A POSITRON NAMED PRISCILLA
Trapping and Manipulating Atoms
by T. A. Heppenheimer

"There's plenty of room at the bottom," the physicist Richard Feynman declared in 1959. Speaking at the annual meeting of the American Physical Society, he envisioned an advanced technology whereby small machines would build yet smaller ones that would go on to build tinier ones still. Each of these generations might minister in turn to the diminishingly small fleas that have smaller ones to bite them, in the poem by Jonathan Swift, but Feynman had a different goal in mind. He raised, in his words, "the possibility of maneuvering things atom by atom. … It is something, in principle, that can be done; but, in practice, it has not been done because we are too big."
Today, however, we are beginning to take significant steps toward achieving Feynman's vision. A new instrument, the scanning tunneling microscope, already has picked up and dropped single atoms into specific locations. It has even dragged such atoms across an underlying surface. Physicists, working with lasers, have caused
individual atoms to move slowly and to stop on demand, permitting measurements of exquisite precision.
THE SCANNING TUNNELING MICROSCOPE
How is it possible to manipulate atoms on a surface, as if they were a child's toy blocks? The beginning of an answer lies in appreciating that, when seen at close quarters, an atom is not a hard-shelled object like a billiard ball. It lacks a distinct surface; instead, it surface is fuzzy. The fuzziness, in turn, results because the properties of an atom's perimeter derive from the behavior of its outer electrons. If those electrons could move outward only to a distinct radius and no farther, the atom indeed would resemble a billiard ball. In fact, these electrons have some likelihood of being found at greater distances from the nucleus. This likelihood or probability drops off rapidly as the radius increases, but that merely means that the electron density falls off quickly, rather than dropping off abruptly, as one proceeds to greater radii. The region of this falloff in electron density contains the fuzzy periphery.
The same is true of a surface formed of atoms. It does not resemble a cobblestoned pavement, with individual atoms as the stones. It, too, is fuzzy, as if the cobblestones had an overlay of cotton. Again, the fuzziness represents a region where the electron density is falling off sharply but is not terminating abruptly. In manipulating atoms on a surface, then, physicists work with the fuzziness, surrounding both the atoms and the surface.
This fuzziness has a thickness on the order of an angstrom (symbol Å), or 10−8 centimeters. This is a very small thickness. 10,000 times finer than the smallest details that appear in an electronic microchip. Indeed, an angstrom is as much smaller than a postage stamp as the stamp is smaller than the state of Texas. Manipulations made with atomic precision nevertheless must achieve such accuracy, and this immediately rules out any type of mechanical system with moving parts. Even if built with the care of a watchmaker, such a mechanism would amount to carrying out delicate surgery on the eye using the clanking machinery of a Mississippi steamboat. Hence, it would appear at the outset that one must accomplish the impossible: To build a movable mechanism that has no moving parts.
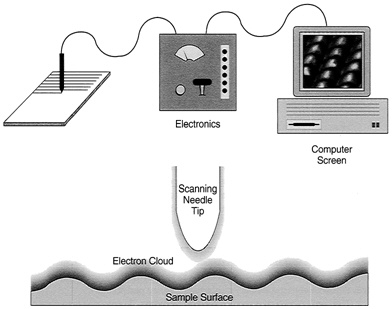
FIGURE 2.1 Principle of the scanning tunneling microscope. Bands of electronic fuzziness surround both sample surface and needle tip, leading to a flow of current when these bands overlap. Feedback control maintains the current at constant value, raising and lowering the tip while it scans across the surface. The tip scans in a raster pattern, while its vertical motion follows the contours of surface atoms. The image on the computer screen, showing these atoms, amounts to a plot of vertical needle motion during the raster scan.
The answer lies in piezoelectricity. There are materials with the property that, when squeezed or compressed, produce measurable voltages. The reverse is also true: When one applies even a slight voltage, they change length. The change can be no more than a fraction of an angstrom in a piezoelectric rod having a length measured in centimeters, but that is just what we want. A similar effect could be achieved by using rods that expand and contract in response to small temperature changes, but piezoelectric materials offer quick response with no need to wait while a rod warms or cools. Such rods then can push a sensor or manipulator across a surface, raising or lowering it within the fuzzy layer.
The sensor demands attention in its own right. It features a needle with a very sharp tip, able to probe individual atoms. A sewing needle is much too coarse; it would look to an atom like the Washington Monument. But tungsten, a very hard metal, offers tips of sufficient sharpness.
This needle, mounted in a holder or sensor head and guided by the piezoelectric rods, constitutes the essential feature of the scanning
tunneling microscope. The needle rides above the surface to be studied at a distance of 4 to 8 Å. The fuzzy layers of the surface and needle tip then just barely overlap; they overlap a good deal more strongly if the needle approaches the surface by as little as a fraction of an angstrom.
As little as 0.1 volts, applied to the piezoelectrics, can move the needle by a full angstrom. In addition, a small voltage applied to the needle causes an electric current to flow from the surface, as a consequence of the overlapping regions of fuzziness. This is called a tunneling current. It increases rapidly as the tip comes closer to the surface; if the tip comes 1 Å closer, the current goes up by a factor of 10. Atoms are typically some 3 Å in diameter, which means that, if the tip moves downward by no more than the width of an atom, the tunneling current increases as much as a thousandfold. A feedback loop, measuring this current, keeps the needle tip at a desired height above the surface (see Figures 2.1 and 2.2).
Gerd Binnig and Heinrich Rohrer, at the IBM Zurich Research Laboratory in Switzerland, built the first scanning tunneling microscope
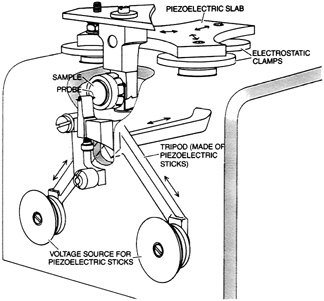
FIGURE 2.2 Example of a scanning tunneling microscope. The probe features a fine-pointed needle. The piezoelectric tripod scans the needle over the sample surface while adjusting its height to an accuracy as great as a thousandth of an atomic diameter. The piezoelectric slab and electrostatic clamps, at the top, provide coarse adjustment by moving the sample to approximately the right location. From ''The Scanning Tunneling Microscope," by G. Binnig and H. Rohrer. Copyright © 1985 by Scientific American, Inc. All rights reserved.)
in 1981. A principal problem for them was to prevent disturbances due to vibration; footfalls of people in the lab could resemble the San Francisco earthquake at atomic scale, while the vibrations of ordinary speech could resound like Big Ben tolling the hour. They succeeded, however, and at 2 a.m. one morning in mid-March, Binnig made his first observation with the new instrument. He had worked for weeks to overcome difficulties with a balky control system, but his reward came as he observed steps of atomic scale on a surface of gold. Later, observations of silicon showed bumps corresponding to single atoms, along with vertical resolutions, normal to the surface, as fine as 0.5 Å. Similar results have been seen in studies of nickel (see Figure 2.3).
