3
Global Chemical Weathering on Glacial Time Scales
LEE R. KUMP AND RICHARD B. ALLEY
Pennsylvania State University
INTRODUCTION
Scoured valleys, jagged peaks, expansive moraines, and thick deposits of till bear firm testament to the erosive power of glaciers. The effects of glaciers on chemical denudation rates are less visible. The products of chemical weathering are either dissolved solutes, which are efficiently transported to the sea, or clays, which thicken the soil mantle or are transported away by streams.
Intuition gives little extra information on changes in chemical weathering on glacial time scales. A number of environmental variables influence chemical weathering rates globally. Many of these are studied in the laboratory: temperature, chemistry of the dissolving fluid, surface area, fluid transport rates. However, many others are peculiar to the natural setting. In calculating global chemical weathering rates one typically measures the composition and discharge of the world's major rivers (Meybeck, Chapter 4, this volume). Each of these rivers represents a mixture of contributions from different lithologies and climatic and tectonic regimes. The flux of dissolved material from a given river then depends on the geographical distribution of lithologies relative to climate patterns, rates of tectonic activity (uplift), vegetational zonations, and heterogeneities in the intensity of physical weathering processes that produce reactive materials. Thus, to scale up from laboratory measurements to calculations of global weathering rates requires much additional information on environmental conditions (Lerman, Chapter 2, this volume).
It is only after we have determined how these factors have varied that we will be able to make an estimate of the changes in global chemical weathering rates on glacial time scales. Our initial interpretations may be wrong. For example, cooler temperatures and the coverage by glaciers of a large portion of the land surface might suggest that chemical weathering rates were lower during glaciations (e.g., Broecker, 1983). However, other factors may have more than compensated for these changes: the production of fine, easily weathered material by glaciers and the increase in land area due to eustatic sea-level fall.
This chapter is a summary of what is presently known about variations in chemical weathering variables on glacial time scales, with a focus on the time period from 18 ka (1000 yr before present) to today. We begin with a discussion of climate change, in terms of both global and regional changes in temperature, precipitation, evaporation, and runoff. Both empirical data and modeling studies are considered. Next we assess the areal changes in rock exposure due to coverage by glaciers, sea-level fall, and the geographic distribution of rock types. The production and transport of fine-grained material by glaciers is then considered as an important variable in global weathering, followed by an assessment of the role of subglacial chemical weathering in the global scheme.
Because these factors are changing simultaneously on glacial time scales, it is important that we consider their combined effects on chemical weathering. This we do in the next section by speculating on weathering feedbacks with vegetation, the transport of fine-grained material across climatic zones, the coverage of key rock types by glaciers, and the effect of latitudinally specific land area changes. We then close with a summary and a discussion of the implications of these effects for glacial/interglacial changes in oceanic and atmospheric chemistry, and climate. Uncertainties in our knowledge of many important parameters are sufficiently large that we can draw few firm conclusions, but we hope to have identified important areas for further research.
FACTORS THAT INFLUENCE GLOBAL CHEMICAL WEATHERING
Climate
A large body of observational and model evidence is available on differences between the climate of the Wisconsinan glacial maximum of about 18 ka and the modern climate. Convergence of results from different techniques allows some comparisons to be made with confidence (e.g., CLIMAP, 1976; Manabe and Broccoli, 1985; Kutzbach and Guetter, 1986; Rind, 1987; COHMAP, 1988). Globally, these include (where trends are known more accurately than absolute changes) the following:
-
the ice-age world was a few degrees colder than today. Ice-age cooling was smallest in the tropics (≈ to 5°C), increased to the poles (≈ 5 to 10°C) and was quite large on the midlatitude ice sheets (tens of degrees Celsius);
-
lower ice-age temperatures reduced both precipitation and evaporation below modern values (by about 10 percent); and
-
any changes in globally averaged soil moisture were smaller than the uncertainties in our knowledge.
Regionally, during the ice age (Figure 3.1),
-
soil moisture was significantly less than today in a large area of Eurasia south and east of the Fennoscandian ice sheet, and in a smaller area of North American south of the Laurentide ice sheet. These drier areas probably developed because of the combined effects of anticyclonic circulation over the ice sheets and of the ice sheets acting as cold traps for water vapor and reducing precipitation nearby;
-
the southwestern United States was significantly wetter than today, probably owing to a southward shift in storm tracks caused by the topography of the Laurentide ice sheet;
-
other regional changes occurred: soil moisture was probably reduced in equatorial regions, causing reduction in extent of ice-age tropical rain forests, but increased in some areas at higher southern latitudes; and
-
precipitation over ice sheets was reduced substantially compared to modern precipitation in the previously glaciated regions.
The evolution from ice-age to modern climates was not monotonic (Figure 3.2). In particular, a peak in Northern Hemisphere summer insolation around 9 ka caused strengthened monsoonal circulation and large soil-moisture increases compared to today and to the glacial maximum across the northern monsoon belt (primarily Africa and Asia), with decreased monsoonal circulation and soil moisture over smaller areas of land in the Southern Hemisphere (Kutzbach and Guetter, 1986; COHMAP, 1988).
SOIL MOISTURE CHANGES
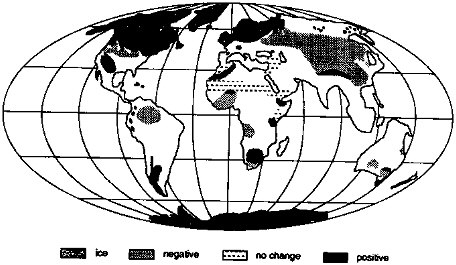
FIGURE 3.1 Changes in soil moisture, 18 ka relative to today. Areas marked "positive" were wetter 18 ka than today. Maximum extent of glacier ice during the last glacial episode is also shown (Kutzbach and Guetter, 1988; COHMAP, 1988; ice extent from Broecker, 1983).
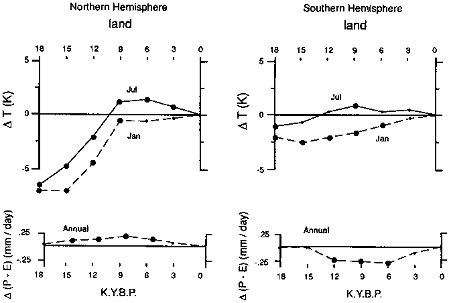
FIGURE 3.2 Seasonal temperature and mean-annual net precipitation [∆(P-E)] changes in the (a) Northern and (b) Southern Hemispheres, calculated by a general circulation/climate model, for the period 18 ka to present [abscissa in thousands of years before present (K.Y.B.P. = ka); from Kutzbach and Guetter, 1988].
Erosional Area
Glacial Coverage At the height of the last glaciation, vast areas of North American and Europe were covered with thick sheets of glacier ice (Figure 3.1). In addition, expanded mountain glaciers at this time covered smaller areas of Eurasia, North America, South America, Africa, and New Zealand. In sum, about 30 percent of the present land area was glaciated 18 ka (Flint, 1971).
