2
Surficial Weathering Fluxes and Their Geochemical Controls
ABRAHAM LERMAN
Northwestern University
ABSTRACT
Surficial fluxes of materials from chemical weathering of the bedrock and soils are controlled by the following factors: volume flows and residence times of water on the continents; areal exposure of major rock types; rates of dissolution of minerals reacting with surface water and groundwater solutions; neutralization of acidity brought in part by atmospheric precipitation and in part produced within the weathering zone of the rock; and such major climate-controlled factors as temperature, and carbon fixation by plants and its storage in soils. Variations in some of these factors on the Earth's surface in recent time provide bracketing values for the environmental changes that were occurring between the time of the latest glaciation maximum (18,000 yr ago) and the beginning of the time of anthropogenic effects. The weathering fluxes based on field-analytical observations and experimentally determined dissolution rates of major silicate and carbonate minerals are analyzed for their mutual consistency. Neutralization of acidity by weathering reactions and subsequent increases in the alkalinity of waters reflect the geochemical reactivity of different rock types. The alkalinity-pH relationships of waters reacting with different rock types indicate that the system is exposed to CO2 pressures from the level of the present atmosphere to levels more than 10 times higher. The formation of new minerals in soils as a by-product of weathering of the primary source rocks provides a temporary storage reservoir that modifies the surficial runoff fluxes in response to environmental changes, of magnitudes comparable to those that occurred in postglacial time. The role of weathering reactions as geoflux buffers is compatible with the rates of soil formation and the rates of chemical denudation on global, continental, and regional scales.
INTRODUCTION
The surficial geofluxes, as understood in this volume, are the fluxes of solid, gaseous, and dissolved materials occurring at the Earth's surface. This chapter deals specifically with the geofluxes associated with chemical weathering (or weathering, for short) — an assemblage of processes that are related to biogeochemical reactions between waters and minerals or biogenic solids in the surficial sections of the continental crust. Some, and sometimes practically all, of the products of weathering are transported by running surface water and groundwater, which amounts to a release of the crustal materials to the surficial environment, and their subsequent transport on the continents and off the continents to the oceans.
In this volume, various aspects of material transport on global or continental scales have been addressed for the dissolved and suspended materials in rivers (Meybeck, Chapter 4; Milliman and Syvitski, Chapter 5); for sediment transport, crustal denudation, and river flows in Glacial times (Andrews and Syvitski, Chapter 7; Kump and Alley, Chapter 3; Baker, Chapter 6); for eolian transport (Rea et al.,Chapter 8); and for the chemical, biogenic, and detrital material fluxes in the oceans (Dymond and Lyle, Chapter 9; Hay, Chapter 1; Martin and Sayles, Chapter 10; see also Schneider and Kellogg, 1973; Milliman, 1993). In view of the importance of continental waters to the weathering geofluxes, the present chapter addresses the following aspects of the weathering releases:
-
the residence times of surficial water flows on the continents;
-
the main mechanisms of chemical weathering of surficial crustal rocks and soils;
-
the dependence of weathering processes on the major environmental changes, comparable to those that occurred since the latest glaciation peak, 18,000 yr ago;
-
the magnitudes of variation in the weathering geofluxes that are controlled by water-rock chemical interactions and water flow; and
-
the resistance to change displayed by the surficial geofluxes when the surficial environment is exposed to major environmental perturbations.
Water flow is the main agent of material transport from the continents to the oceans and it accounts for the major fraction of the masses of solid and dissolved materials transported from the continents to the oceans: about 90 ± 5 percent of the global material flux from land to the oceans is due to water runoff from the continental surface, and the remaining 10 ± 5 percent of the flux are carried by winds (Garrels and Mackenzie, 1971; Goldberg, 1971; Lerman, 1979; Drever, 1988; Meybeck, Chapter 4, this volume; Milliman and Syvistski, Chapter 5, this volume; Rea et al.,Chapter 8, this volume).
Water as a transport agent of continental materials is unique in its continuous movement in a cycle from the oceans to the atmosphere and land, with two-way precipitation and evaporation flows, its occurrence on the Earth's surface as a liquid, gas, and solid; and its ability to react chemically with minerals and biogenic materials, dissolving or altering them in the process. The dual characteristic of water as a substance interacting chemically with the crustal solids and as transport agent carrying dissolved and solid materials is its most important feature in the context of the surficial geofluxes. The flows of ions and electrons occurring in chemical reactions between water and solids underlie such macroscopic processes as dissolution and precipitation, oxidation and reduction, ion exchange, recrystallization of minerals, and the entire complex of biological primary production and respiration.
Ideally one would like to be able to estimate the magnitudes of the surficial geofluxes under different environmental conditions for the past, present, and future by relying on conceptual and mathematical models of the surficial weathering and transport processes. For example, an adequate model for estimation of the weathering geofluxes should ideally be based on fundamental knowledge of such processes as the mechanical disaggregation of rocks by moving ice sheets, mineral dissolution and precipitation rates under different temperatures, chemical composition of flowing waters as controlled by inorganic chemical reactions and biological processes, and the principles of fluid transport by water and wind. Such straightforward estimates, however, derived from "bottom-up" models based on fundamental data and leading to reliable conclusions on regional, continental, or global scales have been achieved more often as exceptions rather than as rules. The complexity of the surficial environment and changes occurring within it on different physical and time scales make many of the estimates based on fundamental principles useful only as orientational values.
On a time scale of some 16,000 yr, from the peak of the last glaciation about 18,000 yr ago to early human times that preceded the spreading of agriculture and the later industrial activity, a number of major environmental changes were likely to affect the surficial releases by the weathering geofluxes. These changes included the reduction of the ice-sheet cover of the continents in the Northern Hemisphere (Kump and Alley, Chapter 3, this volume), the rise of global sea level by some 80 to 120 m; inundation of the continental shelves; an increase in the continental drainage area; changes in the areal exposure of crystalline and carbonate rocks (Meybeck, 1984); possible changes in the volumes of water discharge from land to the oceans; broad
changes in the zonation of the global climate, temperature, and distribution of the vegetation types (NGS, 1988); and likely changes in the storage patterns of the organic material in soils.
The broad global and continental-scale changes in the environment, as listed in the preceding paragraph, remain distinct from the shorter-term events that may have pronounced effects on the physical environment and the major material transport paths. Catastrophic events, such as volcanic eruptions, earthquakes, or floods, may profoundly change the physical environment on a more or less great regional scale, affecting in the process the weathering releases through changes in the water drainage pattern, type of rock exposure, or vegetation cover. The entire scope of anthropogenic activities includes a long and continuously growing list of environmental perturbations that may affect to a variable degree the background surficial weathering and transport, on scales from global to regional. Such a list may begin with the possible global climate change due to anthropogenic increases in the atmospheric greenhouse gases and the anticipated changes in temperature and continental water discharge; continue to the anthropogenic accumulation on land and in waters of reduced metals (iron, copper, zinc, and others), chemically reactive calcium-silicate phases in constructional cements, and by-products of the mining and organic chemical industries; and go on to the chemical changes due to fertilizers in soils, and a changing residence time of organic carbon on land due to reduction of the forest-covered area of the continents.
The physical environment of the surficial weathering releases that is considered in this chapter is shown schematically in Figure 2.1. Water evaporating from the ocean surface precipitates as snow and rain; meltwater and net atmospheric precipitation (precipitation less evaporation) flow over the land surface, penetrate underground, reside for variable lengths of time in lakes, and continue to the oceans. Chemical interactions between water and mineral or biogenic solids result in net releases to, and transport by, water flow.
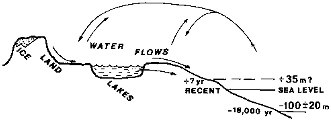
FIGURE 2.1 Continental waters and domains of surficial weathering fluxes.
GLOBAL AND ZONAL WATER FLOWS
Surficial Flows and Storage in Lakes
The volume flow or discharge of water from land to the oceans varies greatly over the globe. To allow for differences between water discharge from land areas of different sizes, a more meaningful measure of volume flow is the specific discharge or discharge per unit area q:

where Q is water discharge (km3/yr) and A is the surface area (km2) of a drainage basin as measured on a map.
Specific water discharge values for 5°-wide latitudinal zones of the globe have been compiled for water flows from the continents to the oceans, as well as to the internal drainage basins, by Baumgartner and Reichel (1975). Specific discharge is taken equal to the difference between the annual amounts of atmospheric precipitation and evaporation, and as such it includes both the surficial runoff and the groundwater flow. The values of specific discharge are representative of the water volumes flowing to the oceans from each latitudinal zone if the volume storage on the surface and underground remains constant. An increase in the annual amount of net precipitation (i.e., precipitation less evapotranspiration from land) may be expected to be initially distributed in some greater storage of water on the land surface and underground, ultimately finding its way in the flow to the oceans. A large uncertainty associated with estimates of the length of time it would take for the surface runoff to respond to a major change in the amount of water input to land from the atmosphere is related to the knowledge of the volumes and residence times of surficial water and groundwater.
The value of the surficial global water discharge to the oceans is 3.74 x 104 km 3/yr, excluding the discharge from Greenland and Antarctic, and the global drainage area for discharge to the oceans is nearly 1 x 108 km2 (Baumgartner and Reichel, 1975; Meybeck, 1984; Table 2.1). The volume of global continental ice and snow, excluding the Antarctic and Greenland, is about 122,000 km3 (Barry, 1985). Faster melting of the continental snow and ice sheets at a rate of 1 percent per year would initially increase the discharge from land by up to 3 percent. Increased storage capacity of the meltwater in big periglacial lakes, such as the big lakes occurring on the margins of the Canadian shield and the older lakes (Baker, Chapter 6, this volume), might reduce this estimate of an initial increase in global discharge. Ultimately, however, continuous melting would add more water to the continental surface and likely
exceed its storage capacity for making new lakes. The present-day continental ice and snow volume, 122,000 km3, is almost equal to the global volume of freshwater lakes, 125,000 km3 (Table 2.1).
The estimate of the freshwater lake volume (Table 2.1) excludes such big bodies of brackish or saline waters as the Caspian Sea, Aral Sea, Great Salt Lake, and other saline lakes. There are relatively few large-volume lakes in the world, with water volumes greater than 1000 km 3—10 or 11—and even fewer (3) with volumes greater than 10,000 km3. The 10 largest lakes account for about 61 percent of the total freshwater lake volume, and lakes of volume greater than 1 km3 account for 67 percent of the total (Lerman and Hull, 1987). It is difficult to decide where a line should be drawn defining a lake of ''a minimum size." A conventional practice of classifying lakes by their surface areas rather than volume does not give the measure needed for the water retention times on land. An arbitrary cutoff volume of about 1 km3 was adopted here for lakes, and the plot in Figure 2.2 of the water residence times for a sample of such lakes indicates a modal class of residence times between about 3 and 10 yr, with a mean of about 5.5 yr. The geographic distribution of the lakes, particularly of the bigger ones, is very uneven: most of the biggest lakes of known water residence time occur in the Northern Hemisphere, such as the Laurentian Great Lakes discharging to the North Atlantic and Lake Baikal discharging via the Angara River to the Arctic Ocean.
TABLE 2.1 Water Residence Times on Land and Background Data
Parameter |
Value |
References and Comments |
|
Global water discharge to Oceans (QT) |
3.74 X 104 km3/yr |
Baumgartner and Reichel (1975) Meybeck (1984) |
|
Continental drainage area for discharge to oceans (AT) |
9.9913 X 107 km2 |
Baumgartner and Reichel (1975)| Meybeck (1984) |
|
Continental ice and snow cover (without Greenland and Antarctic) |
1.22 X 105 km3 |
Barry (1985) |
|
Freshwater lakes |
|
Herdendorf (1982) and additional References in Lerman and Hull (1987) |
|
Volume (VL) |
1.25 X 105 km3 |
|
|
Surface area (AL) |
8.55 X 105 km2 |
|
|
Drainage basin area (ADB) |
=2.5 X AL km2 |
|
|
Water residence times |
|
This chapter |
|
Freshwater lakes (tL) |
= 5.5 yr |
|
|
Rivers without major lakes in their basins (tr) |
0.65 to 6.5 yr |
|
|
Global average for discharge to oceans (t) |
0.3 to 3 yr |
|
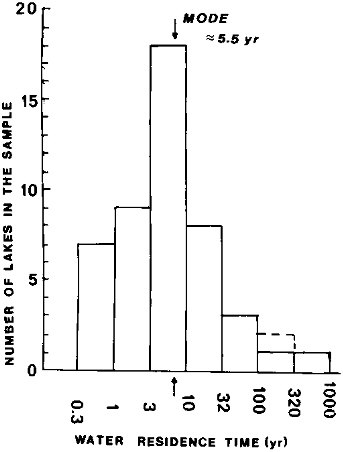
FIGURE 2.2 Residence times of water in lakes of volume >1 km3. Residence time equals volume/outflow. From data tabulated in Lerman and Hull (1987).
Residence Times of Zonal Water Discharge
The residence time of water on the continents may be expected to vary greatly, from the short flow times for water in river channels to the much longer times for waters in deeper aquifers. For big rivers, the residence times of waters in the main river channel are relatively short, if estimated as times of flow, at velocities of 10° cm/s, over distances of 103 km—less than one year. For the latitudinal zonal runoff from the continents, the water residence time is numerically equal to the volume pore space of the surface rocks through which it flows, divided by the volume discharge. The net thickness of the porous layer for surface flow may be bracketed between 10 cm and 1 m, corresponding to a two- to fivefold variation in thickness of a rock or soil layer, of porosity 50 to 20 percent. Then the mean residence time of water, computed as shown below, is bracketed by a factor of 10 (Eq. 2.2):

This cumulative distribution of the zonal water residence times is shown in Figure 2.3. The median residence time is
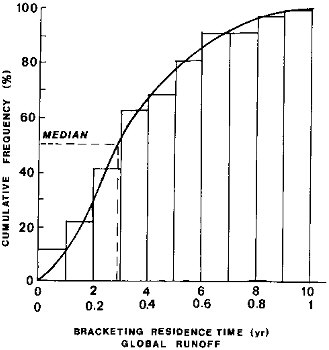
FIGURE 2.3 Residence times of water on the continents with respect to discharge to the oceans. Water runoff (discharge per unit area, q) data for 5°-wide latitudinal zones, from 80° N to 80° S (Baumgartner and Reichel, 1975), and Eq. (2.2). Assumed net thickness of the discharge conducting layer, 10 to 100 cm, results in a tenfold range of residence time values on the abscissa.
about 3 yr at the long end and about 0.3 yr at the short end of the estimates. In arriving at these estimates, it should be borne in mind that small values of specific discharge q that are characteristic of dry or cold high latitudes, produce longer residence times, accounting for the conclusion from Figure 2.3 that 50 percent of the surface discharge may have residence times longer than 3 yr.
Residence Times on a Global Scale
Global discharge to the ocean includes the rivers that drain the major lakes and lake systems, as well as river and land-surface flows that do not pass through big lakes in their drainage basins. Estimates of the two kinds of discharge may be arrived at as follows.
As shown schematically in Figure 2.4, the total water discharge to the oceans QT is the sum of a discharge from the lakes (QL) and discharge from rivers without lakes (Qr):
QT = QL + Qr (km3/hr) (2.3)
A fraction of the total flow passing through lakes is a ratio of the global freshwater lake volume VL and the mean residence time τL (data in Table 2.1):

The straight river discharge, from Eq. (2.3), is therefore

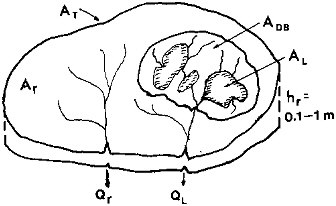
FIGURE 2.4 Global land drainage area for discharge to the oceans (AT), surface area of freshwater lakes (AL) and their drainage basins (ADB), and the remaining area for rivers without major lakes in their drainage basins (Ar). A surface layer of rock and soil for conductance of the discharge has a net thickness hr = hф, or bulk thickness times porosity.
The residence time of the river discharge portion Qr is

