5
Geomorphic/Tectonic Control of Sediment Discharge to the Ocean: The Importance of Small Mountainous Rivers
JOHN D. MILLIMAN
Virginia Institute of Marine Studies
JAMES P. M. SYVITSKI
Bedford Institute of Oceanography
ABSTRACT
Analysis of data from 280 rivers discharging to the ocean indicates that sediment loads/ yields are log-linear function of basin area and maximum elevation of the river basin. Other factors controlling sediment discharge (e.g., climate, runoff) appear to have secondary importance. A notable exception is the influence of human activity, climate, and geology on the rivers draining southern Asia and Oceania. Sediment fluxes from small mountainous rivers, many of which discharge directly onto active margins (e.g., western South and North America a most high-standing oceanic islands), have been greatly underestimated in previous global sediment budgets, perhaps by as much as a factor of three. In contrast, sediment fluxes to the ocean from large rivers (nearly all of which discharge onto passive margins or marginal seas) have been overestimated, as some of the sediment load is subaerially sequestered in subsiding deltas. Before the proliferation of dam construction in the latter half of this century, rivers probably discharged about 20 billion tons of sediment annually to the ocean. Prior to widespread farming and deforestation (beginning 2000-2500 yr ago), however, sediment discharge probably was less than half the present level. Sediments discharged by small mountainous rivers are more likely to escape to the deep sea during high stands of sea level by virtue of a greater impact of episodic events (i.e., flash floods and earthquakes) on small drainage basins and because of the narrow shelves associated with active margins. The resulting delta/fan deposits can be distinctly different than the sedimentary deposits derived from larger rivers that discharge onto passive margins.
This paper is reprinted with permission from the Journal of Geology, 1992, volume 100, pp. 525-544. The only changes are that references were updated, references were reformatted to be consistent with others within this volume, and the two data tables and associated references do not appear here; the interested reader is referred to the original paper for these tables.
INTRODUCTION
Estimating the flux and fate of fluvial sediments discharged to the ocean has proved to be difficult, as rivers for which we have at least some data account for only about two-thirds of the land area draining into the ocean. Small rivers (drainage basins <<10,000 km 2) drain only about 20% of the land area, but they number in the many thousands (Figure 5.1) and, as will be seen in this paper, collectively they may contribute much more sediment than previously estimated. Previous attempts (e.g., Holeman, 1968; Milliman and Meade, 1983) assumed that global sediment flux could be calculated by extrapolating the yield of large and medium-sized rivers over large regions. By failing to take into account adequately smaller rivers, however, this assumption led to mistaken conclusions regarding seaward flux of fluvial sediment.
To predict the sediment load of a small river, we need to understand the interaction of numerous factors, including climate, precipitation (both average and peak), discharge (volume and velocity), basin geology, human impact, and the size of the drainage basin. Many workers have tried relating sediment load (or yield-load normalized for basin area) to net and/or gross precipitation, with varying results (see review by Walling and Webb, 1983). For small basins in the western United States, Langbein and Schumm (1958) showed that yields are high with low precipitation (where vegetation is too sparse to retard the erosive capacity of heavy rain and runoff), decrease in areas of medium precipitation, and then increase with higher levels of precipitation. A better relationship was seen between the annual variability of rainfall and sediment transport (Douglas, 1967), with basin relief also having an effect (Fournier, 1960). Other workers, however, have noted a variety of sediment transport trends relative to precipitation (e.g., Ahnert, 1970), leading Walling and Webb (1983, p. 84) to conclude that, "Current evidence concerning the relationship between climate and sediment yield emphasizes that no simple relationship exists."
In this paper we explore fluvial sediment discharge with respect to basin area and basin elevation. Both of these factors have been analyzed previously, but separately. For example, Ruxton and McDougall (1967) found that denudation rates in the Hydrographers Range (Papua New Guinea) are directly related to local relief. Pinet and Souriau (1988) found that the solid load of a river correlated well with mean basin elevation but not with environmental factors (such as rainfall). Potter (1978), Inman and Nordstrom (1971), and Audley-Charles et al. (1977, 1979) showed that large rivers (and their deltas) drain orogenic belts, but mostly discharge into intracratonic basins and trailing edge margins (see Dickinson, 1988, for a detailed review). These latter papers seem to have been overlooked by most geologists and oceanographers.
An inverse relationship between sediment yield and drainage basin area also has been noted (e.g., Schumm and Hadley, 1961), and Wilson (1973) suggested that sediment yield depends mainly on land use and basin area (not precipitation). Milliman and Meade (1983) reported that sediment yield increases by about seven-fold for every order of magnitude decrease in drainage basin area, but this correlation considered only rivers with sediment loads >15 million tons (mt)/yr, thereby excluding rivers with smaller sediment loads.
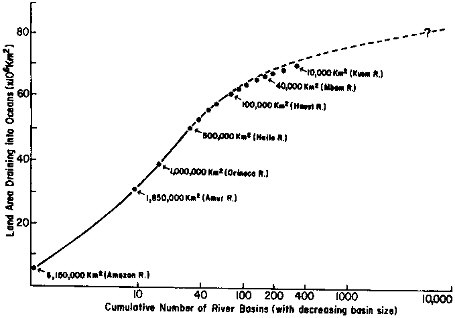
FIGURE 5.1 Cumulative drainage basin area of the world's 400 largest rivers with decreasing basin size. Data from Unesco (1978), this paper, and various IAHS publications. The largest river basin (Amazon) accounts for about 6 x 106 km2 of the 90 x 106 km2 land area (estimated by Milliman and Meade, 1983) draining into the oceans; the next nine largest rivers drain an additional 32 x 106 km2 of land. (Numbers by the dots indicate the drainage basin area for that particular river.) The remaining 20 x 106 km2 of the land surface are probably drained more than 10,000 small rivers.
