3
Manufacturing: Materials and Processing
Materials as a field is most commonly represented by ceramics, metals, and polymers. While noted improvements have taken place in the area of ceramics and metals, it is the field of polymers that has experienced an explosion in progress. Polymers have gone from being cheap substitutes for natural products to providing high-quality options for a wide variety of applications. Further advances and breakthroughs supporting the economy can be expected in the coming years.
Polymers are derived from petroleum, and their low cost has its roots in the abundance of the feedstock, in the ingenuity of the chemical engineers who devised the processes of manufacture, and in the economies of scale that have come with increased usage. Less than 5 percent of the petroleum barrel is used for polymers, and thus petroleum is likely to remain as the principal raw material for the indefinite future. Polymers constitute a high-value-added part of the petroleum customer base and have led to increasing international competition in the manufacture of commodity materials as well as engineering thermoplastics and specialty polymers.
Polymers are now produced in great quantity and variety. Polymers are used as film packaging, solid molded forms for automobile body parts and TV cabinets, composites for golf clubs and aircraft parts (airframe as well as interior), foams for coffee cups and refrigerator insulation, fibers for clothing and carpets, adhesives for attaching anything to anything, rubber for tires and tubing, paints and other coatings to beautify and prolong the life of other materials, and a myriad of other uses. It would be impossible to conceive of our modern world without the ubiquitous presence of polymeric materials. Polymers have become
an integral part of our society, serving sophisticated functions that improve the quality of our life.
The unique and valuable properties of polymers have their origins in the molecular composition of their long chains and in the processing that is performed in producing products. Both composition (including chemical makeup, molecular size, branching and cross-linking) and processing (affected by flow and orientation) are critical to the estimated properties of the final product. This chapter considers the various classes of polymeric materials and the technical factors that contribute to their usefulness. In spite of the impressive advances that have been made in recent years, there are still opportunities for further progress, and failure to participate in this development will consign the United States to second-class status as a nation.
MATERIALS
Structural Polymers
The familiar categories of materials called plastics, fibers, rubbers, and adhesives consist of a diverse array of synthetic and natural polymers. It is useful to consider these types of materials together under the general rubric of structural polymers because macroscopic mechanical behavior is at least a part of their function. Compared with classical structural materials like metals, the present usage represents a considerable broadening of the term. As shown in Table 3.1, man-made plastics, fibers, and rubber accounted for U.S. production of about 71 billion pounds in 1992 (Chemical & Engineering News, 1993), and production has tripled over the last 20 years. The price received by the original manufacturer ranges from roughly $0.50 to several dollars per pound, depending on the material. At $20 per barrel, crude oil costs about $0.06 per pound, and so conversion to polymers represents considerable value added. Because these materials go through several manufacturing steps before reaching the final consumer, the ultimate impact on the national economy is measured in the hundreds of billions of dollars each year.
TABLE 3.1 U.S. Production of Some Man-Made Structural Polymers, 1992
|
Pounds (billions) |
Plastics |
57.6 |
Fibers |
9.1 |
Rubber |
4.2 |
SOURCE: Data from Chemical & Engineering News (1993), p. 44. |
These materials have many different chemical and physical forms, such as cross-linked versus non-cross-linked, crystalline versus amorphous, and rubbery versus glassy. More recently, structural polymers having liquid crystalline order have become important. Structural polymers are rarely used in the pure form but often contain additives in small quantities, such as antioxidants, stabilizers, lubricants, processing aids, nucleating agents, colorants, and antistatic agents or, in larger quantities, plasticizers or fillers. There is rapid growth in the areas of blends and composites. In composites, a material of fixed shape, such as particles (filler) or fibers, is dispersed in a polymer matrix. The filler or fiber may be an inorganic material or another organic polymer. Blends (or alloys) on the other hand consist of two or more polymers mixed together and differ from composites in that the geometry of the phases is not predetermined prior to processing. Some polymers are used for many different purposes. A good example is poly(ethylene terephthalate), or PET, which was originally developed as a textile fiber. It is now used in film and tape (virtually all magnetic recording tape is based on PET), as a molding material, and as the matrix for glass-filled composites. One of its largest uses is for making bottles, especially for soft drinks. PET is also used in blends with other polymers, such as polycarbonate.
Plastics
The word "plastic" is frequently used loosely as a synonym for "polymer," but the meaning of "polymer'' is based on molecular size while "plastic" is defined in terms of deformability. Plastics are polymeric materials that are formed into a variety of three-dimensional shapes, often by molding or melt extrusion processes. They retain their shape when the deforming forces are removed, unlike some other polymers such as the elastomers, which return to their original shape. Plastics are usually categorized as thermoplastics or thermosets, depending on their thermal processing behavior.
