1
National Issues
This chapter discusses some of the direct societal benefits that derive from the field of polymer science and engineering and illustrates how this specialized area can contribute to the solution of some of the pressing problems now facing the United States. In terms of commerce, polymers constitute about one-third of the U.S. chemical industry, and the same fraction describes the contribution of polymers to the chemical industry's favorable balance of payments. Polymers have become an essential and ubiquitous part of our lives: clothing, car parts, boat hulls, tennis racquets, aircraft frames, telephones, computers, human body prostheses, and numerous other material goods that we take for granted are composed largely of polymers. This widespread use is a gratifying confirmation of the success of the polymer science and engineering community, but it carries with it the need to continually focus on maintaining the research and development effort that will keep the United States at the forefront of this dynamic field.
The word "polymer" is derived from the Greek roots "poly" and "mer," which mean "many parts." Polymeric substances are composed of many chemical units called monomers, which are joined together into large molecular chains consisting of thousands of atoms. The monomers can be connected in linear chains, branched chains, or more complicated structures, each variety yielding interesting and useful properties. Most polymers are derived from petroleum and are based on the chemistry of carbon, although some polymers have noncarbon-based compounds (e.g., silicones have backbones composed of alternating silicon and oxygen atoms, with organic—carbon-containing—side groups attached to the silicon atoms).
The large size of polymer molecules is the key factor that distinguishes
polymers as a class of materials. Hydrocarbons, ranging from natural gas, to gasoline, to paraffin wax, to polyethylene, illustrate the effect of molecular size. As a consequence of molecular size alone, an enormous change in physical properties is observed—from gases, to fluids, to waxy solids, to tough and durable building materials of many uses.
Cotton, linen, hemp, wool, and natural rubber are examples of polymers that occur in nature, while synthetic (or man-made) polymers include nylon, epoxies, polyethylene, Plexiglas, Styrofoam, Kevlar®, and Teflon®. Unless otherwise indicated, the term "polymer" refers to synthetic polymers throughout this report. The term "plastics" is often used as a synonym for synthetic polymers, although some synthetic polymers are not plastic in the sense of being permanently deformable.
Not surprisingly, the applications of polymers vary widely. Given their role in such vital national concerns as economic competitiveness, transportation and energy, and defense, it is necessary to keep in view the opportunities and needs associated with polymer science and engineering, so that appropriate efforts can be made to sustain U.S. well-being and leadership. Currently, however, there are many critical issues, both financial and political. For example, industrial competition has led many corporations to cut back on long-range research in favor of more immediate bottom-line results. University research budgets are under pressure from many directions. Federal funding, in which military research and development has always been a major factor in the United States (as distinguished from other industrialized nations), is in retreat with the end of the Cold War. The need for continued investment for future benefits has never been so great, nor the prospects for such investment more threatened.
The extent to which contributions from polymer science and engineering can be brought to bear on pressing national problems depends in part on the extent to which the field can maintain its strength. Continuing strength will depend on several factors that need to be addressed:
-
Will government policy encourage a state of health in the polymer industry?
-
What level of research and development spending is necessary for the long term to enable effective competition with other nations?
-
How can funding for university, industry, and government laboratories facilitate the development of new technologies and products that will benefit society?
-
How can state-of-the-art industrial infrastructure be maintained for processing and production equipment?
-
How should production, distribution, use, and disposal of polymeric materials be managed to ensure protection of the environment and the health of the public?
RESEARCH FUNDING
Research funding for polymer science and engineering is not reported separately in most surveys. In the absence of such figures, a look at total U.S. research and development expenditures is useful. As shown in Figure 1.1, growth in expenditures for research and development from 1975 to 1985 has been followed
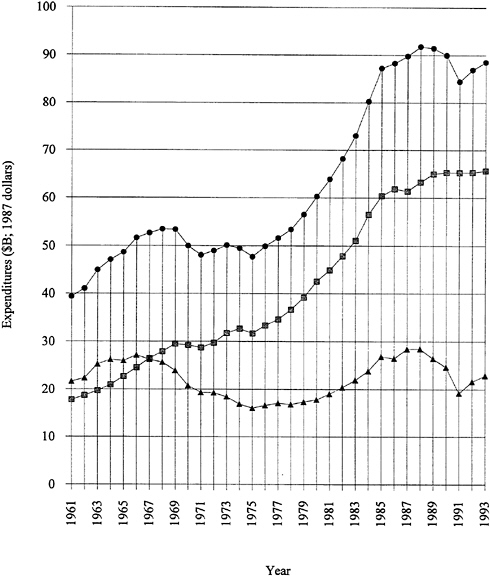
FIGURE 1.1 Expenditures for U.S. industrial R&D, 1961 to 1993. Funded by industry, shaded squares; funded by government, solid triangles; total, solid circles. SOURCE: Data from National Science Board (1993).
by several years of almost level funding. Note that Figure 1.1 is plotted in 1987 dollars; that is, inflation has been factored out. The industry-funded fraction has remained consistently strong, even during the recent recessionary period. It should be emphasized that more than 70 percent of the funded research and development is "development." Applied and basic industrial research expenditures are given in Figure 1.2. The trends are similar to those for overall industrial research and development, and the fraction of the total is 20 to 25 percent for applied research and 4 to 5 percent for basic research. The ratios among the components have remained remarkably constant over two decades. Figure 1.3 exhibits data for industry-funded research and development in universities and nonprofit institutions. University programs are likely to fall into the basic research or applied research categories. The continued growth of total U.S. research and development in difficult times is heartening.
Recent data show that U.S. expenditures in 1992 for research and development were up by 7 percent over 1991 (Business Week, 1993a) and that this strength covered a broad range of industries. Only telecommunications, metals, and fuels posted declines. Foreign expenditures for research grew by 8 percent. Details for a selected list of U.S. producers and users of polymers are given in Table 1.1. The budget figures in Table 1.2 show that funding for polymer research and development is modest (FCCSET, 1992).