The instrument that emerged from this work found quick application in making atomic-scale maps of surfaces. A common procedure involved a feedback control that would measure the tunneling current as the needle scanned over the surface, automatically adjusting the height of the needle to keep that current constant. The varying voltage fed to the vertical piezoelectric element, to adjust the needle height, then would correspond to the surface details seen while scanning. In the words of Binnig and Rohrer, "By sweeping the tip through a pattern of parallel lines a three-dimensional image of the surface is obtained. A distance of 10 centimeters on the image represents a distance of 10 Å on the surface: A magnification of 100 million." By contrast, a conventional optical microscope achieves a magnification of 2000, which is 50,000 times coarser.
"There they were, like tennis balls lying on the floor. Atoms!" recalls Calvin Quate of Stanford University, who in 1982 became one of the first American investigators to use this device. His excitement was understandable, for just after World War II the physicist Edward Teller had written an alphabet book for the nuclear age:
A is for atom. It is so small
No one has ever seen it at all.
The scanning tunneling microscope was not the first instrument to give direct views of individual atoms; using specialized techniques, physicists had seen tungsten atoms during the 1950s. But with this new microscope, physicists could see atoms at will, within a wide range of materials. Binnig and Rohrer went on to share the 1986 Nobel prize in physics for their achievement.
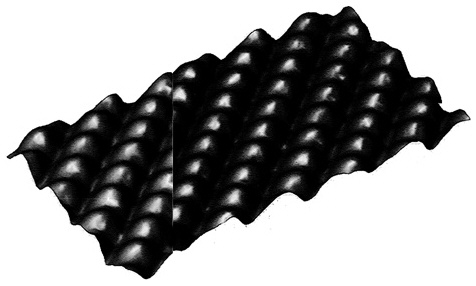
FIGURE 2.3 Surface of nickel as seen by a scanning tunneling microscope. Bumps correspond to individual atoms. (Courtesy of Donald Eigler, IBM.)
"It is quite remarkable that something like this, which you can build yourself, you don't need very fancy machines, just common stuff and a little bit of glue, fits in the palm of your hand, yet it can provide you with remarkable, unprecedented images of atoms on surfaces," says Don Eigler of the IBM Research Division's Almaden Research Center in California, another leader in this field. "It's a great set of eyes," he adds. In his hands it has also become a tool for manipulating atoms.
TOTE THAT ATOM, LIFT THAT MOLECULE
Eigler and his colleagues have operated their equipment under conditions of high vacuum and temperatures close to absolute zero. The vacuum protects their surfaces against contamination, allowing them to remain atomically clean. The low temperatures, in turn, encourage atoms to stay put rather than hopping around, as they would do if the equipment were warmer.
At the outset, Eigler faced the issue of identifying particular atoms. "They don't come with labels on them," he warns. "We start off with an atomically clean surface and we observe the mean number of defects and what kinds they are. Then we put down a lot of the atoms that we want to study, and we see that they all appear to be a particular kind. From that, we learn to identify what they look like."
An important set of experiments have involved xenon atoms on a surface of nickel. Xenon is an inert gas; it does not readily take part in chemical reactions. Still, that does not mean that its atoms lie loosely on the surface like ping-pong balls; they indeed experience forces, though these are weak. One of them is the Van der Waals force, which results when electrons surrounding an atom show greater density on one side than the other. Such shifts in charge density can result from interactions of atoms with their neighbors. A second force arises from overlap of the
fuzziness of a particular atom with that of a neighbor. This overlap means that the two atoms share electrons to a slight degree, and the force that results resembles that of a chemical bond.
Such forces come into play both in attaching a xenon atom to the underlying surface and in attracting it to a pointed tip. Eigler notes that the tip can operate in two modes: Imaging and manipulation. In the imaging mode the tip rides above the surface at a distance of several angstroms, mapping the location of xenon atoms without disturbing them. When manipulating, the tip simply goes lower; more properly, it uses the feedback system to position itself so as to receive a stronger tunneling current, which is the measure of this closeness. "That increases the magnitude of the attractive force between the atom and the tip," Eigler adds. The consequence is that the atom remains attached to the surface but can also follow the tip as it moves about. The atom does not rise from the surface but acts somewhat like a heavy steel ball that can roll, with the tip attracting and guiding it as if the tip were a magnet.
"We find that the ability to slide a xenon atom over a nickel surface is independent of both the sign and the magnitude of the electric field, the voltage, and the current," Eigler writes. "It does, however, critically depend upon the separation between the tip and the atom." This leads to a simple procedure for repositioning such atoms, one by one: Locate them using imaging; lower the tip to attract an atom of interest; move the tip as desired; and then raise the tip. The atom will then stay behind, and the low temperatures of the surface will keep it there (see Figure 2.4).
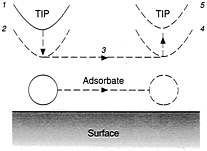
FIGURE 2.4 Dragging an atom across a surface, in five steps: (1) locate atom and place tip over it; (2) lower tip to increase its force on the atoms; (3) move tip sideways, pulling atom with it; (4) atom is now in desired position; (5) raise tip, leaving atom in place on surface. (Courtesy of Donald Eigler, IBM.)