where, as shown in Figure 2.4, hr is the net thickness of a layer through which water flows (hr = 10 to 100 cm) and Ar is the drainage area of the rivers without lakes. This drainage area is the difference between the global total drainage area AT and the sum of the global lake surface area AL and the area of the lake drainage basins ADB (Table 2.1):
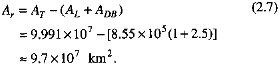
The total area of the lake surface and drainage basin is small relative to the land area, and the factor of 2.5 assumed for the drainage basin to lake surface area does not significantly affect the estimate. From the preceding values, an estimate of the residence time of straight river flow from the continents to the oceans is, from Eq. (2.6),
τr = 0.65 to 6.5 yr.
This estimate for the residence time of the direct runoff is comparable to the estimate of the mean water residence time in lakes, the lower and upper bounds reflecting the factor of 10 in the assumed range of the effective thickness of the water-conducting surficial layer hr.
Summary of Water Flows and Residence Times
Mean residence times of water on the land surface, partitioned into a river flow component passing through lakes and a component running off directly to the oceans are of the same order of magnitude: a mean of 5.5 yr for the lake outflow and 6.5 yr for an upper-end estimate of direct runoff. The mean residence time for lake-draining rivers does not well represent the very wide range of lake residence times that extends up to several centuries and their geographically uneven occurrence.
If the water residence time (τ) estimates are taken at their face value, the response times would be short (years to decades) for changes in the chemical characteristics of surface flows—changes in inputs from chemical weathering or other sources. A measure of t is a characteristic time of the system response to changes in the chemical inputs.
WEATHERING FLUX
A Conceptual Mode
Water flowing over the land surface and within a shallow conductive layer of some thickness within the rocks and soils reacts with minerals, producing some dissolution that results in a net transport of dissolved materials. Figure 2.5 illustrates schematically the direction of the surficial water discharge as it passes through a porous layer of some net thickness hr, assumed to be bracketed between the values of 10 cm and 1 m. A material balance equation for the process depicted in Figure 2.5 should take into account the various processes that either add or remove solutes in the runoff. Such processes include inputs of dissolved materials from dissolution of bedrock and soil minerals; inputs from atmospheric precipitation; and either storage of dissolved materials in, or their removal from, the pools of exchangeable cations in secondary minerals in soils or the biomass (Drever, 1988, p. 224; Wright, 1988). The transport balance equation for net weathering as shown in Figure 2.5 may be written in the following form:
[Transport in solution] =
[bedrock and soil mineral dissolution] ±
[leaching from, or storage in, secondary minerals] ±
[input from, or storage in, vegetation] +
[input from atmospheric precipitation].
Soil is a product of chemical alteration of the bedrock and organic matter accumulation from vegetation, and it contains some of the primary minerals of the bedrock, some newly formed secondary minerals (such as clays), and organic matter (humus) (Sposito, 1985). Thus the entry in
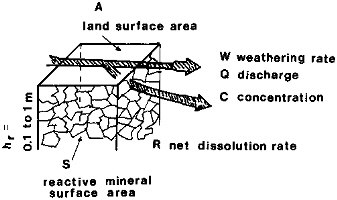
FIGURE 2.5 Water flow through a porous surface layer of bulk thickness h and net thickness hr = h, as in Figure 2.4. A is the horizontal projection or map area; S is the surface area of minerals in contact with water flow within the layer h.
the balance equation labeled bedrock and/or soil mineral dissolution represents total possible input from dissolution processes. If the pool of secondary minerals in soils and the mass of vegetation are constant—that is, the rate of soil growth is balanced by its erosion rate and the vegetation growth is balanced by its decay—then the flux of dissolved material in solution is equal to the rate of its supply from mineral dissolution. The question of a constant mass of secondary minerals in soils and of vegetation deserves further notice.
Experimental work and field studies demonstrate that addition of acid waters to rocks and soils generally results in greater weathering (Likens et al., 1977; Cronan, 1985; Folster, 1985; Wright, 1988; Probst et al., 1990, 1992). The same authors have also recorded that, on geologically short time scales of months to a few years, higher water acidity variably results in an increased uptake of cations by vegetation and increased leaching from the so-called "cation pool of soil." The exchangeable cations in soils, however, represent only a very limited pool relative to the bedrock that is available to dissolution and release to the weathering flux: it has been estimated that at the observed rates of cation dissolution from soils, the entire cation-exchange pool would be exhausted in about 50 to 100 yr if there were no replenishment from the bedrock dissolution (Schnoor and Stumm, 1986). Therefore it is important to emphasize that prehuman background weathering fluxes are effectively those of the rock mineral dissolution rather than a transient perturbation of the pool of exchangeable cations in the secondary minerals of soils.
Weathering Flux Equations
Mass and Linear Weathering Rates Based on the conclusions of the preceding section, the flux of dissolved materials in global runoff is treated as a measure of bedrock and soil weathering. The rate of weathering is usually expressed as mass loss per unit area of the geographic rock surface per unit of time:

where W is the net weathering rate (mol or g/cm2/s), C is concentration in solution (mol/cm3), q is specific discharge defined in Eq. (2.1) (cm/s), R is a mineral dissolution rate (mol/cm2/s), A is a horizontal or map surface area (cm2), and S is the reactive surface area of the rock in contact with water, as shown in Figure 2.5 (cm2). The mass weathering rate W can be converted to a linear rate Wh (cm/s) that represents a vertical recession of a solid surface due to dissolution:

where ρ is the mineral density (mol/cm3) and ф is the rock porosity (0 - ф < 1). For a solid of zero porosity and uniform density, the linear dissolution rate is the quotient W/ρ. The preceding relationships for the rate of weathering are simolifications that do not reflect the detailed nature of the various mechanisms of mineral-water interactions (Murphy et al., 1989).
Rates of weathering of different rocks and soils, and continental units are summarized in Table 2.2. These rates have generally been estimated in the literature sources by using procedures similar to the computational method of Eq. (2.8): the rates are based on the analytically determined concentrations of dissolved constituents in surficial and shallow-ground runoff, and water discharge data summed for the drainage areas of regions, continents, and the globe.
Weathering rates W, computed as a product of concentration of dissolved constituents in river water and river discharge, produce near-linear plots in graphs of log W as a function of log q for sets of rivers draining geologically diverse terrains (Berner and Berner, 1987; Meybeck, Chapter 4, this volume). The log-log plots of W against q also indicate considerable variability in river-water concentrations of solutes that reflects differences in lithology and hydrology of the drainage basins. An approximately linear relationship between log W and log q is a feature that holds for the river basins where concentration and discharge values presumably reflect averages over periods of years. On a shorter time scale, however, concentrations of land-derived materials in rivers may depend pronouncedly on seasonal water discharge.
Surface Areas: Reactive Rock (S) and Land Surface (A) The ratio of the reactive surface area of mineral grains exposed to water below a unit of land surface area, as shown in Figure 2.5, appears as a quotient S/A in the weathering rate Eq. (2.8). The reactive surface area of minerals is not necessarily equal to the physical surface area of the grains, as commonly determined by the gas adsorption method. White and Peterson (1986) concluded that the reactive surface areas of minerals, computed from laboratory dissolution experiments, may be smaller that the physical grain areas by a factor of as much as 100. A simple estimate of a representative range of values for the area ratio S/A may be obtained as follows. The physical surface areas of common types of sediments are in the range of 1 to 20 m2/g (104 to 105 cm2/g). The column of a rock or sediment through which discharge is conducted (Figure 2.5), may be characterized by some thickness h, effective porosity ф and mineral density ρ. The mass of
TABLE 2.2 Chemical Weathering Rates (W) for Different Rock and Soil Types, from Different Areas (see also Figure 2.7)
|
Weathering Rate |
|
|
Weathering |
Mass/Area/Time (W) |
Length/Time (Wh) |
References and Comments |
Global mean |
33 to 40 g/m2/yr |
14 ± 1 mm/ka (ka = 1000 yr) |
Meybeck (1988), mass rate converted to Linear rate for bulk density, ρ ≈ 2.5 to 2.7 g/cm3 |
Continental averages |
|
|
Garrels and Mackenzie (1971) |
Africa |
24 g/m2/yr |
|
|
Asia |
32 g/m2/yr |
|
|
Australia |
2 g/m2/yr |
|
|
Europe |
42 g/m2/yr |
|
|
North America |
33 g/m2/yr |
|
|
South America |
28 g/m2/yr |
|
|
Shields and sediments |
— |
5 to 30 mm/ka |
Stallard (1988) |
Silicate rocks |
— |
80 mm/ka |
Stallard (1988), Andean drainage |
Carbonates and shales |
— |
200 mm/ka |
Stallard (1988), Andean drainage |
Evaporites |
— |
700 mm/ka |
Stallard (1988), Andean drainage |
Volcanics |
18 g/m2/yr |
7 mm/ka |
Drever (1988), andesitic terrain in Absaroka Mts., Wyoming |
Sandy-silty soils |
0.05 to 0.27 eq/m2/yr |
7 to 40 mm/ka |
Cronan (1985) |
Small stream watersheds and catchment areas |
0.024 to 0.20 eq/m2/yr |
4 to 30 mm/ka |
Fölster (1985), data compiled for areas in United States, Norway, Sweden, England, and Germany |
Various soils |
|
|
|
Loess soils |
0.04 to 0.10 eq/m2/yr |
6 to 15 mm/ka |
Fölster (1985) |
Soils in lake drainage basins |
0.06 eq/m2/yr |
9 mm/ka |
Wright (1988), literature data for Adirondack Mts., New York, and |
Deglaciated terrains |
0.93 eq/m2/yr |
140 mm/ka |
Cascade Mts., Oregon |
NOTE: Conversion of the weathering rates in units of g/m2/yr to units of vertical recession of the land surface, in millimeters per 1000 yr (mm/ka), was done by dividing the mass rate by a rock density value of about 2.5 g/cm3; see Eq. (2.9). The rates in eq/m2/yr are often based on concentrations of four cations (Ca2+, Mg2+, Na+, and K+) in runoff. To convert to millimeters/year, the values in eq/m2/yr are multiplied by averaging factors of 1.5 mol/eq and molar density of minerals (100 cm3/mol), the latter based on round-figure values of about 250 g/mol for feldspars and a density of 2.5 g/cm3. Such Wh estimates are good to a factor of about two. |
such a column may be estimated by using representative values of