Most of the areas covered were poleward of 40°N, with most of the rest being poleward of 40°S. Mountain glaciers expanded at all latitudes, especially in North America, South America, and Africa, and despite limited areal extent might have been important in affecting global chemical weathering rates.
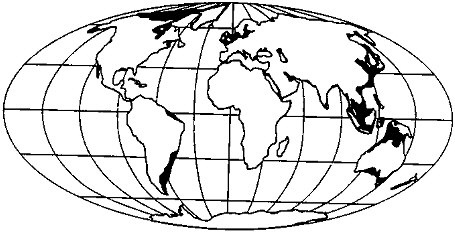
FIGURE 3.3 Areas of subaerial shelf exposure at 18 ka (by assuming a 130-m sea-level lowstand).
Shelf Exposure As the Wisconsinan ice sheets grew, sea level fell, progressively exposing more and more of the continental shelf region (Figure 3.3), which then became subject to glaciation at high latitudes and chemical weathering processes at lower latitudes. At full exposure, the continental shelves represent about 13 percent of the total land area of the planet; the majority of this was exposed at the glacial maximum. Interestingly, this ''new land" would have appeared preferentially in the tropics; approximately 40 percent of the total shelf area falls between 20° north and south of the equator (Figure 3.4).
Some of the more important shelf emergences include the Amazon and southeastern South American shelves, the perimeter of the Gulf of Mexico, and a large area of southwestern Asia and Oceania. Today, land areas adjacent to
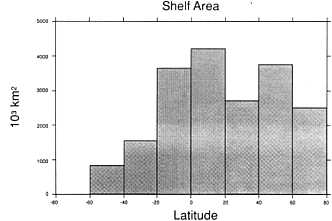
FIGURE 3.4 Shelf area as a function of latitude (South Pole is -90°; Rand McNally, 1977).
these shelves experience intense chemical weathering; such weathering may have occurred on the expanded land areas at the last glacial maximum. If so, then global chemical weathering rates actually could have been higher during the glacial period, although one must consider climate change as well.
Rock Types Exposed
A quick glance at a geological map confirms that the distributions of rock types exposed on the surface of the Earth is nonuniform. Nevertheless, these heterogeneities are typically ignored in models of geochemical cycles (e.g., Berner et al., 1983). In considering chemical weathering changes on glacial time scales, however, it is of interest to determine the changes in the areal distribution of rock types that occurred as a result of coverage by glaciers and the relationship of these changes to climate change.
The most important rock types in terms of their contributions to the global chemical weathering flux are limestones and rocks containing silicate minerals (shales and igneous and metamorphic rocks). Limestones are important because they dissolve easily during attack by groundwater; the streams with the highest dissolved loads (excluding those weathering evaporites subject to intense evaporation) are those passing through carbonate terrains (Gibbs, 1970; Holland, 1978; Berner and Berner, 1987). Silicate rocks are important because during their weathering (Eq. 3.1) CO2, undergoes net consumption:
Weathering reactions (on land)

Precipitation reactions (in ocean)

Net CO2 consumption (Eq. 3.1 + Eq. 3.3 + Eq. 3.4)

Although CO2 is consumed during carbonate weathering (Eq. 3.2), there is no net consumption on the time scale that the oceanic CaCO 3 budget is achieved (thousands of years; Broecker and Peng, 1987). The ocean is saturated with respect to CaCO3, so the products of carbonate weathering precipitate on reaching the sea (Eq. 3.3).
The present distribution of limestone is shown in Figure 3.5 (after Snead, 1980). This common rock type appears on all continents. There is a general lack of exposure of carbonates at high northern latitudes: thus, their
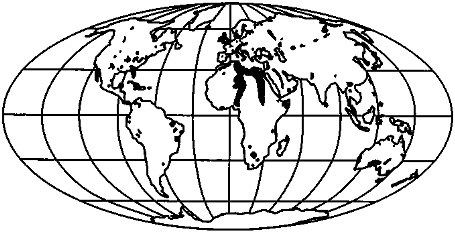
FIGURE 3.5 Major limestone exposures of the world (Snead, 1980).
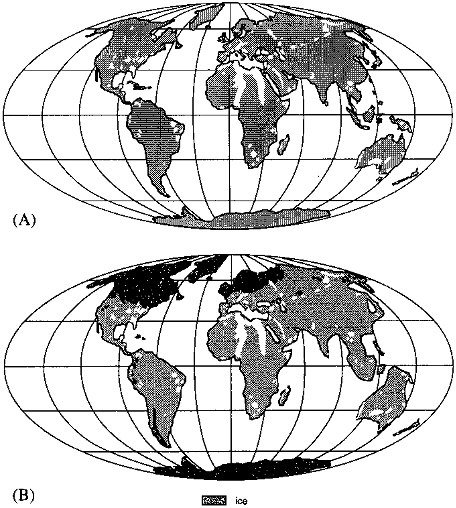
FIGURE 3.6 Noncarbonate exposures of the world (see Figure 3.5): (A) today and (B) at 18 ka.
outcrop areas were not significantly affected by the last glaciation. The glaciation of Europe is probably most significant, because chemical denudation there is most influenced by carbonate weathering. Yet even on this continent, most of the exposures are equatorward of the furthest extent of ice during the last glaciation. We must also consider the increase in limestone exposures due to sea-level fall; much of the increase in land area at low latitudes is the result of the exposure of carbonate platforms (Florida, Yucatan, Bahamas, northwest Australian shelf, Oceania). The presence of Pleistocene sinkholes and other karst features attests to the extensive dissolution of these limestones and the deep groundwater circulation during glaciation (e.g., Bloom, 1978, p. 146).
In contrast to its effect on carbonate exposure, glaciation caused a significant reduction in the area of exposure of silicate rocks (Figure 3.6). (All nonlimestone exposures are considered as "silicate" rocks.) The expansive shield region of North America was completely covered, as were the exposures in Scandinavia and Great Britain. Although one may thus conclude that the potential for CO2 consumption was reduced by glaciation, an accurate assessment of the overall effect depends on the corresponding change in climate on regional scales. We will consider this coupled change below.
Glacier Weathering and Erosion Processes
Temperature and Water Production Glaciers and ice sheets are among the most complex and variable systems on Earth. The most important factor controlling processes at glacier beds is temperature. A glacier frozen to a rigid bed does little or no geomorphic work (e.g., Gellatly et al., 1988); it halts the action of subaerial weathering processes and reduces erosion rates essentially to zero. Onset of basal melting facilitates glacial erosion, at typical rates of 1 mm/yr (Boulton, 1979) and extreme rates as high as 10 mm/day for short periods over restricted areas (Humphrey, 1986).
The controls on basal temperature are not simple. Temperate glaciers (those entirely at the pressure melting point) commonly form in regions of high snowfall with mean annual temperatures near or even above freezing (Porter, 1977), especially in maritime climates. Farther inland, glacier existence usually requires mean annual air temperatures and near-surface ice temperatures below freezing over at least part of the glacier (the accumulation zone). Heat then is supplied to the glacier bed by geothermal flow and by viscous dissipation ("friction") of ice flow. Heat is removed by upward conduction through the ice to its cold surface; the rate of conduction is affected by vertical and lateral ice flow. The balance of these processes determines the basal temperature (see review in Paterson, 1981, Ch. 10).