In this fashion Eigler and his colleagues have placed such atoms on the surface as if they were lightbulbs on a theater marquee, spelling out the letters IBM. More in the nature of basic research, these researchers have studied the fundamental behavior of such atoms in small groups, carrying out observations that in no way could have previously been performed. In one of them they built a row of seven xenon atoms (see Figure 2.5). In Eigler's words,
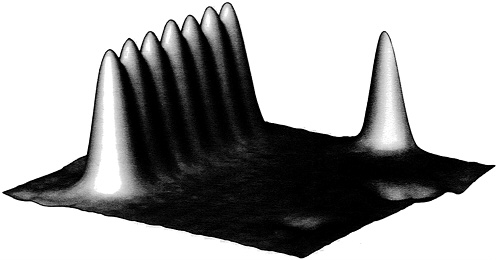
FIGURE 2.5 Scanning tunneling microscope image showing seven xenon atoms on a surface of nickel. (Courtesy of Donald Eigler, IBM.)
We observe that the apparent spacing between the xenon atoms is 5.0 ± 0.2 Å. This corresponds to just twice the spacing of atoms in the underlying nickel surface. From this we deduce that such linear chains of xenon atoms order commensurately with the underlying nickel lattice. This indicates that the in-plane xenon-nickel interaction dominates over the in-plane xenon-xenon interaction. In solid xenon the spacing between atoms is just 4.4 Å. Accordingly, attempts to make a more compact linear array of xenon atoms along the rows of nickel atoms met with failure. It was found that in order to pull a xenon atom off the end of the chain the tip had to be lowered closer to the atom than was necessary to move a lone xenon atom. Thus, we learn that the xenon-xenon interaction along the chain is attractive.
This last observation, that adjacent xenon atoms attract, does not hold as a general rule for other atoms or molecules. Molecules of carbon monoxide show no such mutual attraction when adsorbed on platinum, regardless of their separation.
This technique can reposition more than single atoms, for such atoms sometimes bind weakly to form groups, resembling a rack of billiard balls. "We've been able to drag around rafts of 12 atoms," Eigler asserts. In addition, the scanning tunneling microscope can do more. It can lift an atom from the surface and drop it in a desired location.
ATOMIC SWITCHING
The pertinent discovery dates to February 1990. To pick up a xenon atom, one holds the tip close to it and applies a pulse of +1 volts. That causes the atom to jump from the surface and attach itself to the tip. The tip then moves to a new location, and one applies a pulse of −1 volts. The atom then jumps back to the surface.
Having discovered how to do this, Eigler's group has gone on to use this arrangement as a switch of ultimately small size in which the active element consists of no more than a single atom. When the atom lies on the surface, beneath the tip, the arrangement is in a low-conductance mode. When the atom jumps to the tip, the system switches into high conductance. In a representative experiment these two states, respectively, passed a current of 1.2 × 10−8 and 9 × 10−8 amperes, repeatedly turning the high current on and off as the single xenon atom jumped back and forth between tip and surface in response to the voltage pulses (see Figure 2.6).
Eigler regards this as a proof of concept for an atom switch. Such switches, in turn, offer a possible route whereby the manipulation of atoms could win a niche in the world of technology. As people at IBM generally appreciate, miniature switches are the basic elements of computers. Transistors serve this role in today's designs, but there are
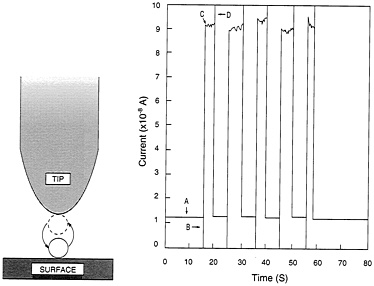
FIGURE 2.6 An atomic switch. Left, the switch operates by transferring an atom back and forth between surface and tip, through application of voltage pulses. Right, plot of current through the switch. A, low-conductance state with xenon atom on surface. B, +0.8-volt pulse transfers atom to the tip. C, resulting high-conductance state with greatly increased current flow. D, −0.8-volt pulse returns atom to the surface and reestablishes the low-conductance state. (Courtesy of Donald Eigler, IBM.)
limits to how small they can be, and switches of tinier size could in time become objects of great interest. Already, at least two research topics have grown out of Eigler's work.
At Stanford University, Thomas Albrecht, a graduate student working with Calvin Quate, has used standard techniques of microfabrication to create the tunneling microscope on a chip. Such a device could serve as an Eigler-type switch. Albrecht's instruments do not scan but rely on a sharp tip that rides at the end of a cantilever arm 1000 microns in length that is fabricated from layered aluminum and piezoelectric zinc oxide. Some 200 such devices can fit on a 3-inch silicon wafer, a standard in the electronics industry. Furthermore, because they are so small, they are not affected by vibrations from the outside world.
Eigler himself envisions that such switches could confine a movable atom within a molecular lattice, with zeolites and fullerenes being possible choices. Then there is the matter of the fine conducting connections that would carry flows of current to and from the switch, and these conductors or leads must dissipate the heat that will arise within them due to electrical resistance. "Will it work or will it fry?" Eigler asks. The question then becomes one of investigating the conducting and heat dissipation properties of leads built to nanometer dimensions, on a scale of perhaps tens of angstroms. Here, too, the scanning tunneling microscope offers help.
Chris Lutz, a colleague of Eigler at IBM's Almaden center, has used this instrument to move atoms of platinum on a surface of platinum. To do this, he had to bring the tip particularly close to the atoms so as to apply enough force to drag them across the surface. This represents a rather demanding experiment because platinum atoms would tend to bind somewhat strongly to a surface of the same material. It opens the way to working with atoms of different metals, assembled atop an insulator to produce microscopic patches. In Eigler's words, "We have the possibility someday of being able to study how electrons move through such small structures, and that's a very exciting direction for us."
LIMITATIONS OF THE TECHNIQUE
These techniques offer unparalleled opportunities to study the behavior of atoms adsorbed on surfaces individually or in small groups. In particular, a variant of the basic instrument, the atomic force microscope, measures the forces applied in moving such atoms and thus gives quantitative information. However, little in this area is easy. Atoms
sometimes are lost while being pulled to new locations, as they become trapped in defects within the atomic structure of the surface. That is somewhat like having a steel ball drop into a knothole within a wooden table. Even when this doesn't happen, there are other problems. It has not proven possible to drag an atom over the atom-size step formed by a patch of atoms previously in place on the surface. From this perspective, physicists are like the pyramid builders of ancient Egypt, puzzled as to how to slide a stone block on top of an existing layer built of such blocks.