and a mass of

The area ratio within such a material column is, approximately,
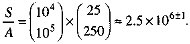
From the preceding estimates of the surface area in a 0.1- to 1-m-thick layer, the ratio of S to unit map-surface area A is

A more accurate computational estimate of the rock surface area S can be made if the particle-size and shape distributions for the surface rock layer are known. In Figure 2.6 are shown the particle-size distributions of a granite in Wisconsin and of a residual soil. The weathered rock is, as expected, characterized by larger particle sizes than the overlying soil, and the size distribution for the rock is pronouncedly skewed toward larger particles. The particle-size spectral distributions in Figure 2.6 indicate
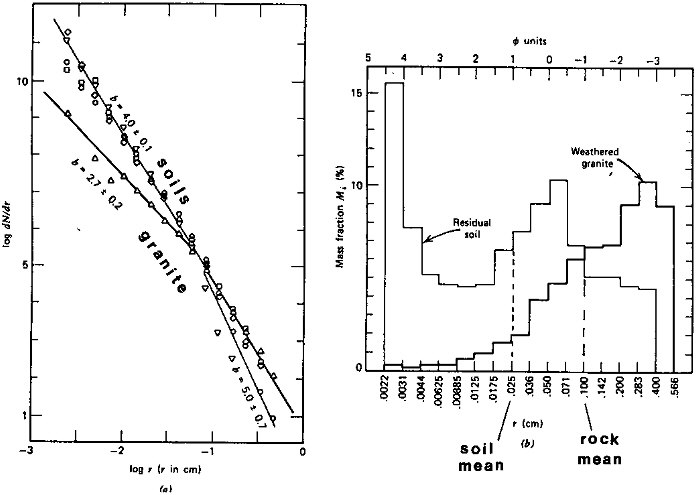
FIGURE 2.6 Particle-size distributions for a Wisconsin granite, its residual soil, and residual soils on other crystalline rocks. Right: frequency histogram for granite and soil; left: particle-size spectra for the granite and residual soils. From Lerman (1979) with permission of John Wiley & Sons, New York.
that the smaller particles in soils may become more abundant at the expense of the larger particles in the parent rock.
The total surface area of particles in a sediment column of unit cross-sectional area and height h can be written as

where ф is the sediment layer porosity, h is the bulk thickness (i.e., solid particles and pore space) per unit area of the layer, s(r) is the surface area of a particle of a characteristic linear dimension r, f(r) is the number of particles with size between r and r + dr occurring in a unit volume of the sediment layer (particle-size distribution is dN = f(r) dr, where N is a number of particles per unit volume), and r1 and r2 are the lower and upper size limits of the particle sample.
For spherical particles of a mean radius r, the ratio of the areas S/A is

The number of spherical particles of radius r in a unit volume of solid, 1-ф, is

which gives, in combination with Eq. (2.12),

The mean particle sizes for a weathered rock and a residual soil on it may be taken from the data in Figure 2.6: (rock) r ≈ 0.1 cm, (soil) r ≈ 0.025 cm.
By using the preceding estimates, the surface area quotient S/A can be computed as