Although the broad-brush statistics suggest that U.S. research and development funding is strong, closer analysis provides a darker picture. The National Science Board (NSB) has recently published a major study of U.S. industrial science and technology funding, and its conclusions are much less optimistic than those suggested by the foregoing figures (NSB, 1992). The issues are complex, and the reader is referred to the NSB document. The NSB panel concluded that
-
U.S. industrial research and development is in trouble,
-
Spending is lagging,
-
Expenditures are not well allocated, and
-
Research and development is not utilized effectively.
The committee also finds it difficult to reconcile the positive statistical evidence for research and development support by industry with committee members' direct knowledge of layoffs and large reductions in the research staffs of industrial laboratories in the early 1990s. Virtually all of the top spenders in the polymer research areas (and this includes most of the major industrial research organizations) have offered programs to reduce staff. These programs have been humanely framed as early retirement opportunities, but the result is fewer researchers. At the other end of the scale, hiring is being curtailed by industry, academia, and government. New Ph.D.s are having a difficult time finding employment. These facts seem inconsistent with reported increases in research and development budgets. Although the results of statistical surveys can lag
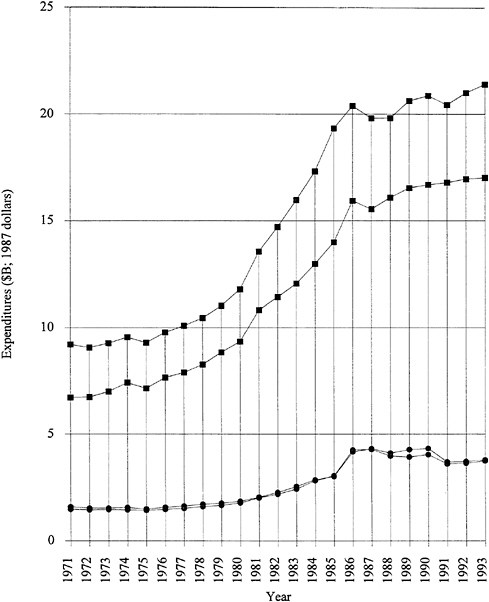
FIGURE 1.2 Applied and basic industrial research expenditures, 1971 to 1993. Applied research—industry funded, solid squares; applied research—industry performed, shaded squares; basic research—industry funded, solid circles; basic research—industry performed, shaded circles. SOURCE: Data from National Science Board (1993).
TABLE 1.1 U.S. Industry Sales and Expenditures for R&D in 1992, Selected Companies
Company |
Sales ($B) |
Expenditures for R&D ($B) |
Percentage Change in Expenditures for R&D from 1991 to 1992 |
Allied |
12 |
0.3 |
-16 |
Dow |
19 |
1.3 |
+11 |
du Pont |
37 |
1.3 |
-2 |
Eastman Kodak |
20 |
1.6 |
+6 |
Exxon |
103 |
0.6 |
-8 |
General Electric |
56 |
1.4 |
+13 |
Goodyear |
12 |
0.3 |
-1 |
Grace |
5.5 |
0.15 |
+1 |
3M |
14 |
1.0 |
+10 |
Monsanto |
7.8 |
0.65 |
+7 |
Phillips |
12 |
0.1 |
-19 |
Rohm & Haas |
3.1 |
0.2 |
+9 |
Union Carbide |
4.9 |
0.16 |
-1 |
Chrysler |
36 |
1.1 |
+10 |
Ford |
100 |
4.3 |
+16 |
GM |
131 |
5.9 |
+1 |
AT&T |
65 |
2.9 |
-7 |
IBM |
65 |
5.1 |
+2 |
SOURCE: Adapted from Business Week (1993a). |
TABLE 1.2 Advanced Materials and Processing Program: R&D Funding (then-current-year dollars) by Materials Class
|
FY 1991 Expenditure ($M) |
FY 1992 Appropriations ($M) |
FY 1993 President's Budget ($M) |
Polymers |
83 |
83 |
93 |
Bio/Biomolecular materials |
144 |
166 |
187 |
Composites |
185 |
183 |
207 |
Ceramics |
137 |
132 |
151 |
Electronic materials |
172 |
162 |
177 |
Optical and photonic materials |
143 |
133 |
139 |
TOTAL |
864 |
859 |
954 |
SOURCE: Federal Coordinating Council for Science, Engineering, and Technology (1992). |
prevailing conditions, the committee believes that the discrepancy is more complex.
Is it possible that research and development expenditures now include a broader range of categories? Shifting terminology may also be contributing to the discrepancy. Research has come to include work that is specific to a project and is not expected to reveal new concepts of broad-scale impact. Research and development productivity is increasingly measured in terms of time to market, and time horizons for projects have been progressively reduced (Business Week, 1994). This approach could have severe consequences for longer-range projects that provides for the future. Another industrial factor that is not healthy for science is the tendency to reduce central research organizations by assigning research and development people to specific product-line responsibilities. As the research staff is dispersed, the viable nucleus is destroyed. The staff becomes diffuse, and opportunities for essential cross-fertilization are lost. Documentation of these effects is difficult, but the committee is convinced of the reality of the danger to research at large and to polymer research in particular. A recent article (Business Week, 1994) comments: "U.S. industry has cut its long-term research by 15% since 1986." The article emphasizes the high rate of growth of Japanese research and development spending, especially that for basic research.