Sometimes the basic technique fails completely. Eigler recalls trying to move oxygen atoms on a platinum surface. In his words, the result was "no luck. In order to move the oxygen, the tip had to be so close that the forces between the tip and the surrounding platinums were enough that when we moved the tip sideways, the whole surface got destroyed. There is a case where we cannot get oxygen under control." The experiment might well have set a world record for the finest line ever scribed in a metal surface, but that was not its purpose.
Even when atoms and molecules are better behaved, there are problems in working with them. In chemistry a topic of vast interest involves reactions that take place on surfaces. Many catalysts provide such surfaces within the petrochemical industry, and chemists hope to study such reactions at the level of individual atoms and molecules by using the scanning tunneling microscope. As a prelude to such work, Eigler has tried to produce a molecule of carbon dioxide by coaxing carbon monoxide into combination with an atom of oxygen.
The two species do not react spontaneously; we see this in the polluted air of our cities, where carbon monoxide in motor vehicle exhaust resists this reaction even though there is plenty of oxygen in the surrounding air. At the molecular level, there is an energy barrier whereby one must apply energy to the oxygen atom, or to the carbon monoxide, for the reaction to proceed. "We went about trying to build carbon dioxide," Eigler reports. "We could not put enough force on the carbon monoxide to make it react with the oxygen." It was not difficult to manipulate carbon monoxide molecules; they demanded less force than platinum atoms, but that wasn't enough. Eigler sees other possibilities: Bashing the reactants into each other, catalyzing with the tip, zapping with photons or with energetic electrons from the tip. So far, however, he has yet to produce his carbon dioxide.
Further limitations come into play because there is no way to accomplish precision navigation over a surface. Typically, one deals with material samples of centimeter size. Within this vastness, one produces microscopic images of regions perhaps a hundred angstroms in length
and width, or 10−6 centimeters. That is like examining in great detail a meter-size patch of soil in the rangeland of Texas with nothing more than a Rand McNally highway map to help you to find it again. Similarly, physicists have no reliable way to find their way back to a particular spot if they relocate the tip. As Calvin Quate put it, "We see things that seem so distinctive, but unless we leave the microscope just as it is, we'll never see them again."
Despite these caveats, the scanning tunneling microscope offers unparalleled vistas in the study of atoms on surfaces. Moreover, similar vistas exist in studying atoms in free space. Here the goal is to make highly precise measurements of fundamental properties. That is not possible when the atoms adhere to a surface for the surface disturbs these properties in complicated ways. As a result, atoms in empty space represent a topic in their own right.
A POSITRON NAMED PRISCILLA
As an example of what can be achieved, Hans Dehmelt of the University of Washington has carried out exquisitely precise studies of individual electrons. Electrons carry an electric charge, which allows them to respond readily to electric fields; they also respond easily to a magnetic field. This makes it possible to trap such particles and to hold them in place for long periods. Dehmelt has also trapped the positron, which amounts to an electron with positive charge. Indeed, because positrons do not exist naturally on earth, he has succeeded in showing that a particular positron under study has had no opportunity to change places with a different one. He has held that particle in place for as long as 3 months, and he has named it Priscilla. Writing in the journal Science, Dehmelt notes that "the well-defined identity of this elementary particle is something fundamentally new, which deserves to be recognized by being given a name, just as pets are given names of persons."
Working both with Priscilla and with electrons, he has made measurements of a basic quantity known as the g-factor. This is the ratio of magnetic moment to spin angular momentum. In appropriate units this quantity is a simple number; Dehmelt's value, published in 1987, is accurate to 12 decimal places:

A basic prediction of physics is that this value should be the same for both positron and electron. Dehmelt finds the ratio of values:

Hence, within the errors of measurement, the two g-factors indeed are equal to 12 significant figures. Dehmelt describes this as "the most stringent test" of this equality and hence of the underlying theory.
This theory, known as quantum electrodynamics, is among the most accurate in all of physics. It postulates that the electron exists as a simple point, having zero diameter, and Dehmelt's work makes it possible to critique this assumption. By regarding this theory as correct in all particulars, a modified g-factor whose value is exactly 2.000 can be defined. The experimental value is

Hence, within the errors of measurement, this g-factor is not exactly equal to 2.000. The difference can serve to infer an experimental value for the electron radius: 10−20 centimeters. That is some 10 million times smaller than a proton or neutron. Yet if one believes this inference, it opens the most far-ranging prospects.
Protons and neutrons, with radii of some 10−13 centimeters, most definitely are not geometric points. Indeed, they have a complex internal structure, featuring three quarks that bind together through exchanges of other particles known as gluons. This binding force, in turn, is the subject of its own physical theory, known as quantum chromodynamics. And if the electron also has a finite radius, this could mean that it too might be a composite entity, having a layer of structure below what we now study and observe. Such a structure, in turn, might apply as well to the quarks themselves, for physicists regard quarks and electrons as equally elementary.
This would amount to nothing less than a new level of detail in subatomic physics. In this century we have proceeded along a succession of steps: First atoms, then constituent particles such as protons and neutrons, then quarks. The next step, if it is real, could add a domain of subquarks that combine to yield electrons and, perhaps, the quarks we study. Moreover, if the electron indeed has a dimension of 10−20 centimeters, one can infer that the forces between such subquarks are 107 times stronger than those between the quarks in a proton or neutron. This follows from a principle of quantum mechanics: Processes that take place in diminishingly small regions require correspondingly higher energies.
THE PROBLEM OF PRECISION
These measurements, and the resulting inferences, all stem from Dehmelt's ability to trap and hold individual charged particles. In dealing with atoms, however, similar accuracies in measurement demand entirely different approaches. The basic technique for the study of atoms, in use for over a century, has been spectroscopy: The detailed observation of spectral lines. The common sodium light fixture, seen along highways, has a close relation to the usual apparatus. Here an electric current vaporizes sodium metal and adds energy to the atoms, exciting them and causing them to glow brightly at characteristic wavelengths. In this case the principal spectral lines are bright yellow in color, while other elements have lines with other wavelengths and different colors. When observed through a spectroscope, excited sodium shows those lines clearly.
