottom-water circulation. Restricted ocean basins—those just beginning to open (such as the Red Sea), or nearing closure (like the modern Mediterranean), or left as remnants of former oceans (such as the Black Sea)—are strong candidates for anoxia. Through analogy, this recognition guides oil exploration to sites of ancient anoxic conditions. Climate exerts a strong control over the processes of nutrient flux and anoxia, so studying the distribution of organic-rich rocks contributes to an understanding of ancient climatic processes—and vice versa.
Buried organic material changes within sedimentary basins. Temperature and the duration of burial control complex modifications, called maturation, producing a diverse group of organic compounds. The most important compounds, for the purpose of forming fossil fuel resources, are the kerogens. Different kinds of plant matter yield different kerogens that, in turn, are capable of producing different proportions of oil and gas. The kinetics of the maturation process vary with the complex organic compounds of the specific kerogen involved, but generally oil is produced from less mature kerogens than gas. Some kerogens are specifically oil prone and some specifically gas prone from the beginning. According to their maturity, source rocks may be incapable of producing any fluid hydrocarbons at shallow depths, capable of producing oil at intermediate depths, and capable of producing gas at greater depths. At very great depths kerogens become overmature, having released all their mobile hydrocarbons, and eventually become hydrogen-free graphite.
In oil and gas exploration the optical properties of vitrinite, a dark glassy material occurring in most
coals and in some kerogens, provide a valuable index to the level of maturity. Vitrinite reflects more light as it becomes more mature, so the comparison of measurements with standard samples can indicate whether a particular rock is immature, mature, or overmature. There is no point in looking for oil where the rocks are still immature, where no hydrocarbon has been generated, or where all hydrocarbons have been driven off. Both surface outcrop samples and drill-hole cuttings are used in this way to help guide exploration. A variety of other thermal indicators of sediment maturity are also used, ranging from the color of contained microfossils such as conodonts and the annealing of fission tracks to the proportion of radiogenic argon driven from fragmented microcline crystals after deposition.
The formation of oil and gas from buried organic matter is thermally controlled, so in oil exploration it is important to be able to characterize the thermal state of sedimentary basins through time. A useful distinction can be made between basins formed by extension, a process that thins the lithosphere and generally brings hot deep-lying rock nearer to the surface, and those formed by shortening, a process that thickens the lithosphere and initially produces a cooler crust. In the former circumstance, the first sediments deposited in the basin are likely to mature rapidly through the oil-generation phases, but in the latter circumstance an initially cold pile of sediments gradually warms, with oil maturation taking place later in the basin's evolution. Sedimentary basins formed at continental ruptures—rifts where oceans have failed to open and Atlantic-type margins where oceans have successfully formed—are likely to contain petroleum source rocks that attained maturation within a few tens of million of years of deposition. The main producing basins in China are of this type, as are the Gabon and Angola basins of West Africa. For sedimentary basins formed in association with the shortening of continental collision, the maturation process is much slower. In the case of the Anadarko basin in Oklahoma, for example, a 380-million-year-old source rock began to generate abundant oil and gas only when deeply buried 100-million-years later.
The relative integrity of geological records preserved in sedimentary basins makes them ideal natural laboratories for multidisciplinary studies, including depositional processes, earth history as recorded in sediments, biological evolution including extinction, climatic variation in space and time, thermal structure and evolution, and lithospheric for addressing these issues in sedimentary basins are exceptional because it is within those basins that petroleum, coal, uranium, and water resources are largely concentrated. Seismic reflection data are available in explored sedimentary basins, and oil wells and their geophysical logs complement and permit calibration of seismic results.
Basins can be classified in many different ways. One simple way is to recognize that basin development is controlled by the plate structure of the lithosphere. For example, the Arabian oil-bearing basin lies at a convergent plate boundary where Asia and Arabia are colliding, and the Suez basin lies in a rift zone where Africa and Arabia are beginning to separate. Extending this idea to cover plate evolution through time, and recognizing that the dominant result of plate tectonic processes at the surface is the cyclic opening and closing of ocean basins, lead to a simple classification of basins related to that cycle. Thus, sedimentary basins develop by various means: in rifts as continents begin to rupture, at Atlantic-type margins when continents have ruptured, and at convergent margins in association with the island-arc and continental collisions that reduce the areas of ocean basins and lead to their eventual closing.
Several hundred sedimentary basins are recognized worldwide, but most oil and gas are produced from fewer than 50 basins. The double challenge now is to establish which of the remaining basins will become major producers in the next decades and how to produce more from those already in an advanced state of development.
The question of the origin of petroleum leads to the study of organic matter likely to be involved in petroleum formation and to the examination of source rocks in various stages of maturity. Particular oils and gases formed from the maturation of particular source materials are available to the investigator for complementary study near well-documented sites of exploitation. A challenge lies in establishing what happens to the fluids between the place of generation and the place of eventual entrapment—during migration.
Migration of Petroleum Within Sedimentary Basins
Oil and gas migrate from their places of generation through permeable rocks and through fractures. Globules of petroleum move when their buoyancy exceeds the minimum capillary pressure necessary to displace the water already filling the pores and fractures of the rock. The globules are less dense than water and are driven by buoyancy and
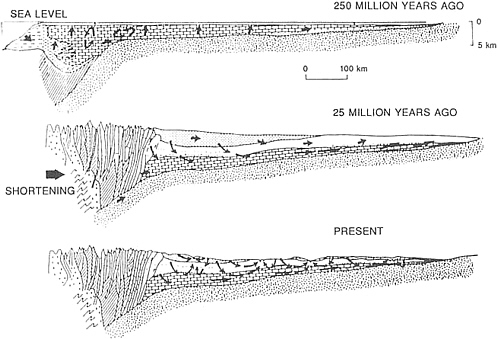
FIGURE 4.15 Three cross sections showing the development of the Alberta Basin; arrows show the sense of fluid flow in these sections.
hydrodynamic forces. Dominant fluid-driving forces are the gravitational head created by topographic relief above sea level and the compactional head generated by subsidence and compression below sea level. Oil and gas stop moving when they reach a capillary pressure equilibrium or when they are trapped by capillary seals that reduce or prevent further migration.
The Alberta Basin, including its southward extension into Montana, provides an example of how fluid flow has evolved as a sedimentary basin developed (Figure 4.15). Some 250-million-years ago the west coast of North America was the site of an Atlantic-type margin. Sediments were deposited and various fluids moved through the deposits, both processes occurring largely in response to sea level changes or down-warping of the coast. From about 200-million-years ago, a complex succession of island arcs colliding from the west built a range of mountains along that margin, and a foreland basin formed in front of the rising mountain belt in response to the loading of the crust by the mountains. Sediments eroded from the mountains, filling the basin, and fluids moved through the basin accordingly. Compressional compaction of deeply buried sediments beneath the shortening mountains further enhanced fluid migration.
During the first phase, thermally driven free convection of waters in contact with seawater dissolved some minerals and deposited others, leading to a variety of changes that together are called diagenesis. Within the Alberta basin these changes included replacing some of the calcium in calcite with magnesium to form dolomite, a process that often reduces rock volume and yields more pore space to form potential reservoirs, and cementing sands to form sandstones, a process that reduces porosity. Sulfide mineral deposits were formed locally in the sediment wedge by fluid flow under this regime. In areas where rapid deposition of impermeable sediments confined water-filled porous materials, overpressures—which eventually are dissipated through faulting or seepage—may have developed. Oil formation in the Atlantic-type margin took place as cold, organic-rich sediments were deeply buried and warmed in the Earth's conductive
gradient. Oil and gas migration would have taken place over short distances.
The construction of a mountain load on the edge of the continent buried fluid-bearing rocks to great depth, and buoyancy carried the fluids long distances—hundreds but not thousands of kilometers—through permeable beds and along thrust faults in the foreland basin to join locally generated oil and gas. Finally, at least in part in response to flexural relaxation after the shortening in the mountain belt had ceased, the mountains were elevated to about their present heights, and the active, regionally extensive, gravitationally driven flow system was established.