RIVER DATA
We began by assuming that the topographic/tectonic character of a river basin plays the major role in determining its sediment load/yield, and that sediment yield was partly determined by basin area. Rather than using mean basin elevation as the topographic parameter, we used maximum headwater elevation, because in many rivers much of the sediment load comes from mountains where the river originates. The Amazon is a widely cited example, in which >80% of the sediment load is derived from the Andes, which constitute only about 10% of the river basin area (Gibbs, 1965; Meade et al., 1985). Also, maximum elevations can be estimated quickly from a topographic map. Ahnert (1970) pointed out the strong correlation between local relief and denudation (see review by Summerfield, 1991), but such a calculation becomes difficult when dealing with the number and diversity of rivers cited here.
We subdivided river basins into five categories based on the maximum elevation within the hinterland: high mountain (headwaters at elevations >3000 m), mountain (1000-3000 m), highland (500-1000 m), lowland (100-500 m) and coastal plain (<100 m). Based on a preliminary analysis of the yields, mountainous rivers, comprising the largest data set, were subdivided into three categories: Asia and Oceania (generally with very high sediment loads/yields); the high Arctic and non-alpine Europe (with low sediment loads/yields); and the rest of the world (i.e., North and South America, Africa, the Alps, and Asia Minor, Australia, etc.) Clearly this classification is not without problems. For example, in terms of relief, a small island with elevations of 800-900 m probably should be considered mountainous, not upland. Still, as seen in the following analysis, our elevation-based classification seems valid.
Geomorphologists and hydrologists often use the terms "yield," "sediment yield," or "specific yield" to compare sediment loads between disparate river basins by normalizing sediment load relative to size of the river basin (t/km2/yr). Waythomas and Williams (1988) argue, however, that statistically the comparison of yield versus basin area can give spurious results, since area is common to both axes; they propose the comparison of sediment load and basin area instead. In this paper, data are presented in terms of both yield and load.
Our data base consists of the loads and yields for 280 rivers (Table 1, Milliman and Syvitski, 1992). Collectively these rivers account for >62 x 106 km2, or about two-thirds of the land surface draining into the ocean (Milliman and Meade, 1983). Basin sizes range from <200 km2 to >6,000,000 km2, and loads vary from <0.02 to >1000 mt/yr. Where discharge values are available, we have converted them to runoff (discharge/basin area). The data come from many sources and from a wide variety of techniques, and therefore the quality is variable. Moreover, many of the data are recycled: for example, some of the data used by Lisitzin (1972) are from Strakov (1961), some of which came from Lopatin (1950) and early IAHS/Unesco compilations.
Modern river sediment loads seldom represent natural loads. Sediment discharge changes as erosion levels change or sediment is stored (i.e., river diversion projects). With the exception of Arctic rivers, where human civilization has had minimal impact, most rivers reflect the results of human activity on the erosional capacity of the rivers, both through deforestation and poor soil conservation (see Milliman et al., 1987) and urbanization (Meade, 1982). In contrast, the increased diversion and damming of many rivers has decreased sediment discharge dramatically. The Nile and Colorado deliver no sediment to the ocean, and many other rivers, such as the Mississippi, Zambesi, and Indus, have experienced markedly decreased sediment discharges in recent years. Sediment loads of other rivers have decreased because of other human activities; for example, present-day bed loads of some northeast Italian rivers are 1.5 to 20 times lower than they were in the early 1950s because of legal and illegal riverbed dredging (Idrosser, 1983; I.N. McCave written communication, 1991). Often these human impacts work in conflicting ways: dams on the Ganges have decreased sediment discharge, whereas increased erosion in the mountains of Nepal (from deforestation) has increased the load of the confluent Brahmaputra (Hossain, 1991). In this paper we cite sediment loads of rivers prior to river diversion (at least, where data are available). However, the values given in this paper still reflect increased soil erosion and thus probably are higher than they would be in natural conditions.
RESULTS
Plots of runoff versus basin area, load versus runoff, and load/yield versus basin area (Figure 5.2) show a variety of trends. Runoff decreases with increased basin area (Figure 5.2a), probably because larger river basins tend to include a greater proportion of "lowland," with reduced precipitation and increased evapotranspiration (D. Walling, written communication, 1991). Also, our data for smaller rivers are biased toward rivers with high runoff, as small rivers with low runoff are seldom gauged. With respect to sediment load versus runoff, we find the same random relationship noted by Walling and Webb (1985) for load versus precipitation (Figure 5.2b). In contrast, load/yield vary directly/indirectly with basin area, although the scatter is considerable (Figure 5.2c, d).
When we divide the rivers into the seven topographic categories, a number of trends show much better correlation.
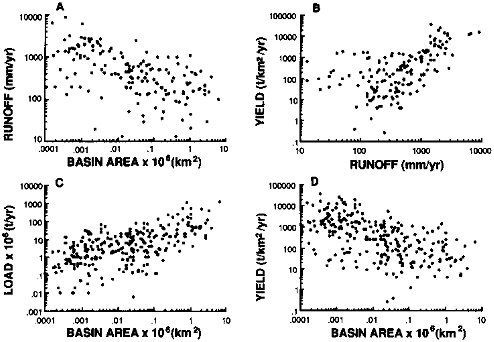
FIGURE 5.2 Runoff versus basin area (A); sediment yield Vs runoff (B); and load (C); and yield (D) versus basin area for the rivers listed in Table 2 (Milliman and Syvitski, 1992). Note the generally inverse relationship between runoff and yield with basin area the strongly positive correlation between load and basin area, and the great amount of scatter for yield versus runoff.