The lines contain fine detail. Each of them is made up of closely spaced subsidiary lines, somewhat as a newspaper photo is actually an ordered collection of small dots. However, this detail ordinarily cannot be seen, and the reason lies in the Doppler effect. This comes into play when we hear the lonesome whistle of a passing train, rising in pitch as it approaches and then falling as the train recedes. Atoms in a hot gas are in motion, some toward us and others away from us, and their emissions of light behave similarly. They shift to higher frequencies when approaching and to lower ones when receding. The result is that spectral lines broaden or smear out, destroying their fine detail. Nor can one easily get rid of these motions; they result from the fact that the gas is hot. Indeed, their velocities measure the temperature.
Physicists can ease this problem with cleverness. Rather than confine the hot gas within a vessel, it can escape through a nozzle, forming a well-collimated beam of atoms that moves at right angles to the line of sight of the spectroscope. That gets rid of the main part of the Doppler broadening but leaves two subtle effects that also broaden spectral lines. The first stems from Einstein's theory of relativity. Atoms in the beam continue to have different velocities, and relativistic effects then dictate that they experience the passage of time at slightly different rates. This leads to slightly different frequencies in the various atoms' spectral lines, and again the result is a broadening of the lines from the atomic beam. The second effect stems from the fact that within the beam individual atoms are in view only momentarily as they fly across the field of view. Quantum mechanics then dictates that there is a corresponding uncertainty in the true frequency of any particular feature in a
spectral line. This uncertainty causes the features to blur and the lines, again, to broaden.
The use of this transverse atomic beam offers some help; the resulting line broadenings at least are generally less severe than those that arise from observing a simple hot gas, with its full-blown Doppler effect. But to study the lines in their full detail, one must contrive to get rid of the motion of the atoms. That means cooling them close to absolute zero. One cannot do this in a chamber, however; the atoms would condense on the cold walls and then would have different properties than those a physicist seeks to observe. Hence, one must chill the atoms as they fly through space. Lasers can do this, and the principal approaches rely on turning the Doppler effect from an adversary into an advantage.
LASER COOLING
The words ''laser cooling" sound like a contradiction in terms. We ordinarily think of the laser as a source of intense heat. Those of the Pentagon's "Star Wars" program, for instance, are to have sufficient energy to destroy a missile in flight. But when directed against atoms rather than missiles, a laser indeed can act to slow them down, which is the same as cooling them.
To do this, one begins by appreciating that the frequency of a spectral line represents a condition wherein atoms absorb photons of light particularly readily. Such photons, having that frequency, come from a laser tuned to the appropriate wavelength. Then, after absorbing such a photon, the atom rapidly reemits it, which puts the atom in a condition to absorb another one.
The absorbed photons all come from one direction, that of the laser beam. The reemitted photons, however, fly off in every direction. During both absorption and reemission, the atom feels a force sufficient to change its velocity; for sodium atoms this change amounts to 3 centimeters per second. The absorbed photons produce a cumulative effect, combining to slow the atoms. The reemitted photons, by contrast, lead to no more than small changes in each atom's path through space, because they produce no combined or collective effect. Here, then, is a powerful technique for slowing and cooling a beam of atoms.
Right at the outset, though, there are problems. The usual procedure tunes the laser to a frequency just below that of a spectral line and points the laser directly into the oncoming beam. Due to the Doppler shift, atoms in flight see a laser wavelength close to that of their spectral line and indeed absorb the photons quite readily. But as the atoms slow
down, two effects can break the close match between laser and spectral-line frequencies, rendering this slowing process ineffective.
The first effect applies particularly to sodium, which is a common atom used in such studies. The process of cyclic absorption and reemission of photons depends on the atom returning to a particular ground state following each photon emission. In that state an atom quickly picks up a new photon to continue the cycle. However, sodium atoms have some tendency to fall into a slightly lower ground state, associated with a different spectral line. The atoms then do not readily absorb the laser's photons and fly onward without slowing down.
The most straightforward solution is simply to use a second laser, tuned to the adjacent spectral line. Its photons then are absorbed in the usual fashion. However, at the National Institute of Standards and Technology (formerly the National Bureau of Standards), William Phillips and Harold Metcalf have introduced a different approach. They impose a magnetic field on the sodium atoms to suppress the transition to the unwanted ground state. The atoms then cycle between ground and excited states in the desired fashion, and a single laser suffices to slow them.
Still, there is a second effect that breaks the match between laser and spectral line wavelengths. This again involves the Doppler shift, for as atoms slow, this shift becomes less effective and the laser wavelength, as seen by the atoms, moves away from the desired value. The result again is that the atoms fail to absorb the oncoming photons. Again, though, both optical and magnetic methods exist to deal with this.
The optical method, introduced by V. I. Balykin at the Institute of Spectroscopy in Moscow, subjects the laser to a rapid frequency change as the atoms decelerate. This keeps the laser in tune with the spectral line as the atoms slow, with the line shifting as a result of the Doppler effect. This technique, known as "chirp cooling," produces slowed atoms in bunches. It works well with the two-laser method for avoidance of the unwanted ground state.
The magnetic method again has resulted from work by Phillips, Metcalf, and their colleagues. It relies on the Zeeman effect, whereby a magnetic field causes a spectral line to split in two. As the field increases, the two halves of the line move farther apart. This offers a means whereby a magnetic field can produce a predetermined shift in a spectral line. In practice, the field initially is strong but diminishes along the atoms' line of flight, as they slow. The atoms then stay in tune with a cooling laser of fixed frequency. This laser, in turn, yields slowed atoms in a continuous stream, rather than in bunches (see Figure 2.7).
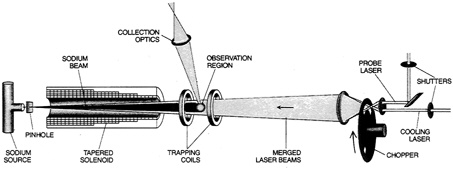
FIGURE 2.7 Layout of apparatus for use of a magnetic field in slowing and trapping sodium atoms. The atomic beam comes from the left; the laser beam, which slows the atoms, comes from the right. A tapered solenoid applies a magnetic field that diminishes with distance, imposing a Zeeman effect that adjusts the atoms' spectral-line frequency to match the constant frequency of the laser. A pair of magnetic coils then trap the atoms, which have nearly zero velocity. From "Cooling and Trapping Atoms," by W.D. Phillips and M. J. Metcalf. Copyright © 1987 by Scientific American, Inc. All rights reserved.)