Consider first the central region of a large ice sheet, such as the Laurentide, Fennoscandian, or the modern Antarctic and Greenland ice sheets. Near the center, flow velocities are low and viscous dissipation is small compared to geothermal heating. When the ice sheet is sufficiently large and thick (more than a few hundred kilometers in diameter, >1000 m thick) to affect atmospheric circulation significantly, surface temperature and accumulation will decrease with increasing ice-sheet elevation. Surface air temperature will decrease approximately at the atmospheric lapse rate (≈1° C/100 m). Precipitation usually will be limited by the ability of the cold air to transport water vapor onto the ice sheet; in modern East Antarctica, accumulation varies exponentially with temperature (Robin, 1977).
In the absence of ice flow, the insulating ability of ice would cause the difference between bed and surface temperatures to increase by about 2° C/100 m of ice thickness, whereas surface temperatures cool only about 1° C/100 m; the result would be rapid bed warming with increase of ice thickness and thawed beds beneath all but the thinnest glaciers. However, flow processes cause surface ice to move down into an ice sheet in accumulation areas. (Ice spreads and thins under the gravitational stresses caused by its weight and surface slope; new snowfall balances this thinning in a steady ice sheet and exceeds it in a growing ice sheet.) This ice motion advects the surface cold downward and chills the bed.
This vertical advection effect scales approximately with (b/h)1/2, where b is accumulation rate and h is ice thickness (Robin, 1955). A thin, high-accumulation ice sheet has a cold bed in the central regions, but its behavior becomes more like that of a stagnant ice layer as the ice thickness increases and the accumulation rate drops. For likely conditions in the center of a continental ice sheet initiated in a subfreezing climate, the bed will remain frozen until the ice thickness exceeds ≈ 3000 m.
The 3500-m-thick East Antarctic ice sheet is underlain by a wet bed in places (Oswald and Robin, 1973) but almost as great a thickness of ice in Greenland shows widespread freezing to its bed (Radok et al., 1982; Robin, 1983). The bed of the West Antarctic ice sheet is largely thawed; however, it occurs in a recently active tectonic region, which probably has high geothermal flux (Alley and Bentley, 1988), and rests on low bedrock (typically 500 to 2000 m below sea level; Drewry, 1983), allowing thick ice to have a low, warm surface. By simple analogy and by physical reasoning, it is likely that the Laurentide and Fennoscandian ice sheets were frozen to their beds in central regions until their sizes increased close to their maximum values (Hooke, 1977; Sugden, 1977).
If basal melting occurs beneath the central part of an ice sheet, it will be slow. A typical geothermal flux will melt about 5 mm/yr of ice in the absence of heat conduction into the ice; beneath cold ice, a melt rate <1 mm/yr will be more typical.
An ice sheet thins away from its center, which from the discussion above would tend to cool the bed, and colder ice formed at higher elevation is transported to lower regions with higher surface temperatures by ice flow, also cooling the bed. However, flow velocities and viscous dissipation increase outward, tending to warm the bed. Local details of bed topography, geothermal flux, accumulation rate, and other factors are likely to be more important in controlling basal temperature than any "typical" variation along flow, although zones of net basal freeze-on of meltwater (e.g., Byrd Station in West Antarctica; Gow et al., 1979) or frozen bed will occur downstream of thawed regions in many cases.
At some point near the ice-sheet margin, flow tends to funnel into fast-moving, often relatively thin ice streams or outlet glaciers. These channelized flows may occur between regions of bare rock outcrop, but often occur between thin ice ridges nourished by local accumulation but not by flow from upstream. Viscous dissipation in ice streams is strong, typically melting O(10 to 100 mm/yr) [O(x) signifies "of the order of magnitude of x"]; interstream ridges are likely to be frozen to their beds. This situation can give rise to selective linear erosion, with the ice stream carving a channel between ridges experiencing little or no erosion (Sugden, 1978).
Surface melting on ice sheets is limited to warm areas at lower elevations and latitudes. Following the onset of melting, meltwater initially drains into surface snow and refreezes. Sufficient melting will cause surface runoff. Such runoff can drain to the bed if the ice is sufficiently thin, warm, and crevassed. At present, surface melting is common and supplies water to the bed on many mountain glaciers, but this is restricted to a relatively narrow band around the coast of Greenland and is almost absent in Antarctica. Where surface melting occurs it generally dominates the glacier water budget; rates of O(1 m/yr) are common.
Glacial-geologic evidence from the Laurentide and Fennoscandian ice sheets indicates the presence of frozen beds, wet beds, and surficial meltwater at various times (e.g., Sugden and John, 1976); however, most of the data reflect processes near the ice margin during retreat. A likely history is that large areas of the Wisconsinan midlatitude ice sheets were frozen to their beds during growth. The bed probably thawed in central regions during the glacial maximum. Thawed beds existed beneath channelized ice flows across large areas of the southern margins and smaller areas of the northern margins, but frozen beds occurred between these channelized flows. Surficial meltwater reached the bed in large quantities in marginal areas, especially on the southern margin during ice-sheet retreat. (For a similar but more exhaustive review of much of this material, the interested reader is referred to Hooke, 1977; Sugden, 1977; and Hughes, 1981).
Carbon Dioxide in Glacial Meltwater The amount of CO2 available in ice for chemical weathering can be estimated reasonably well and is not large (e.g., Stauffer and Berner, 1978; Neftel et al., 1982; Oeschger and Stauffer, 1986). During the transformation of snow to ice without melting, the ice traps about 10 percent air by volume with the prevailing atmospheric CO2 concentration. This is retained in the air bubbles as CO2 (or in some form such that CO2 gas is recovered if the ice is crushed under vacuum), for a total of about 10-6 mole CO2/kg ice (CO2:H2O molar ratio ≈ 2 x 10-8, within a factor of two to three depending on atmospheric CO2 partial pressure, atmospheric pressure when air was trapped, and other variables). By comparison, rainwater in equilibrium with the atmosphere has a CO2 concentration an order of magnitude or more higher than this. Refrozen surficial meltwater in glaciers falls between the ordinary ice and rainwater CO2 concentrations.
When ice is melted beneath a glacier, water production rates are low, flow velocities are slow, and flow paths to the ice front typically are long; thus, it is likely that chemical weathering continues to completion and consumes the CO2 in the meltwater. Wet-based glaciers are reasonably efficient at eroding unconsolidated sediment in some areas and depositing it elsewhere (see below), so it is unlikely that organic-rich soils will remain in contact with a glacier and supply abundant CO2 to meltwaters over any significant time.
