The Greening of Industrial Ecosystems. 1994.
Pp. 217-227. Washington, DC:
National Academy Press.
The Two Faces of Technology: Changing Perspectives in Design for Environment
SHELDON K. FRIEDLANDER
The tension between technology and environment has become a central political and economic issue both nationally and internationally. This issue has come to a head over the last 30 or 40 years, starting with the popular ecological works of Rachel Carson and the air pollution discoveries of Arie J. Haagen-Smit.
The British author C. P. Snow (1971) wrote cogently about what he called the "two faces" of technology, benign and threatening. "All through history it has brought blessings and curses: . . . It was true when men first made primitive tools and clambered out into the open savannah: one of the earliest uses of those tools seems to have been for homicide. It was true of the discovery of agriculture: which transformed social living, but also made some sort of organized armies practicable. It was true of the first industrial revolution. Perhaps the sharpest example of this two-faced nature of technology is the effect of medicine. . .. It has reduced infantile mortality, even in the poorest countries. . .. Yet it has led us straight into the flood of population which is the greatest danger of the next fifty years."
Snow did not refer directly to the technology-environment confrontation emerging at the time he wrote these words. However, he went on to write: "The only weapon we have to oppose the bad effects of technology is technology itself. There is no other. It is only by the rational use of technology—to control and guide what technology is doing—that we can keep any hopes of a social life more desirable than our own: or in fact of a social life which is not appalling to imagine." This paper takes up this theme as it applies to the design of engineering systems for pollution prevention.
There will have to be major changes in the way we design technological
systems—both engineering processes and products—for minimum pollution. This represents one of the greatest challenges currently facing the engineering profession. The process has barely begun, and only faint outlines of how and where we are going are visible. As a starting point, we ought to identify those engineering design procedures or paradigms that can best be adapted to the design of clean technologies. In some cases, existing procedures suitably modified will be directly applicable. An example taken from chemical engineering is given below. In other cases, it will be necessary to invent new procedures. This may well be true of important classes of consumer product design.
In what follows, extensive use is made of chemical engineering design paradigms to follow both the conversion of raw materials to chemical products and the simultaneous generation of pollutants. The chemical engineering approach makes use of material balances, chemical kinetics, thermodynamics, and transport processes to track the conversion of a set of reactants (raw materials) into a set of products. The power of the approach makes it applicable not only to chemical plants and refineries but also to power plants, microelectronics processing, aerospace factories, and other industries that make extensive use of chemical processes. It can be applied at several different but interacting scales (Figure 1). An example at the largest scale is the work of Ayres (1989) on "industrial metabolism," which makes extensive use of material balances in tracking the large-scale flow of metallic elements through various industry sectors and into the environment.
Macroscale analyses (such as "industrial metabolism" or the closely related "industrial ecology") are useful in developing national and international strategies for reducing material losses to the environment and planning future technological development. On a mesoscale—the individual chemical plant or petroleum refinery—the approach is used to design plants for the conversion of raw materials, such as crude petroleum, or a particular chemical feedstock, such as propylene, to desired products. Finally, at the microscale this approach is employed in the design of individual chemical reactors, employing differential balances on elemental reactor volumes. The three scales of organization are closely linked as shown in Figure 1.
While instructive, rates of flow of chemical species through industry and environment tell only part of the story. Chemical compound form and the physical and chemical properties of the mixtures may strongly affect public health and ecology. In addition, concentrations change by orders of magnitude during processing and environmental transport and transformation. The concentration has a determining influence on the economics of recovery and reuse (see Allen and Behmanesh, in this volume).
The extension of the chemical engineering paradigm to the formation and control of undesired chemical species—pollutants—is in some cases straightforward at the meso (manufacturing plant) and micro (chemical processes) scales. However, as discussed later in this paper, the design skills required for predicting
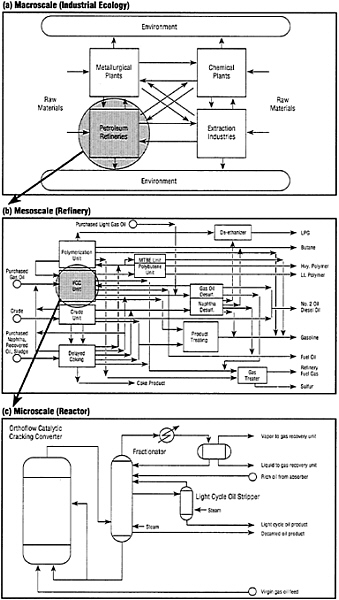
FIGURE 1
The flow and transformation of chemical substances in industry is a key feature in waste minimization or pollution prevention. Analysis of such flows can be conducted at (a) the macroscale (industrial metabolism or industrial ecology), (b) the mesoscale or individual manufacturing plant (e.g., refinery), or (c) the individual chemical reactor. Scales (b) and (c) correspond to chemical process engineering.