Within a sodium beam, atoms initially fly at speeds of some 1000 meters per second. Since each encounter with a photon can slow such an atom by three centimeters per second, every atom must absorb and reemit some 30,000 photons to come close to a standstill. Fortunately, this cyclic process repeats so rapidly that the atoms decelerate at rates of some 106 meters per second squared, or 100,000 times greater than the acceleration due to gravity. That suffices to bring them to a stop in as little as a millisecond, along a range of only 50 centimeters.
OBSERVATIONS OF IONS
Similar techniques suffice to cool ions as well as atoms, and ions offer several advantages. Being electrically charged, it is possible to hold them for long periods by applying electric fields. Indeed, Hans Dehmelt, who has pursued his lengthy involvement with Priscilla the Positron, has similarly trapped a barium ion named Astrid. The opportunity to trap ions electrically, in turn, means that one can first trap them and then cool them, which can be easier than the cool-first, trap-later procedure with atoms. A third advantage is that ions that resist laser cooling can nevertheless reach low temperatures by storing them in a trap along with other ions that indeed respond to the laser. This technique, known as sympathetic laser cooling, treats the coolable ions as a type of ice, able to receive heat from the other ions and hence to slow their motions as well.
As an example of the measurements that then become possible, David Weinland and colleagues, at the National Institute of Standards and Technology, have carried out studies on a single positively charged
ion of mercury, Hg+. This species shows a strong spectral line with wavelength close to 2800 Å, corresponding to a frequency of some 1.07 × 1015 hertz. The natural line width is only 43 megahertz, but at room temperature Doppler effects broaden the line to 3 gigahertz, blurring out its details. Weinland, by contrast, has cooled his Hg+ ion, which has no name, to 0.006 Kelvin, or degrees above absolute zero. More recently, he claims to have reached 0.00024 K. Working with an adjacent spectral line at 2815 Å, his group has observed line widths as narrow as 30 kilohertz, some 100,000 times finer than the room temperature line. Nor is this the limit; the 2815-Å line has a natural width of as little as 1.6 hertz, which might be approached in practice using a laser with sufficient stability in its frequency.
TRAPPLING ATOMS
Atoms are electrically neutral; hence, unlike ions, one cannot trap them by using electric fields. Perhaps the simplest trap involves three sets of laser beams, oriented respectively to define x, y, and z axes and intersecting within a small region of space. These lasers are tuned to just below the frequency of a strong spectral line. An atom in motion, whatever its direction, will absorb photons more effectively and hence will experience a drag force that acts to slow it down. Indeed, provided its velocity is small, the force it feels will increase with its speed. The atom then behaves as if it were held within a viscous fluid that resists its motion. Steven Chu of Stanford University, who first demonstrated this technique while at AT&T Bell Laboratories, calls this approach "optical molasses," noting that the idea of using lasers to cool neutral atoms was first proposed by Ted Hansch and Arthur Schawlow.
A different technique takes advantage of the fact that while atoms carry no electric charge they do have electrical properties. Light, such as comes from a laser, carries a rapidly varying electric field that oscillates at its frequency. With a wavelength of 5000 Å, for instance, which is a reasonably typical value, the frequency is 6 × 1014 hertz. Electrons within an atom tend to oscillate in response to this field. The consequence is a rapidly varying redistribution of charge within the atom that indeed makes it responsive to electric forces.
This effect resembles what happens when you rub a comb with fur and use it to attract small bits of paper. Rubbing the comb gives it a charge of static electricity. The bits of paper, like atoms, carry no such charge; but when the comb approaches, it carries an electric field that redistributes the electrons within the paper. The paper then acquires an
induced charge and flies toward the comb, collecting at a place on the comb where the static charge is strongest.
In 1968 the Soviet physicist Vladilen Letokhov proposed that atoms immersed in laser light, with their rapidly varying redistribution of charges, would behave like the bits of paper. This would happen if the laser were tuned to a frequency well away from that of any spectral line; the atom's electrons then indeed would oscillate in response to the light's rapidly changing electric field. By contrast, if the laser had a spectral-line frequency, the electrons would tend to absorb and reemit photons, which would quickly push them out of the trap. Then, just as the paper bits are attracted to the strongest region of charge on the comb, the atoms feel an attraction toward the strongest or most intense region of laser light. One produces such an intense region simply by bringing the light to a sharp focus.
In practice, one locates this focus within the intersection of the sets of beams that form optical molasses. The lasers then feature two different frequencies: There are molasses beams that are close to a spectral line, along with the focused beam that is well away from such a line. The resulting arrangement can then produce a large increase in the density of trapped atoms. At AT&T Bell Laboratories, for example, molasses beams alone give a density of 106 atoms per cubic centimeter. The focused beam then raises this density a millionfold.
These techniques, however, leave something to be desired. The volume within the focus is quite small; it can be as low as a billionth of a cubic centimeter. In addition, these traps leak atoms badly. The reason is that, although they are immersed in a vacuum, a physicist's vacuum is far from perfect. It contains residual atoms that bump into the trapped ones and knock them away. Some improvement is possible; better vacuums give longer trapping times. In addition, Chu at Stanford reports creating a "super molasses" by misaligning his laser beams in a particular way. This holds particular atoms for as long as a minute, compared with storage times with conventional molasses of closer to 1 second. But in seeking long storage times together with large volumes, researchers have turned to the use of magnetic fields or a combination of magnetic and laser fields.
Atoms, even when left alone, have a small ability to act like bar magnets and to respond to a magnetic field. This effect is weak and comes into play only at vanishingly low temperatures, but such temperatures characterize laser-cooled atoms, which means that magnetic traps can operate successfully. Such a trap features two current-carrying rings, mounted like barrel hoops, with the current in one ring flowing in
a direction opposite to that of the other. This creates a magnetic field that has zero strength on the centerline midway between the two rings and that increases in all directions away from this point. An early trap of this type, built at the National Institute of Standards and Technology, held atoms within a volume the size of a golf ball. More recent traps, using stronger magnetic fields and better vacuums, have featured larger volumes. These have held atoms for a number of minutes, even hours.