Thermoplastics
Thermoplastics are polymers that soften and flow upon heating and become hard again when cooled. This cycle can be repeated many times, which makes reprocessing during manufacturing or recycling after consumer use possible using heat fabrication techniques such as extrusion or molding. The polymer chains in thermoplastics are linear or branched and do not become cross-linked as in the case of thermosets. While there are many different chemical types of thermoplastics, those made from only four monomers (ethylene, propylene, styrene, and vinyl chloride) account for about 90 percent of all thermoplastics produced in the United States (Figure 3.1). Of these four types, polypropylene has grown most rapidly in recent years—production has increased eightfold over the past two decades. Thermoplastic polyesters, primarily PET, are growing even more rapidly at the present time (driven mainly by

FIGURE 3.1 U.S. production of thermoplastics by type, 1990. SOURCE: Reprinted with permission from Chemical & Engineering News (1991), p. 54. Copyright© 1991 by the American Chemical Society.
packaging applications), with current sales nearly one-quarter of those for polypropylene. For the long term, the majority of commodity thermoplastics are expected to follow their traditional growth (Chemical & Engineering News, 1992), with continued opportunities for both process and product innovation. Future activities will focus strongly on recycling. In the case of PET, recycling can be accomplished by chemical depolymerization to monomers or oligomers followed by repolymerization to PET or other products. Such processes are currently in use for products that come into contact with food, while simple reprocessing is used for less critical products.
The so-called engineering thermoplastics, which include the higher-performance, more expensive polymers such as the polyacetals, polycarbonates, nylons, polyesters, polysulfones, polyetherimides, some acrylonitrile butadiene styrene (ABS) materials, and so on, have generally exhibited stronger growth than the commodity plastics (see Table 3.2). These materials generally have greater heat resistance and better mechanical properties than the less expensive commodity thermoplastics and, therefore, are used in more demanding applications, such as aircraft, automobiles, and appliances. A major area of development is
TABLE 3.2 Pounds of Selected High-volume Engineering Thermoplastics Sold in the United States, 1981 and 1991
|
Pounds (millions) |
Percentage Increase |
|
|
1981 |
1991 |
Since 1981 |
Thermoplastic polyesters |
1,230 |
2,550 |
107 |
Acrylonitrile butadiene styrene |
968 |
1,130 |
17 |
Nylon |
286 |
556 |
94 |
Polycarbonate |
242 |
601 |
148 |
Poly(phenylene oxide)-based alloys |
132 |
195 |
48 |
Polyacetal |
88 |
140 |
59 |
SOURCE: Data from Modern Plastics (1982, 1992). |
new blends or alloys of engineering plastics that are designed for specialty market products and are usually quite tough and chemical resistant. (The area of blends and alloys is reviewed separately below.) New products and advances in processes have resulted from the ring-opening polymerization of cyclic oligomers; for example, new developments in polycarbonates are particularly noteworthy. Other new products can be expected based on copolymers, and entirely new polymers are under development.
A further category sometimes referred to as high-performance engineering thermoplastics commands even higher prices for yet higher levels of performance. These include highly aromatic polymers such as poly(phenylene sulfide), several new polyamides, polysulfones, and polyetherketones. Development of new molecular structures has dominated this sector. Polymer chains with quite rigid backbones have liquid crystalline order, which offers unique structural properties as described below.
Figure 3.2 shows the major categories of use for thermoplastics. Approximately one-third are used in packaging, primarily containers and film. The data in Figure 3.2 are dominated by the huge volume of the five or so commodity thermoplastics; hence, the products with greater value based on engineering or advanced thermoplastics do not emerge in true proportion to their contribution to the national economy.
To understand the diversity of products and opportunities that is possible, it is useful to review developments that have occurred in thermoplastics based on ethylene, one of the simplest monomers possible. Commercial production of polyethylene commenced in England during the early 1940s using a free radical process operating at very high pressures (30,000 to 50,000 psi). The structure proved to be far more complex than the simple textbook repeat unit, –CH2 CH2–, would suggest (Figure 3.3). The backbone has short-and long-chain branches. The short-chain branches, typically four carbons long, interfere with the ability
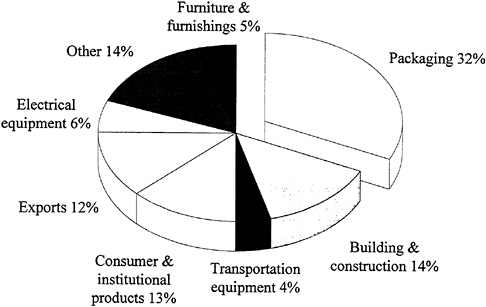
FIGURE 3.2 Categories of uses for thermoplastics in the United States, 1990. SOURCE: Reprinted with permission from Chemical & Engineering News (1991), p. 56. Copyright© 1991 by the American Chemical Society.
of the chain to crystallize, thus affecting solid-state properties, while the long-chain branches (comparable in length to the backbone itself) mainly affect melt rheological or flow properties that influence processing behavior. Because the short-chain branches reduce crystallinity and, thus, density, this material is called low-density polyethylene (LDPE). In the late 1950s, a linear or unbranched form of polyethylene was developed as a result of advances in coordination polymerization catalysis. An accidental finding by K. Ziegler in the early 1950s at the Max Planck Institute of Mulheim, Germany, resulted in a fundamentally new approach to polyolefins. It was found that transition metal complexes could catalyze the polymerization of ethylene under mild conditions to produce linear chains with more controlled structures. As a result, this polymer was more crystalline with higher density, and it became known as high-density polyethylene (HDPE). Similar catalytic procedures were used by G. Natta to produce crystalline polypropylene. The properties of this polymer are a result of unprecedented control of the stereochemistry of polymerization.