INTERNATIONAL COMPETITIVENESS
Polymers make up a major portion of the chemical industry in the United States. In 1992 the U.S. chemicals group posted exports of $44.0B and imports of $27.7B, for a positive balance of $16.3B (Chemical & Engineering News, 1993, p. 71). The corresponding figures for plastics were $10.3B and $4.3B, for a positive balance of $6.0B. (The aircraft industry is the only other major U.S. industry with a significant positive balance of trade.) This trade position is healthy and has been growing, but it may have peaked in 1991. It should be noted that U.S. chemical earnings, profit margins, and return on equity fell over the last 4 years, as markets declined in the recessionary economy (Chemical & Engineering News, 1993, p. 48). A detailed interpretation of these statistics is beyond the scope of this report, but it seems clear that the United States is strong in chemicals and in plastics and that this favorable position is in part the result of the U.S. chemical industry's consistent and vigorous commitment to research.
The United States is an acknowledged leader in the polymer markets. The 70 billion pounds of plastic and other polymeric materials produced by the United States annually represent well over half the world's output and more than double the product of the European Community (Chemical & Engineering News, 1993, pp. 44, 74, 80). However, of the world's largest chemical companies, all of which make polymers, 4 of the top 5 and 7 of the top 10 are based in foreign
countries (Chemical & Engineering News, 1993, pp. 50, 75). Further, over half of the 25 companies receiving the largest number of U.S. patents in 1991 were based outside the United States (Business Week , 1993b, p. 57). Both patterns indicate strong challenges to U.S. supremacy in the polymer area.
Another area of concern is the U.S. polymer processing industry. Film, fiber, and molded parts constitute three of the largest uses of polymers and the portion of the market that enjoys the greatest value added. However, the equipment required to process the raw polymer into these forms is produced largely in foreign countries, primarily Germany and Japan, and is then purchased by U.S. suppliers and sold under their own label. The Institut für Kunststoffverarbeitung in Aachen, Germany, for example, teaches polymer processing at all levels and develops equipment and processing methods in cooperation with the large German chemical companies. Will nationalism and regional trade advantages combine to give the large chemical companies in such countries a competitive advantage in developing new polymer applications through close association with the equipment manufacturers? Will such developments be protected by patents and thus further erode the U.S. balance of trade?
Two fields of specialty polymers—silicones and fluoroelastomers—developed rapidly after World War II under the leadership of U.S. companies. These high-performance, high-value-added materials have become standards in high-performance equipment used under extreme temperatures or harsh operating conditions. Advances in both fields have been rapid, and U.S. patent positions have been strong, thus effectively keeping foreign companies from gaining market share. But there are strong signs that foreign competition in silicones and fluoroelastomers is gaining, and the steady increase in research and development spending overseas, particularly in Germany and Japan, is serving notice that our lead in this specialty polymer field may be ending. Both Germany and Japan now spend nearly 3 percent of their gross domestic product on research and development, whereas the United States is spending about 2 percent (NSF, 1992).
What is true for silicones and fluoroelastomers is also true for composites. The last four decades have seen continuous growth in composites for a variety of uses. In the early stages, glass fiber was the material of choice because of its good characteristics and low price. As strength-to-weight ratios and stiffness became more important, new fiber reinforcement materials were needed, primarily for uses related to aviation. The ultimate choice for widespread use was carbon fiber. The early work on carbon fibers was done in the United States, but it was not long before foreign companies were also doing research in the field, and today the leading producers are outside the United States, mostly in Japan.
The foregoing competitive problems are merely examples of the difficulties facing the United States as we move toward a global economy. Certainly, every effort must be made to rejuvenate the U.S. industry for polymer processing equipment.
EDUCATION IN POLYMER SCIENCE
The subject of scientific literacy in the United States has generated considerable recent discussion. Within the scientific community, there is a strong sentiment that science education for the nonscientist is woefully inadequate, even at the college level, where few students are exposed to more than introductory courses. The general topic of science education is outside the scope of this report, but concerns about education in polymer science and engineering are closely related. The scope of the problem is large, and modifying the U.S. educational system will require a major cooperative effort over an extended period of time.
Even among most professional scientists, the past level of education in polymer science has been extremely low. This is surprising because it is estimated that about half of practicing chemists and chemical engineers work with polymers at some point in their careers. In the past, most polymer education has occurred as on-the-job training, but the situation is changing. Because of the complexity and interdisciplinary nature of modern polymer science, professionals trained in traditional academic fields cannot be immediately productive upon moving into polymer research or engineering. To fill the need for more professionals with expertise in polymers, the education of future scientists and engineers will have to be modified, and ways will have to be developed for practicing researchers to become aware of rapid progress in macromolecular synthesis, processing, and applications.
Because most students in the United States are educated in traditional areas, it is likely that the key to introducing undergraduates to polymer science will be the natural development of polymer science as an important component of these traditional areas. Exciting and innovative research becomes part of the general curriculum over time. As new applications, strategies for synthesis, theoretical methods, and characterization techniques for polymers become more accessible to faculty in traditional fields, polymer science will emerge as part of the core education in science.
The steady growth of polymers in U.S. industry has resulted in a major increase in polymer science and engineering faculty during the last 40 years. More recently, there has been a significant increase in the number of faculty identified with traditional fields who are conducting research in polymer science. This shift will likely result in the incorporation of concepts of polymer and macromolecular science into a broad range of chemistry and chemical engineering courses.
The number of academic polymer researchers in the United States and Canada can be estimated from survey data published by the Plastics Institute of America (1992), which lists 48 colleges and universities with polymer programs, of which 43 are in the United States. U.S. universities have a total of 548 faculty who are engaged in polymer research. This number is a lower limit because
many faculty doing research on polymers are not affiliated with specific polymer science and engineering programs. Even so, a large number of colleges and universities have no faculty with expertise in the polymer area.
A recent survey of the number of polymer faculty appointed from 1985 to 1991 reports that 43 such faculty have been appointed each year, on the average, with no discernible upward trend (Bikales, 1993). It is interesting to note that about half of these appointees have had previous experience in industry. This trend implies that awareness of industrial problems concerned with polymers is growing in many universities. The new faculty have been hired mostly by departments of chemistry, chemical engineering, and materials science, and, in isolated cases, other departments.