For example, the orographic control of precipitation can be seen from the fact that higher elevation rivers have greater maximum values of runoff versus basin area (Figure 5.3). The greater scatter of runoff with decreasing basin size reflects the influence of local climate (i.e., precipitation versus evaporation) in small basins. While the trends of sediment load/yield versus runoff vary with topography (Figure 5.4), the correlation coefficients (r2) between load/yield and runoff within any topographic category are not meaningful (Table 2, Milliman and Syvitski, 1992).
Log-linear trends within our seven topographic categories were determined from best-fit regression analysis. In accordance with the well-accepted method for not allowing spurious data to influence the slope of the regression, points that fell more than one standard deviation from the determinant (y axis as load or yield) were plotted but not considered in determining the variance accounted for by the best-fit curve (Table 2, Milliman and Syvitski, 1992). Our philosophy was simple: we could not be sure of what errors were hidden within sediment load data, we assumed little error in the x-axis (drainage basin area), and we wished to discount as few data points as possible.
In Table 2 (Milliman and Syvitski, 1992), <10% of the rivers were discounted on the basis of having either load or yield values more than 1 standard deviation from the mean. In fact, deviations from the predicted norm often reflect either unique fluvial/drainage basin conditions or possible erroneous data bases; various examples are discussed below.
For load/yield versus basin area, the correlations with the various topographic categories are generally good, ranging from 0.70 to 0.82 (load versus area) and 0.62 to 0.89 (yield versus area) (Figures 5.5 and 5.6; see Table 2, Milliman and Syvitski, 1992). The relatively poor correlation coefficients (r2 = 0.81 for load, but 0.32 for yield) for coastal plain rivers, however, suggest that basin area plays little or no role in determining sediment discharge from these low-lying rivers.
Mountainous rivers have greater loads and yields than do upland rivers, which in turn have greater loads and yields that lowland rivers (Figures 5.5 and 5.6), although there is some overlap in values. For example, mountainous rivers with basin areas of about 10,000 km2 have sediment yields between 140 and 1700 t/km2/yr (e.g., Negro, Porong), whereas yields for similar-sized upland rivers are 60-250 (e.g., Sabin, Tone), and lowland rivers 20-60 (e.g., Cape Fear River). With the exception of two rivers (Waiapu and Niger), no upland, lowland or coastal plain river has a sediment load >20 mt, even though more than 25 upland and lowland rivers have drainage basin areas >100,000 km2. In contrast, nearly 60 mountainous rivers have loads ≥20 mt (Table 1, Milliman and Syvitski, 1992). Mountainous rivers draining South Asia and Oceania
have much greater yields (2-3 fold) than rivers draining other mountainous areas of the world, and an order of magnitude greater than rivers draining high-Arctic and European mountains (Figure 5.5).
The trend of increasing sediment yield with decreasing size of mountainous rivers becomes less pronounced in river basins less than about 4000 km2 in area, as seen by the relative number of rivers that fall >1 standard deviation from the mean (Table 1, Milliman and Syvitski, 1992). Some very small rivers in New Zealand and Taiwan, for example, have yields much lower than expected, while others have much higher yields; together they account for one-third of the deviating rivers designated in Table 1 (Milliman and Syvitski, 1992). Slaymaker (1987) noted a decreased sediment yield in rivers <1000 km in western Canada. This variance of sediment yield in very small river basins probably reflects the dominance of single types of geology or microclimate in small basins, whereas larger river basins are modulated by a greater range of conditions.
With the exception of the high Arctic, latitude does not appear important. Equatorial rivers (e.g., the Tana in Kenya) do not have significantly higher yields than rivers of similar size in higher latitudes e.g., the Susitna in Alaska). High-Arctic mountainous rivers whose headwaters rise in the Arctic (e.g., Colville, Babbage), however, have much lower yields than Arctic rivers whose headwaters are in lower latitudes (e.g., Copper, Yukon, MacKenzie). The reason is not clear, but it may be related to lower levels of precipitation and shorter periods during which the rivers can transport sediment (Milliman and Syvitski, unpublished data).
DISCHARGE OF SEDIMENT BY WORLD RIVERS
North America
Most rivers draining eastern North America are upland, lowland, or coastal plain rivers, with correspondingly low sediment loads. Much of the sediment leaving the contiguous United States and Canada comes from three large rivers—the Mississippi, MacKenzie, and Colorado (now dammed)—and smaller west coast rivers (e.g., Eel, Columbia, Fraser), most of which drain mountains. Large discharges of sediment also come from rivers draining western Canada and Alaska; the Susitna, Cooper, and Stekine rivers, for example, collectively drain an area <4% that of the Mississippi, but discharge nearly a third as much sediment (Table 1, Milliman and Syvitski, 1992); the many other rivers along this coast also must contribute large amounts of sediment: the average thickness of Holocene sediment on the southeast Alaskan shelf is 55 m (Molnia et al., 1978) and fjords into which many of these rivers discharge have Quaternary sediment thicknesses >500 m (Syvitski et al., 1987).
South America
Eastern South America is drained by four major rivers (Magdalena, Orinoco, Amazon, Parana) all having their headwaters in the Andes Mountains. Collectively they drain more than half the continent (10 of 17 million km2). In contrast, rivers draining the western Andes are less known, but collectively their sediment discharge may be of the same magnitude as the larger rivers draining eastward
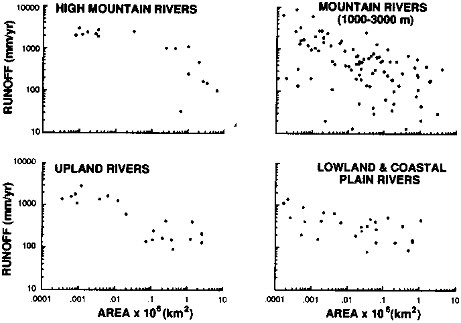
FIGURE 5.3 Variation of runoff versus basin area for rivers within four topographic categories. Note the decreasing maximum runoff values with increased river basin area and with lower elevations.