Because of the effects of molecular structure on crystallinity, HDPE is as much as 5 times stronger and 1 order of magnitude stiffer than LDPE. The newer material did not replace the older one; it was used for different purposes. In the 1970s, the high-pressure LDPE process became increasingly expensive relative to the lower-pressure HDPE process. The cost factor plus innovations in
catalysts and process technology led to a new material that had most of the attributes of LDPE but was produced by a more economical low-pressure process similar to that used for HDPE. It is a copolymer of ethylene and an alpha-olefin (like butene-1, hexene-1). Thus, short-chain branches of controlled length and number are introduced into the chain without any long-chain branches, and the material is called linear low-density polyethylene (LLDPE; see Figure 3.3). Production of this material grew at a rate of about 20 percent per year during the 1980s to current usage of about 5 × 109 pounds per year. As a result, the production of LDPE initially declined, but its production has been growing again since 1986. Construction of new high-pressure production facilities may be required in the next decade to meet demands. Currently this is the only process by which copolymers can be made with polar monomers such as vinyl acetate or acrylic acid. HDPE is fabricated primarily by molding. Blow-molded food bottles and auto gasoline tanks constitute major markets. Very large containers made by rotational molding represent a specialized growth area. A process known as "gel spinning" has been commercialized, which produces fibers of ultrahigh-molecular-weight polyethylene. The less crystalline LDPE and LLDPE are primarily extruded into film products, with each having specialized uses. New technology based on single-site metallocenes holds promise for the production of a new range of products.
This brief review of the history and future prospects for olefin polymers illustrates the need for research of all types (e.g., catalysis, process, and structural characterization) in order to capitalize on economic opportunities. These materials are complex in terms of molecular structure, and so there are many ways to tailor their behavior provided the basic knowledge and tools for structural determination are available and are integrated with innovative process technology. Much of the present research is directed toward the design of catalysts that yield materials that are easier to process. Rapid progress has resulted from an integration of catalyst synthesis and reactor and process design. As a recent example, a new polyolefin alloy product has been developed by exposing a designed catalyst to a series of different olefin monomer feeds to produce a polymer particle that is composed of polymers with different properties. Extrusion of those particles results directly in a polymer alloy.
Structural thermoplastics are a vital part of the national economy, and considerable opportunity remains for economic growth and scientific inquiry. New specialized materials will continue to offer rewards in the marketplace. At the high-performance end, several entirely new polymer structures are likely to emerge over the next decade. A major part of the growth in "new" materials will be in the area of blends or alloys. The vitality of thermoplastics cannot be judged only on the basis of the introduction of what might be called "new materials." Continuous improvement and diversification of existing polymers constitute another measure. One source estimates that the number of "grades'' of existing polymers tripled during the 1980s (Chemical & Engineering News,
1991). This trend is expected to continue but will require greater sophistication in terms of process technology, characterization, and structure-property relationships (especially modeling) than has been required in the past.
Thermosets
Thermoset materials are broadly defined as three-dimensional, chemically resistant networks, which in various technologies are referred to as gels, vulcanizates, or "cured" materials. Applications as diverse as coatings, contact lenses, and epoxy adhesives can be cited. Thermosets are defined here as rigid network materials, that is, as materials below their glass transition temperature. Thermosets are formed when polyfunctional reactants generate three-dimensional network structures via the progression of linear growth, branching, gelation, and postgelation reactions. The starting monomers must include at least some reactive functionality greater than two, which will ensure that as the reaction proceeds, the number of chain ends will increase. They will eventually interconnect to produce a gelled network material. This process may be followed by observing the viscosity increase as a function of time or from the percent reaction completed. In many cases, this can be predicted mathematically. As the gel begins to form, the soluble fraction decreases and eventually is eliminated altogether.
An important consideration with respect to rigid thermosetting networks is the extensively studied interrelationship between reactivity, gelation, and vitrification. As the reaction proceeds, the glass transition temperature rises to meet the reaction temperature, and the system vitrifies; that is, the motion of the main chain stops. At this point, the reaction essentially stops for all practical purposes. This has been conveniently described in terms of a time-temperature-transformation cure diagram. Thermosetting systems can be formed either by chain or step polymerization reactions. The chemistry of thermoset materials is even now only partially understood, because they become difficult to characterize once they reach the three-dimensional insoluble network stage. Thermal and dynamic mechanical methods have been widely used to characterize these materials, and solid-state nuclear magnetic resonance (NMR) has begun to have some impact on this problem.