Further evidence of the growth of graduate training in polymer fields is shown in Figure 1.4. The number of doctoral degrees granted for polymer research has been steadily rising, with an average increase of about eight per year. Polymer chemistry accounts for about two-thirds of the total number of polymer graduates.
As the introduction of polymer faculty into traditional areas takes place, other actions are assisting the evolution in polymer education. Many of these changes are being fostered by professional societies.
-
Curriculum guidelines. The inclusion of polymer topics in curricula was endorsed by the 1971 and 1983 guidelines of the American Chemical Society's Committee on Professional Training (ACS-CPT). The 1988 and 1992 guidelines include polymer science and polymer science laboratory as recommended but not as required advanced courses in chemistry. Polymers are strongly recommended as a subject, both for integration into general chemistry curricula and as advanced courses, although the ACS-CPT does not require such incorporation for chemistry curricula to be accredited.
-
Course and textbook development. A major obstacle to the integration of polymer concepts and examples into existing curricula has been to the lack of polymer-related material in textbooks. A number of new textbooks now provide a chapter on polymers and other macromolecules, most often as topics in physical and general chemistry. POLYED, the joint education committee of the ACS divisions of Polymer Chemistry and Polymeric Materials, has begun a textbook project in which textbook authors and polymer faculty will be encouraged to cooperate. Course development could be facilitated by providing opportunities for interaction between polymer and nonpolymer faculty to help the latter to learn about polymers. These faculty teach most of the fundamental courses and could be encouraged to teach additional courses that have a major polymer content.
-
University faculty development. How can professors who lack formal training in polymer science enhance their knowledge base so that they can incorporate polymers into their lecture or laboratory courses? Several alternatives are
-
now available. Two- or three-week summer courses that include laboratory experience have been offered to college-level faculty for several years. In addition, the ACS's POLYED Committee has developed workshops, demonstrations, and new curricular materials.
-
Precollege faculty development. Several scientific professional organizations have developed programs for primary and secondary teachers (and sometimes their students) that are based on presentations by research scientists from different fields of interest. Some of these activities have a particular focus on polymers. One example is the two-hour polymer workshop and teaching package on polymer chemistry for pre-high school teachers and students that was developed by the National Science Teachers Association. Another program has been developed by the Intersociety Polymer Education (IPED) Task Force, representing the Society of Plastics Engineers and the Polymer Chemistry, Polymeric Materials, and Rubber divisions of the ACS. The IPED program, entitled ''Polymer Ambassadors," involves high school teachers as "ambassadors" in North Carolina, Nebraska, Missouri, and Ohio. The ambassadors take part in activities such as visits to elementary and middle school classrooms and workshops for students and teachers.
-
Continuing education. Many of the chemists and engineers employed by industrial and government organizations work with polymers, even though they received no formal training in polymer science and engineering. To provide needed professional education for this part of the work force, a variety of continuing education courses have been developed. The "Polymer Short Course Catalog" (available from the POLYED Committee of the ACS) lists 113 short courses offered by 28 universities on various aspects of polymer science and engineering. Other technical societies also offer short courses on a variety of topics relevant to polymer education. Video courses are also available. The question remains, however, whether this approach to professional education should serve as a supplement to or as a substitute for training at the postsecondary level.
-
Technical training. While the United States excels in the production of Ph.D.s in basic science and engineering, the numbers of technicians and scientific assistants trained have not been as great as in other technically advanced countries. Because many of our junior colleges and trade schools have evolved into four-year colleges and universities, there is now a shortage of institutions for educating workers at different levels of training vital to U.S. development and production efforts. The United States does have some technical high schools and two-year colleges, but many of those have fallen behind the needs of industry and do not have the personnel or facilities to adequately train workers needed to participate in polymer development and to carry out sophisticated production operations. By using Ph.D.s to carry out tasks that could be performed by well-trained technicians, the country underutilizes the talents of its highly trained
-
scientists and engineers. Such practices unnecessarily drive up research and development costs and thus jeopardize efficient production of new technologies.
It has been assumed that industry could train its workers for such tasks. However, on-site training may not be adequate to keep pace with rapid increases in technical sophistication. At the same time, the quality of science literacy among graduates from U.S. secondary schools has been compromised. The solution to this problem could involve the development and improvement of technical schools and junior colleges for those who are interested in a technical job, not necessarily a Ph.D. or M.S. position.
-
Industry-university interaction. Many polymer faculty have previous industrial experience and are aware of industrial issues. Others could benefit from enhanced mechanisms of interaction. Several methods already exist for supporting awareness of industrial research problems in the polymer field: (1) University-industry centers have been established at a number of research universities to investigate research problems of interest to the industrial partners. These centers are particularly valuable for the university partners as long as the research results can be published in a timely fashion. (2) Some industries have hired university faculty as summer researchers. This is a fruitful way to introduce college and university faculty to industrial problems. (3) Opportunities have been provided for industrial researchers to enter academic laboratories for short visits.
Strengthening the competitive position of the United States in polymer science and engineering will require meeting the following future education-related challenges:
-
Education of the general public in science, including polymers and other materials. Such an education is essential for meaningful participation in public decisions that involve scientific concepts. The familiarity of polymers makes this area a particularly accessible one in which to introduce scientific concepts.
-
Employment of more scientists and engineers with expertise and interest in polymer science and engineering as professors in colleges and universities.
-
Facilitation of exchange between academic and industrial laboratories. Polymer science research has been very strong in industrial laboratories.
ENVIRONMENT
The last few years have seen a growing national recognition that Earth's resources are finite and that we share in the responsibility for preserving a reasonable environment for future generations. Unfortunately, no clear, single path of action has been found, because environmental issues often can be solved only locally or on a short-term basis. Although the problems are of massive proportions and too little is currently known, considerable effort is being made, and
there are good prospects for progress. Certain aspects of polymer use can be beneficial in this work.