During burial in a subsiding sedimentary basin, rocks are measurably altered, largely by inorganic chemical processes. Flow patterns, temperature fields, and patterns of chemical reaction between rock and fluid are all interdependent. The flow of fluids through sedimentary rocks modifies the rock matrix, changing porosity and permeability as some minerals dissolve and others are deposited. Elevated temperatures foster the maturation of organic material, producing organic acids that can dissolve matrix, thus enhancing porosity. And coating of a mineral grain surface with iron oxide minerals may prevent further matrix deposition and thus maintain porosity. The processes and interactions that can happen are very diverse, and the sequence of events may prove critical to the formation and preservation of exploitable oil and gas resources.
Geologists treat all these changes as part of a single process, diagenesis. Diagenesis is important in the exploration for energy resources because there is a need to predictively model the distribution of mature source rocks in a basin and to understand the patterns of change through time that may alter porosity and permeability in reservoir rocks. Just as maturation of source rocks depends on time and temperature, so apparently do the diagenetic changes that reservoir rocks undergo during subsidence and burial. Aided by seismic and sequence stratigraphy, the understanding of diagenesis provides a powerful exploration tool.
Mathematical modeling of fluid flow in sedimentary basins represents an important complement to the study of diagenesis. The tradition of modeling began decades ago in groundwater research, when simple electrical conductivity analogs of water flow were successfully developed. Modeling studies have been extended to the migration of oil and gas only within the past 35 years. Modern computational facilities are being used to simulate transient fluid flow and heat transport with a continuum approach. Current challenges include coping with fractured rock, scaling heterogeneities, and assessing rock physics data such as the quality of available permeability. Scale invariance, recognized as plausible in many properties of sedimentary basins, is leading to successful application of fractal methods.
Two-dimensional models of coupled fluid and heat transport have been used to develop pictures of ore-forming processes, geothermal processes, and regional oil migration. Two-dimensional models of paleohydrology have been published for important basins such as the Alberta, Williston, Illinois, Gulf Coast, North Sea, Suez, and Rhine graben and, on a smaller scale, for the Denver and Palo Duro basins. Geochemical change has been addressed in a few studies, including quartz cementation of sandstone, hydrothermal mineral vein deposition, and uranium ore deposition within sediments.
Fully three-dimensional study of flow in sedimentary basins lies in the future, as access to supercomputers and the establishment of appropriate codes permit the incorporation of compositional and physical variations.
Exciting though the possibility of a full description of fluid flow in a sedimentary basin may be, there is also a need to model the full development of the basin itself as a response to loading of the lithosphere. Basin subsidence, both under mechanical load and in response to cooling—as well as compaction and deformation of the basin fill—and variation in rate of fill in response to sea level change and uplift of neighboring mountains are all being accommodated in partial and two-dimensional sedimentary basin models. The properties of the fully integrated three-dimensional dynamic basin model are beginning to be defined, and realization of such models should help in revolutionizing many aspects of oil exploration.
Traps: Where Oil and Gas Stop
The critical link in the accumulation of hydrocarbons is entrapment. After maturation, expulsion from the source rock, and migration through buoyant fluid flow, hydrocarbons will resort to any available path to the surface and escape—unless they are trapped. Porous material in which hydrocarbons can accumulate, surrounded by an impermeable seal that prevents further updip flow, constitutes a trap.
The simplest of traps is an anticlinal fold, an arch-like flexure of rock layers. The hydrocarbons migrate to the crest of the fold, because they are less dense than formation waters and are trapped by an
impermeable seal—often a layer of shale or salt. Anticlines were recognized to have the capability of trapping fluid hydrocarbon early in the development of the petroleum industry. At first, surface anticlines were sought and drilled, but two developments rapidly expanded the possibilities. Anticlines beneath the surface began to be located by geophysical methods, and other entrapping structures were recognized, particularly structures in which faults contribute to enclosure of the hydrocarbons.
The large majority of discovered hydrocarbon reserves have been found in structural traps. Exploration in frontier basins today continues to be dominated by the drilling of structural prospects, once the potentially favorable source and reservoir stratigraphy have been identified. Despite a long history of experience and success, many unsolved problems of extreme structural complexity remain. For example, significant potential accumulations are associated with thrust belts (such as those found in Wyoming), which may yield giant oil and gas fields. Thrust belts are relatively unexplored, or unsuccessfully explored, because of the great difficulty in acquiring and interpreting the seismic data to form a recognizable subsurface picture. Major industrial and academic research programs in structural geology and seismic technology are aimed at this unsolved problem.
Improvements in seismic data are also important in aiding the discovery of hydrocarbons in formations more subtle than the familiar structural traps, such as the stratigraphic trap. One of the world's largest oil fields lies in a stratigraphic trap, East Texas, discovered in 1930. Stratigraphic traps depend on a wedge-shaped termination to a reservoir unit at a seal. The revolution in seismic stratigraphy over the past decade has promoted the location of stratigraphic trap geometry. Stratigraphic relationships can now be interpreted directly on a seismic reflection record, and inferences relative to the position of reservoir-prone and seal-prone lithologies can be made. The power of this approach in defining the subtle stratigraphic trap remains very promising, and some substantial new discoveries have been made.
Combined structural and stratigraphic traps provide very attractive exploration targets. Representative of such a trap would be a submarine fan reservoir sand along the flank of an anticline, down-dip from the crest of the structure. In years past the submarine fan would not have been recognized, and prevailing exploration practice would not consider drilling a well anywhere but on the crest of the anticline. We now have the technical ability to search for potential stratigraphic traps defined by position relative to structure. This should greatly broaden production possibilities from structures that were formerly explored only in the conventional way, many of which are now productive despite the absence of reservoirs at the crest of the structure.
The timing of the formation of the trapping configuration relative to the timing of generation and migration of the hydrocarbons is critical. Traps are of no value unless they exist prior to the migration of hydrocarbons. The integration of maturation modeling, which concerns predicting the timing of hydrocarbon generation, with the delineation of drillable traps is vital to a sound and successful exploration program. It is also a further instance of the importance of the integrated basin analysis approach to petroleum exploration.
Oil Shales and Tar Sands
The hydrocarbons used to fuel the industry and transportation networks of twentieth-century societies have migrated from source rocks and been trapped beneath the surface before they could be altered into unproductive compounds. Scientists have identified, and are attempting to utilize, those hydrocarbons still sequestered within the source rocks as well as those that have changed too much to be exploited by known methods. Examples of these are oil shales that are exposed source rocks and tar sands that are fossil compounds altered by exposure to near-surface agents.
Some shales contain exceptionally large amounts of kerogenic organic matter, and for more than a century small quantities of oil have been distilled from oil shales in various parts of the world. In the United States, interest has focused on the 50-million-year-old oil shales in the Green River lacustrine deposits of Colorado, Utah, and Wyoming that contain an estimated 2 trillion barrels of oil in the richest beds. The oil is in the form of solid kerogen, however; without the slow gentle thermal maturation necessary to fluidize the kerogen, the desirable components remain locked in a mixture of sandstones and clays and in layers of precipitated carbonates and evaporites. Production of oil from oil shale is not economical at present, but interest for the future persists because the quantities of oil locked in these rocks represent many times the world's reserves of conventional petroleum. Any recovery method would require the application of high-temperatures and large quantities of water,
however, and tremendous amounts of spent shales would accumulate on the landscape. One calculation suggests that recovering all the oil from just U.S. sources would leave enough spent shale to cover the entire globe to a depth of about 3 m.
If fluid hydrocarbons escape entrapment, they migrate toward the surface. Once the compound approaches the level at which it interacts with the atmosphere, groundwater, or soil biota, it may accumulate in sedimentary deposits and form tar sands—thick concentrations of viscous degraded crude oil that has saturated detritus until it became an asphalt. Traditionally researchers assumed that the oil's fluid nature was altered through oxidation, with lighter fractions escaping on contact with the atmosphere. Recent studies, however, suggest a bacterial influence acting at depth that removes particular components from the migrating fluid. While research into biological agents that transform hydrocarbons may not solve the problems inherent in exploiting tar sand deposits, it may lead to solutions for cleaning up oil spills and other environmental dangers associated with the use of petroleum products.