Thermoset materials make up approximately 15 percent of the plastics produced in the United States. Figure 3.4 shows recent data on the production of the various types of thermosets and their uses. Phenolics make up the largest class of thermoset materials. Some polyurethanes are classified as thermosets, although many urethane and urea materials can be produced in linear thermoplastic or soluble forms, such as the well-known elastomeric spandex fibers. Urea-formaldehyde-based materials continue to be significant and, in fact, were the systems used in the first "carbonless" paper. Unsaturated polyesters are derived from maleic anhydride and propylene glycol, which are then dissolved in styrene and cross-linked into a network. They have gained significant importance in
automobiles and construction. The resulting glass-reinforced composites are frequently called sheet molding compounds (SMC).
Thermoset materials, although smaller in total volume than the thermoplastics, are used in a number of very high performance applications, such as matrix resins or structural adhesives in composite systems such as those used for aerospace applications. These composites are normally reinforced with glass, aramid, or carbon fibers. Important examples of such matrix materials include the epoxies, bismaleimides, cyanates, acetylenes, and more recently, benzocyclobutene systems. The existing database for matrix resins and structural adhesives is much more established for thermosets than it is for high-performance thermoplastics such as the poly(arylene ether ketones), certain polyaryl imides, and poly(phenylene sulfide). Major research needs in the area of polymer-based composites include better ways to improve the toughness of thermosetting systems and better techniques for processing those formed from high-performance thermoplastics.
Advances in processing and toughening thermosets are occurring on several fronts. Methods for generating the network have been investigated by many organizations. The most conventional methods involve use of a thermal-convection-oven-type curing, often in autoclaves. However, recently there has been considerable effort in electromagnetic (or microwave) processing of high-performance polymeric matrix resins, particularly for structural adhesives and composite structures. An approach for "toughening" that has been investigated over the last 10 years involves the incorporation of either rubbers or reactive engineering thermoplastics into networks, such as epoxies, to develop a complex morphology. Here the added material is dispersed as isolated domains or forms co-continuous morphologies. Most of the original studies focus on rubber toughening, and an extensive body of literature deals with utilization of carboxyl functional nitrile rubbers to toughen epoxy adhesives. More recently, advantages associated with the utilization of engineering thermoplastics have been realized. These include, for example, the ability to retain stiffness and thermo-oxidative stability, as well as in some cases, chemical resistance. These properties are often severely diminished with rubber-toughened thermosetting systems. Fracture toughness can be significantly improved. This is significant in terms of improving the durability of advanced organic materials utilized in structural adhesives and composites. The interfacial adhesion between the separate polymer phases, as well as their proportions, morphology, and molecular characteristics, is of prime significance in improving fracture toughness.
Other forefront areas include the development of new chemistries and, in particular, better characterization of leading candidate materials. The bismaleimides are considered to be somewhat more thermally stable than the epoxy materials and are being seriously considered for various applications, such as the high-speed civil transport airplane, which is planned for commercialization in the next 10 years. Aspects of the flammability of these materials are also crucial.
Aryl-phosphine-oxide-containing materials show considerable promise for producing advanced organic materials with significantly improved flammability resistance. A new development is the possibility of bridging organic and inorganic materials to produce organic-inorganic composite networks.
Elastomers
Elastomers, or rubbers, are soft and compliant polymers that are able to experience large, reversible deformations. Only long-chain polymers are capable of this type of elasticity. Elastomers are typically amorphous, network polymers with lower cross-link density than thermoset plastics. Most thermosets can be made to function as elastomers above their glass transition temperatures.
Historically, elastomers have played an important role in the industrialization, prosperity, and security of the United States. Synthetic elastomers were born of necessity during World War II, when the United States was cut off from most of its supplies of natural rubber in Southeast Asia. Low-temperature emulsion polymerizations were developed to produce highly successful synthetic rubbers, particularly styrene-butadiene copolymers. In one of the most remarkable success stories in modern industry, a production capacity of 1.5 billion pounds per year was reached in 1945. This industry continues in the United States (see Table 3.3 for production figures for various types of synthetic rubber) and in other industrialized countries. Annual production figures have declined in recent years owing to a number of factors, including advances in the use-life of tires.
Originally, all elastomers were thermosets or chemically cross-linked materials, and so their flexibility in processing, especially reprocessing or recycling, was severely limited. Thermoplastic elastomers represent a current major growth area that comprises a growing number of chemical concepts. The first materials were styrene-based block copolymers that phase separate at the molecular level to produce relatively hard polystyrene domains, which act as temporary, physical
TABLE 3.3 U.S. Production of Synthetic Rubber, 1992
|
Pounds (billions) |
Ethylene-propylene |
0.45 |
Nitrile |
0.16 |
Polybutadiene |
1.02 |
Styrene-butadiene |
1.75 |
Othera |
0.86 |
SOURCE: Date from Chemical & Engineering News (1993). a Includes, for example, butyl, polychloroprene, polyisoprene, silicone, and other synthetic elastomers. |
cross-links. The resulting elastomer is thermoplastic, and it is possible to reprocess it by simply heating it to above the glass transition temperature of polystyrene. It is thus a reprocessible elastomer. These materials are the result of the development of anionic polymerization methods, which are now practiced on a large scale in spite of the tremendous experimental difficulties associated with the organometallic initiators used in this process. Similar concepts have been implemented commercially for polyurethanes, polyesters, polyether-amides, and so on. Other versions are the so-called dynamically vulcanized blends of plastic and rubber that can be molded or extruded like thermoplastics. Current characterization techniques do not permit probing all of the potentially critical structural issues of such complex materials.