undesired by-products will frequently be beyond those normally applied to the high-yield conversion of raw materials to desirable products.
Changing design perspectives reflect the response of the engineering profession to a shifting national consensus toward further reducing emissions from man-made sources. The public, while still eager to share in the benefits of technology, has become much less forgiving of the associated environmental consequences. The history of this shifting national consensus is discussed in the next section, which sets the stage for the new design synthesis now under way.
HISTORICAL PERSPECTIVES: FRANKLIN AND THOREAU
The two schools of thought regarding the benefits of technology and its environmental effects can be summarized in a much simplified way as follows:
One: Technological development is fundamentally good and proceeds inexorably because it makes life better for mankind; undesirable side effects can be avoided or contained.
Two: There are limits to technological growth imposed by environmental, energy, and resource constraints; development must be slowed because of uncertainty in the side effects of technology.
The tension between these views has been reflected in the writings of some of our greatest scientists and intellectuals since colonial days. Examples are Benjamin Franklin, a leader in the development of applied science, and Henry David Thoreau, often skeptical of the benefits of technology.
Benjamin Franklin, the first great U.S. scientist, made fundamental contributions in many branches of science, especially electricity, where he introduced the concept of positive and negative charges. But he was also an applied scientist and engineer who delighted in inventing new devices to satisfy human needs.
Franklin incorporated environmental and safety concerns in the design of his inventions. He designed his stove for lower emissions and higher fuel efficiency than conventional stoves. To promote the Franklin stove, he wrote and published a pamphlet entitled "An Account of New-Invented Pennsylvania Fireplaces: Wherein their construction and manner of operation is particularly explained, their advantages above every other method of warming rooms demonstrated; and all objections that have been raised against the use of them answered and obviated."
He noted that his stove "cures most smokey chimneys, and thereby preserves both the eyes and the furniture. . .. It prevents the fouling of chimneys; much of the lint and dust that contributes to fouling must pass through the flame where it is consumed" (Franklin, 1744). Franklin had a good appreciation of the importance of designing technological systems for low emissions.
Henry David Thoreau, who came a century later, was this country's greatest proponent of the limits-to-growth, small-is-beautiful school. Thoreau was the archetypical skeptic when it came to the benefits of technology. Indeed this was a
central theme of Walden, or, Life in the Woods. Referring to the new telegraph cable, for example, he wrote, "We are eager to tunnel under the Atlantic and bring the old world some weeks nearer to the new; but per chance the first news that will leap through into the broad, flapping American ear will be that Princess Adelaide has the whooping cough."
In 1843 Thoreau reviewed a remarkable book by one J. A. Etzler, a German immigrant to the United States, called The Paradise Within the Reach of All Men, Without Labor, by Powers of Nature and Machinery : "I promise to show the means of creating a paradise within ten years, where everything desirable for human life may be had by every man in superabundance, without labor and without pay."
Etzler's idea was to develop solar "burning mirrors" to boil water and produce steam; he proposed to build huge windmills and tide mills to generate power. He foresaw large-scale desalination of water and heavy earth moving and leveling equipment. He called for mass immigration and federal subsidies for railways, canals, and power plants. "Man is powerful but in union with many. Nothing great, for the improvement of his own condition or that of his fellow men, can ever be effected by individual enterprise."
Thoreau was impressed, in spite of himself, by the breadth of Etzler's vision and his supporting calculations, However, he rejected the basic materialism and loss of individualism expressed in Etzler's work: "The chief fault of this book is that it aims to secure the greatest degree of gross comfort and pleasure merely." I emphasize the word "merely"; for Thoreau, comfort and pleasure were not the greatest good. Self-sufficiency and harmony with nature were more important.