Exploration for Oil and Gas: The Integrated Approach
The earliest oil and gas discoveries were accidental. Natural gas wells several hundred meters deep were drilled in Sichuan, China, nearly 1,000 years ago, following gas discoveries during salt production. The oldest wells in North America were drilled near oil seeps. Technical understanding has subsequently played a progressively larger role in exploration, ranging from recognition of anticlinal traps at the turn of the century through increasing application of geophysical methods to the sophisticated array of instruments now used in the sondes lowered into drill holes to log wells. The use of micropaleontological, geochemical, structural, stratigraphic, sedimentary, and diagenetic understanding over the past 80 years illustrates the growing role of diverse branches of geology in petroleum exploration. Geophysics has grown even more rapidly. Gravity and magnetic measurements continue to be useful, but the field is dominated by seismic techniques closely linked to advanced computational capabilities. These high-technology applications have become universal and critical in modern oil and gas exploration.
Much current research is directed at the analysis of unconformity-bounded depositional sequences (see Figure 4.14). Work of this kind, termed sequence stratigraphy, establishes a hierarchy of such unconformity-defined units to be identified in many basins. Understanding the way in which sedimentary rocks are layered has proven extremely valuable in exploration for petroleum and coal resources. Mounded shapes are diagnostic of submarine fan deposits, and nested sigmoidal packages often indicate advancing delta complexes at the mouths of ancient river systems. Both of these environments may contain sandstones that have high potential as reservoir rocks for the entrapment of oil and gas.
Unconformity-bounded depositional sequences at all scales are associated with cycles in which the position of the ancient shoreline is observed—within the sedimentary record—to move alternately in a landward and then a seaward direction. Some scientists have related these oscillatory variations to global sea level change, but others suggest that vertical motions of the crust, variations in sediment supply, and climatic fluctuations are just as important. The importance of sequence stratigraphy to this controversy is that the formation of sequence boundaries requires changes of relative sea level, not merely of sediment input. By comparing the ages of sequence boundaries in numerous basins in different parts of the world, it should be possible to distinguish sequence boundaries of eustatic, or global sea level, origin from boundaries of only local significance. One of the major research opportunities afforded by the emerging field of sequence stratigraphy is, therefore, the attempt to define the history of sea level change.
The importance of this new technique to all petroleum exploration efforts cannot be overemphasized. It provides geoscientists not only with a tool for interpreting the depositional systems within a basin, including potential reservoir and source intervals, but also with a means of making temporal correlations where no other control exists. And where independent calibration does exist, precise and internally consistent correlations can be more firmly established.
The need to characterize the basin environment as fully as possible in oil exploration together with the wealth of information in seismic and well records, has propelled oil company geologists and geophysicists to the forefront in developing a new approach to understanding sedimentary basin fill. That approach is termed seismic stratigraphy.
Seismic stratigraphy is a way of interpreting the sedimentary fill of a basin. The fundamental concept is to project into the Earth a known controllable source of seismic energy at a precisely known
location and then to record the scattered seismic waves returning to the surface. As the seismic waves penetrate the crust, a small fraction of their energy is scattered back to the surface as they encounter differences in acoustic properties related to changes in rock formations. In principle, these returned signals, which contain detailed information about the subsurface geology, can be processed by computer to produce an acoustic image—a seismic reflection profile—of the subsurface down to depths of several kilometers. The profile is not a true geological cross section, but the gross geometry of stratal layers can be determined from it because these surfaces bound rocks with sharply contrasting acoustic properties.
Sedimentary rocks are composed of wedge-shaped, almost tabular, bodies bounded by discontinuities or unconformities that may extend regionally. In many cases an unconformity is associated with the pinching out of overlying and underlying layers. Stratal or layer discontinuities are subtle and difficult to observe, even in outcrop exposures, but often can be identified from good-quality seismic data. By piecing together information from a grid of intersecting seismic profiles and incorporating data from wells and boreholes, stratigraphers can often interpret the three-dimensional layered geometry in the sedimentary basins. Recognition of regional unconformities is especially significant because rocks above an unconformity are everywhere younger than rocks below the unconformity. The geometric shapes recognized in seismic reflection data can, therefore, be interpreted as depositional units, commonly referred to as depositional sequences (see Figure 4.14).
Reflection seismic data represent the single largest class of scientific data collected in digital form. More than 1 million data tapes are recorded annually worldwide, and the processing and analysis of this immense volume are a major commercial driving force behind the development of the advanced scientific computer industry in the United States. Even with the recent downturn in the petroleum industry, reflection seismology is still the single largest scientific and technical activity of seismologists worldwide.
In modern seismic data acquisition, the signal emanating from the energy source, or shot point, is digitally recorded at hundreds of locations on the surface. Because the scattered signals are very weak, the shot point is moved, and the shot is repeated hundreds, even thousands, of times, resulting in millions of data points being recorded per linear kilometer. Conventional seismic data are thus collected in long linear segments called seismic lines, which give a two-dimensional cross-sectional picture of the sedimentary section. To approximate the three-dimensional Earth, a network or grid of lines is recorded. A single regional marine survey, for example, might include 5,000 km of such data—an enormous volume of data by any standard.
Probably the most significant technical advance in petroleum earth sciences in the past decade has been the extension of reflection seismology from two dimensions to complete three-dimensional volumes. The processing of these data produces subsurface images of unprecedented geological detail. In every case where this technique has been applied, substantial revisions have been made to the geological interpretation—with subsequent economic effects. The volume of data recorded for three-dimensional surveys, however, is much larger than that for conventional two-dimensional grids covering the same area. State-of-the-art surveys now collect more than 1 billion data points per square kilometer. Processing these data stresses the largest computer systems available and often takes up to 1 year for large surveys.
Despite the cost and volume associated with three-dimensional seismology, even larger surveys are being recorded, with some exceeding 1,000 km2 each. It is predicted that by the mid-1990s the Gulf of Mexico will have been covered completely with three-dimensional surveys. This data set, once assembled, will provide an unparalleled view of an entire major producing basin and could revolutionize detailed understanding of the complete petroleum system.
Well logging is the term for acquiring complex measurements in a drilled borehole. Vast arrays of sophisticated devices are lowered into the well, in effect creating a remote-controlled downhole laboratory. Data are continuously collected on the electrical, acoustical, and nuclear properties of the rock formations that have been penetrated by the bit.
These data are processed and analyzed to yield a very detailed description of the type of rock and its porosity, and the presence or absence of oil or gas. This complex analysis, termed formation evaluation, is the primary means by which geologists determine whether a well will be a dry hole or a producer. All exploration wells are extensively examined using these critical data as well as selected samples or cores.
Well-log measurements and reflection seismic data comprise the essential subsurface measurements collected for modern hydrocarbon exploration and development. Integrated into the modern
geological framework of basin analysis, they form the observational backbone for predicting detailed sedimentary structure and stratigraphy.
More Oil from Producing Fields
Oil and gas exploration, development, and production proceeded rapidly in the United States by comparison with the rest of the world. Consequently, the oil fields of the conterminous states are now more depleted than their counterparts elsewhere. Most U.S. oil reserves are in discovered fields and are considered to be nonrecoverable resources. This presents a unique opportunity and challenge for improved technology, because recovery from most hydrocarbon fields in the world today ranges from only 15 to 55 percent. An immediate need to develop a better understanding of reservoir heterogeneity, in situ rock and fluid properties, and enhanced oil recovery techniques is apparent.
The most significant trend in the U.S. oil industry has been the shift in effort toward recovery of a maximum amount from existing fields and established areas. In the major oil companies a majority of earth scientists are now engaged in production-or exploitation-related activities instead of the more traditional exploration-related areas. New giant fields—those with recoverable reserves greater than 100 million barrels—in the United States are likely to be found only in remote areas and have associated greater costs. However, there are numerous old giant fields that have yielded only a fraction of their original oil. Hence, billions of barrels of domestic reserves could be added by the use of enhanced recovery techniques. Horizontal drilling has increased reserves in some areas. Complemented by discoveries from more detailed exploration within existing producing regions, the motivation for the shift of effort to established areas is clear.
New types of well-logging tools and interpretational techniques are developing constantly. These developments now allow the location of previously unrecognized oil and gas zones in both actively producing and abandoned wells. The potential for increasing reserves is enormous. The current focus of the domestic oil industry is commonly referred to as reservoir management, and most major companies have actually restructured their organizations in this direction—establishing interdisciplinary teams that include geologists, geophysicists, and reservoir engineers. The teams are charged with characterizing the established fields and reservoirs and then using the information to manage further development drilling and the application of enhanced recovery techniques. In addition, such teams explore for untapped reserves that become economically viable because of their proximity to existing producing facilities.