The latter estimate of the area ratio S/A is lower than the estimate in Eq. (2.10), emphasizing the uncertainties involved in the estimates of two fundamental parameters: the thickness of the rock-soil layer conducting most of the surficial water flow and the surface area of the reactive solid fraction.
Mineral Dissolution Rates (R)
Terminology For a discussion of weathering processes in mineral-water systems, a brief clarification of some of the concepts and terms is given:
-
Dissolution is a process of transfer of mass from a solid to the aqueous phase. A rate of dissolution is measured in units of mass per unit of surface area of a dissolving mineral per unit of time, commonly in units of mol/cm2/s.
-
Solubility of a solid phase is a measure of the mass that can be dissolved in an aqueous solution at an equilibrium with the solid. Units of solubility are those of concentration—mol/cm3, for consistency with the units of the dissolution rate.
-
Leaching is a broader term, frequently used in soil science and environmental engineering literature, and it denotes transfer of some of the components of the solid to solution. Thus, leaching may indicate a net result of such combinations of processes as ion exchange, dissolution, and precipitation; recrystallization of a solid and release of some of its minor constituents; or a preferential dissolution of some of the solid components that is also referred to as incongruent dissolution.
Dissolution Rates of Silicate and Carbonate Minerals As a generalization, highly soluble minerals are often also characterized by relatively faster dissolution in water. This observation has historically lead to a not-always-valid conclusion that the rate of dissolution of a solid is directly related to "its distance from saturation" or to the difference between instantaneous concentration in solution and concentration at saturation. In such a mode, dissolution is initially relatively fast, but it slows down as an equilibrium or saturation is approached.
The common evaporite minerals halite (NaCl), gypsum (CaSO4-2H2O), and anhydrite (CaSO4) dissolve faster than carbonates and silicates in pure water (Van Name, 1929); and the surficial environment, evaporite deposits containing these minerals also weather faster than rocks made of silicates and carbonates (Garrels and Mackenzie, 1971, with references to earlier observations; Colman and Dethier, 1986; Stallard, 1988, 1989). The rates of dissolution of a number of silicate minerals are listed in Table 2.3. Additional information on dissolution rates of these and other silicate minerals can be found in the publications referenced in the table.
A somewhat striking characteristic of the dissolution rates of the common silicates, even for a small sample of minerals listed in Table 2.3, is a variation by a factor of up to 105 in the rates from one mineral to another, quartz
TABLE 2.3 Dissolution Rates R Silicate Minerals.
|
|
|
Dependence of R on [ H+]n |
|
|
Mineral |
Formula |
Log R (mol SiO2/cm2/s) |
n for Acid Range |
n for Alkaline Range |
Reference |
Albite |
NaAlsi3O8 |
– 15.8 to – 14.3 |
0.5 |
–0.3 |
Chou and Wollast (1985) |
Anorthite |
CaAl2Si2O6 |
– 15.5 to – 13 |
1.0 |
–0.3 |
Brady and Walther (1989) with additional references |
Chrysotile |
Mg3Si2O5(OH)4 |
– 16.0 to – 15.5 |
— |
–0.3 |
Bales and Morgan (1985) |
Diopside |
CaMgSi2O6 |
– 14.5 to – 12 |
0.5 |
— |
Schott et al. (1981) |
Forsterite |
Mg2SiO4 |
– 13.8 to – 12 |
0.5 |
–0.3 |
Blum and Lasaga (1988) |
Quartz |
SiO2 |
– 16.3 to – 14.5 |
~0 |
–0.3 |
Brady and Walther (1989) |
Nepheline |
NaAlSiO4 |
– 12.5 to – 10.5 |
1.0 |
–0.3 |
Tole et al. (1986) |
NOTE: Lower values of R apply to the near-neutral pH range from about 5.5 to 7.5. Rates increase as the pH becomes lower or higher than near-neutral (see Figure 2.7). |
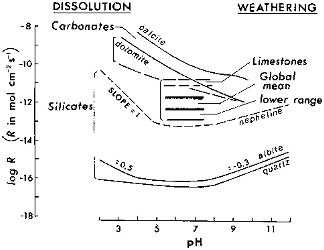
FIGURE 2.7 Dissolution rates of silicates and carbonates as a function of pH. Band bracketing dissolution rates for silicates are from data in Table 2.3. For carbonates, data of Plummer et al. (1978) are used for calcite, and of Chou et al. (1989) for calcite and dolomite. Chemical weathering rates shown for comparison with the experimental values are from Table 2.2. Adopted from Lerman (1979).
having reportedly the lowest rates at 25°C. The band of dissolution rates of silicates is plotted in Figure 2.7.
For carbonates, the experimentally determined rates of dissolution for calcite and dolomite (Plummer et al., 1978; Chou et al., 1989), shown in Figure 2.7, are significantly higher than those for silicates; this feature finds its reflection in the weathering rates of carbonate rocks, which are generally higher than those of crystalline silicate rocks.
HYDROGEN-ION AND TEMPERATURE DEPENDENCE OF DISSOLUTION RATES
The pH and Natural Acidity
The rate of dissolution of a mineral is generally some function of the environmental conditions, such as the composition of the aqueous solution and temperature. The H+ concentration (a characteristic of the aqueous solution composition) and the temperature are two of the environmental variables addressed in this section.
The silicate and carbonate mineral dissolution rates are plotted in Figure 2.7 as bands bracketing the higher and lower values of the dissolution rates of the individual minerals. For the silicates it should be noted that in acidic solutions of pH ≤ 5.5, the dissolution rates increase in proportion to a power of the hydrogen-ion concentration as [H+]0.5 to [H+]1.0. The solution pH at which an increase in the dissolution rate begins has been reported to vary from mineral to mineral, occurring between a pH of about 5.5 and lower.
In the near-neutral pH range from approximately 5.5 to 7.5 or 8, the dissolution rates are about constant near the low end of each range shown in Table 2.3. In alkaline solutions, above pH 7.5 to 8, the dissolution rates increase in proportion to [H+]-0.3. Theoretical interpretations of the dependence of silicate dissolution rates on a power of [H+] have been advanced through the mechanisms of surface reactions and transition-state theory (Wollast and Chou, 1988; Schott and Petit, 1987).
Carbon dioxide is the main atmospheric gas that determines the background acidity of atmospheric precipitation and fresh waters exposed to the atmosphere. Variations in the reported CO2 content of the atmosphere during the past 18,000 yr indicate an increase from about 180 parts per million by volume (ppmv) or a PCO2 of 1.8 x 10-4 atm, through the preindustrial-age value of about 280 ppmv, to the present concentration of 345 ppmv (Barnola et al., 1987; Siegenthaler and Oeschger, 1987). Such variations in the carbon dioxide partial pressures could have had only very minor effects on the pH of pure rainwater, which indicates a decrease of about 0.14 pH unit:
PCO2 = 1.8 x 10-4 atm: pH = 5.73 (5°C) and 5.79 (25°C),
PCO2 = 3.4 x 10-4 atm: pH = 5.59 (5°C) and 5.65 (25°C).
For ocean water, in anticipation of a continued anthropogenic CO2 increase in the atmosphere, Whitfield (1974) estimated that a rise from 313 to 453 ppmv would lower the pH of surface ocean water by about 0.08 pH unit, from 8.24 to 8.16.
Additional sources of background natural acidity in atmospheric precipitation are the oxidation products of reduced nitrogen and sulfur emissions, which yield H2SO4 and HNO3, and some HCl emissions from active volcanoes. On the land surface, the primary sources of acidity are the oxidation of pyrite (FeS2) to produce sulfuric acid; the release of organic acids by living plants and humus; and the oxidation of organic matter, which mostly contributes CO2 to groundwaters. The importance of the latter process to weathering releases is discussed later.
For purposes of visualization in Figure 2.7, where an increase in the silicate dissolution rate is shown to begin at pH ≈ 5.5, a decrease in the pH of a solution by 1 pH unit may result in a three- to tenfold increase in the dissolution rate.
Temperature Effects on Dissolution Rates
The warming trend since the glaciation peak about 18,000 yr ago translates into a rise in mean global temperature from about 10 to 16°C at present (Lorius et al., 1985; Jouzel et al., 1987). Considerably greater variations in temperature char-
acterize the Northern Hemisphere at present. Any direct effects of temperature on the dissolution rates of silicate minerals in the bedrock and soils may overlap the effects of increased biological productivity and carbon fixation by plants, with an attendant increase in bacterial activity in soils that may be a significant contributor to weathering. An increase in the rate of net primary production of land plants by a factor of 4 to 5 occurs for a move from cold regions (0 to 5°C, carbon fixation rate 500 to 750 g/m2/yr) to the humid tropics (about 25°C, 2000 to 2700 g/m2/yr) (Lieth, 1975). An increase in bacterial activity from colder to warmer latitudes is also indicated by the lower humus content in tropical soils because of its faster decomposition than in temperate and northern latitudes (Post et al., 1982). Coincidentally with the north to south increase in plant productivity by a factor of 4 to 5, an increase by a similar factor in the dissolved SiO2 of rivers from the tundra to the humid tropics has been reported by Meybeck (Chapter 4, this volume); from about 3 to 15 mg/liter. A similar increase may be anticipated in an inorganic silicate-water system due to a temperature rise from 5 to 25°C, as shown below.
The dependence of the rate of a dissolution reaction on temperature is an Arrhenius-type equation

where the subscript 0 denotes a reference temperature and reaction rate, R is the dissolution rate (mol/cm2/s), T is temperature (kelvin), R is the gas constant, R = 8.314 ∞ 10-3 kJ/mol/°C, and ∆E is an activation-energy parameter for the reaction (kJ/mol). The values of ∆E for commonly occurring silicate minerals, in the pH range from about 4 to 8, vary from 38 kJ/mol for diopside (Table 2.3) to 72 kJ/ mol for quartz (Rimstidt and Barnes, 1980; Schott and Petit, 1987; Brady, 1991). By taking a mean of this range, with the temperature increasing from 5 to 25°C, the dissolution rate increase can be computed as
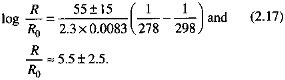
As pointed out previously, an increase in the silicate dissolution rate by a factor of about 5 due to a temperature increase from the tundra to the tropics coincides with an increased rate of carbon fixation by plants. It must also be viewed in light of the experimental results on the dissolution of silicate and other types of minerals by soil bacteria (Eckhardt, 1985; Berthelin, 1988), where bacterially induced rates of dissolution were reported as up to 100 times faster than in the absence of bacteria.
WEATHERING AND DISSOLUTION
The estimates of mean weathering rates for the continental surface are plotted for comparison with the experimental dissolution rates for silicates and carbonates in Figure 2.7. The surface area for global discharge is given in Table 2.1. The global estimates and estimates for the major continental drainage systems fall within a bracketing range of about a factor of 10 of each other. The global mean, as may be expected, falls between the slower-weathering silicate rocks and the faster-weathering limestones.
The differences between the weathering rates based on field-analytical data and the experimentally determined dissolution rates of the individual minerals (Tables 2.2 and 2.3; Figure 2.7) are, in many cases, difficult to explain (Deike, 1989). For example, Paces (1985) and Velbel (1985) concluded that field dissolution rates, based on the best reasonable estimates of the mineral surface areas, are much slower than the rates indicated by laboratory results.
The weathering rates of sandy-silty soils, volcanic rocks and crystalline silicate rock terrains (Table 2.2) are in the range of about 18 to 200 g/m2/yr. The dissolution rates of common silicate minerals given in Table 2.3 are much lower, 10 to 10-5 g/m2/yr. For dissolved SiO 2 in rivers, taken as a measure of the weathering rate of crystalline bedrock, the computed weathering rate W is also considerably higher than the experimentally determined dissolution rates R of the common silicate minerals:

The big differences between the weathering and dissolution rates may be reconciled by the surface-area ratio factor, S/A, of comparable magnitude, as discussed earlier. However, any such agreement between the weathering rates may fortuitously overlap the effects of other biogeochemical factors in the weathering process.
For the global flux of calcium in rivers, the following fractions have been attributed to different rock types (Wollast and Chou, 1988, based on data in Wollast and Mackenzie, 1983):
from carbonate weathering: 68 percent
from silicate weathering: 12 percent
from evaporites: 14 percent
from sulfide oxidation: 6 percent.
In the preceding balance of calcium sources in weathering, about 80 percent should be accounted for by weathering of carbonate and silicate rocks. The outcrop area of carbonate rocks on continents at present time is about 16 percent of the total area (Meybeck, Chapter 4, this volume). The weathering rate W for calcium in carbonate and silicate rocks can be written as a weighted sum of weathering rates (compare Eq. 2.8):

where the subscripts c and s denote carbonates and silicates, respectively, and x is the fraction of the total drainage area due to each rock type. Further refinement, such as inclusion of the individual dissolution rates of limestones, dolostones, and different types of silicate rocks, is not justified for this simple computation.
By using the ranges of carbonate and silicate dissolution rates from Figure 2.7, and the preceding estimates of the carbonate outcrop area and the percentage contributions of rock types, the weathering rate W is
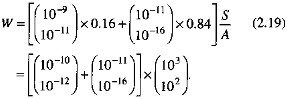
An upper bound of the weathering rate W from the preceding equation is 10-7, a value much greater than the mean chemical denudation rate of about 10-12 mol/cm2/s, shown in Figure 2.7 and Table 2.2.
In this computation, the same value of the S/A ratio was arbitrarily taken for the carbonate and silicate rocks. A more careful inspection of the numerical terms in the computation of W would show that the first term of the sum representing carbonate dissolution is the main contributor to the high value of W, which is further magnified by the surface-area ratio term S/A >>1. This contribution would have been reduced if the S/A value for rocks were small; for example, surface dissolution without water penetrating the rock is an approximation to dissolution of dense limestone or marble surfaces exposed to rain or running water (Reddy, 1988). However, theoretical and empirical estimates of the rock surface areas (S), the ratio S/A, and the volume of water flow through the rocks are such that regional or global estimates of the chemical denudation rates based on them have large margins of uncertainty.
NEUTRALIZATION OF ACIDITY BY WEATHERING RELEASES
Background — Alkalinity [Alk], Acidity [Acy], CO2, and pH
Observations of weathering in small drainage basins of streams and lakes exposed to acid rain, and experimental results from acid water leaching of soils in lysimeters show that variable, yet significant fractions of imported acidity, measured as [H]+, are neutralized by reactions of water with minerals (Likens et al., 1977; Henriksen, 1980; Wright, 1983, 1988; Folster, 1985; Paces, 1985; Colman and Dethier, 1986; Schnoor and Stumm, 1986; Berner and Berner, 1987; Drever, 1988; Norton et al., 1989; Velbel and Romero, 1989). These conclusions corroborate the older and broader ideas that crustal weathering is a chemical acid-base titration on a planetary scale (Sillen, 1961, p. 551; Stumm and Morgan, 1981; Holland, 1984).
Acidic streams and acidic lakes exist because neutralization reactions in some areas are not fast enough. Whenever waters react with carbonate and/or silicate rocks, consumption of the hydrogen ions from solution is balanced by releases of chemically equivalent amounts of other metal cations. The released cations may be transported away by flow or they may in part be stored in secondary minerals and vegetation of the drainage area, as discussed in an earlier section. More generally, H+ -sensitive reactions are those that produce changes in the alkalinity of the solution (function [Alk] defined below), and they result in concentration increases for cations derived from the reacting minerals.
One of the simpler definitions is that alkalinity is the acid neutralization capacity of a solution. A more specific definition is that alkalinity is the difference between the concentrations of the H+-independent cations and anions in solution. Extensive textbook-level discussions of alkalinity of natural waters are given by Stumm and Morgan (1981) and Drever (1988). The main ions in natural waters that are H+ dependent include the anions bicarbonate, carbonate, and hydroxyl. In certain continental waters, phosphate ions may also contribute to the alkalinity. In acidic waters, the positively charged aluminum hydroxide aqueous complexes add to the balance along with the hydrogen ion. Anions of organic acids may also be important in waters enriched in dissolved organic carbon.
In a simplified general case, alkalinity is the difference between the following anion and cation concentrations, given in equivalents per liter:

The preceding form of alkalinity, which is based on the carbonate, hydroxyl, and hydrogen ions, is also known as the carbonate alkalinity.
In acidic solutions, where concentrations of the bicarbonate, carbonate, and hydroxyl ions are negligibly low, the hydrogen ion is dominant and the values of [Alk] become negative. Thus for acidic solutions, the negative alkalinity is defined as acidity [Acy]:
-[Alk] = [Acy]. (2.21)
TABLE 2.4 Parameters Used in Computation of Alkalinity [Alk] (for more complete definitions of alkalinity, see text)
![]() |
Note: Small brackets [ ] denote concentration in moles per liter. SOURCE: Stumm and Morgan (1981). |
It should be noted that in the presence of other positively charged species, such as aluminum hydroxide complexes, the acidity would be greater than the hydrogen-ion concentration alone. The acidity values [Acy] are generally greater than indicated by the pH measurements in many natural acidic waters and human-produced discharges.
Alkalinity, as defined in Eq. (2.20), is a function of two variables: the pH and the total dissolved inorganic carbon in solution. Table 2.4 lists explicit equations for alkalinity and the auxiliary parameters defining it in terms of the hydrogen-ion concentration, and either total dissolved inorganic carbon (CT) or the CO2 pressure at equilibrium with the solution (PCO2).
During weathering, as hydrogen ions are consumed by reactions with minerals and cations are released into a solution open to the atmosphere, the alkalinity increases, and its increase is reflected in the higher concentrations of dissolved inorganic carbon (CO2, HCO3-, and CO32-). For a given partial pressure of CO2 at equilibrium with a solution, a higher pH corresponds to higher [Alk] values. This relationship holds for continental waters exposed to atmospheric CO2, as was documented for freshwater and saline lakes (Lerman and Stumm, 1989). A similar relationship between alkalinity and pH is shown in Figure 2.8.
For natural freshwaters approximated by dilute aqueous solutions, the two curves of [Alk] versus pH in Figure 2.8 represent present atmospheric CO2 and a partial pressure 10 times higher, approximating the values in soils near the plant-root zone. Waters remaining in contact with the bedrock longer and, possibly, developing higher levels of
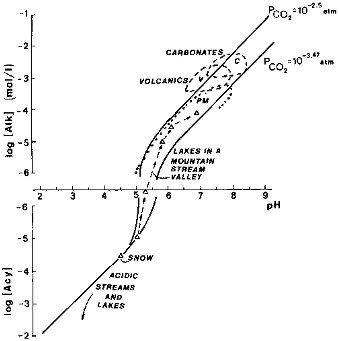
FIGURE 2.8 Alkalinity [Alk] and acidity [Acy] of waters open to an external CO2. NOTE: vertical scale near [Alk] = 0 interrupted for values between 10-6 and -10-6 moles/liter to allow for the logarithmic [Alk] scale. [Alk] values in this range are ≈0. —•— sequence of Alpine-valley lakes (Schnoor and Stumm, 1986). Acidic rivers and lakes (Berner and Berner, 1987; Norton et al., 1989). PM, V, C are rivers from plutonic and metamorphic, volcanic, and carbonate terrains (Meybeck, 1984).
biological productivity show a progressive increase in [Alk] and pH values: a sequence of small Alpine lakes represents a progression from acidic waters of the source stream emerging from a snow field through several lakes of lengthening water residence time, each occurring downstream of the preceding (Schnoor and Stumm, 1986). Concentrations of inorganic solutes in water emerging from snow fields and glaciers are generally very low (Raiswell, 1984).
On a physically larger scale, streams flowing through crystalline rock terrains have as a whole lower alkalinity and pH values than rivers draining volcanic rocks. Waters in carbonate drainage basins show the highest [Alk] and pH values of the three classes (Figure 2.8).
In acidic waters, up to a pH of about 5.5, that have not (or not yet) become neutralized by chemical weathering reactions, for all practical purposes an increase in dissolved CO2 does not affect the [Alk] or [Acy] value of the solution. In less acidic and near-neutral waters, at pH≥5.5, smaller changes in dissolved CO2 may more significantly affect the [Alk] and pH values of the solution. The range of dissolved CO2 values in rivers and freshwater lakes varies from some degree of undersaturation with atmospheric carbon dioxide to values higher than the saturation by a factor of about 3, corresponding to an equilibrium PCO2 = 1000 ppmv (Kempe, 1988). The higher PCO2 values in such rivers, computed from the carbonate- and hydrogen-ion equilibria, are associated with supersaturation of river waters with respect to calcite (CaCO 3), a phenomenon variably controlled by primary productivity or discharge of carbon dioxide-supersaturated waters from the subsurface (Holland, 1978, pp. 105-107; Kempe, 1988).
SUMMARIZING DISCUSSION
The weathering releases of dissolved materials from bedrock and soil to continental waters, viewed as a process of acid neutralization and mineral dissolution, are the basis of any conceptual model that ties the surficial transport fluxes into one interactive system. An increase in input of acidity to the bedrock should, in a steady state system, result in faster dissolution rates and greater amounts of solutes transported in flow. Because the residence time of surface runoff from the continents is short, months to years, the effects of higher dissolution rates might be expected to show in the runoff on time scales comparable to those of the runoff residence times. Although historical data on dissolved loads of streams and rivers in preindustrial times are difficult to come by, some modern analogues to the magnitudes of past changes in weathering are provided by chemical surveys of lakes over the past 40 to 60 yr.
Data on the pH of freshwater lakes on the Scandinavian shield, going back to late 1930s, indicate a continuous decrease in the lake water pH by about 1 pH unit in 25 yr. This suggests, as pointed out in an earlier section, that the rates of water-rock neutralization reactions do not always keep pace with an environmental change. Another set of historical records going back to the 1920s is the result of surveys of lake-water chemistry in a population of 145 lakes in Wisconsin and a number of lakes in the Adirondack Mountains of New York (Kramer et al., 1986): during the 50- to 60-yr period since the 1920s and 1930s, there have been very small changes in the mean values of alkalinity in the two regions. In the Wisconsin lakes, [Alk] has increased by about 0.04 x 10-3 eq/liter; in the Adirondack Mountains the change was a decrease by 0.04 X 10-3 to 0.07 X 10-3 eq/liter. These numbers, compared to the [Alk] values of >10-4 eq/liter shown in Figure 2.8, indicate a very small change over a half a century of accelerating acid-producing emissions.
Another longer-term effect may reflect the stabilizing capacity of the rock-soil-water system that tends to keep the weathering release rates nearly constant on time scales of 103 to 104 yr. The rates of soil formation on time scales of 103 to 106 yr, summarized by Brunsden (1979), range from 60 to 450 mm/1000 yr for soils in subtropical regions. Slower rates of formation characterize ferralites (15 to 45 mm/1000 yr) and duricrusts on granite (9 mm/1000 yr). These rates overlap the rates of chemical weathering given in Table 2.2. Thus, on time scales of 103 to 104 yr, changes in the rates of dissolution of the bedrock, which might have been caused by combinations of environmental and biological factors, could be in part taken up in a faster development of the residual soil profiles rather than only in higher solute concentrations in runoff.
One of the major trends of industrial times is an increase in the acidity of atmospheric precipitation caused by emissions of nitrogen and sulfur oxides from burning of fossil fuels. However, an additional component of potential acidity resides in the living and dead organic carbon on land. On a global scale, from tropical to cold climatic zones, carbon density in soils increases by a factor of about three, as shown in Figure 2.9. This trend runs opposite to the declines in net primary productivity and standing crop of the plant mass, from the tropics to the tundra, over a temperature range from about 25 to 5°C (Lieth, 1975; Post et al., 1982). The opposite trends of carbon storage in soils and in the living phytomass are shown in Figure 2.9 by the curve labeled carbon density ratio. The soil/biomass carbon ratio is generally higher in colder climates than in warmer. This trend also accounts for the longer residence times of carbon in colder soils, where net primary productivity is lower than in the tropics. The soil carbon is a potential source of additional acidity, both organic and inorganic, that in a warmer climate of the future may promote a faster development of the regolith and contribute to a greater dissolved load in riverine flow.
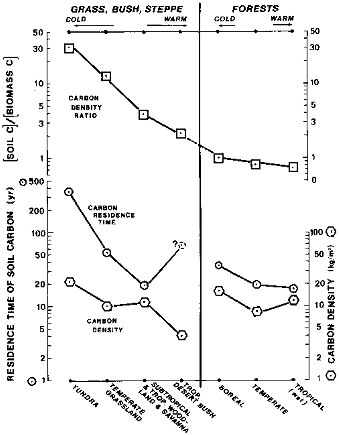
FIGURE 2.9 Condition of constant alkalinity as controlled by changes in PCO2 and pH, left ordinate (Eq. (2.25) and Table 2.4). Range of PCO2 for surficial waters and soil groundwaters, right ordinate.
In judging by the acidity or alkalinity values of surface land waters at the present time, newly formed acidity may be effectively neutralized in carbonate-mineral terrains, but it may persist for longer periods in waters that are in contact with crystalline silicate rocks.
ACKNOWLEDGMENTS
It is a pleasure to acknowledge James Drever's (University of Wyoming) careful review of an earlier manuscript and his helpful comments. John Walther (Northwestern University) made available to me his data on mineral dissolution rates, which at the time not been published. David Armstrong and Anders Andren (University of Wisconsin) brought to my attention the historical data on the waters of the Midwest and Adirondack mountains. In writing this chapter, I benefited from several opportunities to air some of the results at seminars in other institutions, and from discussions of the subject matter with Fred Mackenzie (University of Hawaii), Michel Meybeck (Universite Pierre et Marie Curie, Paris), and Bertrand Fritz (Centre de Geochimie de la Surface, CNRS, Strasbourg). This work was supported in part by NSF Grant EAR-9316133.
REFERENCES
Bales, R.C., and J.J. Morgan (1985). Dissolution kinetics of chrysotile at pH 7 to 10, Geochimica et Cosmochimica Acta 49, 2281-2288.
Barnola, J.M., D. Raynaud, Y.S. Korotkevich, and C. Lorius (1987). Vostok ice core provides 160,000-year record of atmosphere CO2, Nature 329, 408-414.