Under certain circumstances, surficial meltwater can remain in contact with both rock debris and atmospheric CO2. For example, compressive stresses near ice fronts may cause folding or thrusting of basal debris to the ablating ice surface. Under such open-system conditions, meltwater can obtain CO2 from the air as it is consumed in weathering; total CO2 consumption then can exceed initial CO2 content of the water (Raiswell, 1984). This process continues beyond the glacial margin in proglacial streams and differs from nonglacial streams only in that (1) glacial streams often transport material with more total area of fresh, unweathered mineral surface than similar nonglacial streams (see below); but (2) glacial streams generally lack CO2 input from organic-rich soils and thus have much lower CO2 concentrations than equivalent nonglacial streams.
Observations bearing directly on glacial chemical weathering are relatively scarce. Edmond (1973) and Hurd (1977) showed that Antarctic glaciers contribute very little to the dissolved silica in ocean waters adjacent to the Antarctic. Mountain glaciers are observed to have two meltwater systems: one with slow drainage and limited CO2 in which chemical weathering proceeds to equilibrium and solute concentrations are relatively high, and one with rapid, channelized drainage of surficial water in which solute concentrations are low, whether that drainage occurs on, in, or under the glacier (Collins, 1979; Raiswell, 1984). Proglacial mixing of these waters allows the CO2- bearing surficial meltwaters to weather silt supplied by the basal waters, but the extent to which equilibrium is reached and further CO2 is taken from the air is not known well. Ford (1971) found that glacial meltwater above tree line in limestone regions of the Canadian Rockies was saturated with respect to CaCO3 at only 50 to 90 mg/liter, compared to groundwater saturation at 100 to 265 mg/liter below tree line and creek and lake saturation at 100 to 140 mg/liter below tree line.
Glaciated basins in high mountains in maritime climates have high denudation rates (= 1 mm/yr) and chemical weathering is faster than the global average, but runoff is very high (4 m/yr is typical; Corbel, 1959; Reynolds and Johnson, 1972). No data are available on how much of that chemical weathering is associated with soil processes in unglaciated parts of the basins; the work of Ford (1971) suggests that much of it may be. In addition, glaciers in such maritime climates often have abundant englacial and supraglacial debris from rockfalls along with abundant meltwater; thus they are poor analogues for all except the narrow margin of a continental ice sheet.
Based on this discussion, chemical weathering in contact with continental ice sheets can be summarized as follows:
-
large areas of ice sheets are frozen to their beds and slow or stop chemical weathering, especially during ice-sheet growth;
-
central ice-sheet regions with thawed beds produce <O(1 mm/yr) basal meltwater, with CO2 available for weathering equivalent to that in <O(0.1 mm/yr) rainwater, and this CO2 generally is consumed in rock weathering;
-
fast-moving marginal regions produce O(10 to 100 mm/yr) basal meltwater over perhaps 10 percent of the ice
-
sheet, with CO2 equivalent to O(1 to 10 mm/yr) rainwater over this area, and this CO2 also is consumed in rock weathering;
-
ablation zones along ice-sheet margins may produce O(1 m/yr) surficial meltwater with CO2 concentration between that of ice and rainwater, and more atmospheric CO2 is available to this open system; however, high flow velocities and scarcity of supraglacial debris generally prevent rapid chemical weathering; and
-
glacial meltwater generally does not gain significant CO2 from soil processes, whereas soil processes in unglaciated regions often increase CO2 by an order of magnitude or more from rainwater values (Cawley et al., 1969). (In this respect, it is worth noting that much of the glacial discharge from the southern margins of the Laurentide and Fennoscandian ice sheets drained into climatically dry regions caused by the ice sheets, where soil processes producing CO2 may have been slowed compared to more humid regions.)
Taken together, these points suggest that continental ice sheets reduce chemical weathering significantly—probably by one or more orders of magnitude—compared to preglacial or postglacial rates in the glaciated regions. However, some marginal regions of continental ice sheets occupy areas that are marine during interglaciations, so local increases of chemical weathering can accompany glaciation.
Erosion Rates Although it seems likely that ice-sheet glaciation reduces chemical weathering in the glaciated region, there is no general agreement on the effect on physical weathering and erosion rates. This question is of interest because fresh, reactive mineral surfaces produced subglacially possibly undergo enhanced chemical weathering beyond glacier margins.
Subglacial erosion rates span the entire range of terrestrial values, as noted above. Glacier erosion involves three major processes: plucking, abrasion, and subglacial fluvial action (e.g., Sugden and John, 1976, part III). Plucking is the glacial removal of clasts from the bed. Plucking is most effective where freeze-thaw processes cause ice to attach to previously jointed rock on the downstream sides of bumps in the bed, so that the ice motion pulls blocks free. Neither freezing-on nor preexisting bumps are absolutely necessary for plucking, but glacial stresses are sufficiently small that preexisting jointing or unconsolidated sediments are necessary for plucking. However, a glacier may contribute to the formation of joints through freeze-thaw processes, fluctuating water pressures, or erosion causing formation of unloading joints (sheeting).
Abrasion is the process by which glacially transported clasts scratch and gouge other clasts or subglacial bedrock, wearing away contact points and typically forming silt-sized particles. In the classical theory of abrasion (Hallet, 1979, 1981), the rate of abrasion of bedrock caused by a clast in basal ice increases with the clast velocity over the bed and the clast force on the bed. The clast force assumes that value needed to shove the clast upward into the softer, plastically deforming ice at the rate at which surrounding ice in contact with the bedrock is melted away or strained downward. Observations also show that clast-clast interactions in deforming subglacial till (see below) cause rapid abrasion and silt generation (Boulton et al., 1974), and that abrasion of bedrock occurs beneath deforming till (MacClintock and Dreimanis, 1964). Abrasion requires a source of clasts (from plucking, supraglacial sources, or reworking of preexisting unconsolidated sediments) because the abrading clasts are themselves abraded and must be replaced.
Erosion by subglacial streams is similar to that by proglacial streams. It is limited primarily to marginal regions where abundant channelized surficial meltwater reaches the bed, but it can be quite active there (e.g., Sugden and John, 1976, Ch. 15).
Glacial shear stresses can deform unconsolidated subglacial sediments. Such sediments may be created by other subglacial processes of erosion, or the glacier may advance over preglacial or proglacial sediments. Subglacial deformation of unconsolidated sediments can be a very efficient mechanism of erosion/transport (Boulton, 1979; Alley et al., 1989), moving far more material than other subglacial processes of erosion. Glaciers thus erode unconsolidated materials more efficiently than they erode bedrock.
Direct glacial deposits (tills) contain all grain sizes but exhibit modal peaks in the silt range (abrasion) and in the pebble-cobble range (plucking). Glaciofluvial deposits are sorted according to fluvial processes.
Much debate has centered on the depth of erosion achieved by the Pleistocene ice sheets of northern midlatitudes, especially the Laurentide ice sheet (e.g., White, 1972; Gravenor, 1975; Sugden, 1978; Bell and Laine, 1985). Estimates vary by an order of magnitude.