Polymers are organic materials that are usually manufactured from petroleum feedstocks using processes that are environmentally benign or that can be made more environmentally compatible without crippling economic consequences. The polymer industry has successfully adopted many environmentally favorable processes, providing advantages over competing materials as described in the vignette "Paper or Plastic?," which also touches on some of the complicated issues involved in environmental decision making.
Similar complexities are evident in comparisons of polymers with other materials, such as metals and glass. Metal smelting plumes affect the atmosphere for hundreds of miles downstream, and land damage and the disposal of tailings from mining and refining operations are serious problems. Metal refining and glass making require large amounts of energy. Thus, from an environmental standpoint, comparisons of production processes may lead to a preference for using polymers rather than metals or glass. In addition, products fabricated from polymers often weigh less than those made from competing materials. Energy savings favor plastic for bottles over glass, when the energy requirements for production, transportation, and recycling are considered. Substitution of polymers for metals in aircraft and in automobiles results in weight reductions that translate to fuel efficiencies over the life of the vehicle. These efficiencies afford important operating economies and contribute to efforts aimed at decreasing U.S. dependence on petroleum.
Natural materials are not always better than synthetic materials from an environmental point of view. A comparison of fiber production is instructive. The natural fibers cotton and wool make heavy demands on agricultural resources, including land use, fertilizer (from petroleum), and fuel for transportation. Maintenance of clothing made from synthetic fibers requires less hot water. The trade-offs are complicated, but synthetic fibers offer many advantages.
A challenge to the use of all materials is posed by their disposal after use. National concern about the use of landfills has resulted in increased emphasis on recycling programs and a reluctance to use "once-through" products that must be sent to landfills. On a volume basis, polymer waste makes up about 20 percent of current landfill input (by weight, the percentage is lower), and methods must be devised to cope with this large and growing problem. Polymers degrade very slowly in landfills, but under typical landfill conditions even paper does not degrade rapidly enough to match the rate at which it enters the landfill. Although paper and other biodegradable materials degrade much more rapidly under composting conditions, this approach is not generally feasible. Polymers can be made that are degradable at a more rapid, controlled rate, but these materials are not competitive in cost or in other properties, such as durability. Recycling of materials is environmentally attractive but in many cases has not proved to be economically viable. Frequently, products can be made with less expense from
PAPER OR PLASTIC? While your groceries are being rung up in the supermarket checkout line, the bagger will probably ask you, "Paper or plastic?" Corporations, too, face this decision when packaging their products. In November 1990 the McDonald's Corporation, largely in response to pressure from the public and from environmental groups, made the decision to replace Styrofoam "clamshell" hamburger containers with paperboard boxes. Although immensely symbolic, this decision was not necessarily the right one to minimize the environmental impact of the packaging material. When deciding which product is more environmentally friendly, the environmental consequences of all of the steps of its manufacture, use, disposal, and eventual degradation must be considered, and the results can be surprising. In many ways, the use of paper may be more polluting than the use of plastic, as several studies of their comparative life histories have shown. Paper is made from wood pulp, a renewable resource, and Styrofoam is made from nonrenewable fossil fuels. But the manufacture of a paper container consumes as much fossil fuel as goes into making a Styrofoam one, so the raw-materials toll of making a paper box includes all the hydrocarbon costs of making a plastic clamshell, plus whatever forest degradation is caused by timber harvesting. And, of course, clear-cutting and other unsound logging practices greatly increase this toll by increasing erosion and fouling streams. The manufacturing process uses other resources, too—one study estimates that manufacturing a Styrofoam clamshell uses 30 percent less energy, and generates 46 percent less air pollution and 42 percent less water pollution, than does manufacturing a paperboard box. And since paper products generally weigh more than their plastic counterparts, more fuel is needed to ship an equivalent number of paperboard boxes to the restaurant, and to pick up the used ones later. There is also the performance issue—Styrofoam is a better insulator than paperboard, so the food stays hotter longer. Food in paperboard containers will need to be reheated sooner, consuming more energy. Once the product has been used, there is the problem of disposal. Paper products that have been treated to repel grease have very low recycling value, as the coating agents interfere with the recycling process. And it has recently been discovered that paper in landfills degrades much more slowly than had been thought—newspapers thrown out in the 1950s were still readable when unearthed some 30 years later. Plastic, however, can easily be recycled into new products or used as a fuel that burns cleaner than coal or oil, thus recovering the fossil-fuel value of the petroleum in the plastic. Much of the objection to Styrofoam stems from the belief that its manufacture requires the use of chlorofluorocarbons (CFCs) that harm the ozone layer. This is no longer true, as industry stopped using CFCs in Styrofoam manufacture many years ago. In summary, replacing Styrofoam with paperboard, which on the surface seems a simple, environmentally friendly decision, involves a complex set of environmental trade-offs. So, paper or plastic? Making the correct decisions requires a comparative analysis of the environmental impacts of the life histories of the alternative products. Your decision at the supermarket is similarly complex. |
raw materials. In part, this observation may reflect our imperfect accounting systems, in which the producer bears no responsibility for the cost of disposal. Manufacturing firms are now moving to design and engineer new products with an eye to the need for recycling at the end of product life. The European automobile industry is a leader in this area, and U.S. automobile manufacturers have formed the Polymer Recycling Consortium to coordinate similiar work.
Recycling often involves the production of lower-grade products from recycled feedstocks. This degradation in quality has several sources: the recycled material may have been degraded to some extent in the first use, it can be further degraded by reprocessing, and the mixtures of polymers that are frequently formed during recycling often have seriously diminished physical properties and appearance. Success in recycling depends very much on the chemistry of the polymer involved and on the purity of the scrap. One outstanding example is polyester soda bottles, whose recycling has been aided by "bottle bills" that encourage their return in relatively uncontaminated form. Labeling of plastics at the time of manufacture has been initiated to facilitate separation, and methods of automated recognition and separation are now being sought. Factory scrap is often fed back into the feed supply to achieve an economic advantage with no sacrifice in quality. Despite considerable effort and progress, however, recycling of plastics generally poses a major unsolved problem.