The most significant technological development in the past decade supporting reservoir management has been the advance of three-dimensional seismology. The large investment for three-dimensional seismic acquisition and processing is often easily justified on economic grounds because of its leverage against the much larger costs of development and field production. The technical base for three-dimensional seismology should continue to grow, along with the capability to record ever larger surveys and produce more detailed images with ever larger computers.
Entirely new seismic technologies are also now in the research and development phase. The most promising is the area of cross-well seismology, in which the active seismic experiment is carried on between wells instead of on the surface. Producing an image from these data is conceptually similar to medical CAT scan technology. The key advantages of the technique are that much higher seismic frequencies can be propagated between wells than from the surface, and measurements can be made within an existing and operating oil field. Moreover, time-lapse repeat measurements can be made with great precision and have been tested for monitoring the advance of hot steam fronts in enhanced production projects that attempt to flush extra hydrocarbons from an otherwise depleted field. Although still in the research phase, an entirely new industrial application of seismology is developing.
Characterization of complex reservoirs for the development of geological and fluid behavior models could lead to optimal hydrocarbon recovery from existing reservoirs. The problems are not different from those generally addressed in modern oil field research and embrace such general considerations as the flow of fluids through porous media. General practice has been to expect such research to be conducted by the 10 or so major oil companies. However, major companies are focusing increasingly on larger targets, and the needed research is lagging.
Coal: An Abundant Fuel Resource
The United States is rich in coal and its reserves are sufficient to support consumption at the present rate for centuries. Most U.S. coal is used in the generation of electricity; it accounts for more than
one-half of all electricity produced. The future of the industry depends not only on traditional market variables but also on coping with the environmental effects of mining and burning coal. Public perception about coal and technological advances in efficient utilization are likely to have major influences on the future of the industry.
Geoscientists contribute to the progress of the coal industry by addressing the origins of coal beds and the evolution of their genetic environments. For example, researchers have observed that although sulfur content varies greatly within regions of the country, there is a tendency for the younger coals in the western United States to be lower in sulfur than are the older coals in the Midwest and the Appalachians. The higher-sulfur coals are often associated with marine strata, suggesting that the sulfur originated as sulfate in seawater. The sulfate was reduced to sulfide by bacteria and then incorporated into the peat that eventually developed into coal. Coals that formed in river systems far removed from marine conditions tend to be lower in sulfur content.
Although researchers understand the origin of some sulfur-rich coal, they have not yet worked out how best to cope with the problems it presents. Much of the sulfur is contained as organic sulfur in very fine-grained iron sulfides, and to separate the coal from the sulfur-bearing minerals would require grinding it into very small particles. Such a procedure is not economical at present. Coal research is dominated by the quest to identify the coal-forming environment and trace its subsequent history. Characterizing the coal physically and chemically on scales ranging down to the submicroscopic is essential for interpretation of its origin and history as well as determination of its technological properties. Such characterization is vital to determine whether coal can be economically mined, cleaned, and used either in conventional combustion or in some other way that may prove economical.
Origin and Development of Coal
Coal is composed largely of nonmarine plant material deposited in swamps. Understanding the depositional environments leading to peat formation and the subsequent diagenetic processes that determine coal type is important in predicting coal characteristics in advance of exploitation. The properties of coal are determined, in part, by the kinds of plants or parts of plants that dominated the original peat accumulation. As with petroleum source rocks, the study of coal distribution has been an important part of paleoclimatological research.
The salinity, pH, sulfate, and bacterial content of water in which peat accumulates largely determine the chemical characteristics of the resulting coal, including its sulfur and nitrogen content. Some of the mineral matter, the ash—which includes part of the sulfur—is derived from plants, but most consists of stream- or wind-transported material, and a large amount of it may enter the deposit long after the swamp has disappeared. Peat deposits in broad coastal freshwater swamps may result in thick coal beds of considerable areal extent. Swamps or marshes in an area of active delta deposition are episodically inundated by flood waters that deposit clay, silt, or sand on previously accumulated peat. The resulting coals contain splits—thin coal seams separated from the main seam by a layer of different sediments—that may reduce their minability. Abandoned stream channels sometimes accumulate thick peat deposits of elongate geometry.
Plant material is further altered chemically and physically by heat and pressure. Coals are ranked progressively upward, from lignite to anthracite, according to the degree of such metamorphism. Rank increases as the fixed carbon content increases and the percentage of volatile matter and moisture decreases.
Considerable research on the origin of coal involves study of the depositional environments of modern peat formation and is aimed at identifying physical and chemical changes during the coal-forming process. Discoveries of peatlands with incipient coal formation in Indonesia were detected through extensive mapping and coring. Studies are developing more sophisticated models for future investigations. Detailed outcrop and subsurface studies of individual coal basins are similar to the basin analysis methods used in the petroleum industry; they aim at defining depositional environments and subsequent coalification processes for model building.
Exploration for Coal
Coal exploration does not have the search component inherent in mineral and petroleum exploration because the limits of most coal-bearing beds in U.S. fields have long been known. Nonetheless, enhanced time-stratigraphic depositional models aid in predicting the location, geometry, and quality of coals. Despite these advantages, the inherent heterogeneity of the material makes sampling of coal deposits a difficult task; thus, development of accurate sampling techniques and analysis of statistics are important in the mining of coal. Where coal beds are nearly horizontal and laterally continuous
over large areas, conventional methods can be used to ascertain the thickness and character of beds. Samples, cores, and geophysical logs are analyzed to determine basic characteristics. Where coal beds have complex geometries, other evaluation techniques may be necessary, such as seismic mapping, closely spaced drilling, and exploratory pits. During exploration, the environmental data required to obtain permits for mining are established, including the trace element content of the coal and surrounding formations, groundwater parameters, mineralogy, flora and fauna content, and soil properties.
More than one-half of the coal mined in the United States is produced from surface mines. Contour, area, or open-pit mining methods are used where coal seams of sufficient thickness and quality are near the surface, which is generally within 50 to 70 m. Surface mining of steeply inclined seams requires special planning that is specific to the conditions at the site. Where coal seams are deeper, shafts must be constructed to reach the coal and allow room-and-pillar or longwall mining methods. The latter method usually results in eventual subsidence of the land surface. An important research problem involves the correlation of geological parameters with the extraction method to allow optimal coal recovery while still mitigating the effects of subsidence on the hydrology and productivity of overlying soils.
Coal can be converted to liquids and gases by a number of different chemical, thermal, combination, or other treatment processes. Liquefaction produces feedstocks for chemicals such as benzene, ammonia, methanol, and acetic acid, as well as sulfur-free petroleum. Some of these processes are available commercially, while others are in various stages of laboratory or pilot plant testing.
Some coal beds contain large quantities of methane gas, which can be produced directly. There is current research into using bioengineered microbes and other methods to produce methane gas from the coal. This form of energy exploitation may be applicable to coal beds that have exceptionally large quantities of contained gas or that are too deep or too thin to be mined economically by conventional methods.
Limitations of Coal
Direct combustion of coal to produce electricity or steam heat requires further research toward the development of higher efficiency standards and cleanup techniques for hot and cold gas combustion and conversion facilities. Research in clean coal technology involves scientists and engineers specializing in combustion chemistry and mechanical engineering, but geologists have a critical role in characterizing what coals will burn most efficiently. Advanced combustion technologies to minimize sulfur dioxide and nitrogen oxides, as well as particulate emissions, have been under active development. These technologies include fluidized-bed combustion, staged slagging combustors, and limestone injection multistage burners. Combustion technology research must be pursued to gain a better understanding of capture mechanisms and to obtain reaction rates. Geoscientists continue studies to select sorbents with optimum reactivity, such as lime, limestone, dolomite, and other natural and man-made carbonates. In addition, desulfurized fuels produced by coal conversion processes require combustion testing to develop acceptable burning properties.
In the past 10 years considerable advances have been achieved in hot gas desulfuration using mixed metal oxides, which absorb sulfur and can be regenerated in a multicycle operation. Efforts should continue to develop reliable and cost-effective processes for cleanup of gas-borne constituents such as chlorine, sulfur, nitrogen, alkali compounds, and fine particulates, especially from fluidized-bed combustors, high-pressure gasifiers, and other technologies that promise higher efficiencies in electricity generation.