Based on the volume of sediments on land and in marine basins around the Laurentide region, Bell and Laine (1985) estimated denudation of at least 120 m (and perhaps as much as 200 m) over the past 3 million years (m.y.) during which major Northern Hemisphere continental glaciation occurred. Glacial sediments on land account for only one-fifteenth of the total. They further estimated that fluvial processes in the absence of glaciation would have produced little more than 10 percent of this sediment. Based on these estimates, the glacial erosion rate over the entire Laurentide region and the entire 3 m.y. is only about 0.05 mm/yr. However, glaciers were absent for some periods, and were frozen to their beds or depositing rather than eroding in some areas when present.
If we speculate that wet-based glaciation occupied only one-quarter of the Laurentide region for one-quarter of the glacial period (possible but unproven numbers), then the wet-based erosion rate was about 1 mm/yr, similar to mountain-glacier rates (Boulton, 1979).
It must be noted, however, that some geological observations on land are not consistent with 120 to 200 m of glacial erosion in North America over the past 3 m.y., but seem to indicate only glacial stripping of regolith with little bedrock erosion (e.g., Gravenor, 1975; Sugden, 1978). If so, then glacial denudation rates are similar to interglacial rates, but glacial rates are controlled by physical processes whereas chemical weathering is more important during interglacials.
Analogy to the modern Antarctic ice sheet is not very helpful. Documented sedimentation of material transported by the ice sheet is quite slow, indicating slow subglacial erosion. However, data from the best-studied drainage basin of West Antarctica suggest a subglacial erosion rate of O(0.1 to 1 mm/yr), with deposition localized at the grounding line beneath several hundred meters of floating ice shelf (Alley et al., 1989). The generality of this result is unknown.
To summarize, then,
-
ice in wet-based accumulation zones of glaciers and ice sheets erodes and transports unconsolidated materials efficiently; the cumulative effect of midlatitude ice sheets in eroding bedrock remains uncertain;
-
sediments of demonstrable glacial origin from the Laurentide ice sheet indicate denudation rates similar to subaerial values in the region today; and
-
an order of magnitude more sediments than those of demonstrable glacial origin may have been produced by the Laurentide ice sheet and deposited in marine environments.
COUPLED CHANGES
From glacial times until the present, virtually all of the important factors in global chemical weathering experienced significant changes. The planet warmed, the glaciers receded and exposed new materials to weathering, the continental shelves flooded, and precipitation and runoff fields experienced significant perturbations. The net effect of all of these changes on the global rate of chemical denudation is difficult to assess. In this section we will examine some of the coupled changes that occurred, glacial to recent, and finally make an assessment of their net effect.
The Climate/Rock Type Connection
The highest chemical weathering rates on the present Earth occur in regions of high rainfall, high relief, and with limestone and evaporite (e.g., the Yangtze and Brahmaputra rivers) or recent volcanic lithologies (Berner and Berner, 1987). These regions do not occur at high northern latitudes today (or in the recent geologic past) so they did not experience direct glacial coverage during the Wisconsin glaciation. In fact, few of these regions of high chemical denudation fell in zones of decreased soil moisture 18 ka (compare Figures 3.1 and 3.5). Thus there is no clear reason to suspect that glacial, global chemical weathering rates were reduced relative to today, on this basis.
In terms of a climate feedback, silicate weathering is paramount, for as shown above, it is during the weathering of this rock type that net CO2 consumption occurs (averaged over thousands of years). Walker et al. (1981) and Berner et al. (1983) have argued that the balance of CO2 consumption during silicate weathering and production during volcanism regulates atmospheric CO2 concentrations, and thus climate, on time scales longer than a few thousand years. This time scale represents the residence time of atmospheric CO2 with respect to recycling by the carbonate-silicate geochemical cycle (about 3000 yr). Of course, on these short time scales one must consider ocean/atmosphere exchange to be fast, so that perturbations in weathering and volcanism on thousand-year time scales are damped. However, the residence time of the entire ocean/atmosphere carbon reservoir with respect to the geochemical cycle is on the order of 100,000 yr, so we must consider changes in this cycle as potential climate modifiers on glacial time scales.
Where is CO2 being consumed today? For a region to qualify as a significant CO2 sink it should be dominated by silicate exposures and be in a region of high runoff and high physical erosion. By excluding those regions of Figure 3.6 that (1) lie in areas where runoff averages less than 500 mm/yr and (2) are in areas undergoing >100 tons/km2 of physical erosion per year, a map of CO2 sinks is produced (Figure 3.7).
Rapid consumption of CO2 occurs over a relatively restricted part of the land surface. The more important regions include the southeastern United States, the Andes, and southeast Asia. Regions typically assumed to be areas of high chemical weathering (e.g., Amazonia) do not appear, for they have developed thick soil mantles, and chemical weathering rates are actually low.
Of these areas, none fall within a region of higher soil moisture at 18 ka (Figure 3.1), and some probably experienced drier conditions (especially those in southeast Asia and South America). This would suggest that perhaps CO2 consumption rates were somewhat diminished by glacial climates, a negative feedback.
Forest Coverage and Weathering
The presence of forests on land enhances chemical weathering rates by their effect on the soil environment (e.g.,
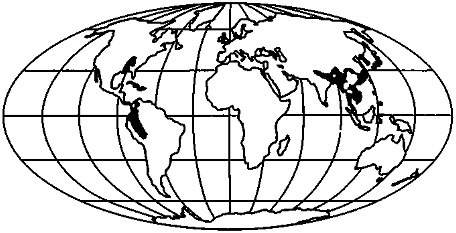
FIGURE 3.7 Modern CO2 sinks defined as regions of "silicate" exposure (Figure 3.6) that have high physical erosion rates (Snead, 1980; Broecker, 1983) and high runoff (UNESCO, 1978).
Knoll and James, 1987). Root respiration produces CO2 in the soil and, aided by bacterial decomposition of forest litter, generates CO2 pressures that are 10 to 100 times the atmospheric value (Cawley et al., 1969; Holland et al., 1986). In addition, roots aid in the physical breakdown of the bedrock. Organic acid production in forest soils also increases the solubility of minerals. These effects would be diminished if the areal extent of forests were diminished.
A marked effect of glaciation on forests is the loss of the temperate deciduous and evergreen forests (e.g., Broecker, 1983). These forests today cover a large fraction of North America, Europe, and Asia (Figure 3.8A); during glacial maximum they were largely removed, either from being overrun by glaciers or from increased aridity (Figure 3.8B).
The areal extent during glacial time of the tropical rainforests of South America and Africa was also reduced due to aridity. This reduction was partially offset by the expansion of tropical forests, especially in Oceania, into shelf regions that became exposed as sea level fell.
In total, it seems that the biological enhancement of chemical weathering rates was significantly reduced during glacial times due to restriction of the areas of temperate and tropical forests. However, these areas were largely replaced by grasslands; the enhancement factor of this ecosystem type should be less, but relative enhancements are poorly known. The implied reduction of chemical weathering rates should, over thousands of years, have tended to increase atmospheric CO2 concentrations due to (1) decrease in the carbonate ion concentration of the ocean, caused by the decrease in the rate of supply of alkalinity from weathering, and thus an increase in the equilibrium pCO2 (e.g., Broecker and Peng, 1987); and (2) an excess of CO2 production by volcanism over consumption by silicate weathering (e.g., Volk, 1987). This feedback is negative; it would tend to counter the climate perturbations producing glacial conditions.