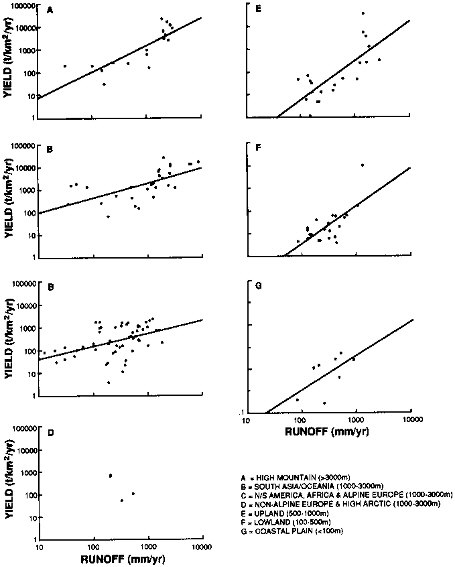
FIGURE 5.4 Relation of sediment yield and runoff for the seven topographic categories of river basins listed in Table 1 (Milliman and Syvitski, 1992). The equations for the slope plus the correlation coefficients are given in Table 2 (Milliman and Syvitski, 1992). In nearly all instances, the correlation coefficients are poor and the deviations from the trend are numerous.
(smaller area but higher yields). If the average river draining the western sides of the mountains is 15,000 km2, then the average sediment yield would be about 1200 t/km2/yr (Figure 5.6c), equaling a sediment discharge of 2.4 bt/yr (1200 multiplied by an area of 2 x 106 km2). This calculated sediment flux may be unrealistically high, as the arid parts of the western slope may contribute little sediment to the sea; nevertheless, the total sediment discharge from western South American rivers probably is much higher than the 168 mt estimated by Milliman and Meade (1983). At present we can cite only one west coast river, the Chira (Peru), and the data represent only two years of measurement, for one of which, however, the load was 75 mt (yield 3700 t/km2, Burz, 1977).
Europe
Europe is generally regarded as having the lowest sediment flux to the sea (e.g., Holeman, 1968; Milliman and Meade, 1983). However, the Alps (a collision orogen) are a major sediment source, and the short rivers draining south into the Mediterranean have high to very high yields, generally 500 to >1000 t/km2/yr (Table 2, Milliman and Syvitski, 1992). For example, the little known Semani River (Albania) has more than twice the annual discharge (22 mt) of the collective sediment discharges of the well-known north-flowing rivers Garonne, Loire, Seine, Rhine, Weser, Elbe, Oder, and Vistula, most of which drain upland or lowland terrain. Many rivers draining north from
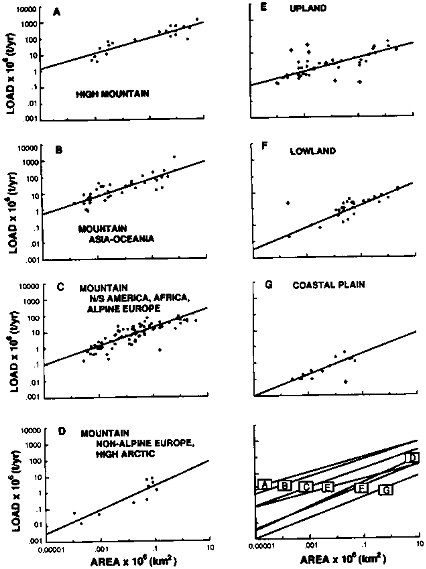
FIGURE 5.5 Variation of sediment load with basin area for the seven topographic categories of river basins listed in Table 1 (Milliman and Syvitski, 1992). For all river types the correlation is strong (r2 ranging from 0.70 to 0.82). Note that only 13 rivers deviate by more than one standard deviation from the computed means.
the Alps are tributaries to the Danube, the largest river in Europe. The Rhine is the only large alpine river that drains north to the sea, but most of its sediment load is trapped in Lake Constance; upstream of Lake Constance, the river has a sediment yield consistent with other alpine rivers, but downstream of the lake, its yield is similar to a lowland/coastal plain river (Holeman, 1968).
USSR and Asia Minor
The large rivers of the former Soviet Union draining north to the Arctic Sea (Ob, Lena, and Yenesi) are generally considered to have anomalously low sediment yields (see Milliman and Meade, 1983). However, their sediment yields and those of other Russian rivers correlate well with other upland and lowland rivers throughout the world (Figure 5.6; Table 1, Milliman and Syvitski, 1992). Russian and Ukrainian rivers draining south into the Black Sea are considered lowland rivers, with correspondingly low sediment loads.
Although poorly documented in western literature, the rivers draining the Caucasus Mountains and the Anatolian and Taurus mountains in Turkey have high sediment yields, which is to be expected from rivers draining the same collision orogen as the Alps. Before dam construction in the 1950s, the three largest Turkish rivers emptying into the Black Sea discharged an estimated 50 mt of sediment annually (Hay, 1987). Collectively, in fact, the rivers draining northern Turkey and the western Caucasus Mountains may contribute more sediment to the Black Sea than the Danube and southwestern Russian and Ukranian rivers.