With regard to theory, there is a need for a better understanding of the topology of the network structure that is required for the recoverability exhibited by elastomers. More specifically, we need to know how to characterize entanglements and their effects on mechanical properties. Such topological features would be expected to have large effects on both equilibrium and dynamic properties, and their control could help greatly in the design of more competitive elastomers. Although the deepest insights into rubberlike elasticity will almost certainly come from molecular theories, phenomenological approaches are also frequently useful, particularly for practical purposes. These theories attempt to fit stress-strain data using a minimal number of parameters, which are then used to predict other mechanical properties. There is also a need for more experimental data on deformations other than simple elongation and swelling, which (because of their simplicity) are the ones used in the overwhelming majority of elasticity studies. One benefit would be additional, discriminating data for evaluating elasticity theories. Another would be the better understanding of the properties of elastomers for conditions under which they are frequently used. An understanding of segmental orientation of chains in deformed networks is essential for an understanding of strain-induced crystallization. Such crystallization greatly enhances the mechanical properties of an elastomer, and its control could be of considerable competitive advantage. Advances in theory, as well as additional experiments, are required for progress in this area.
There is increasing interest in the study of elastomers that also exhibit mesomorphic behavior, from liquid crystalline entities either in the chain backbone or in the side chains. These materials combine some of the most intriguing properties of liquid crystalline molecules of low molecular weight with the elastomeric properties of polymeric networks. Materials with this unique behavior should be exploited. An example is the orientation of an anisotropic phase by a mechanical force, in analogy to the use of electric or magnetic fields on low-molecular-weight mesogens.
A new subject in the area of rubberlike elasticity is the phenomenon of gel collapse, in which a swollen network abruptly deswells (shrinks) in response to a relatively small change in its environment. The collapse can be triggered by
changes in temperature, pH, ionic strength, or solvent composition. In films and fibers the collapse is rapid enough for these systems to have potential applications as mechanical switches, artificial muscle, mechanochemical engines, and so on. Exploiting this new development will require advances in theory, as well as additional experiments in actual functioning devices.
A particularly challenging problem is the development of a more quantitative molecular understanding of the effects of filler particles, in particular carbon black in natural rubber and silica in siloxane polymers. Such fillers provide tremendous reinforcement in elastomers in general, and how they do this is still poorly comprehended. Certainly the bonding between the reinforcing phase and the elastomeric matrix is critical. Investigation of the bonding between the biopolymer elastin and the collagen fibers that are threaded through it for reinforcement could provide valuable insights into this problem. A related problem exists in the hybrid organic-inorganic composites.
Finally, there is a need for more high-performance elastomers, which remain elastomeric to very low temperatures but are relatively stable at very high temperatures and resist hostile environments. The elastomeric ethylene-propylene-diene monomer (EPDM) rubbers, made by copolymerization of ethylene, propylene, and a diene using Ziegler catalysts, are particularly resistant to ozone. The polysiloxanes are one of the most important classes of high-performance elastomers and are being developed and improved in most industrialized countries. The fluoroelastomers are another class that is under intense development. Polyphosphazenes have rather low glass transition temperatures in spite of the fact that the skeletal bonds of the chains are thought to have some double-bond character. There are, thus, a number of interesting problems related to the elastomeric behavior of these unusual semi-inorganic polymers.
Fibers
A fiber may be defined as a structure whose length is much greater than its cross-sectional dimension. The diameter of fibers is characterized in dTex, a unit of linear density corresponding to the weight in grams of a 10,000-meter length of the fiber (the same units are used to describe individual filaments and multifilament yarns). Typical filament dTex values run from 1 to 10. One gram of a 1-dTex filament is over 5 miles in length.
As shown in Table 3.4, fiber production in the United States is on the order of 9 billion pounds annually. The value of the U.S. fiber industry is in the range of tens of billions of dollars, an amount that is multiplied manyfold by the time the fibers reach the consumer market in products ranging from ropes to textiles to automobile tires. Some typical applications of fiber are listed in Table 3.5.