Complementary studies are needed for development of an integrated approach to solving problems of coal use such as the control of disposal and the use of waste materials from coal cleaning, fluidized-bed, and other advanced combustion processes. Problems in user equipment that are attributed to the corrosive effects of noxious elements such as chlorine need to be minimized; methods for fine grinding and dewatering of coal need to be improved; and methods for particle-size enlargement or pelletization of fine coal need to be refined.
Preparation of coal for the consumer market includes removal of contaminants such as mineral matter, sulfur, or other undesirable elements without substantially changing the general organic structure of the coal. Physical cleaning, such as washing, gravity separation, or flotation, remains the most economical and widely used technique. Geoscientists study the form, size, and distribution of the mineral matter contained in coals to guide the efforts of mineral preparation engineers in evaluating the efficiency of different processes, including oil agglomeration and column flotation, and of different reagents. Research continues on innovative
wet and dry methods, such as magnetic or electrostatic removal, to maximize removal of iron sulfides. Considerably more research is needed to improve the removal of organic sulfur. Chemical, thermal, and microbial cleaning that breaks carbon-to-sulfur bonds and that can be combined in a two-step process with physical cleaning is being sought. Other approaches being investigated include genetic engineering to design efficient, organic, sulfur-consuming microbes and the use of microbes to alter the surface of iron sulfides to allow for its removal by physical cleaning processes, such as flotation.
Termination of the government-supported Synthetic Fuels Corporation in the early 1980s and recognition of the high cost associated with upgrading of single fuels from coal has led to the research concept of the coal refinery. In a coal refinery a combination of solid and multiphase products and fuels would be produced from coal by chemical, thermal, or other treatments. To date, research into production of a clean solid fuel, with simultaneous coproduction of gases and/or liquids, has not produced an economically viable and technically acceptable product for combustion in conventional utility equipment. Issues such as agglomeration of some coals during heating also must be addressed. Future coal refinery efforts may well depend on a better understanding of organic composition and the role of mineral matter; these will help identify the most selective and mildest forms of treatment necessary to produce an appropriate mix of marketable solid and multiphase products.
Every stage of coal development, from exploration to consumption, affects the environment. For example, acid mine waters can be neutralized, but predicting the movement of near-surface groundwater in surface or underground mining is more difficult. The effects of such elements from fluidized-bed combustion wastes on groundwater quality is not known. Other research should focus on the technical viability of disposing of scrubber sludge wastes in abandoned or existing underground mines, considering the potential effects on groundwater and surface subsidence.
Sources of Energy from the Internal Engine
All of the previously discussed energy sources are driven primarily by the external engine influencing earth systems. But the Earth's internal engine also produces vast amounts of energy that invite exploitation. Early humans were well aware of the heat coming from below the surface—volcanoes were the chimneys ventilating the forge of the god Vulcan, and thermal springs and geysers were considered sure signs of a sacred presence. Many societies attribute healing powers to naturally heated springs; popular spas have grown around such locations as the retreat established by the ancient Romans at Bath, England, and the early-twentieth-century hot-spot, Saratoga Springs, New York. Attempts to use the warm waters for heating homes and workplaces have traditionally been small in scale. Since the discovery of radioactive energy resulting from the decay of radioactive elements within the Earth, scientists have hoped to use internal heat generated by this process and offer a new, cheap, unlimited energy source to society. Research continues to perfect, and expand, both methods of harnessing energy from within the Earth.
Nuclear Energy
A theoretically promising method of harnessing the internal heat generated by the natural radioactivity of the Earth is to mine uranium minerals, extract uranium from such concentrations, and assemble a critical mass in a reactor. Heat generated by the fission process (the spontaneous or induced splitting of atomic nuclei with consequent release of energy) can, when controlled within a reactor, be used to generate electricity. This method of generation is widely used in the world today. In France nearly three-quarters of all electricity is nuclear generated. In the United States the proportion is much smaller and is currently declining. Uranium-powered reactors remain important to the United States, and a few other countries, for powering nuclear submarines and other vessels.
One reason for its limited use in the United States is that nuclear power may not be economically competitive. This lack of competitiveness stems from a variety of causes, some of which are relevant to earth science research. Uranium ores were mined on a substantial scale in the United States for about 30 years but are not marketable today due to the availability of higher-grade, lower-cost ores from abroad. Worldwide uranium exploration over the past 50 years has profited from government interest in access to fissionable material and from the fact that the natural radioactivity of the element makes concentrations unusually easy to detect. Uranium occurs in both oxidized and reduced states and is highly mobile in aqueous solutions in its oxidized state. Most uranium mined in the United States has come from ancient stream channels on the Colorado Plateau, in Wyoming, and on the Texas coastal
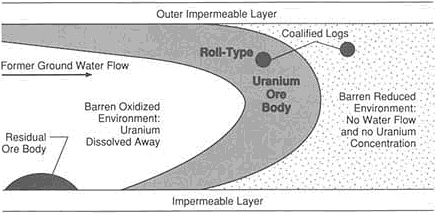
FIGURE 4.16 Schematic cross section of uranium-ore roll and surrounding rocks in Wyoming. Because uranium-ore rolls are of variable width, no scale is given.
plain. In these areas deposition of uranium minerals was localized where oxidized groundwater, carrying uranium in solution, came in contact with organic reducing materials, such as plant matter in the form of logs or leaves. Anaerobic sulfate-reducing bacteria may have been important in precipitating uranium minerals at the reduced surface (Figure 4.16). The transport and deposition of uranium present yet another example of the importance of fluid flow through porous media in geologically significant environments. Research related to uranium deposits is, in many ways, similar to the basin analysis that dominates research in the petroleum industry.
The nuclear power industry faces a continual challenge in seeking options to dispose of waste materials. This costly need is another reason for the decline in the use of uranium as a means of electric power generation in this country. Geoscientists are active in research related to this problem, since the currently preferred disposal option is to deposit radioactive waste materials beneath the surface. The possible interaction of fluids with buried radioactive waste and the subsequent migration of those fluids through porous rocks to the biosphere must be projected over the long intervals during which hazardous material can remain radioactive. Related research opportunities occur where radioactive wastes have already been emplaced. Both of these areas of research involve fluid flow and chemical interaction in porous media. While some policy makers strive for complete isolation of nuclear wastes over very long durations, some researchers feel that such materials must be regarded as byproducts that will be used as resources in the future. This opinion demands accessibility to radioactive wastes; many environmentalists agree that accessibility is desirable but for the purpose of monitoring—not with an eye toward exploitation.
Nuclear power plants add no carbon dioxide to the atmosphere, in contrast to all fossil fuel plants. As our understanding of the possible effects of carbon dioxide emissions emerges over the next decade, it is possible that nuclear power plants may become more acceptable. There is a need to be ready for this eventuality, and additional research on uranium mineralization and waste isolation should be undertaken now.
Geothermal Energy
Another method of harnessing the energy generated within the Earth by radioactive decay is the direct extraction of heat from hot rocks that remain buried (Figure 4.17). Energy generated from this source is called geothermal energy, and research on its extraction is an active field in the geosciences. Most of the geothermal energy used in the world today comes from volcanic areas and areas of active extensional faulting where hot, or partly molten, rock approaches the surface closely enough to be accessible by drilling. Natural steam or hot water, confined by an impermeable seal and heated by the magma or hot rock, is extracted from holes drilled into the geothermal system and is converted at the surface to electricity.
The combination of crustal features required for a geothermal energy supply occurs rarely: hot rock near the surface with a reservoir of confined water that is large, permeable, shallow, adequately recharged for a life of decades, and low in dissolved solids. Proximity to an energy market is also an advantage. Geothermal energy has been used successfully for generating electricity in California, Mexico, Japan, Italy, New Zealand, Iceland, and Tibet, but large-scale economic geothermal production is not widespread. Problems arise because of depletion of reservoirs and undesirable environ-
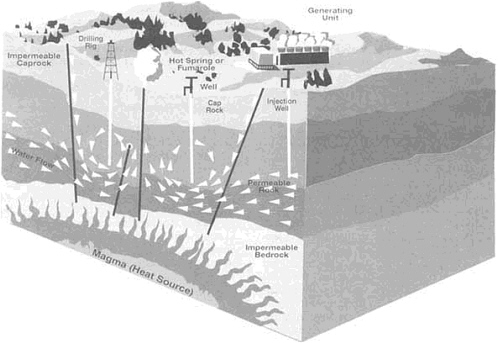
FIGURE 4.17 Development of an active geothermal field.
mental effects. An exciting challenge for the geoscientist is to locate exploitable geothermal resources lacking obvious surface manifestations. Remote sensing (especially by infrared techniques that reveal heat), geochemical, and geophysical methods are being developed and tested for this purpose.