Incineration is another option for disposal that can be attractive in certain circumstances. Polyolefins, for example, have high fuel value and can be burned cleanly and be disposed of with carbon dioxide and water as the only products. But other polymers pose more difficult problems for incineration. Combustion of poly(vinyl chloride), for example, produces corrosive and toxic smoke that requires complex and expensive scrubbing, followed by subsequent disposal of the scrubbings. Also, the fuel content of this polymer is lower, and net energy consumption is required. Other polymers present different scrubbing problems, and mixtures of polymers complicate incinerator operating strategies. Some progress is being made, but the advances are evolutionary. Nevertheless, until improved strategies for recycling have been implemented, incineration offers many advantages for disposal of plastics.
In recent years, there has been an active effort to invent new polymers that will have the needed properties during use but will decompose by some specific process, such as biodegradation or photodegradation, when disposed of. Some progress has been reported, but the requirements for conflicting properties means that this approach will not be applicable to every situation. Is it reasonable, for example, to hope for a high-strength fishing net that falls apart only after it has been lost or discarded? On the other hand, there may be special situations in which degradable polymers will be valuable, a good example being the use of plastic "six-pack rings" for packaging soft drink and beer cans. A plastic that degrades when exposed to sunlight for just a few weeks presents a minimal hazard to the environment. Another possible example is that of polymers based
in part on starch. These materials are often water sensitive and may offer a way to produce biodegradable polymers. Such materials would be more susceptible to composting than most synthetic polymers, but their properties appear to be limited. In principle, polymers that degrade at controlled rates are possible, but the logistics of their use can be a problem. In addition, if degradable polymers are mixed with other polymers, the resulting mixture will be less satisfactory for recycling.
HOUSING AND CONSTRUCTION
Polymers have made important inroads into the construction of housing and other buildings, despite the difficult competition presented by traditional materials—such as wood, brick, stone, concrete, glass, asphalt, plaster, slate, iron, and copper—that are economical and are entrenched in terms of aesthetics, artisans' skills, and existing U.S. building codes. The market has been penetrated by synthetic polymer products, often used in combination with traditional materials, across a broad range of applications. Demonstration houses already exist in Massachusetts and New Jersey that showcase an impressive array of uses, as pointed out in the vignette "Plastic Houses."
As the acceptance of factory-built (prefabricated) structures grows, economies of scale will arise that will strongly favor the use of polymeric materials in many areas. Complicated shapes can be fabricated economically if the costly tooling can be depreciated over large numbers of copies. Synthetics offer greater uniformity than natural materials, a factor that will grow in importance as factory production increases. Further, as synthetics continue to improve, it is likely that polymers, composites, and combinations will become superior in those properties that are valued by the consumer.
Polymers have already become dominant in fabrics for household furnishings. Carpeting, draperies, and upholstery are now usually made of synthetic polymers because of their superior properties. Substitutions are also evident in appliances such as refrigerators, coffee makers, and mixers.
Progress in the use of polymeric materials in housing will probably continue to be incremental, as each application is tested on the basis of its merits with existing materials of choice. Although societal inertia is particularly large in the construction trades and housing, the movement to increased use of polymer-based materials is unmistakable.
ENERGY AND TRANSPORTATION
The United States consumes energy out of proportion to the size of its population, and a large fraction of this energy is consumed by our extensive transportation system. While rail is still significant for shipping freight, the highway network is the defining transportation mode for the twentieth century. Automobiles
PLASTIC HOUSES The new house you buy 20 years from now could be made almost entirely of plastic. A demonstration house, built to show the feasibility of such a scheme, and to test new materials and construction methods, already exists. And the house is environmentally friendly—high-strength plastics, such as polycarbonate and acrylonitrile butadiene styrene (ABS), reclaimed from junked cars and discarded packages, were melted down and reused to construct everything from the outside walls and shingle roof to the toilet bowls. Even the concrete in the basement is 60 percent recycled plastic. On the interior, standard-sized plastic polyphenylene oxide wall panels are held to the studs with Velcro for easy access to plumbing, electrical, heating, and other utilities. Light switches and electrical outlets can be formed right into the panels. Heater ducts, molded into the panels and abutting a metallized plastic film just inside the wall's surface, turn the whole wall into a radiator. And the modular system allows panels incorporating built-in cabinets, desks, shelves, and so on to be added whenever, and wherever, they are needed. The kitchen and bathrooms feature lightweight, superdurable countertops made of concrete-plastic, an alloy of poly(ethylene terephthalate) and poly(butylene terephthalate) aggregate, finished with a silicone sealer so stains and spills wipe away. Standardized sizes, modular units, and snap-together plumbing make remodeling the kitchen as easy as rearranging the furniture. The subfloors throughout the house are blown foam tiles, made of poly(phenylene oxide), imprinted with a pattern of raised squares like a waffle iron. The channels between the squares accommodate the plumbing, and the squares themselves are like the knobs on a Lego® block, allowing items like bathtubs and cabinets to be snapped into place. The windows are actually sheets of a transparent liquid crystal sandwiched between panes of polycarbonate plastic 250 times stronger than glass. The liquid crystal turns from clear to cloudy at the flick of a switch, making curtains unnecessary for privacy. And a tinted film of a polyester liquid crystal on the windows prevents excessive heat buildup indoors and keeps carpets and furniture from fading. The parts for such houses would be manufactured in two plants. Large, centralized plants would receive the raw, recyclable plastic, grind it up, and form it into new roof, wall, floor, and foundation panel stock on a continuous-feed line. The stock would be cut into 8 by 40 foot panels and shipped to local finishing factories, where the panels would be customized according to the architects' specifications for the houses being built in the area. Bundles of finished panels, cut to size and with doors and windows already installed, would be shipped to the construction site, ready for quick assembly. The ease and economy with which such houses could be constructed may give new meaning to the term "affordable housing." |
for personal transportation and, increasingly, trucks for freight have become ever more important. Air transport dominates business travel, much long-distance personal travel, and priority parcel and freight delivery. All of these forms of transportation depend heavily on oil as the basis for fuel.