Over the past several decades, a number of trends have become evident in the commodity fiber business:
TABLE 3.4 U.S. Production of Synthetic Fibers, 1992
|
Pounds (billions) |
Acetate |
0.50 |
Acrylic |
0.44 |
Nylon |
2.55 |
Olefin |
1.99 |
Polyester |
3.58 |
SOURCE: Data from Chemical & Engineering News (1993). |
TABLE 3.5 Typical Applications of Fiber
Use |
Polymer |
Clothing |
Polyester, nylon, polypropylene, acrylics, spandex |
Carpets |
Nylon, polypropylene, polyester |
Protective garments |
Aramids, fluoropolymers, polyethylene |
Synthetic paper |
Polyethylene |
Ropes, cables |
Polypropylene, nylon, aramids |
Golf shafts, fishing rods |
Carbon, aramids |
Cement reinforcement |
Polyethylene |
Brake linings |
Aramids, acrylics |
-
Synthetic fiber volumes have grown at the expense of natural fibers. The drivers are lower costs and technical improvements, which allow the synthetics to emulate desirable natural fiber aesthetics while exhibiting superior in-use performance.
-
The commodity markets are divided primarily among nylon, polyester, and polyolefin, with polyester emerging as the largest. Cost-performance and environmental considerations have led to a diminution in the use of cellulosics and acrylics.
-
The introduction of a new commodity fiber is generally regarded as unlikely.
This same time period has seen the rapid growth of high-performance fiber technologies. These technologies fall into three classes:
-
High-modulus, high-strength fibers based on rodlike, liquid crystalline (nematogenic) polymers. The most common examples are the lyotropic aramids and the thermotropic polyesters. These fibers are characterized by tensile moduli greater than 70 gigapascals (GPa), tensile strengths on the order of 3 to 4 GPa, and low properties in compression or shear.
-
Morphological manipulation of conventional polymers, such as high-molecular-weight
-
polyethylene or poly(vinyl alcohol), to achieve fibers with levels of mechanical performance similar to those of the liquid crystalline polymer fibers.
-
Polymeric precursor fibers that can be converted to other chemical forms after spinning. The most common examples are acrylic fibers that can be converted to carbon fibers and a variety of silicon-containing polymeric fibers that can be converted to silicon carbide or silicon nitride fibers.
Typical applications of high-performance fibers are composite reinforcement, ropes and cables, and antiballistic clothing. As a group, these fibers represent successful technical developments, but they have proved less commercially attractive than once believed for a variety of reasons.
The spinning process can be described as follows. A polymer is first converted to a liquid through melting or dissolution, and the liquid is then continuously forced through a spinnerette (a plate with many of small holes) to form filaments. Most polymeric fibers are semicrystalline. If the polymer forms a stable melt, the process is called melt spinning. For polymers that degrade prior to melting, the polymer is spun from a solution; if the solvent is evaporated, the process is termed dry spinning; if the solution is coagulated in a nonsolvent bath, the process is termed wet spinning. Removal of the spinnerette from the wet spinning coagulation bath is the innovation known as dry-jet wet spinning. In practice, the spinnerette holes are usually much larger than the desired final filament diameter; hence the filaments are stretched either during and/or after the spinning process. The ratio of final filament velocity to the initial filament velocity is termed the drawdown ratio. The principal parameters controlling the as-spun structure and, hence, properties of the as-spun filament are the rate of cooling and the applied stress. These parameters control the extent of molecular chain orientation (time to orient/time to relax) and the degree of crystallinity achieved during spinning. Crystallinity once formed can be further oriented by stretching and perfected through annealing. Key structural elements are the amount and orientation of crystalline regions, the orientation of noncrystalline regions, and connectivity between regions, tie molecules, and so on.
Careful control of the sequence in which chains are oriented and crystallized has a profound effect on the microstructure produced. Such controlled processing allows, for example, the decoupling of crystalline and noncrystalline orientation, enabling fibers with high tensile modulus (correlated with high crystalline orientation) and low thermal shrinkage (correlated with low noncrystalline orientation) to be produced. Typical spinning speeds are thousands of meters per minute, typical melt drawdowns are on the order of 100, and typical solid-state draw ratios range from about 2 to 6 in conventional processing to greater than 50 in the production of certain high-performance products. High-performance fiber processing is characterized by maximizing axial chain orientation and minimizing
structural defects formed during spinning, giving rise to mechanical performance that approaches theoretical limits. To control friction and static behavior in subsequent processing, a variety of oils or other surface treatments are applied to the fibers prior to take-up. The many complex processing steps of fibers add to the stress-temperature history of the fiber and hence significantly modify the end-use properties of the material.
To a large extent, the conditions employed in spinning, in addition to the particular chemistry of the polymer being spun, determine the end-use performance of a fiber. Work on future fibers will focus on producing cost-performance improvements and product variants through morphological control rather than new chemistries. With the huge lengths of fibers produced, process robustness and property uniformity have always been major issues; future products will make more use of advanced computerized process control and will operate in areas of property response that are less sensitive to minor process variation. Elimination of downstream process steps will lead to additional cost-performance improvements, for example, on-line texturing and surface modifications to meet specific friction or adhesion requirements.