Other uses of geothermal energy have been developed. Hot waters are used for space heating, agriculture, and mariculture in Iceland. Apartments in the suburbs of Paris and elsewhere in Europe are warmed by groundwaters. Boron from geothermal waters has been marketed in Italy. Possible developments in the future extend to extracting heat from hot dry rocks by injecting cold water and recovering heated water or steam.
RESOURCE DEPENDENCY
Geothermal energy use is an obvious example of how developing better comprehension of rock-fluid interactions can lead to efficient resource exploitation. Throughout this chapter more subtle interactions have been discussed. The concentration of traditional minerals, the accumulation of fossil fuels, and the deposition of uranium minerals all result from rocks and fluids interacting chemically, thermally, and mechanically. As scientists study this crucial and fundamental field, the problems associated with each of these resources will be solved one by one, and society will become better equipped to live on the Earth's surface without permanently harming it.
The need for resources has always been a major stimulus for scientific advance, providing both economic reward and intellectual challenge. The discoveries made and the problems presented by the resource industry have kept scientists busy explaining the hows and whys. Once an explanation becomes established, further discoveries and data accumulation by industry either ratify or reject prevailing opinions about how crust has evolved from the matter and energy of the core and mantle. An explanation that holds provides a model from which the resource industry can speculate confidently on the locations and forms of future discoveries.
Historically, advances in knowledge gained through collaborations between scientific theory and social necessity have led to greater understanding of natural systems. More recently, scientists have considered the reciprocal relationships between natural systems and human activity within
those systems. Consideration of these relationships may result in predictions of natural disaster potential and in responsible planning that can avoid suffering and loss of life. But scientific consideration of the relationship between natural systems and human endeavors can also provide assessments of those activities that threaten the environment—disasters caused by humankind.
RESEARCH OPPORTUNITIES
The Research Framework (Table 4.1) summarizes the research opportunities identified in this chapter and in other disciplinary reports and references. These topics, representing significant selection and thus prioritization from a large array of research projects, are described briefly in the following section.
Resource recovery is restricted to the top 10 km of the crust, and most of it is from much shallower depths. Understanding of the structure of the shallow crust and of the processes operating to form and modify that crust, especially those involving the flow of water through rock, provides a certain unity to resource research. It is convenient to define three arbitrary depth zones (with gradational boundaries) from the surface downward. The most widespread and generally used resources are land and water; the most dominantly used water is at or near the surface (0 to 10 m). At intermediate depths (10 to 100 m) groundwater development and surface mining are major activities, and remote sensing of properties becomes increasingly important. The shallow crust, at depths of 100 m to 10 km is the domain of deep mining, deep groundwater, and the petroleum industry. It is here that remote (geophysical) sensing from the surface by a wide range of techniques and advanced logging of boreholes become critical in understanding the crust.
Making the best use of mineral and energy resources calls for understanding the earth systems of which they form a part and for appreciating the need for waste isolation and the environmental consequences of mineral development. For these reasons, the research opportunities identified for earth systems outlined at the ends of Chapters 2 and 3, and those related to environmental considerations at the end of Chapter 5, are relevant to much research on depletable resources. The focus for this chapter is deliberately restricted to topics identifiable as important under Objective B: ''Sustain Sufficient Resources." Many research topics relate to more than one Research Theme. The comprehensive topic of "Sedimentary Basin Analysis," for example, involves all the research themes. Similarly, the subject of "Water-Rock Interaction" relates to at least themes I-IV and has particular importance in basin analysis and in modeling of ore formation.
Sedimentary Basin Analysis
The material carried out of drainage basins is deposited in the sedimentary basins of the world. It is from these sedimentary basins—both active basins currently being filled and ancient basins that were filled long ago—that the world's oil, gas, coal, and groundwater, together with many of its mineral deposits, including its uranium deposits, are produced. Research in resources should continue to stress a multidisciplinary basin analysis approach, emphasizing detailed studies of specific depositional environments in outcrops and cores in conjunction with physical measurements of rock properties.
Organic Geochemistry and the Origin of Petroleum
The processes of maturation of organic material and diagenesis merit continued study by observation, experiment, and modeling. Such phenomena as natural cracking and migration require emphasis. The whole forms an important activity in relation to the broad field of sedimentary basin analysis.
Kinetics of Water-Rock Interaction
Understanding the kinetics of water-rock interaction is a research frontier critical to sustaining both water and mineral resources. Mineral surface catalysis, poisoning effects, and complex mineralogy make this understanding hard to achieve in systems at temperatures from 100° to 500°C; at less than 100°C, organisms, organic material, and materials added to groundwater by humans play corresponding roles.
Water Resources
Analysis of Drainage Basins
Surface waters are best understood in terms of drainage basins that allow such processes as erosion, deposition, and water and sediment transport to be addressed in an appropriately integrated way. An immediate resource challenge is presented by the
TABLE 4.1 Research Opportunities
|
|
Objectives |
|
|
Research Areas |
A |
B. Sustain Sufficient Resources Water, Minerals, Fuels |
C |
D |
I. Global Paleoenvironments and Biological Evolution |
|
■ Evolution of mineral deposits through time ■ Coal petrology and quality |
|
|
II. Global Geochemical and Biogeochemical Cycles |
|
■ Most of the opportunities listed under III below relate to integral parts of biogeochemical cycles ■ Organic chemistry and the origin of petroleum |
|
|
III. Fluids in and on the Earth |
|
■ Kinetics of water-rock interaction ■ Analysis of drainage basins ■ Water quality and groundwater contamination ■ Modeling water flow and hydrocarbon migration ■ Source-transport-accumulation models ■ Numerical modeling of depositional environments ■ In situ mineral resource extraction ■ Crustal fluids |
|
|
IV. Crustal Dynamics: Ocean and Continent |
|
■ Sedimentary basin analysis ■ Surface and soil isotopic ages ■ Prediction of mineral resource occurrences ■ Concealed ore bodies ■ Intermediate scale search for ore bodies ■ Exploration for new petroleum reserves ■ Advanced production and enhanced recovery methods ■ Coal availability and accessibility ■ Concealed geothermal fields |
|
|
V. Core and Mantle Dynamics |
|
|
|
|
|
|
Facilities - Equipment - Data Bases |
|
|
|
|
■ Deep seismic studies, three-dimensional data, complementary advanced well logging ■ Advanced supercomputing facilities ■ Continental scientific drilling ■ Oceal drilling ■ Space-borne measurements, LANDSAT, SPOT, HIRIS, magnetometer, gravity survey ■ Geophysical techniques, side-looking radar from aircraft ■ Laboratory equipment with high spatial resolution and high-precision analytical capability ■ Geographic information systems ■ Data bases on topography, geology, geochemistry, and geophysics |
drainage basins of the western United States. Policy decisions on the allocation of surface waters are driven by jurisdictional considerations. Recognition of the drainage basin as the fundamental scientific unit, provides a basis for effective communication with decision makers in providing information useful for the allocation of scarce surface waters.
Surface and Soil Isotopic Ages
The development and application of new techniques will allow ages to be assigned to surfaces and shallow rock materials, which should improve understanding of both the processes and the rates of landscape modification. Improvement in the ability
to quantify these ages in years will revolutionize understanding, which is becoming more urgent as appreciation of the possibility of anthropogenically modified climatic change spreads.
Water Quality and Groundwater Contamination
To reach a realistic baseline on water quality nationally, chemical and physical measurements must be made at a vast network of stations. To assess change, frequent repeat measurements will be needed. Part of the national water quality problem relates to the relatively small volumes of rock where toxic and radioactive waste materials have entered the groundwater. Challenges in this frontier area range from asking whether there may be some places where the wisest course may be to do nothing to assessing possible roles for novel techniques, such as remediating organic wastes using microorganisms, including special genetically engineered microbes.