Barry, R.G. (1985). Snow cover, sea ice, and permafrost, in Glaciers, Ice Sheets and Sea Level: Effect of a CO2-Induced Climatic Change, Committee on Glaciology, National Academy Press, Washington, D.C., pp. 241-247.
Baumgartner, A., and E. Reichel (1975). The World Water Balance, Elsevier, Amsterdam, 179 pp.
Berner, E.K., and R.A. Berner (1987). The Global Water Cycle: Geochemistry and Environment, Prentice-Hall, Englewood Cliffs, N.J.
Berthelin, J. (1988). Microbial weathering processes in natural environments, in Physical and Chemical Weathering in Geochemical Cycles, A. Lerman and M. Meybeck, eds., Kluwer Academic, Dordrecht, pp. 33-60.
Blum, A., and A. Lasaga (1988). Role of surface speciation in the low-temperature dissolution of minerals, Nature 331, 431-433.
Brady, P.V. (1991). The effect of silicate weathering on global temperature and atmospheric CO2, Journal of Geophysical Research 96, 18101-18106.
Brady, P.V., and J.V. Walther (1989). Controls of silicate dissolution rates in neutral and basic pH solutions at 25°C. Geochimica et Cosmochimica Acta 53, 821-832.
Brunsden, D. (1979). Weathering, in Process in Geomorphology, C. Embleton and J. Thornes, eds., Annold, pp. 73-129.
Chou, L., and R. Wollast (1985). Steady-state kinetics and dissolution mechanisms of albite, American Journal of Science 285, 963-993.
Chou, L.R.M. Garrels, and R. Wollast (1989). Comparative study of the kinetics and mechanisms of dissolution of carbonate minerals, Chemical Geology.
Colman, S.M., and D.P. Dethier, eds. (1986). Rates of Chemical Weathering of Rocks and Minerals, Academic Press, New York.
Cronan, C.S. (1985). Chemical weathering and solution chemistry in acid forest soils: Differential influence of soil type, biotic processes, and H+, in The Chemistry of Weathering, J.I. Drever, ed., D. Reidel, Dordrecht, pp. 175-196.
Deike, R.G. (1989). Dolomite dissolution rates and possible Holocene dedolomitization of waterbearing units in Edwards Aquifer, south-central Texas, 28th International Geological Congress, Abstracts 1, 323.
Drever, J.I. (1988). The Geochemistry of Natural Waters, Prentice-Hall, Englewood Cliffs, N.J.
Eckhardt, F.E.W. (1985). Solubilization, transport, and deposition of mineral cations by microorganisms—efficient rock weathering agents, in The Chemistry of Weathering, J.I. Drever, ed., D. Reidel, Dordrecht, pp. 161-174.
Fölster, H. (1985). Proton consumption rates in Holocene and present-day weathering of acid forest soils, in The Chemistry of Weathering, J. I. Drever, ed., D. Reidel, Dordrecht, pp. 197-210.
Garrels, R.M., and F.T. MacKenzie (1971). Evolution of Sedimentary Rocks, W.W. Norton & Co., New York.
Goldberg, E.D. (1971). Atmospheric dust, the sedimentary cycle and man, Geophysics 1, 117-132.
Henriksen, A. (1980). Acidification of fresh waters — A large scale titration, in Ecological Impact of Acid Precipitation, D. Drablos and A. Tollan, eds., SNSF Project, Oslo-As, pp. 68-74.
Herdendorf, C.E. (1982). Large lakes of the world, Journal of Great Lakes Research 8(3), 379-412.
Holland, H.D. (1978). The Chemistry of the Atmosphere and Oceans, John Wiley & Sons, New York.
Holland, H.D. (1984). The Chemical Evolution of the Atmosphere and Oceans, Princeton University Press, Princeton, N.J.
Jouzel, J., C. Lorius, J.R. Petit, and others (1987). Vostok ice core: A continuous isotope temperature record over the last climatic cycle (160,000 years), Nature 329, 403-408.
Kempe, S. (1988). Freshwater carbon and the weathering cycle, in Physical and Chemical Weathering in Geochemical Cycles, A. Lerman and M. Meybeck, eds., Kluwer Academic Publishers, Dordrecht, pp. 197-224.
Kramer, J.R., A.W. Andren, R.A. Smith, A.H. Johnson, R.B. Alexander, and G. Oehlert (1986). Streams and lakes, in Acid Deposition: Long-Term Trends, National Academy Press, Washington, D.C., pp. 231-299.
Lerman, A. (1979). Geochemical Processes — Water and Sediment Environment, John Wiley & Sons, New York.
Lerman, A., and A.B. Hull (1987). Background aspects of lake restoration: Water balance, heavy metal content, phosphorus homeostasis, Schweiz Zeitschrift Hydrology 49, 148-169.
Lerman, A., and W. Stumm (1989). CO2 storage and alkalinity trends in lakes, Water Research 23(2), 139-146.
Lieth, H. (1975). Modeling the primary productivity of the world, in Primary Productivity of the Biosphere, H. Lieth and R.H. Whittaker, eds., Springer, New York, pp. 237-263.
Likens, G.E., F.H. Bormann, R.S. Pierce, J.S. Eaton, and N.M. Johnson (1977). Biogeochemistry of a Forested Ecosystem, Springer-Verlag, New York, 146 pp.
Lorius, C., J. Jouzel, C. Ritz, L. Merlivat, N.I. Barkov, Y.S. Korotkevich, and V.M. Kotlyakov (1985). A 150,000-year climatic record from Antarctic ice, Nature 316, 591-596.
Meybeck, M. (1984). Les fleuves et le cycle geochimique des Elements, These d'etat (no. 84-35), Ecole Normal Superieure, Laboratoire de Geologie, Universite Pierre et Marie Curie, Paris, France.
Murphy, W.M., E.H. Oeklers, and P.C. Lichtner (1989). Surface reaction versus diffusion control of mineral dissolution and growth rates in geochemical processes, Chemical Geology 78, 357-380.
National Geographic Society (1988). Vegetation cover of the world 18,000 b.p., on the map Endangered Earth, National Geographic Society, Washington, D.C.
Norton, S.A., F.H. Howd, C.V. Guidotti, T.A. Haines, and R.B. Davis (1989). Influence of Conway Granite (Triassic?) in New Hampshire on biogeochemical response to acidic precipitation, 28th International Geological Congress, Abstracts 2, 524.
Paces, T. (1985). Sources of acidification in Central Europe estimated from elemental budgets in small basins, Nature 315, 31-36.
Plummer, L.N., T.M.L. Wigley, and D.L. Parkhurst (1978). The kinetics of calcite dissolution in CO2-water systems at 5° to 60°C and 0.0 to 1.0 atm CO2, American Journal of Science 278, 179-216.
Post, W.M., W.R. Emmanuel, P.J. Zinke, and A.G. Stangenberger (1982). Soil carbon pools and world life zones, Nature 298, 156-159.
Probst A., E. Dambrine, D. Viville, and B. Fritz (1990). Influence of acid atmospheric inputs on surface water chemistry and mineral fluxes in a declining spruce stand within a small granitic catchment (Vosages Massif, France), Journal of Hydrology 116, 101-124.
Probst A., D. Viville, B. Fritz, B. Ambroise, and E. Dambrine (1992). Hydrochemical budgets of a small forested granitic catchment exposed to acid deposition: The Strengbach catchment case study (Vosages Massif, France), Water, Air and Soil Pollution 62, 337-347.
Raiswell, R. (1984). Chemical models of solute acquisition in glacial meltwaters, Journal of Glaciology 30, 49-57.
Reddy, M.M. (1988). Acid rain damage to carbonate stone: A quantitative assessment based on the aqueous geochemistry of rainfall runoff from stone, Earth Surface Processes and Landforms 13, 335-354.
Rimstidt, J.D., and H.L. Barnes (1980). The kinetics of silica-water reactions, Geochimica et Cosmochimica Acta 44, 1683-1700.
Schneider, S. H., and W.W. Kellogg (1973). The chemical basis for climate change, in Chemistry of the Lower Atmosphere, S. I. Rasool, ed., Plenum, New York, pp. 203-249.
Schnoor, J.L., and W. Stumm (1986). The role of chemical weathering in the neutralization of acidic deposition, Schweiz Annals of Hydrology 48, 171-195.
Schott, J., and R.A. Berner (1985). Dissolution mechanisms of pyroxenes and olivines during weathering, in The Chemistry of Weathering, J.I. Drever, ed., D. Reidel, Dordrecht, pp. 35-54.
Schott, J., and J.C. Petit (1987). New evidence for the mechanism of dissolution of silicate minerals, in Aquatic Surface Chemistry, W. Stumm, ed., John Wiley & Sons, New York, pp. 293-315.
Schott, J., R.A. Berner, and E.L. Sjoeberg (1981). Mechanism of pyroxene and amphibole weathering—I. Experimental studies of iron-free minerals, Geochimica et Cosmochimica Acta 45, 2123-2135.
Siegenthaler, U., and H. Oeschger (1987). Biospheric CO2 emissions during the past 200 years reconstructed by deconvolution of ice core data, Tellus 39B, 140-154.
Sillen, L.G. (1961). The physical chemistry of sea water, in Oceanography, M. Sears, ed., American Association for the Advancement of Science, Washington, D.C., Pub. No. 67, pp. 549-581.
Sposito, G. (1985). Chemical models of weathering in soils, in The Chemistry of Weathering, J.I. Drever, ed., D. Reidel, Dordrecht, pp. 1-18.
Stallard, R.F. (1988). Weathering and erosion in the humid tropics, in Physical and Chemical Weathering in Geochemical Cycles, A. Lerman and M. Meybeck, eds., Kluwer Academic, Dordrecht, pp. 225-246.
Stallard, R.F. (1989). Weathering processes and chemical composition of tropical rivers from diverse tectonic settings , 28th International Geological Congress, Abstracts 3, 169.
Stumm, W., and J.J. Morgan (1981). Aquatic Chemistry, John Wiley & Sons, New York.
Tole, M.P., A.C. Lasaga, C. Pantano, and W.B. White (1986). Factors controlling the kinetics of nepheline dissolution, Geochimica et Cosmochimica Acta 50, 379-392.
Van Name, R.G. (1929). The velocity of dissolving of crystals in liquids, in International Critical Tables 5, McGraw-Hill, New York, pp. 55-60
Velbel, M.A. (1985). Geochemical mass balance and weathering rates in forested watersheds of the southern Blue Ridge, American Journal of Science 285, 904-930.
Velbel, M.A., and N.L. Romero (1989). Amphibolite weathering and cation budgets in southern Blue Ridge and Colorado Front Range, USA, 28th International Geological Congress, Abstracts 3, 291.
White, A.F., and M.L. Peterson (1986). Role of reactive-surface-area characterization in geochemical kinetic models, in Geochemical Processes at Mineral Surfaces, J.A. Davis and K.F. Hays, eds., American Chemical Society Symposium Series 323, pp. 461-477.
Whitfield, M. (1974). Accumulation of fossil CO2 in the atmosphere and the sea, Nature 247, 523-525.
Wollast, R., and L. Chou (1988). Rate control of weathering of silicate minerals at room temperature and pressure, in Physical and Chemical Weathering in Geochemical Cycles, A. Lerman and M. Meybeck, eds., Kluwer Academic, Dordrecht, pp. 11-32.
Wollast, R., and F.T. Mackenzie (1983). The global cycle of silica, in Silicon Geochemistry and Biogeochemistry, S.R. Aston, ed., Academic Press, New York, pp. 39-76.
Wright, R.F. (1983). Acidification of freshwaters in Europe, Water Quality Bulletin 8, 137-142.
Wright, R.F. (1988). Influence of acid rain on weathering rates, in Physical and Chemical Weathering in Geochemical Cycles, A. Lerman and M. Meybeck, eds., Kluwer Academic, Dordrecht, pp. 181-196.