Polymers play a significant role in energy and transportation, primarily through the savings in weight that are possible as polymers replace steel and
POLYMERS IN LUBRICANTS The multi-grade oil in your car's engine is not just the gooey remains of some long-dead dinosaur sucked from the sands of Saudi Arabia. It also contains a polymer additive, usually about 1 percent in concentration, that modifies the viscosity-temperature relationship of the base oil. This esoteric-sounding bit of engineering is critical to your car's health. As those who have ever heated maple syrup for their pancakes know, the hotter a viscous liquid is, the runnier it gets. Unfortunately, what your car needs is an oil whose viscosity is essentially constant—low enough to be pumped through the engine, yet high enough to lubricate and protect the moving parts—no matter what the temperature is. But an unmodified light oil that is runny enough to pump when the engine is cold will be much too thin to lubricate it once it warms up. (Typical engine operating temperatures hover around 150°C.) Similarly, a heavy oil that lubricates well at 150°C is simply too viscous, like molasses in January, to work in a cold engine. Fortunately, polymers have come to the rescue. Even low concentrations of a polymer in a solvent—including oil—can increase its viscosity quite a bit. It is possible to make a solution whose viscosity does not change very much with temperature. The mechanism for this ability of polymer additives to provide us with multi-grade motor oils is complex; in fact, quite a few mechanisms may be operating at the same time. Some of these polymers can simultaneously be used as dispersants for water and the sulfuric acid that gets into the motor oil from burning sulfur-containing fuels. These are usually graft copolymers of an oil-soluble polymer with a water-soluble polymer. These principles say nothing about how much polymer to use, or how long its molecules should be, or what they should be made of. In practice, the chemically active environment of a hot engine, which is sufficient to break down many polymers, and the oil solubility and cost of manufacturing those polymers that can withstand a hot engine narrow the choices considerably, but there are more subtle considerations. The shearing force felt by a polymer molecule trapped between a static engine block and a plunging piston may be enough to tear the molecule apart. As the polymer gets longer, its susceptibility to shear increases, putting an upper limit on its size. However, smaller polymers have less thickening power. It takes more of a smaller polymer to produce the same increase in viscosity, and so some balance must be struck between the polymer's effect on viscosity and its length. The polymer is not the only additive, either. Other chemicals, such as dispersants, antioxidants, and wax crystal modifiers, are also needed. These additives, unfortunately, interact with the polymer to diminish its effectiveness in cold engines. For this reason, and because it is easier and cheaper to add one thing to an oil instead of several things, there is considerable interest in creating multifunctional polymers that could take over the roles of the other additives, or at least not interact with them. There is plenty of exploration left in the oil business for creative polymer chemists. |
aluminum in vehicle structures. Polymer composites are 5 to 7 times less dense than steel and 2 to 3 times less dense than aluminum. The advantages of weight reduction will continue to be most critical for automobiles and aircraft. Automobile weight is substantially in the vehicle itself, while for trucks and freight trains the weight is largely in the payload. Impressive fuel-saving reductions in the weight of automobiles over the last two decades are attributable to the substitution
of polymers and polymer composites as well as high-strength steel alloys, and much of the credit for improved fuel efficiency can be traced to the introduction of these advanced materials. Present-generation automobiles are about 10 percent polymer by weight, and the potential for further substitution is substantial.
The potential is greater yet for aircraft. For each pound saved in the weight of the airframe, the payload can be increased by a pound, and over the life of the aircraft the payback is large. Polymeric materials have made important inroads in general aviation. The airframe of the Beech Starship, for example, is almost entirely polymer and polymer composite, demonstrating the viability of polymers for all nonengine aircraft parts. Less progress has been made in commercial and military aircraft, which follow more conservative design philosophies. But the potential is evident, and extensive substitution can be predicted with confidence.
Further progress in substituting polymers in the transportation area will be based on the high strength and durability of polymers and polymer composites. Further progress in manufacturing processes, repair procedures, and failure mode control will enhance the use of polymers. There is no fundamental reason that polymeric materials cannot be introduced in all areas other than those that expose the materials to very high temperatures, such as engine parts and some aircraft surfaces. One of the best-established uses of polymers is the use of polymeric elastomers in tires, a critical application that affects safety.
Polymers are widely used in the form of foamed insulation, especially in buildings and refrigerators. Although there are concerns about fire and smoke hazards, this use will probably increase. The use of chlorofluorocarbons (CFCs) as blowing agents and foam cell fillers has largely been phased out to reduce ozone layer depletion. Polymers are also used as components in other energy devices and equipment, such as solar systems, fuel cells, batteries, and even reactors. Applications of polymers are discussed in the vignettes "Polymers in Lubricants" and "Polymers for Oil Recovery."
Polymer science and engineering will continue to have a significant impact on energy conservation and on transportation. There is further need for development of better materials having unique properties for applications in these areas. Many will be high-value-added applications, thereby justifying a significant research effort and the use of more expensive materials.
NATIONAL DEFENSE
The military uses polymers widely in diverse applications. These include clothing such as bulletproof vests and helmets, now made exclusively of such polymers as Kevlar® and Spectra®. Structures such as tents, huts, and bridges are made of polymers, as are aircraft, ground vehicles, and naval structures.