THE ATOMIC FOUNTAIN
Nevertheless, whether trapped magnetically or optically, atoms are not in the pristine state that physicists would prefer to study. These trapping techniques all perturb the atoms in various ways. When caught in molasses, they are continually absorbing and reemitting photons, and their electrons are jumping up and down between energy levels. Use of the focused beam introduces rapidly varying oscillations in their electron densities. The magnetic trap, in turn, changes the spectral lines through the Zeeman effect. As a consequence, a current trend is to collect atoms in such a trap but not to study them there. Instead, a quick burst of tuned laser light can launch them out of the trap and into ballistic trajectories, within a vacuum. And just as a football has hang time when punted, these atoms have hang time within the chamber where they are available for study, away from the trap. The time for study approaches a full second if the chamber measures a meter from top to bottom. That is virtually an eternity; in principle, it suffices to permit measurement of spectral features down to line widths as narrow as 0.2 hertz. This is because longer observation times yield greater accuracy and precision.
In this fashion, Chu and his colleagues have built an atomic fountain. The apparatus collects atoms in a trap for some one-half second and then launches them upward at some 2 meters per second. Near the top of their trajectories, the instrument probes these atoms by applying two microwave pulses, separated in time by 0.25 seconds. These produce a transition between two closely spaced energy states, with the separation in time yielding superb measurement accuracy (see Figure 2.8). "In our first experiment," Chu notes, "we measured the energy difference between two states of an atom with a resolution of two parts in 100 billion." Line width was a mere 2 hertz. Furthermore, repeated observations made over 15 minutes resolved the center of the pertinent spectral line to 0.01 hertz. Current work with cesium atoms is improving the resolution still further, to as much as one part in 1015.
Chu has also gone on to develop an "atomic funnel" that produces a
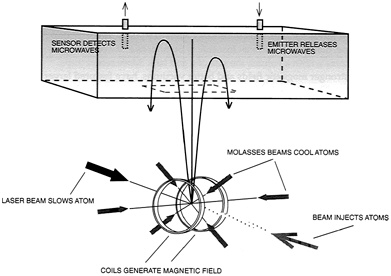
FIGURE 2.8 An atomic fountain. Atoms are held in optical molasses within a magnetic trap. A pushing beam launches them onto ballistic trajectories within a vacuum chamber. Radio frequency probes then excite the atoms from one energy state to another. (Adapted from Chu, "Laser Trapping of Neutral Particles," Scientific American, Feb. 1992.)
relatively dense beam of very slow atoms that can feed a continuous atomic fountain. "The Stanford funnel accepts atoms with a large velocity spread and cools them into a localized and collimated beam," he writes. "With our funnel, a beam of atoms was produced with a flux of 109 atoms per second at a velocity of 270 centimeters per second and a temperature of 2 × 10−4 Kelvin."
The pursuit of ever-lower temperatures represents another area of current activity. This work relies on a subtle point: It is not the absolute values of atomic velocity that define their temperature but rather the velocity spread or range of variation about an average. Hence, to achieve ultralow temperatures, it suffices to greatly reduce this spread, even if the atoms continue to show a well-defined (and uniform) velocity. This work takes advantage of today's relatively long trapping times for atoms, which makes it possible to drive their velocity spread close to zero. Working with a colleague, Mark Kasevich, Chu reports producing a collection of sodium atoms with a velocity spread of only 0.027 centimeters per second, corresponding to an effective temperature of 2.4 × 10−11 Kelvin.
SOME USES OF PRECISION
What do such measurements offer to the working physicist? Their great precision offers the prospect of new time and frequency standards that have considerable improvements in accuracy. The present time standard is an atomic clock that relies on cesium atoms. It keeps time with an error of one part in 1013, corresponding to a few ten-thousandths of a second over a human lifetime. That certainly is far better accuracy than anyone needs for ordinary purposes, but there are situations where even this is barely adequate. Tests of Einstein's relativity, for instance, demand all the precision a physicist can achieve. Now the atomic fountain offers the prospect of a thousandfold improvement, reducing the error to one part in 1016.
Chu notes that this would make it possible to determine whether the constants of physics may be changing slowly with time. These constants include the speed of light, the charge on the electron, and Planck's constant in quantum mechanics. Standard physical theories hold that they all should indeed remain invariant, but it would be useful to check. As with Dehmelt's study of the radius of the electron, such a check might show that some of our basic ideas are wrong. Chu notes that such an experiment could feature atomic clocks of very great precision that would rely on distinctly different physical principles. One clock, based on optical transitions, would have its frequency determined primarily by quantum electrodynamics (QED), while another, based on the hyperfine splitting of certain spectral lines of atoms, would be determined by a combination of QED and nuclear forces. If the strength of the nuclear forces changed relative to the electromagnetic forces, the two very precise clocks would begin to "tick" at different rates.
Other types of observation would involve novel aspects of quantum mechanics. This branch of physics predicts that an atom has wave properties similar to those of photons of light. This is different from saying that the atom absorbs photons having the right wavelength; it means that the atom itself behaves like a wave rather than as a particle. Further, its wavelength increases as the atom cools to ultralow temperatures. This opens the prospect of carrying out experiments with atoms that are done ordinarily with light.
Among these is interferometry, which demonstrates that light consists of waves. Such an experiment conventionally features two thin slits set close together, with a screen behind them. A laser shines through the slits. It does not produce shadows but yields a pattern of alternating light and dark bands on the screen, as a result of interference
effects. One can also carry out a similar experiment using a beam of electrons, which have their own wave properties. Here, too, the result is a pattern of alternating bands. Moreover, it is possible to reduce the intensity of the source of the light, or of the electron beam, so that at any moment only one photon or electron is in flight. Given time, though, the same pattern will form. It would also form using a beam of slow, and ultracold atoms.