Over the last 40 years, there has been a shift in public attitude toward what might be called the Thoreau school of skepticism regarding technological development. During that period Rachel Carson, Arie Haagen-Smit, Mario Molina, Sherwood Rowland, and others have discovered (and popularized) extraordinary and unanticipated side effects of the first order resulting from chemicals released to the environment.
Understanding these effects came from the ability to puzzle out hidden relationships between the quality of the environment and the chemical releases. Scientists were able to determine the chain of events that lead from sources of air or water pollutants to their environmental consequences. A leading role has been played by scientists trained in chemical sciences.
Photochemical smog is a case in point. The origin of photochemical smog was first elucidated in the early 1950s by Haagen-Smit, a bio-organic chemist who worked in the field of taste and odor chemistry. He was asked by the Los Angeles air pollution control authorities to apply his flavor separation techniques to determine the components in the irritating air that people in Los Angeles were complaining about. From several hundred cubic feet of air (an equivalent of the amount a person breathes in one day) he collected a few ounces of condensed liquid. This liquid, mostly water, contained foul-smelling components that he
identified as aldehydes, acids, and organic peroxides. Although these substances were known to be products of incomplete combustion, they were not known to be significant air pollutants. Their discovery in the condensates provided Haagen-Smit with the clues he needed to figure out the origin of the smog: organic compounds present in the air—mostly hydrocarbons—were oxidized through the combined action of oxides of nitrogen and sunlight to form the substances that produced eye irritation and plant and materials damage.
The sources of the hydrocarbons and nitrogen oxides were automobiles, power plants, and refineries. (Now we think there may be significant natural, or biogenic, contributions to the organic vapors.) But the original emissions themselves (primary pollutants) were not the main problem—it was the reaction products that formed later in the atmosphere (secondary pollutants) that caused severe unanticipated environmental effects. The relationships between air quality and emission sources were complex and would have been difficult to predict. Indeed this is a conspicuous characteristic of the modern age of technology/environment interactions—unanticipated side effects resulting from complex chemical and physical processes that occur in the environment. The ability to relate environmental quality to emission sources quantitatively is of central importance in the development of cost-effective methods of pollution control.
The complex relationship between chemical releases and their environmental consequences and the possibility of unexpected side effects are now recognized and feared by the public. Heightened public concern is forcing engineers to change long-established patterns of engineering design.
RETHINKING DESIGN FOR ENVIRONMENT
The unexpected environmental consequences of pollutant releases, the cost of pollution control (currently estimated to be $115 billion annually, or 2.1 percent of GNP, for the United States alone), and continuing population growth and technological development are causing engineers to rethink the basic concepts of engineering design, as they relate to environmentally compatible technologies. There is a growing trend toward waste reduction, or pollution prevention, stressing total system design (as opposed to add-on devices or end-of-pipe treatment) to reduce or avoid waste formation in industrial systems.
In the past, the design of clean technologies has generally meant the use of end-of-pipe treatment or separation devices through which effluent gases or liquids pass on their way to the environment. These devices—electrostatic precipitators, scrubbers, filters, biochemical treatment systems, etc.—are designed to meet government emission standards for particular chemical compounds. They are frequently incorporated after the main features of the technology are designed.
The case for the primacy of pollution prevention over end-of-pipe treatment rests on several factors: avoiding formation of a waste eliminates the need for treatment and disposal, both of which carry environmental risk. Control technol-
ogies may fall or fluctuate in efficiency. Treated effluent streams carry nonregulated residual substances that may turn out to be harmful as a result of unanticipated environmental processes. Secured waste disposal sites eventually discharge to the environment.
Although pollution prevention is an attractive concept, the elimination of manufacturing wastes ("zero discharge") is beyond the capability of modern technology. It is a limiting goal that has, however, been adopted by several major chemical companies. The issue is really how to approach this limiting goal in an expeditious and cost-effective manner. Moreover, we must move beyond the "low-hanging fruit," the relatively simple clean-up and housekeeping improvements that have provided much of the past success in waste reduction.