Modeling Water Flow
Thermal, chemical, and fluid transport models for water need to be integrated into four-dimensional models—that is, models that involve variation with time.
Mineral Resources
Source-Transport-Accumulation Models for Mineral Resources
Mineral resource studies are poised for dramatic advances through broad application of the models that guide modern petroleum research and exploration. Integrated studies, involving all the essential superposed geological processes, offer great opportunities, particularly as they can now draw on a wealth of new global tectonic and geochemical concepts and predict new types of mineral deposits. Quantitative geological mapping offers one of the greatest opportunities for significantly advancing our understanding of mineral resources and their relations to the geological features and processes. New quantitative mine-scale and regional-scale maps are both needed.
Prediction of Mineral Resource Occurrences
Mineral deposit models must be built that contain and identify the most important information for resource prediction, and practical methods must be pioneered for making resource predictions for which the confidence and accuracy can be estimated. Incorporation of organic geochemistry is required. These models are the fundamental building blocks for the advancement of mineral resource sciences. The challenge of preparing sufficiently reliable models is immense.
Numerical Modeling of the Depositional Environment of Ore Bodies
Modern computers have recently made possible numerical modeling of the complex hydrologic, geological, and geochemical systems related to ore formation. For the first time, drawing on reasonably comprehensive thermodynamic/kinetic data bases for water-rock interactions and fluid flow models, it is possible to develop integrated models that reasonably reflect natural systems for flow through media with irregular fractures, accommodating conditions under which liquids boil and allowing fluid compositions to change with time. Progression toward comprehensive computational systems could provide screening of the maze of geological variables to help indicate which are most important to ore formation.
In Situ Mineral Resource Extraction
The extraction of metals from mineral deposits by the circulation of fluids offers the potential for economic mining with potentially lower costs and less environmental disruption. The great potential and challenge of in situ mining are the integration and application of decades of advances in the diverse fields of mineral deposit geology, hydrogeology, geophysics, rock mechanics, geochemistry, structural geology, geoengineering, and chemical engineering. Determination of the effects of organisms and organic geochemistry is important.
Crustal Fluids
A major research objective is to identify and characterize each type of fluid, its distribution, and how it came to be. It would be best to start with fluids in modern environments, including modern ore-forming environments (such as the black smokers and the Red Sea) and shallow crustal settings (i.e., Smackover and Salton Sea brines). Opportunities abound for experimental research into alternate reaction paths, which reactions occur, why those paths are followed, how fast they occur, and what governs their rates. One critically important
objective is the description of metal complexing and mineral solubilities under a variety of geologically realistic conditions. Development of thermodynamic models for appropriate trace elements and volatile components will permit numerical modeling of magma differentiation and possible identification of critical variables affecting ore-bearing fluids.
Evolution of Mineral Deposits Through Time
The evolution of deposits presumably signals changes in the types and/or intensities of processes as well as in the atmosphere, oceans, thermal regimes, climate, and tectonics. Study of the evolution of resource types through time and their relations to other features should therefore guide our understanding of earth processes. In turn, this understanding should sharpen our knowledge of ore genesis and our ability to predict known and unknown deposit types that are associated with particular periods of earth history and particular geological features.
Concealed Ore Bodies
Even the largest of ore bodies occupies a tiny area of the land surface, so production of mineral wealth has been concentrated in those places where surface indications of ore bodies are strongest. A challenging research frontier is to find ways of locating buried ore bodies in the many areas where surface signs are lacking. This is rather like looking for a needle in a haystack, and part of the challenge is to develop coherent exploration strategies that are not prohibitively costly.
Intermediate-Scale Search for Ore Bodies
Mineral explorationists identify this level of search as a research frontier. The characterization of environments likely to be hosts to particular classes of ore body on the basis of recognition of ancient and modern plate tectonic environments has proved to be powerful in distinguishing blocks of land that are promising for exploration on the scale of thousands of square kilometers. On a very local scale new deposits can often be found in close proximity to existing mines. The challenge is to establish criteria permitting recognition of potential ore-bearing areas at scales intermediate between these extremes.
Energy Resources
Exploration for New Petroleum Reserves
In much of the world where the petroleum industry is less mature than in the United States, the challenge lies in exploration for new reserves in new oil fields, calling for an integrated approach to basin analysis that ranges from establishment of the tectonic environments in which basins have developed; through interpretation of depositional, structural, and thermal evolution; to an understanding of oil and gas generation within the basin, of fluid migration, and of related diagenetic change; and finally to definition of the types of traps in which oil and gas are held.
Advanced Production and Enhanced Recovery Methods
Because of the maturity of the petroleum industry in the United States, the challenge is to produce more oil from existing fields in well-known basins. Drilling, well testing, sampling, logging, advanced seismic methods, sedimentology and organic geochemistry, as well as modeling, need to be developed toward an integrated understanding of reservoir structure and dynamics. Advanced production methods such as those involving in situ bacterial modification will call for close interaction between solid-earth scientists and reservoir engineers. If bacterial techniques are to prove useful, they will depend greatly on current frontier research into the organic chemistry of petroleum.
Coal Availability and Accessibility
Total coal resources in the United States are large, but knowing only their size is not sufficient information. Some coals are not minable because their combustion produces unacceptable amounts of toxic material; others are less accessible to mining than appears from simply mapping the distribution of coal beds. The amount of coal that is available for mining and the accessibility of those "available coal resources" are much less than the overall deposit size. This is because of land-use restrictions, mining methods, and the thickness of individual beds. Such studies are being conducted in the Appalachian and Illinois coal basins. For a more realistic assessment of potentially available coal resources, they should be expanded to include all major coal-producing regions of this country.
Coal Petrology and Quality
The increased use of coal in power generation and in other more sophisticated uses requires knowledge of the quality of coal to be used. Microscopic study of the different materials that make up coal is proving important. Different materials characterize different environments of origin and different combustion responses. Information about chemical elements, mineral macerals (organic components), and their interrelationships will allow for more environmentally acceptable and economically efficient use of coal.
Concealed Geothermal Fields
Hot rock, abundant water in a sealed reservoir, and access to a market for the electricity produced must all coexist for a geothermal field to be exploitable. We may have failed to discover geothermal reservoirs with little surface manifestation. The development of remote sensing methods of detection, especially infrared survey from the air, geochemical methods, and geophysics, together make up a challenging field.
Facilities, Equipment, and Data Bases
Facilities
Activities that are too large to be operated by a single agency, university, or national laboratory can be considered facilities, and there are several examples of these in resource research. Access to advanced supercomputing capabilities is needed for testing models of processes in the shallow crust. Deep seismic studies using the consortia approaches that have been so successful in the past decade have a continuing role, and, where continental scientific drilling is perceived as an essential part of research, there is an established mechanism for bringing it into play under the existing interagency agreement. Ships of the University-National Oceanographic Laboratory Systems's (UNOLS) fleet and the Ocean Drilling Program are involved in experiments relevant to resource research, for example, under the RIDGE program. Satellite data from SPOT and Landsat are important in mineral research, and it is important that these be available to researchers on a continuing basis and at reasonable cost. Instruments with the capabilities of the High-Resolution Imaging Spectrometer (HIRIS) and the Advanced Spaceborne Thermal Emission and Reflection Radiometer (ASTER), proposed for as instruments for National Aeronatics Space Administration's (NASA) Earth Observing System, would be marvelous for resource research, as would a low-orbit magnetometer mission, such as ARISTOTELES. Gravity and magnetometry data obtained from aircraft for poorly known continental areas could also be important, but plans for development are at a very early stage.
Equipment
Most of the laboratory equipment that is needed for research in resources is similar to that used in the kinds of laboratory investigation identified in Chapters 2, 3, and 5. Advanced chemical analytical equipment and facilities for isotopic analysis, including mass spectroscopy of organic materials, has become a general need. New methods with high spatial resolution and high-precision analytical capability such as laser-ablated inductively coupled plasma-source mass spectrometry seem likely to be capable of yielding large amounts of useful information. Bacterial research may find a focus in resource and environmental research at this time. The largest field for advanced equipment development is in exploration for petroleum and minerals. Expenditures on mineral and petroleum exploration in the United States annually run at more than $1 billion, much of which is spent on the acquisition and processing of reflection seismic data. Three-dimensional seismic surveys are expected to become much more widely used in the coming decade, and processing and archiving the large quantities of data generated by this practice will present a challenge. The availability of three-dimensional seismic data will contribute greatly to our understanding of the shallow crust and will call for complementary advanced well logging and well-to-well measurements.