There are several advantages to constructing an interferometer based on slowly moving atoms. For example, such an instrument can be a very sensitive inertial sensor. Chu and Kasevich already have built an interferometer that uses slow atoms and that measures the acceleration of gravity with an accuracy of at least three parts in 108. Moreover, Chu expects to achieve a further improvement of a thousandfold. The result could be an atomic standard for gravity measurements that has greatly increased precision. In turn, that could influence the highly practical matter of searching for oil. Changes in the local gravity sometimes point out oil-bearing formations to geologists, and improvements in such gravity measurements might aid the mapping of such deposits.
Ultracold atoms can also assist in opening up other fundamental topics in quantum mechanics. At sufficiently low temperatures, such atoms should undergo a phenomenon called Bose condensation. This is not the condensation that occurs when a gas freezes into a solid. Rather, it is a quantum effect whereby all the atoms would take on the same ground state. Analogs exist in a laser or in superfluid helium, where many of the particles—photons or helium atoms—are considered to be in the same quantum state. Because ultracold atoms take on wave properties akin to those of photons and electrons, they should also undergo Bose condensation. The difference is that the ultracold atoms will form a dilute Bose gas, a new state of matter that has never before been observed or studied.
APPLICATIONS IN BIOLOGY
The direct manipulation of atoms and other elementary particles, with the scanning tunneling microscope, with lasers, and by using electric and magnetic fields, thus opens a host of prospects. These include fundamental tests of basic features in physical theories, new states of matter, studies of chemical reactions at the atomic level, new instruments for the precise measurement of time and of gravity fields, and even a new approach in searching for oil. Moreover, some of the basic laser techniques used in slowing and cooling atoms are also finding
adaptation for studies of the DNA molecule, and even of bacteria and other cells.
A point of departure for this work lies in the fact that micron-size polystyrene spheres can respond to a single focused laser in the same fashion as atoms. These spheres also redistribute their internal electrons in response to the rapidly varying electric field of the laser and then move in the direction of the focus of its light, where its intensity is the strongest. As with atoms, the rule in choosing a laser frequency is simply to avoid one at which the spheres absorb light strongly. But these spheres, unlike atoms, are large enough to be visible through a light microscope. This makes it possible to integrate such a laser with this microscope and to observe the particles while manipulating them.
The basic idea, that of using a single laser beam for this purpose, is that of Arthur Ashkin, of AT&T Bell Laboratories. Chu and his colleagues call it "optical tweezers" and have been using it in studies of DNA. The DNA in a human cell is a meter in total length, yet it coils up to fit neatly within the cell's nucleus. Chu's group has begun its studies of DNA by attaching tiny spheres to each end of a strand of DNA, stretching it out to full length using a pair of optical tweezers. By measuring the force required to stretch the DNA out to a given length, they are able to compare their results to basic models of polymer elasticity. In addition, with this new-found method of manipulating the molecule, they intend to study the function of enzymes that act on the DNA.
They also have spot welded such spheres to a microscope slide, by increasing the laser power, leaving the DNA fixed to the slide and available for further research. Chu notes that these could include studies of the interaction of enzymes with DNA, including those involved with the expression of genes and with the editing out of DNA errors, which could permit the DNA to mutate into genetic nonsense. In another study of the mechanical properties of large biological molecules, Chu's group has been studying the contraction of muscle at a fundamental level. The pertinent molecule is called myosin, and these investigators have been using optical tweezers to study the force of its contraction.
Other investigators have used such tweezers to manipulate cellular organelles. Ashkin and his colleague J. B. Dziedzic have made the surprising discovery that optical tweezers can handle live bacteria and other cells without damaging them. This is possible because the cells are nearly transparent to the laser and are immersed in water, which offers an effective coolant. Indeed, Ashkin has manipulated objects within a cell without puncturing the cell wall. In a potentially important application,
Michael Berns and colleagues, at the University of California at Irvine, have manipulated chromosomes within a cell's nucleus.
WHERE DO WE STAND?
At present, the world of atomic and ion manipulations is largely one of laboratory experimentation. It is not yet possible to consult a Hewlett-Packard catalog for an atomic clock based on these principles or a gravity meter based on interferometry of ultracold atoms. Still less have such instruments served to make fundamentally new measurements or observations, which would stand as important contributions in their own right. Such an assessment even includes Dehmelt's proposal that the electron has a physical diameter and hence may possess a subquark structure. Though intriguing and potentially of great significance, such a suggestion leaves us with no way to study or examine that structure, for its energies are beyond our reach.
As the pertinent instruments and techniques enter widespread use, however, they indeed may bring forth a solid stream of significant findings. Certainly, there already is a considerable breadth to this field, both in methods and in scope for their applications. Significantly, the pertinent equipment is likely to accommodate well to working scientists with respect to size, price, and ease of use. Rather than finding concentration in some ''National Center for Atomic Manipulation," such apparatus is likely to find space on the laboratory benches of physicists, chemists, and biologists. Amid such convenience and low cost, then, these instruments and methods may cease to dazzle. Instead, they will fold into the workday world of investigators, as these people focus instead on the advances they can pursue.
BIBLIOGRAPHY
Binnig, G., and H. Rohrer. 1985. The scanning tunneling microscope. Scientific American 253(Aug.):50–56.
Bollinger, J.J., and D.J. Wineland. 1990. Microplasmas. Scientific American 262(Jan.):124–130.
Chu, S. 1991. Laser manipulation of atoms and particles. Science 253(Aug. 23): 861–866.
Chu, S. 1992. Laser trapping of neutral particles. Scientific American 266(Feb.):70–76.
Dehmelt, H. 1990. Experiments on the structure of an individual elementary particle. Science 247(Feb. 2):539–545.
Feynman, R. 1960. There's plenty of room at the bottom. Engineering and Science 22(Feb.) (Also reprinted in Miniaturization, 1961, H.D. Gilmore, ed., Reinhold Press, New York.)
Itano, W.M., J.C. Bergquist, and D.J. Wineland. 1987. Laser spectroscopy of trapped atomic ions. Science 237(Aug. 7):612–617.
Petit, C. 1989. Beyond the cutting edge. Mosaic 20(Summer):24–35.
Phillips, W.D., and H.J. Metcalf. 1987. Cooling and trapping atoms. Scientific American 256(March):50–56.
Phillips, W. D., P. L. Gould, and P. D. Lett. 1988. Cooling, stopping, and trapping atoms. Science 239(Feb. 19):877–882.