As in civilian aircraft, the use of polymers in military aircraft would reduce their weight, thereby conferring the key competitive advantage of longer range and increased payload (for armaments, ammunition, and electronic equipment).
POLYMERS FOR OIL RECOVERY We have all seen pictures of a "gusher," the spurt of black oil that sometimes erupts from an oil well when it first penetrates the oil-bearing stratum in the rock below. This oil is under great pressure and will continue to flow up the well pipe on its own accord until the pressure equalizes. Once this happens, the oil can still be pumped out, using enormous pumps that look like bobbing birds. Eventually, no more oil can be pumped, but at that point there is still plenty of oil down in the porous rock stratum. Once the pumps have run dry, the oil companies use "secondary" recovery processes—pumping water into the well to displace the oil upward, for example. But water injection does not recover most of the oil that is still down below. The water, being thin and runny, does not push the thick, viscous oil very well. Instead, the water flows around and past the oil, which stubbornly clings to the rock. On average worldwide, only about 25 percent of the oil in a stratum is recoverable by primary and secondary means, although in some cases the yield can be as high as 50 percent. Sometime around 1960, somebody suggested that adding a water-soluble polymer to the water to increase its viscosity might help it push the oil along ahead of it. Sure enough, the thickened water can no longer flow around the oil, and another 10 to 20 percent of the oil in the rock can be recovered. A partially hydrolyzed form of the polymer polyacrylamide is usually used, although xanthan gum—a biopolymer more commonly found as an additive in foods—is also employed. Other polymers are also being developed whose properties are especially designed for enhanced oil recovery. As world oil reserves are depleted, these polymers will become more and more important in the oil companies' quest to get the most from every well. |
Extension of range and payload will occur for each pound of polymer-based material that is substituted for a greater weight of aluminum or titanium. Failure of the airframe material is a significant concern, but polymer matrix and polymer-or carbon-reinforced composites offer a wide range of behavior that could provide an optimal mix of properties. The high skin temperatures that are generated at supersonic speeds present another challenge, but there are many new high-temperature matrices that, used with carbon fibers, could provide novel solutions.
The leverage obtained by using polymers is greater for ground-based military vehicles than for aircraft, because polymer-based composites are being substituted for steel, which is about 5 to 7 times as dense as the composites. Military design philosophy has been strongly influenced by the need for protective armor plates. But the advent of armor-piercing artillery shells suggests shifting to a protective strategy based on light weight for range and speed, coupled with deflection of antitank and antivehicle missiles by features of vehicle architecture, such as low-angle surfaces.
Military marine structures and vehicles are still built largely of steel, which demands heroic defenses against corrosion and very heavy (nonplaning) hull structures. Polymer-based substitution could be highly advantageous in many
respects. It is no accident that civilian boats are now manufactured almost entirely from polymer composites. The potential advantages are attractive for high-speed craft such as hydrofoils, particularly since corrosion problems would be eliminated. As the effectiveness of armor-piercing projectiles reduces the importance of bulk armor, polymer-based materials should come to the fore. Unmanned vehicles will become cheaper and more effective. The performance of shallow-draft vehicles for river and beach landing operations will be enhanced by light weight. Transport of marine (as well as land) vehicles will become faster and easier with lighter weight. Polymer-based hull coatings that afford a low-friction skin for marine vehicles could provide a military breakthrough.
Achievement of a low radar, sonar, optical, or source signature is a critical military objective. Aircraft need a low radar reflective surface. Ships and particularly submarines need a low sonar reflective surface. Land-based vehicles also need these features. Polymer-based materials can be uniquely adapted and tailored to achieve the stealth objective. This is an active but still emerging field.
REFERENCES
Bikales, N.M. 1993. ''Herman Mark's Children." Journal of Polymer Science: Polymer Symposia 75:143-150.
Business Week. 1993a. "R&D Scoreboard: In the Labs, the Fight to Spend Less, Get More." June 28, pp. 102-127.
Business Week. 1993b. "The Global Patent Race Picks Up Speed." August 9, pp. 57-58.
Business Week. 1994. "Who Says Science Has to Pay Off Fast? Japan Is Pursuing Long-Term Projects—Just As the West Backs Off." March 21, pp. 110-111.
Chemical & Engineering News. 1993. "Facts & Figures for the Chemical Industry." Vol. 71, No. 26, June 28, pp. 38-83.
Federal Coordinating Council for Science, Engineering, and Technology (FCCSET) . 1992. Advanced Materials and Processing: The Fiscal Year 1993 Program. A report by the Office of Science and Technology Policy, FCCSET Committee on Industry and Technology. (Available from Committee on Industry and Technology/COMAT, c/o National Institute of Standards and Technology, Rm. B309, Materials Building, Gaithersburg, MD 20899.)
National Science Board (NSB). 1992. The Competitive Strength of U.S. Industrial Science and Technology: Strategic Issues. A report of the NSB Committee on Industrial Support for R&D. NSB 92-138. Washington, D.C.: U.S. Government Printing Office.
National Science Board (NSB). 1993. Science & Engineering Indicators 1993. NSB 93-1. Washington, D.C.: U.S. Government Printing Office.
National Science Foundation (NSF). 1992. National Patterns of R&D Resources: 1992. NSF 92-330. Washington, D.C.: U.S. Government Printing Office.
Plastics Institute of America. 1992. 1991-1992 Directory of Polymer Science and Engineering Programs. Plastics Institute of America, Fairfield, N.J. (Available from Stevens Institute of Technology, Fairfield, N.J.)
Ries, P., and D.H. Thurgood. 1993. Summary Report 1992—Doctorate Recipients from United States Universities. Washington, D.C.: National Academy Press. (The Survey of Earned Doctorates is conducted for the following agencies of the U.S. government: National Science Foundation, U.S. Department of Education, National Institutes of Health, National Endowment for the Humanities, and U.S. Department of Agriculture.)