Mineral explorationists use advanced geophysical research techniques, including audiomagnetotelluric methods, and remote sensing from space and aircraft by methods such as side-looking radar and high-wavelength-resolution infrared spectroscopy are beginning to be used. Geochemical prospecting is emphasizing the use of pathfinder elements as complementary to direct search for metals, and soil gas exploration is developing. All these instrumental developments have a part to play in the integrated effort to understand the properties and processes of the shallow crust, which is the concept unifying resource research at this time.
Data Bases
In many ways the preparation and skilled use of data bases provide the greatest frontier challenge in resource research. This comes about because most resource issues involve handling a diverse variety of spatial data together. For example, hydrologists commonly need good digital topographic data. They also need geological maps of both surficial and bedrock distribution and may need data on water quality and quantity for both surface water and groundwater over an area of interest. The ability to access this kind of information at will and to use it as separate "layers" is the essence of the geographic information systems approach. Once the data are accessible, the challenge is to interpret them by constructing and testing models of the systems under study. In the United States access to important data bases is improving all the time, but there are opportunities for improvements that could revolutionize both the way resource research is done and the likelihood of attaining useful results. For example, mineral explorationists are compiling data bases that enable them to use pattern recognition with advanced computational techniques, including expert systems. Some high-priority data bases for resource research follow.
-
Topographic Data Base. The digital topographic data for the United States available from the U.S. Geological Survey (USGS) is as good as any in the world, but there is a continuing need to update the data base for change and to review its quality, which, simply because of its vast size, is not uniform. On a worldwide scale, digital topographic data are only very locally as good as those of the United States; we draw attention to the recommendations of other Academy committees, which, for rather different reasons, have recommended obtaining better data in various ways, culminating in a dedicated space mission to acquire a coherent high-resolution topographic data set for the land surface of the Earth.
-
Geological Map Data Base. Geological maps of the United States are of varied resolution and uneven quality, and relatively few of them are accessible in digital form. The Association of American Geologists, working with the USGS, has defined an approach to obtaining the kind of geological map coverage that is needed. The need to move more rapidly to improve geological maps of bedrock and surficial deposits in the United States and overseas is regarded
-
Geophysical Data. Data bases for geophysical data are varied and needs are diverse. A major distinction can be made between data acquired for commercial purposes such as petroleum exploration, which is not normally within the public domain, and data acquired by state and federal agencies, which is commonly freely available. Commercially acquired data are to a considerable extent traded within industry, and some publicly held information is useful for commercial activity. In the long run some commercially acquired data may enter the public domain. A serious question is whether nationally useful information may be lost through these current practices, especially for data sets where the cost of archiving in usable form is substantial. To give a single example of a publicly acquired data set that is likely to be important in exploration, airborne magnetic data at high spatial resolution will be important in the search for hidden ore bodies.
BIBLIOGRAPHY
National Research Council Reports
NRC (1981). Studies in Geophysics: Mineral Resources: Genetic Understanding for Practical Applications, Geophysics Study Committee, Geophysics Research Board, National Research Council, National Academy Press, Washington, D.C., 119 pp.
NRC (1982). Studies in Geophysics: Climate in Earth History, Geophysics Study Committee, Geophysics Research Board, National Research Council, National Academy Press, Washington, D.C., 198 pp.
NRC (1983). Opportunities for Research in the Geological Sciences, Committee on Opportunities for Research in the Geological Sciences, Board on Earth Sciences, National Research Council, National Academy Press, Washington, D.C., 95 pp.
NRC (1983). Fundamental Research on Estuaries: The Importance of an Interdisciplinary Approach, Geophysics Study Committee, Geophysics Research Board, National Research Council, National Academy Press, Washington, D.C., 79 pp.
NRC (1984). Studies in Geophysics: Groundwater Contamination, Geophysics Study Committee, Geophysics Research Board, National Research Council, National Academy Press, Washington, D.C., 179 pp.
NRC (1987). Geologic Mapping in the U.S. Geological Survey, Committee Advisory to the U.S. Geological Survey, Board on Earth Sciences, National Research Council, National Academy Press, Washington, D.C., 22 pp.
NRC (1988). Scientific Drilling and Hydrocarbon Resources, Committee on Hydrocarbon Research Drilling, Board on Mineral and Energy Resources, National Research Council, National Academy Press, Washington, D.C., 89 pp.
NRC (1989). Technology and Environment, Advisory Committee on Technology and Society, National Academy of Engineering, National Academy Press, Washington, D.C., 221 PP.
NRC (1989). Margins: A Research Initiative for Interdisciplinary Studies of Processes Attending Lithospheric Extension and Convergence , Ocean Studies Board, National Research Council, National Academy Press, Washington, D.C., 285 pp.
NRC (1989). Prospects and Concerns for Satellite Remote Sensing of Snow and Ice, Committee on Glaciology, Polar Research Board, National Research Council, National Academy Press, Washington, D.C., 44 pp.
NRC (1990). Rethinking High-Level Redioactive Waste Disposal: A Position Statement of the Board on Radioactive Waste Management, Board on Radioactive Waste Management, National Research Council, National Academy Press, Washington, D.C., 38 pp.
NRC (1990). Studies in Geophysics: Sea Level Change, Geophysics Study Committee, Board on Earth Sciences and Resources, National Research Council, National Academy Press, Washington, D.C., 234 pp.
NRC (1990). Studies in Geophysics: The Role of Fluids in Crustal Processes, Geophysics Study Committee, Board on Earth Sciences and Resources, National Research Council, National Academy Press, Washington, D.C., 170 pp.
NRC (1990). Competitiveness of the U.S. Minerals and Metals Industry , Committee on Competitiveness of the Minerals and Metals Industry, National Materials Advisory Board, National Research Council, National Academy Press, Washington, D.C., 140 pp.
NRC (1990). Ground Water Models: Scientific and Regulatory Applications , Water Science and Technology Board, National Research Council, National Academy Press, Washington, D.C., 302 pp.
NRC (1990). A Review of the USGS National Water Quality Assessment Pilot Program, Water Science and Technology Board, National Research Council, National Academy Press, Washington, D.C., 153 pp.
NRC (1990). Ground Water and Soil Contamination Remediation: Toward Compatible Science, Policy, and Public Perception: Report on a Colloquium , Water Science and Technology Board, National Research Council, National Academy Press, Washington, D.C., 261 pp.
NRC (1990). Surface Coal Mining Effects on Ground Water Recharge, Water Science and Technology Board, National Research Council, National Academy Press, Washington, D.C., 159 pp.
NRC (1991). Toward Sustainability: Soil and Water Research Priorities for Developing Countries, Committee on International Soil and Water Research and Development, Water Science and Technology Board, National Research Council, National Academy Press, Washington, D.C., 65 pp.
NRC (1991). Opportunities in the Hydrologic Sciences, Committee on Opportunities in the Hydrologic Sciences, Water Science and Technology Board, National Research Council, National Academy Press, Washington, D.C., 348 pp.
NRC (1991). Undiscovered Oil and Gas Resources: An Evaluation of the Department of the Interior's 1989 Assessment Procedures, Board on Earth Sciences and Resources, National Research Council, National Academy Press, Washington, D.C., 108 pp. plus appendices.
NRC (1991). Managing Water Resources in the West Under Conditions of Climate Uncertainty, Water Science and Technology Board, National Research Council, National Academy Press, Washington, D.C., 344 pp.
NRC (1992). Water Transfers in the West: Efficiency, Equity, and the Environment, Water Science and Technology Board, National Research Council, National Academy Press, Washington, D.C., 359 pp.
Other Reports
Department of Energy (1991). National Energy Strategy, U.S. Government Printing Office, Washington, D.C., 217 pp. plus appendices.
U.S. Congress, Office of Technology Assessment (1991). U.S. Oil Import Vulnerability: The Technical Replacement Capability, OTA-E- 503, U.S. Government Printing Office, Washington, D.C.
USGS (1987). Geologic Applications of Modern Aeromagnetic Surveys, U.S. Geological Survey Bulletin 1924, 106 pp.