Climate and Glacial Sediment Supply
Wet-based continental ice sheets probably suppress chemical weathering but speed physical erosion, as discussed above. Some results of the growth and decay of a large ice sheet include
-
removal of regolith from central regions, leaving fresh bedrock exposed or covered by a thin layer of glacial sediments;
-
ice-contact deposition of thick sequences of glacially transported sediment in marginal regions, often on top of older regolith, these glacially transported sediments contain much fresh mineral surface area formed by abrasion/comminution and may be deposited in seas, lakes, or on land; some of the terrestrial deposits are flooded by subsequent sea-level rise;
-
fluvial transport of glacially eroded and comminuted sediment into lakes and seas, although typically with significant aggradation of river channels and thus sediment storage [the common occurrence of glacial-age fluvial terraces along modern streams shows that such storage still is occurring (Schumm and Brakenridge, 1987)]; and
-
eolian transport of glaciogenic silt off outwash plains to be deposited as loess in adjacent regions, especially if dry or seasonally dry climates suppress vegetation on outwash and allow desiccation and easier wind erosion (Pye, 1984).
The net result is to increase the chemical reactivity of mineral surfaces in all glacially affected areas. Weathered regolith is thinned or removed beneath the center of the ice sheet to expose fresh bedrock, and comminuted regolith and bedrock are deposited over weathered regolith beneath and beyond the ice margins. Comprehensive data are not available on increases in mineral reactivity from ice-sheet glaciation, but such increases are likely to be significant.
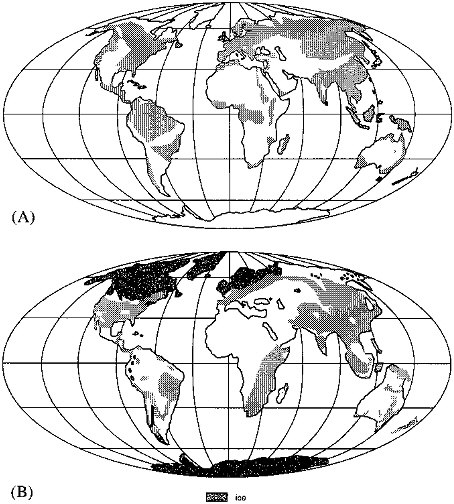
FIGURE 3.8 (A) Regions of present-day forest (Snead, 1980). (B) Extent of forests at 18 ka (National Geographic Society, 1988).
It is of further interest that some glacial-maximum sediment discharge occurred into regions of low soil moisture caused by ice-sheet effects on climate (e.g., COHMAP, 1988), encouraging eolian erosion of outwash plains and loess deposition; source areas not receiving glacial sediments in glacially desiccated climatic regions of Asia may have experienced eolian erosion contributing to downwind loess deposition as well (Pye, 1984). Loess deposits are shown in Figure 3.9, modified from Pye (1984); especially in North America, a little loess extends north of the glacial limit.
These loess deposits typically contain silt-sized, subangular clasts, and are porous, permeable, and relatively unweathered when formed. Subsequent to formation, most have experienced increases in soil moisture and, by inference, in rates of chemical weathering. Some deposits fall within the belt of enhanced monsoonal circulation that peaked around 9 to 12 ka, and these deposits experienced strongly increased soil moisture during that time (compare Figures 3.1 and 3.9; COHMAP, 1988). Loess deposits north of this enhanced monsoonal belt experienced more seasonal climates following deglaciation, with hot, dry summers peaking around 9 to 6 ka. Some loess areas have higher soil moisture today than at any other time since the previous interglaciation, but almost all areas experienced soil-moisture levels higher than those prevailing during loess deposition at some time following deposition (e.g., Kutzbach, 1987; Webb et al., 1987; COHMAP, 1988). The pattern of loess deposition during glacial periods followed by enhanced chemical weathering and soil development on loess has been repeated numerous times, and probably for every Pliocene-Pleistocene Northern Hemisphere glaciation (Fink and Kukla, 1977). The quantitative significance of this pattern for geochemical fluxes is not well constrained, but merits further study.
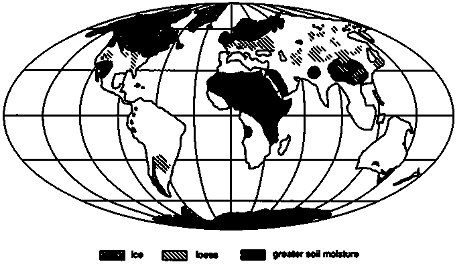
FIGURE 3.9 Loess deposits (after Pye. 1984) and regions with greater soil moisture at 9 ka (based on COHMAP, 1988).
IMPLICATIONS FOR OCEANIC AND ATMOSPHERIC CHEMISTRY AND CLIMATE
To summarize the interpretations given above of the changes in the global environment for chemical weathering 18 ka relative to today, we find that there was
-
no significant, global average change in soil moisture;
-
a small decrease in the global area, but a significant increase in the tropical area, of subaerial exposure;
-
a decrease in the forested area;
-
an increase in carbonate exposures, especially at low latitudes; and
-
a decrease in silicate exposures at high northern latitudes.
To do a convincing job of weighing these factors and determining a net change in the global chemical weathering rate, one would want to make a geographically based geochemical-cycling/climate calculation. In lieu of this, we can merely speculate that these changes in the global chemical weathering rate were roughly compensatory and thus that globally averaged chemical weathering rates during glacial times were similar to those during interglaciations (with large error bars).
The change between 18 ka and today was nonlinear, and some factors that are similar today to their glacial conditions experienced significant perturbations during deglaciation. These probably include
-
an increase in the reactivity of the weathering surface, due to the exposure of "fresh" surfaces (scraped free of regolith or covered with comminuted material) and loess as the glaciers retreated, over about 25 percent of the modern land surface;
-
a peak in the chemical weathering rate of this material in the 0° to 30° N latitude band, at about 12 to 9 ka, when soil moisture values peaked; after this time, weathering rates probably decreased as the fresh surface became covered with a weathered soil; and
-
peaks in chemical weathering of this material corresponding to increasing soil moisture in other bands.
If indeed these perturbations were globally significant, they should have left a record in terms of changes in oceanic or atmospheric chemistry, or in climate. Perhaps the most sensitive indicator of change in the rate of chemical denudation of the continents is the level of the calcium compensation depth (CCD) in the ocean. The depth of this horizon, which separates the region of carbonate sediment accumulation from the deeper region of net carbonate dissolution, is determined by the requirement of carbonate balance of the ocean (Broecker and Peng, 1982; Bender, 1984). For example, an increase in the rate of bicarbonate (and alkalinity) input to the ocean from weathering should cause a depression of the CCD (and an increase in the carbonate ion concentration of the ocean), to ensure that the burial rate of CaCO3 increases and the balance of input and output is thus achieved.