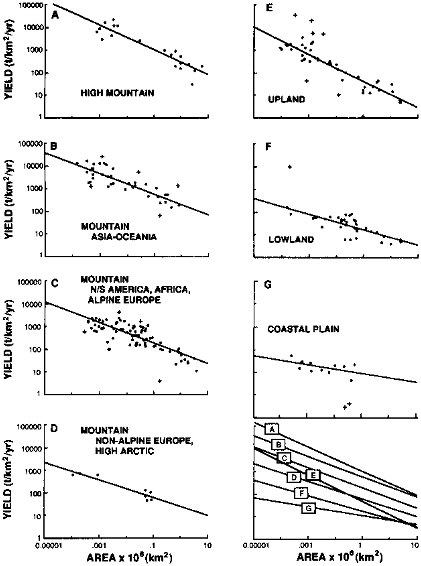
FIGURE 5.6 Variation of sediment yield with basin area for the seven topographic categories of river basins listed in Table 1 (Milliman and Syvitski, 1992). For all river types, except lowland and coastal plain rivers, the correlation is strong (r2 ranging from 0.70 to 0.89).
Africa
Rivers draining Africa discharge a disproportionately small amount of sediment to the sea, although the discharge calculated by Milliman and Meade (1983) is probably low (Walling, 1985). At first it seems incongruous that Africa, one of the highest-standing continents (in terms of average elevation), has a low sediment flux. Only when viewed in terms of drainage basin morphology does the discharge pattern make sense; some large rivers with low loads (e.g., Senegal, Niger) are nonmountainous, and many small rivers in western Africa are lowland rivers, with correspondingly low sediment loads/yields. The major sediment discharge comes from rivers draining the rift mountains in eastern Africa (Nile, Zambesi, Limpopo, Rifiji) or rivers draining the mountains in Morocco, Algeria, and Tunisia (e.g., Mouloura, Sebou, Cheliff). The loads and yields of these rivers compare well with other mountainous rivers of similar size. The lack of rainfall throughout most of central Africa contributes to the low discharge rates (Walling, 1985).
Asia and Oceania
With notable exceptions of the loess-imparted Yellow River basin, the high sediment yield in Asia is restricted to rivers draining the Himalayan Mountains in southern Asia. These loads and yields are substantially higher than all other mountainous rivers of the world (save Oceania). Rivers draining eastern Asia have normal (Korea) or low (Japan) sediment loads relative to other mountainous rivers; dams in Japan may be important in these values. The
Chao Phya has a much smaller load (11 mt) and yield (68 t/km2/yr) than other south Asian rivers of similar size; one wonders if the river basin has anomalous erosion patterns or if the data are erroneous.
Milliman and Meade (1983) used data from Taiwan and New Zealand to suggest that rivers draining the high-standing islands between Australia and Asia have unusually high sediment yields. Assuming a sediment yield of 1000 t/km2/yr, they calculated that these high-standing islands may account for 20% of the global sediment flux to the oceans. In fact, new data from New Guinea, the Philippines, Java, New Zealand, and Taiwan (Table 1, Milliman and Syvitski, 1992) suggest average yields closer to 3000.
Australia
While Australia is nearly as large in area (2.2 x 106 km2) as the islands in Oceania, the continent is generally low standing. Only rivers draining mountainous areas in the north (e.g., Ord) and east (e.g., Murray) appear to have high loads. The fact that much of Australia has an arid climate accentuates the low discharge from rivers, although the yields from the Ord, Murray, and Burdekin compare favorably with other rivers of similar size (Table 1, Milliman and Syvitski, 1992).
IMPLICATIONS
Factors Controlling Sediment Discharge
The data presented in Tables 1 and 2 (Milliman and Syvitski, 1992) and Figures 5.5 and 5.6 clearly show the importance of basin size and topography in terms of sediment discharge. Because sediment yields are strongly dependent upon the size of the drainage basin, they cannot be portrayed accurately on a map; global displays of sediment yields (e.g., Milliman and Meade, 1983; Walling, 1987) essentially reflect topography (as well as basin size)—high yields equate to mountainous areas, low yields to lowlands.
While many of our data need to be re-evaluated and updated, we suggest that topography and basin area have order-of-magnitude control over sediment discharge of most rivers. In contrast, average net precipitation and runoff generally affect sediment discharge to a lesser extent. For example, the Orange, Sous, and Isser rivers, which drain arid basins, have similar or slightly lower sediment yields than mountainous rivers with moderate rainfall, whereas rivers draining areas with very heavy precipitation (e.g., Solo, Purari, Cooper) have slightly higher yields (see Table 1, Milliman and Syvitski, 1992).
The role of sediment erodability (mainly a function of geology, vegetation cover, and human activity) clearly cannot be discounted. High erosion rates throughout much of southern Asia partly reflect poor soil conservation, the result of deforestation and over-farming. Milliman et al. (1987) concluded that the Huanghe's sediment load was an order of magnitude lower before humans began farming the loess hills of northern China. (Saunders and Young [1983] suggested that moderate land use can increase sediment yield by a factor of 2-3, while intensive land use can increase it an order of magnitude.) In contrast, the anomalously low sediment yields of northern European and English rivers at least partly reflect river channel management (see Petts et al., 1989) combined with extensive vegetation cover and relatively low soil erodibility (D. Walling, 1991 written communication). The Oder, for example, has the lowest yield (1.2) of any river cited in this paper.
We should emphasize that elevation or relief is, in some ways at least, only a surrogate variable for tectonism. This paper and others (e.g., Hay et al., 1989) that have emphasized the correlation between topography and sediment yield, relief or elevation is used because it is easily expressed numerically and therefore can be manipulated as a statistical variable. However, the strong correlation between sediment and topographic relief may not indicate that the second is the cause of the first, but rather that both are caused by another factor less susceptible to numerical description—namely, tectonism. It is probably the entire tectonic milieu of fractured and brecciated rocks, oversteepened slopes, seismic and volcanic activity, rather than simple elevation/relief, that promotes the large sediment yields from active orogenic belts.
What Is the Sediment Flux to the Sea?