Environmental considerations will influence future fiber developments in a number of areas. The elimination of solvent-based processing will be driven by stricter emissions standards, as will the elimination of heavy metal catalysis. Novel processes based on very fast melting techniques (e.g., RF heating or lasers) or the use of supercritical carbon dioxide as a polymerization and/or spinning solvent will become more commercially attractive. The reduction of off-specification production will become more important as the cost of waste disposal increases and as easy-to-reclaim fibers grow in importance (e.g., biodegradable cellulosics produced without organic solvents, or poly(ethylene terephthalate) reduced to usable monomers in a process that has become a commercial reality).
The future of high-performance fibers lies in the reduction of costs and the improvement of utilization. The former is best influenced by lower-cost monomers, and the latter through the development of manufacturing technologies that allow cost-effective part production from fiber-reinforced composites. High-performance fiber development will cease to be solely performance driven and will, as in the case of all other fibers, become driven by cost and performance. Silks, produced by worms and spiders, have attracted attention because they possess tensile properties similar to those of high-performance synthetic fibers but with much higher toughness. The use of recombinant DNA techniques allows silks of specific molecular architectures to be produced and their performance to be correlated with specific chemical and physical features. The increased structure-property insights gained from these studies should allow the definition of biomimetic fibers, based on other than naturally occurring amino acids, with greatly improved performance characteristics.
Adhesives
An adhesive is a material that, by means of surface attachment, can hold together solid materials. Adhesives have been used for most of recorded history. They are mentioned in Egyptian hieroglyphics, in the Bible, and in the writings of the early natural philosophers. The physical strength of an assembly made by the use of adhesives, known as an adhesive joint, is due partly to the forces of adhesion, but primarily to the cohesive strength of the polymeric materials used to formulate the adhesive. Thus, the range of strengths available in adhesive joints is limited to the strengths of the polymers useful in the formulation of adhesives. Indeed, the technology of adhesives tracks well with the technology of polymers. As new polymers were synthesized, new adhesives were developed that used those polymers.
Adhesives are typically classified by their use or application. Thus structural adhesives are those materials used to join engineering materials such as metals, wood, and composites. Usually, it is expected that an adhesive joint made with a structural adhesive is capable of sustaining a stress load of 1,000 psi (6.9 MPa) for extended periods of time. Hot melt adhesives are those adhesives that are applied from the melt and whose properties are attained when the adhesive solidifies. Pressure-sensitive adhesives provide adherence and strength with only finger pressure during application. Adhesive tapes are manufactured by applying a pressure-sensitive adhesive to a backing. Rubber-based adhesives are, as the name implies, based on elastomers and are usually applied as a mastic or spray applied from solvent or water. Pressure-sensitive adhesives can be considered to be a subset of rubber-based adhesives.
The ease of application of pressure-sensitive adhesives is superior to all other types of adhesives except possibly hot melt adhesives. Responsivity to finger pressure alone forming a bond is a desirable property, and pressure-sensitive adhesives of sufficient strength to perform structural tasks have been developed recently. One of the major uses of these double-coated foam tapes is to fasten most of the exterior and interior decorative and semistructural materials to the body of an automobile. The use of these foam tapes allows faster assembly and eliminates mechanical fasteners, which are a source of corrosion.
Each of the major classes of adhesives described above can be further classified by its chemistry. Thus, the majority of structural adhesives are based on one or more of the following chemistries: phenolic, epoxy, acrylic, bismaleimide, imide, and protein (derived from blood, soybean, casein, and so on). The majority of hot melt adhesives are based on one or more of the following chemistries: waxy hydrocarbons, polyethylene, polypropylene, ethylene-vinyl acetate, polyamides, and polyesters. Rubber-based adhesives are, for the most part, formulated using neoprene, nitrile, and natural rubbers. Pressure-sensitive adhesives are based on natural rubber, vinyl ethers, acrylics, silicones, and isoprene-styrene block co-polymers. Many paper-binding adhesives are based on dextrin or other
starch-based materials. ''White glue," used for wood bonding, is a poly(vinyl acetate) emulsion.
Adhesives have several advantages over other joining technologies. In general, adhesives have a lower density than mechanical fasteners, and so weight savings can be realized. Polymer-based adhesives have viscoelastic character and are thus capable of energy absorption. The energy absorption manifests itself in the form of dampening of vibrations and in the increase of fatigue resistance of a joint. Adhesives can be used to join electrochemically dissimilar materials and provide a corrosion-resistant joint. Adhesive joining is limited by the fact that an engineering database is unavailable for most adhesive materials.
The strength and durability of an adhesive bond are subject to the nature of the surfaces to be joined. Part of the reason industrial adhesives have been so successful is that methods have been found to clean and treat surfaces to form good bonds. A better understanding of proper surface preparation for adhesives is needed. The major limitations to the broader use of adhesives in industry are the extreme sensitivity of adhesive bonding to surface conditions and the lack of a nondestructive quality control method.