Proponents of pollution prevention (e.g., National Research Council, 1985) have made good use of case studies to illustrate the concept and its application to engineering practice. Various industry sectors have built up a broad base of such studies for practical use. Now, however, we need to find more general scientific and engineering principles on which to base a systems approach to pollution prevention. Generic approaches are of great value in engineering design and badly needed in the organization of research in support of pollution prevention. Generic approaches are essential in engineering education. Engineers are normally taught broadly applicable scientific disciplines, such as thermodynamics and fluid mechanics, which they apply to the solution of design problems.
Basic engineering principles for end-of-pipe treatment and disposal technologies are well established. For example, chemical destruction methods are commonly based on combustion and biochemical (microbial) processes. Separation technologies employ filtration, electrical precipitation, scrubbing, and other recognized physical and physicochemical processes to collect or concentrate wastes before destruction or disposal. Although many technical problems remain, a generally accepted framework exists for guiding research and development to improve the performance of waste destruction and separation technologies. Standardized procedures exist for the design of these systems.
One of the most useful basic design paradigms for pollution prevention comes out of chemical process engineering (National Research Council, 1988). Using material balances, thermodynamics, chemical kinetics, and transport phenomena, we can make very accurate estimates of the rates of conversion of reactants or raw materials to desired products in chemical processes and manufacturing plants (refineries, for example). Yields of desirable products may range from 40 or 50 percent to well over 90 percent depending on the particular process. Skilled design engineers can usually predict these yields fairly closely. Yields of undesirable by-products (pollutants) are much smaller. An example is given in Figure 2 in the form of a rank/order diagram for both desirable products and pollutants from a 50,000 barrel per day petroleum refinery. The figure is based on a composite of data gathered from a variety of sources. Figure 2 shows that undesirable by-products are extremely small relative to desired products and raw material (reac-
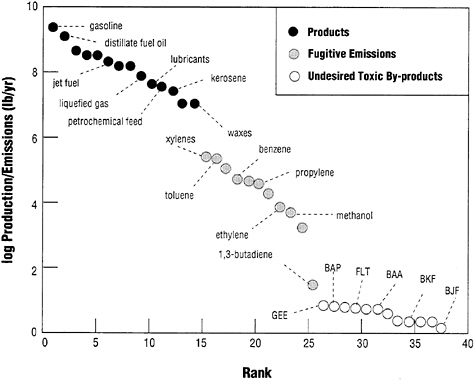
FIGURE 2
Rank/order diagram for outputs from a 50,000 barrel/day petroleum refinery. These estimates are based on composite data from several sources and should be considered illustrative. Normal chemical engineering design procedures based on material and energy balances and chemical kinetics work well in predicting the outputs of desired products. Fugitive emissions of products are difficult to estimate. Releases of pollutant by-products such as polycyclic aromatic hydrocarbons (PAHs) are usually the most difficult to measure or predict. These PAH estimates were based on a material balance using atmospheric data on PAHs and lead with information on PAH and lead emissions in automobile exhaust gases.
tant) inputs to the system. Often, we do not have a good understanding of the mechanisms by which these by-products form, and, sometimes, how they are released to the environment. As a result, the classical engineering approaches, based on material balances, are seriously limited in tracking the sources of undesired by-products. This is true at all scales of industrial organization shown in Figure 1.
All of this means that we will have to sharpen our chemical engineering design skills by several orders of magnitude to go from the raw materials (reactants) to products along pathways of minimum production of undesirable (toxic) by-products, dispersed molecularly or as fine particles. Chemical reaction engineering and recycle theory will take their place along with separation technologies as key engineering sciences for pollution control. This will require major advances
in such fields as applied chemical kinetics and in the dynamics of fine particle formation in gases and liquids. There is no shortcut around the point so well articulated by C. P. Snow in 1971: ''The only weapon we have to oppose the bad effects of technology is technology itself."
Advancing Snow's notion of "the rational use of technology" for pollution prevention will inevitably involve regulatory action (see Housman and Weinberg et al., in this volume). Finally, we need to give highest priority to engineering education, both in systematizing knowledge and in educating the next generation of engineers in pollution prevention basics.
TRENDS IN ENGINEERING EDUCATION
Industry is actively pursuing pollution prevention using case studies and pragmatic, ad hoc approaches. Academic engineering's role should be to systematize this approach to facilitate its application and to introduce it into engineering education. Design for pollution prevention should be a key feature of the education of the next generation of engineers. The concept should be introduced early in the undergraduate engineering curriculum, based where possible on the principles of engineering science. As appropriate, case studies can be used on a limited basis.