This question really has two parts: how much sediment is carried by rivers, and how much escapes the present-day land/estuarine environment? The answer to both is more or less the same—we don't know. The sediment discharged, however, may be more than previously estimated. Milliman and Meade (1983) calculated an annual global discharge of 13.5 bt by extrapolating average sediment yields for documented rivers over large regions with similar topography. However, since the data used by Milliman and Meade came mostly from large rivers, the yields were necessarily lower than if they also had included smaller rivers. In addition, constrained by the lack of data, Milliman and Meade conservatively estimated the yields for mountainous coastal rivers to be 1000 t/km2/yr. The new data presented in this paper suggest that the yields for rivers draining Oceania are probably ≥3000/km 2/yr, meaning that the high-standing islands of Oceania (approximate area of 3 x 106 km2) may be closer to 9 bt than the 3 bt estimated
by Milliman and Meade. Similar percentage increases might hold for southeastern Alaska, western South America, the southern Alps-Caucasus orogen, and NW Africa (e.g., Walling, 1985).
There is another way to calculate the flux: The rivers (listed in Table 1, Milliman and Syvitski, 1992) are greater than 10,000 km2 in drainage basin area, and collectively they discharge (before dam construction) slightly more than 8 bt of sediment annually. River basins <10,000 km2 account for slightly >20% of the total drainage area to the ocean (20 x 106 km2; Figure 5.1). Assuming that the mean drainage basin area of these rivers is 1000 km2, an additional 20,000 rivers would be required to account for the entire 20 x 106 km2. If we assume that 10% of these rivers (i.e., 2000) are mountainous and that of these half drain high mountains and or Asia/Oceania and the other half drain mountains exclusive of the Arctic and non-alpine European, the combined loads of these rivers would be (8 mt/river/yr x 1000 rivers) + (1.5 mt/river/yr x 1000 rivers) (see Figure 5.5), or a total of 9.5 bt/yr. This number is surprisingly close to our estimate for the rivers (mostly small) draining Oceania, but since it does not include southern Asia or western North and South America, our calculation may be too conservative. Although the yields for similar-sized upland and lowland rivers are significantly lower (900 and 90 t/km2/yr, respectively), there are more of them, and the combined small upland and lowland rivers might contribute another 1-2 bt annually. Adding undocumented rivers larger than 10,000 km2 probably would add another 1-2 bt. The combined total suspended discharge conservatively might be 20 bt.
A regional example of the influence of small mountainous rivers in sediment discharge can be seen in southern Europe. Milliman and Meade (1983) pointed out that the rivers draining south from the Alps have much higher yields than those rivers draining northern Europe. Assuming a yield of 120 t/km2/yr and a combined drainage of 0.55 x 106 km2, Milliman and Meade calculated that the southern rivers discharge 66 mt/yr to the Mediterranean Sea. In fact, the sediment loads of southern alpine rivers are much greater: the 24 mountainous rivers listed in Table 1 (Milliman and Syvitski, 1992) drain only 0.22 x 106 km2, but collectively they discharge more than 140 mt of sediment annually. If the values are similar for the remainder of the combined drainage area, total sediment discharge would be 350 mt/yr, five times the value calculated by Milliman and Meade.
Unfortunately, calculating world-wide discharge is more complicated, because not all sediment carried by large rivers reaches the sea: some is stored along the lower reaches of rivers and adjoining deltas. If subsidence rates in the Bengal Delta are 1-2 cm/yr (cf. Milliman et al., 1987; J.R. Curray, oral communication, 1991), for example, 40-80% of the sediment load carried by the Ganges/ Brahmaputra may be sequestered in the subaerial portion of the delta, perhaps explaining the relative lack of Holocene sediment accumulating on the adjacent shelf (Kuehl et al., 1989) and the lack of net progradation of the delta front (Alam, 1987). As a result, it is entirely possible that the present sediment discharge of large rivers has been overestimated.
Because rivers are being dammed at an increasing rate, many of the numbers given in this paper are probably out of date. Pearce (1991) states that 13% of all fluvial discharge is presently dammed. Ironically, with their high sediment yields and therefore (at least relatively) high sediment loads, Asian rivers can fill their dammed reservoirs quickly thereby shortening the lives of these dams more quickly than calculated by the engineers who designed them. But since pre-dam sediment loads for most rivers were artificially high due to human activities in the drainage basins, dam construction, for example on the southeastern US rivers, probably has offset anthropogenically enhanced erosion, and post-dam discharges may not be too different from those prior to European colonization (Meade and Parker, 1985).
Even if the present global flux of river sediment could be calculated, the significance of such a number to either future or past river discharge is questionable. Mid-twentieth century river discharge (to the sea) may have been about 20 bt/yr, nearly half of this amount coming from oceania and another third from southern Asia. But because sediment loads may have increased by a factor of 2-10 since humans began farming (see Saunders and Young, 1983; Berner and Berner, 1987), the annual sediment discharge 2000-2500 yr ago may have been considerably < bt. Extensive human influence in Oceania and southern Asia suggests that sediment loads in this area are disproportionately elevated.
Active Versus Passive Margin Rivers
All rivers with large sediment loads originate in mountains. Most large rivers discharge to the sea along passive continental margins, and they act as point-sources for sediment influx; as a result, large deltas (e.g., Mississippi, Nile, Amazon, Ganges, Indus, Yangtze) form on passive margins or in marginal seas (Audley-Charles et al., 1977; Inman and Nordstrom, 1971; Potter, 1978).
In contrast, rivers that drain mountainous islands and the active edges of continental margins (e.g., western North and South America) or collision margins (southern Europe, southern Asia) are generally much smaller, but collectively they may transport similar amounts of sediment
as do passive margin rivers. In most instances, however, classic deltas do not form, although coalescing deltaic/fan deposits may form along the outer continental margins (e.g., Thornberg et al., 1990). Because these small rivers empty onto active margins, the deposits may be subducted, such that the sedimentary sequences are neither thick nor old. The sedimentary sequences also should experience an accelerated thermal history, thus complicating petroleum maturation.