Broecker and Peng (1987) have assessed the sedimentary evidence (Berger, 1970; Peterson and Prell, 1985) for changes in the CCD over the period of interest (Figure 3.10) and have concluded that
-
there was no significant difference between glacial and interglacial times in the carbonate ion content of the ocean or the depth of the CCD; and
-
there was, however, a several-thousand-year perturbation in these oceanic variables centered on 9 ka, coincident with deglaciation.
The first of these conclusions is consistent with our suggestion that chemical weathering rates were similar during
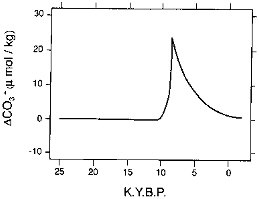
FIGURE 3.10 Perturbation in the carbonate ion concentration of the ocean during the past deglaciation (Broecker and Peng, 1987).
glacial and interglacial times. Broecker and Peng (1987) suggest a change in the rate of nutrient recycling in the ocean to explain the perturbation in the CCD at the close of glacial time. They, and Broecker (1983), do acknowledge that little is known of the potentially important effect of glacial/interglacial changes in continental weathering rates on the carbonate budget of the ocean. We do not propose, based on what we have presented, that such changes are primarily responsible for the CCD perturbation, but they are consistent with it. As our knowledge of oceanic changes in the rate of Quaternary carbonate accumulation improves, perhaps the need will arise to invoke changes in global chemical weathering rates to explain these observations.
Finally, we have found that there is reason to believe that the rate of CO2 consumption during silicate weathering varies on glacial time scales, due both to the direct effects of glacial coverage and erosion, and to the indirect effect of glaciation on climate. In our search for positive and negative feedbacks in glacial climate regulation we must consider this important, apparently negative, and thus stabilizing feedback (Marshall et al., 1988). Too often we think of such geological processes as only acting on million-year time scales or longer; as demonstrated here, changes in chemical weathering rates can affect climate change on 1000 to 100,000 yr periods as well.
ACKNOWLEDGMENTS
This work has benefited greatly from reviews by S. Porter and R. Berner. M. Meybeck also provided helpful comments. The authors acknowledge the support of the National Science Foundation grants EAR-87-20551 to L.R. Kump and DPP-8716016 and DPP-8822027 to R.B. Alley. They thank T. Mistrick of the Pennsylvania State University's Deasy Geographic Laboratory for drafting the figures and D. Detwiler for help in manuscript preparation.
REFERENCES
Alley, R.B., and C.R. Bentley (1988). Ice-core analysis on the Siple Coast of West Antarctica, Annals of Glaciology 11, 1-7.
Alley, R.B., D.D. Blankenship, S.T. Rooney, and C.R. Bentley (1989). Sedimentation beneath ice shelves — The view from ice stream B, Marine Geology 85(2/4), 101-120.
Bell, M., and E.P. Laine (1985). Erosion of the Laurentide region of North America by glacial and glaciofluvial processes, Quaternary Research 23, 154-174.
Bender, M.L. (1984). On the relationship between ocean chemistry and atmospheric pCO2 during the Cenozoic, in Climate Processes and Climate Sensitivity, J.E. Hansen and T. Takahashi, eds., Monograph Series 29, American Geophysical Union, Washington, D.C., pp. 352-359.
Berger, W.H. (1970). Deep-sea carbonate and the deglaciation spike in pteropods and foraminifera, Nature 269, 301-303.
Berner, E.A., and R.A. Berner (1987). The Global Water Cycle: Geochemistry and Environment, Prentice-Hall, Englewood Cliffs, N.J., 397 pp.
Berner, R.A., A.C. Lasaga, and R.M. Garrels (1983). The carbonate-silicate geochemical cycle and its effect on atmospheric carbon dioxide over the past 100 million years, American Journal of Science 283, 641-683.
Bloom, A.L. (1978). Geomorphology: A Systematic Analysis of Late Cenozoic Landforms, Prentice-Hall, Englewood Cliffs, N.J., 510 pp.
Boulton, G.S. (1979). Processes of glacier erosion on different substrata, Journal of Glaciology 23(89), 15-38 (including discussion).
Boulton, G.S., D.L. Dent, and E.M. Morris (1974). Subglacial shearing and crushing, and the role of water pressures in tills from south-east Iceland, Geografisica Annaler 56A(3-4), 135-145.
Broecker, W.S. (1983). The ocean, Scientific American 249, 146-161.
Broecker, W.S., and T.-H. Peng (1982). Tracers in the Sea, Eldigio Press, New York, 690 pp.
Broecker, W.S., and T.-H. Peng (1987). The role of CaCO3 compensation in the glacial to interglacial atmospheric CO2 change, Global Biogeochemical Cycles 1, 15-29.
Cawley, J.L., R.C. Burruss, and H.D. Holland (1969). Chemical weathering in central Iceland: An analog of pre-Silurian weathering, Science 165, 391-392.
CLIMAP (1976). The surface of the ice-age Earth, Science 191, 1131-1136.
COHMAP (1988). Climatic changes of the last 18,000 years: Observations and model simulations, Science 241, 1043-1052.
Collins, D.N. (1979). Quantitative determination of the subglacial hydrology of two Alpine glaciers, Journal of Glaciology 23(89), 347-362.
Corbel, J. (1959). Vitesse de l'erosion, Zeitschrift fir Geomorphologie 3, 1-28.
Drewry, D.J. (ed.) (1983). Antarctica: Glaciological and Geophysical Folio, Scott Polar Research Institute, University of Cambridge.
Edmond, J.M. (1973). The silica budget of the Antarctic circumpolar current, Nature 241, 391-393.
Fink, J., and G.J. Kukla (1977). Pleistocene climates in central Europe: At least 17 interglacials after the Olduvai event, Quaternary Research 7(3), 363-371.
Flint, R.F. (1971). Glacial and Quaternary Geology, John Wiley & Sons, New York, 892 pp.
Ford, D.C. (1971). Characteristics of limestone solution in the southern Rocky Mountains and Selkirk Mountains, Alberta and British Columbia, Canadian Journal of Earth Sciences 8, 585-609.
Gellatly, A.F., J.E. Gordon, W.B. Whalley, and J.D. Hansom (1988). Thermal regime and geomorphology of plateau ice caps in northern Norway: Observations and implications, Geology 16(11), 983-986.
Gibbs, R.J. (1970). Mechanisms controlling world water chemistry, Science 170, 1088-1090.
Gow, A.J., S. Epstein, and W. Sheehy (1979). On the origin of stratified debris in ice cores from the bottom of the Antarctic ice sheet, Journal of Glaciology 23(89), 185-192.
Gravenor, C.P. (1975). Erosion by continental ice sheets, American Journal of Science 275, 594-604.
Hallet, B. (1979). A theoretical model of glacial abrasion, Journal of Glaciology 23(89), 39-50.
Hallet, B. (1981). Glacial abrasion and sliding: Their dependence on the debris concentration in basal ice, Annals of Glaciology 2, 23-28.