Courses related to the selection and design of manufacturing processes and products should incorporate environmental compatibility from the start, along with scientific and economic factors. As a first step, this can be accomplished by appropriate homework and design problems in existing core courses (see, for example, Allen et al., 1992). This is best done within the framework of the existing engineering disciplines. There is no need for a new branch of engineering to deal with the design of environmentally compatible technologies. Indeed, it would be self-defeating for the development of pollution prevention to fail to inculcate all engineering students with these concepts.
For the chemical process engineer, the challenge is to select raw materials and physicochemical pathways that minimize the formation of toxic by-products. The chemical process engineer with sharply enhanced skills in pollution prevention can serve in all branches of industry—not only the chemical and petroleum and related industries—as the designer of clean chemical technologies. Product engineering, particularly as it relates to the "take-back" mode being pioneered in Europe, will require a somewhat different set of skills.
Although engineers focus on technological systems, they should have a good understanding of the interaction between the technological and environmental systems at various scales of industrial organization (Figure 1). Engineers must be able to meet specialists in public health and ecology at least halfway to put together as complete a picture as possible of the environmental effects of technological systems. For this they need a good understanding of the ecological or public health basis of the regulatory standards that apply to the systems they are designing.
While engineering students are attracted by the idea of developing environmentally compatible technologies, they are also attentive to the signals sent by industry. Industry has a stake in encouraging students to acquire the skills in engineering design needed to protect the environment. Industry should make clear its commitment to the systematic incorporation of pollution prevention in engineering education through participation in the professional societies, in procedures for the accreditation of university curricula and in serving on academic engineering advisory committees.
The design of clean technologies offers the United States an opportunity to obtain a competitive advantage on world markets. The U.S. market is so large that the demands placed on processes and products to satisfy our own environmental regulations have a major impact on technology worldwide. It is in our national interest to remain the leaders in identifying existing or potential undesirable environmental effects and to develop regulations based on special U.S. geographic and demographic requirements and the political factors peculiar to our federal system and its relationship to industry. Surely U.S. industry is in a better position to respond to such regulations than foreign industry. To this end, the nation will need an engineering profession highly skilled in advanced methods for designing clean technologies, through education and basic research.
SUMMARY
Changing perspectives on the interface between technology and environment are causing engineers to rethink how they design industrial systems for environmental compatibility. This is in response to a shifting national consensus that has deep historical roots. There is a growing emphasis on system design for pollution prevention rather than end-of-pipe treatment and disposal. To this end, we seek fundamental engineering principles on which to base design, research, and education for pollution prevention. Highest priority should be given to educating the next generation of engineers in pollution prevention basics. Taking the lead in pollution prevention offers the United States an opportunity to develop an important competitive advantage given the size of its markets and its leadership in developing and setting environmental standards.
ACKNOWLEDGMENTS
I wish to thank Dr. Alan Kao, a Ralph M. Parsons Fellow in Pollution Prevention at UCLA, who helped with the preparation of the figures.
REFERENCES
Allen, D. T., N. Bakshani, and K. S. Rosselot. 1992. Pollution Prevention: Homework and Design Problems for Engineering Curricula. New York: American Institute of Chemical Engineers.
Ayres, R. U. 1989. Industrial metabolism. Pp. 23-49 in Technology and Environment, J. H. Ausubel and H. E. Sladovich, eds. Washington, D.C.: National Academy Press.
Franklin, B. (1744) in L. W. Labaree, ed. 1960. The Papers of Benjamin Franklin, v. 2, January 1, 1735 through December 31, 1744. New Haven, Conn.: Yale University Press.
National Research Council. 1985. Reducing Hazardous Waste Generation: An Evaluation and a Call for Action. Board on Environmental Studies and Toxicology. Washington, D.C.: National Academy Press.
National Research Council. 1988. Frontiers in Chemical Engineering: Research Needs and Opportunities. Board on Chemical Sciences and Technology. Washington, D.C.: National Academy Press.
Snow, C. P. 1971. Public Affairs. New York: Charles Scribner's Sons.
Thoreau, H. D. (1843) as quoted in Philip Van Doren Stern. 1970. The Annotated Walden. New York: Clarkson N. Potter, Inc.