These calculated trends still may underestimate the relative importance of small rivers in terms of sediment delivery to the sea: smaller rivers often have no estuaries, are more susceptible to periodic floods and (because of their steeper gradients and proximity to source material) have larger contributions from bedload material, which seldom is included in the sediment load values reported in the literature (e.g., Syvitski and Farrow, 1983). In addition, along active margins earthquakes and volcanic eruptions can result in mudslides and floods that can increase the sediment loads of adjacent rivers. In the four months following the eruption of Mount St. Helens (Washington State), for example, the sediment load of the Cowlitz River (a tributary of the Columbia) was 140 mt, compared to normal annual load for the Columbia of 10 mt (Hubbell et al., 1983); for the few years after the eruption, the Columbia River discharged an estimated 35 mt/yr (Meade and Parker, 1985).
Smaller mountainous rivers are therefore more likely to discharge larger percentages of their sediment loads directly to the sea than do larger rivers. Moreover, the sediment is more likely to escape the narrow shelves to deeper basins during both high and low stands of sea level.
The Santa Clara River (southern California) serves as an example of both the episodicity and shelf-escape possible with small rivers. During 18 yr of monitoring, more than half the total sediment transported by the Santa Clara was carried in three floods, lasting a total of seven days (Milliman, 1991, after Meade, 1992). Following a major flood in 1969, Drake et al. (1972) traced the fate of the discharged sediment as it entered the Santa Barbara Basin and ultimately was dispersed there by a series of slumps and turbidity currents.
If sediment discharged from small mountainous rivers can by-pass active margins during high stands of sea level, then standard models of sequence stratigraphy, which have been so successful in determining the position of eustatic sea level in older sedimentary deposits (e.g., Haq et al., 1987), may have less application off active margins. On the other hand, active margin deposits appear to be far less common in the geological record, probably because many of them are subducted back into the arc/orogens that border the active margins (von Huene and Scholl, 1991).
ACKNOWLEDGMENTS
We thank G.A. Griffiths, D. Walling, R.H. Meade, B. J. Hay, M.B. Collins, and I.N. McCave for supplying unpublished data and pointing out published sources. Meade, McCave, and Walling, as well as M.G. Gross, B.U. Haq, and S.A. Schumm, helped considerably with their comments on earlier versions of this paper. A special thanks to K.W.G. LeBlanc of the geological Survey of Canada for his liaison and data manipulation. JDM acknowledges the National Science Foundation and the Office of Naval Research for long-term support through which many of the data and insights regarding rivers were collected.
REFERENCES
Ahnert, F. (1970). Functional relationships between denudation, relief, and uplift in large mid-latitude drainage basins, American Journal of Science 268, 243-263.
Alam, M. (1987). Bangladesh, in The Encyclopedia of World Regional Geology II, R.W. Fairbridge, ed., van Nostrand Reinhold, Stroudsburg, Pa.
Audley-Charles, M.G., J.R. Curray, and G. Evans (1977). Location of major deltas , Geology 5, 341-344.
Audley-Charles, M.G., J.R. Curray, and G. Evans (1979). Significance and origin of big rivers: A discussion, Journal of Geology 87, 122-123.
Berner, E.K., and R.A. Berner (1987). The Global Water Cycle: Geochemistry and Environment, Prentice-Hall, Englewood Cliffs, N. J., 397 pp.
Burz, J. (1977). Suspended-load discharge in the semiarid region of northern Peru, International Association of Hydrological Sciences, Publication 122, 269-277.
Dickinson, W.R. (1988). Provenance and sediment dispersal in relation to paleotectonics and paleogeography of sedimentary basins, in New Perspectives in Basin Analysis, K.L. Kleinspehn and C. Paola, eds., Springer-Verlag, New York, pp. 3-25.
Douglas, I. (1967). Man, vegetation, and the sediment yield of rivers, Nature 215, 925-928.
Drake, D.E., R.L. Kolpack, and P.J. Fischer (1972). Sediment transport on the Santa Barbara-Oxnard shelf, Santa Barbara Channel, California, in Shelf Sediment Transport: Process and Pattern, D.J.P. Swift, D.B. Duane , and O.H. Pilkey, eds., Dowden, Hutchinson and Ross, Stroudsburg, Pa., pp. 207-331.
Fournier, F. (1960). Climat et Erosion, Presses Universitaires de France, Paris, 201 pp.
Gibbs, R.J. (1965). The geochemistry of the Amazon River system: Part I. The factors that control the salinity and the composition and concentration of the suspended solids, Geological Society of America Bulletin 78, 1203-1232.
Haq, B.U., J. Hardenbol, and P. Vail (1987). Chronology of fluctuating sea levels since the Triassic, Science 235, 1156-1167.
Hay, B.J. (1987). Particle flux in the western Black Sea in the present and over the last 5000 years: Temporal variability, source and transport mechanisms, unpublished Ph.D. thesis, WHOI-MIT, Cambridge, MA., 201 pp.
Hay, W.W., C.A. Shaw, C.A., and C.N. Wold (1989). Balanced paleogeographic maps: Geologische Rundshau 78, 207-242.
Holeman, J.N. (1968). Sediment yield of major rivers of the world, Water Resources Research 4, 737-747.
Hossain, M.M. (1991). Total sediment load in the lower Ganges and Jumuna, Bangladesh University of Engineering and Technology, 15 pp.
Hubbell, D.M., J.M. Laenen, and S.W. McKenzie (1983). Characteristics of Columbia River sediment following the eruption of Mount St. Helens on May 18, 1980, U.S. Geological Survey Circular 850-J, 21 pp.