Holland, H.D. (1978). The Chemistry of the Atmosphere and Oceans, John Wiley & Sons, New York, 351 pp.
Holland, H.D., B. Lazar, and M. McCaffrey (1986). Evolution of atmosphere and oceans, Nature 320, 27-33.
Hooke, R. LeB. (1977). Basal temperatures in polar ice sheets: A qualitative review, Quaternary Research 7(1), 1-13.
Hughes, T.J. (1981). Numerical reconstruction of paleo-ice sheets, in The Last Great Ice Sheets, G.H. Denton and T.J. Hughes, eds., John Wiley & Sons, New York, pp. 221-261.
Humphrey, N. (1986). Suspended-sediment discharge from Variegated Glacier during pre-surge and surge phases of motion, abstract presented to Chapman Conference on Fast Glacier Flow, Whistler Village, British Columbia, Canada, May 4-8.
Hurd, D.C. (1977). The effect of glacial weathering on the silica budget of Antarctic waters, Geochimica et Cosmochimica Acta 41, 1213-1222.
Knoll, M.A., and W.C. James (1987). Effect of the advent and diversification of vascular land plants on mineral weathering through geologic time, Geology 15, 1099-1102.
Kutzbach, J.E. (1987). Model simulations of the climatic patterns during the deglaciation of North America, in North America and Adjacent Oceans During the Last Deglaciation, W.F. Ruddiman and H.E. Wright, Jr., eds., vol. K-3, The Geology of North America, The Geological Society of America, Boulder, Colorado, pp. 425-446.
Kutzbach, J.E., and P.J. Guetter (1986). The influence of changing orbital parameters and surface boundary conditions on climate simulations for the past 18,000 years, Journal of the Atmospheric Sciences 43, 1726-1759.
MacClintock, P., and A. Dreimanis (1964). Reorientation of till fabric by overriding glacier in the St. Lawrence Valley, American Journal of Science 262, 133-142.
Manabe, S., and A.J. Broccoli (1985). The influence of continental ice sheets on the climate of an ice age, Journal of Geophysical Research 90(D1), 2167-2190.
Marshall, H.G., J.C.G. Walker, and W.R. Kuhn (1988). Long-term climate change and the geochemical cycle of carbon, Journal of Geophysical Research 93, 791-802.
National Geographic Society (1988). Endangered Earth (map), National Geographic 174(6).
Neftel, A., H. Oeschger, J. Schwander, B. Stauffer, and R. Zumbrunn (1982). Ice core sample measurements give atmospheric CO2 content during the last 40,000 yr, Nature 295, 220-223.
Oeschger, H., and B. Stauffer (1986). Review of the history of atmospheric CO2 recorded in ice cores, in The Changing Carbon Cycle: A Global Analysis, J.R. Trabalka and D.E. Reichle, eds., Springer-Verlag, New York, pp. 89-108.
Oswald, G.K.A., and G. de Q. Robin (1973). Lakes beneath the Antarctic ice sheet, Nature 245, 251-254.
Paterson, W.S.B. (1981). The Physics of Glaciers, 2nd ed., Pergamon Press, Oxford, 380 pp.
Peterson, L.C., and W.L. Prell (1985). Carbonate preservation and rates of climatic change: An 800 kyr record from the Indian Ocean, in The Carbon Cycle and Atmospheric CO2: Natural Variations Archean to Present, E.T. Sundquist and W. S. Broecker, eds., American Geophysical Union, Washington, D.C., pp. 251-269.
Porter, S.C. (1977). Present and past glaciation threshold in the Cascade Range, Washington, U.S.A.: Topographic and climatic controls, and paleoclimatic implications, Journal of Glaciology 18(78), 101-116.
Pye, K. (1984). Loess, Progress in Physical Geography 8, 176-217.
Radok, V., R.G. Barry, D. Jenssen, R.A. Keen, G.N. Kiladis, and B. McInnes (1982). Climatic and Physical Characteristics of the Greenland Ice Sheet, Cooperative Institute for Research in Environmental Sciences (CIRES), University of Colorado, Boulder, 193 pp.
Raiswell, R. (1984). Chemical models of solute acquisition in glacial meltwaters, Journal of Glaciology 30(104), 49-57.
Rand McNally (1977). The Atlas of the Oceans, Rand McNally and Co., Chicago, 208 pp.
Reynolds, R.C., and N.M. Johnson (1972). Chemical weathering in the temperate glacial environment of the northern Cascade Mountains, Geochimica et Cosmochimica Acta 36, 537-554.
Rind, D. (1987). Components of the ice age circulation, Journal of Geophysical Research 92(D4), 4241-4281.
Robin, G. de Q. (1955). Ice movement and temperature distribution in glaciers and ice sheets, Journal of Glaciology 2(18), 523-532.
Robin, G. de Q. (1977). Ice cores and climatic change, Philosophical Transactions of the Royal Society of London Series B 280, 143-168.
Robin, G. de Q. (1983). General glaciology, in The Climatic Record in Polar Ice Sheets, G. de Q. Robin, ed., Cambridge University Press, pp. 94-97.
Schumm, S., and G.R. Brakenridge (1987). River responses, in North America and Adjacent Oceans During the Last Deglaciation, W.F. Ruddiman and H.E. Wright, Jr., eds., vol. K-3, The Geology of North America, The Geological Society of America, Boulder, Colorado, pp. 221-240.
Snead, R.E. (1980). World Atlas of Geomorphic Features, Krieger, New York, 301 pp.
Stauffer, B., and W. Berner (1978). CO2 in natural ice, Journal of Glaciology 21(85), 291-299 (with discussion, p. 300).
Sugden, D.E. (1977). Reconstruction of the morphology, dynamics and thermal characteristics of the Laurentide Ice Sheet at its maximum, Arctic and Alpine Research 9, 21-47.
Sugden, D.E. (1978). Glacial erosion by the Laurentide ice sheet, Journal of Glaciology 20(83), 367-391.
Sugden, D.E., and B.S. John (1976). Glaciers and Landscape: A Geomorphological Approach, John Wiley & Sons, New York, 376 pp.
UNESCO (1978). World Water Balances and Water Resources of the Earth, UNESCO Press, Paris.
Volk, T. (1987). Feedbacks between weathering and atmospheric CO2 over the last 100 million years, American Journal of Science 287, 763-779.
Walker, J.C.G., P.B. Hays, and J.F. Kasting (1981). A negative feedback mechanism for the long-term stabilization of Earth's surface temperature, Journal of Geophysical Research 86, 9776-9782.
Webb, T., III, P.J. Bartlein, and J.E. Kutzbach (1987). Climatic change in eastern North America during the past 18,000 years: Comparisons of pollen data with model results, in North America and Adjacent Oceans During the Last Deglaciation, W.F. Ruddiman and H.E. Wright, Jr. , eds., Vol. K-3, The Geology of North America, The Geological Society of America, Boulder, Colorado, pp. 447-462.
White, W.A. (1972). Deep erosion by continental ice sheets, Geological Society of America Bulletin 83, 1037-1056.