Idroser (1983). Piano progettuale per la difesa della costa adriatica Emiliano-Romagnola: Vol. IV. Il transport solido fluviale nei bacini tributari dell'adriatico, Bologna, 429 pp.
Inman, D.L., and C.E. Nordstrom (1971). On the tectonic and morphologic classification of coasts, Journal of Geology 79, 1-21.
Kuehl, S.A., T.M. Hariu, and W.S. Moore (1989). Shelf sedimentation off the Ganges-Brahmaputra river system: Evidence for sediment bypassing to the Bengal fan, Geology 17, 1132-1135.
Langbein, W.B., and S.A. Schumm (1958). Yield of sediment in relation to mean annual precipitation, Transactions, American Geophysical Union 39, 1076-1084.
Lisitzin, A.P. (1972). Sedimentation in the World Ocean, Special Publication 17, Society of Economic Paleontologists and Mineralogists, Tulsa, Okla.
Lopatin, G.W. (1950). Erozia y stok nanosov: Priroda no. 7.
Meade, R.H. (1982). Sources, sinks, and storage of river sediment in the Atlantic drainage of the United States, Journal of Geology 90, 235-252.
Meade, R.H. (1992). River-sediment inputs to major deltas, in Rising Sea Level and Subsiding Coasts, J.D. Milliman, ed., Wiley, New York.
Meade, R.H., T. Dunne, J.E. Richey, U. de M. Cents, and E. Salati (1985). Storage and remobilization of suspended sediment in the lower Amazon River of Brazil, Science 228, 488-490.
Meade, R.H., C.F. Nordin, Jr., W.F. Curtis, F.M. Rodigues, C. M. do Vale, and J.M. Edmond (1979). Sediment loads in the Amazon River, Nature 278, 161-163.
Milliman, J.D., and R.H. Meade (1983). World-wide delivery of river sediment to the oceans, Journal of Geology 91, 1-21.
Milliman, J.D., Y.S. Qin, M.E. Ren, and Y. Saito (1987). Man's influence on the erosion and transport of sediment by Asian rivers: The Yellow River (Huanghe) example, Journal of Geology 95, 751-762.
Molnia, B.F., P.R. Carlson, and W.P. Levy (1978). Holocene sediment volume and modern sediment yield, northeast Gulf of Alaska (abs), American Association of Petroleum Geologists Bulletin 62, 545.
Pearce, F. (1991). A dammed fine mess, New Scientist (May 4), 36-39.
Petts, G.E., H. Møller, and A.L. Roux, eds. (1989). Historical Changes of Large Alluvial Rivers: Western Europe, Wiley, Chichester, 365 pp.
Pinet, P., and M. Souriau (1988). Continental erosion and large-scale relief, Tectonics 7, 563-582.
Potter, P.E. (1978). Significance and origin of big rivers, Journal of Geology 86, 13-33.
Ruxton, R.P., and I. McDougall (1967). Denudation rates in northeast Papua from potassium-argon dating of lavas, American Journal of Science 265, 545-561.
Saunders, I., and A. Young (1983). Rates of surface processes on slopes, slope retreat, and denudation, Earth Surficial Processes and Landforms 8, 473-501.
Schumm, S.A., and R.F. Hadley (1961). Progress in the application of landform analysis in studies of semiarid erosion, U.S. Geological Survey Circular 437, 14 pp.
Slaymaker, O. (1987). Sediment and solute yields in British Columbia and Yukon: Their geomorphic significance reexamined, in International Geomorphology 1986, Part I, V. Gardiner et al., eds., Wiley, Chichester, pp. 925-945.
Strakhov, N.M. (1961). Onekotroykh zakonomermostiakh denudatsii i perenosa osadochnogo materiala na ploshchadyakh gymidnykh klimatov, in Sovremennye osadki moei i oceanov, N.M. Strakov, P.L. Bezrykov, and V.S. Yablokov, eds., Izdatelstovo Akademia Nauk SSSR, Moscow, pp. 5-27.
Summerfield, M.A. (1991). Global Geomorphology, Longman Scientific and Technical, Essex (England), 537 pp.
Syvitski, J.P.M., and G.E. Farrow (1983). Structures and processes in bayhead deltas: Knight and Butte Inlets, British Columbia, Sedimentary Geology 36, 217-244.
Syvitski, J.P.M., D.C. Burrell, and J. Skei (1987). Fjords: Processes and Products, Springer-Verlag, New York, 379 pp.
Thornberg, T.M., L.D. Kulm, and D.M. Hussong (1990). Submarine fan development in the southern Chile Trench: A dynamic interplay of tectonics and sedimentation, Geological Society of America Bulletin 102, 1658-1680.
UNESCO (1978). World register of rivers discharging into the oceans, unpublished manuscript.
von Huene, R., and D.W. Scholl (1991). Observations at convergent margins concerning sediment subduction, subduction erosion, and the growth of continental crust, Reviews of Geophysics 29, 279-316.
Walling, D.E. (1985). The sediment yields of African rivers, International Association of Hydrological Sciences, Publication 144, 265-283.
Walling, D.E. (1987). Rainfall, runoff, and erosion of the land: A global view, in Energetics of Physical Environment, K.J. Gregory, ed., Wiley, London, pp. 89-117.
Walling, D.E., and B.W. Webb (1983). Patterns of sediment yield, in Background to Palaeohydrology, K.J. Gregory, ed., Wiley, Chichester, pp. 69-100.
Waythomas, C.F., and G.P. Williams (1988). Sediment yield and spurious correlation — Toward a better portrayal of the annual suspended-sediment load of rivers, Geomorphology 1, 309-316.
Wilson, L. (1973). Variations in mean annual sediment yield as a function of mean annual precipitation, American Journal of Science 273, 335-349.