Adhesive technology can be solidly advanced by the synthesis of new monomers and polymers that extend the range of applicability of adhesive bonding. Thus, new materials should allow adhesives to be more flexible at cryogenic temperatures, more oxidation resistant at high temperatures, stronger at elevated temperatures, and more tolerant of an ill-prepared or low-surface-energy adherent. The engineering aspects of adhesive technology can be solidly advanced by including adhesive technology in university engineering courses and establishing an engineering database. In addition, an easy, nondestructive method of predicting the strength of a joint would be a major advance in the applicability of adhesives. Two drivers of advances in adhesive technology in the near future are economics and the environment. To be environmentally acceptable, new adhesive formulations should contain a minimum of solvent and in some applications should be biodegradable. To be economically attractive, adhesives should be easy to use and should provide a value-added feature to the customer that outweighs the disadvantages cited above.
Blends and Alloys
The time scale for introducing totally new polymers is increasing because the simplest monomers and the processes for converting them into polymers have already been identified and introduced into the marketplace. Furthermore, with increasing regulatory obstacles and the high cost of research, the economic stakes for introducing generically new polymers based on previously unknown chemistry and manufacturing processes have been raised considerably. Because this field was initially dominated by the ready opportunities for chemical innovation, serious development based on the more physical approach of alloying or
blending existing polymers did not begin until the late 1970s and 1980s. Now, the area of polymer blends is one of the routes to new materials that is most actively pursued by the polymer industry.
There are several driving forces for blending two or more existing polymers. Quite often, the goal is to achieve a material having a combination of the properties unique to each of the components, such as chemical resistance and toughness. Another issue is cost reduction; a high-performance material can be blended with a lower-cost polymer to expand market opportunities. A third driving force for blending polymers of different types is addition of elastomeric materials to rigid and brittle polymers for the purpose of toughening. Such blends were the first commercial example of polymer blend technology and, even today, probably account for the largest volume of manufacturing of multicomponent polymer systems. The main problem is that frequently when polymers are blended, many critical properties are severely depressed because of incompatibility. On the other hand, some blends yield more or less additive property responses, and others display certain levels of synergism. The problem is knowing how to predict in advance which will occur and how to remedy deficiencies.
From a fundamental point of view, one of the most interesting questions to ask about a blend of two polymers is whether they form a miscible mixture or solution. The thermodynamics of polymer blends is quite different from that of mixtures of low-molecular-weight materials, owing to their molecular size and the greater importance of compressibility effects. Because of these, miscibility of two polymers generally is driven by energetic rather than the usual entropy considerations that cause most low-molecular-weight materials to be soluble in one another. The simple theories predict that miscibility of blends is unlikely; however, recent research has shown that by carefully selecting or designing the component polymers there are many exceptions to this forecast. The phase diagram for polymer blends is often opposite of what is found for solutions of low-molecular-weight compounds. Polymers often phase separate on heating rather than on cooling as expected for compounds of low molecular weight. Theories to explain the behavior of miscible polymer blends have emerged, but theoretical guidance for predicting the responsible interactions is primitive. With the advent of modern computing power and software development, molecular mechanics calculations of this type are being attempted. Neutron scattering has provided considerable insight about the thermodynamic behavior of blends and the processes of phase separation.
One of the earliest blend products was a miscible mixture of poly(phenylene oxide) and polystyrene. The former is relatively expensive and rather difficult to process. The addition of polystyrene lowers the cost and makes processing easier. Numerous other commercial products are now based on miscible or partially miscible polymer pairs, including polycarbonate-polyester blends and high-performance ABS materials.
For mixtures that are not miscible, the most fundamental issues relate to
phase morphology and the nature of the interface between these phases. Frequently, the unfavorable polymer-polymer interactions that lead to immiscibility cause an unstable and uncontrolled morphology and a weak interface. These features translate into poor mechanical properties and low-value products, that is, incompatibility. When this is the case, strategies for achieving compatibility are sought, generally employing block or graft copolymers to be located at the interface, much like surfactants. These copolymers can be formed separately and added to the blend or formed in situ by reactive coupling at the interface during processing. The former route has, for example, made it possible to make blends of polyethylene and polystyrene useful for certain packaging applications by addition of block copolymers formed via anionic synthesis. However, viable synthetic routes to block copolymers needed for most commercially interesting combinations of polymer pairs are not available. For this reason, the route of reactive compatibilization is especially attractive and is receiving a great deal of attention for development of commercial products. It involves forming block or graft copolymers in situ during melt processing by reaction of functional groups. Extensive opportunities exist for developing schemes for compatibilization and for fundamental understanding of their mechanisms. A better understanding of polymer-polymer interactions and interfaces (e.g., interfacial tension, adhesion, and reactions at interfaces) is essential. Especially important is the development of experimental techniques and better theories for exploring the physics of block and graft copolymers at such interfaces. This knowledge must be integrated with a better understanding of the rheology and processing of multiphase polymeric materials so that the morphology and interfacial behavior of these materials can be controlled.
A wide variety of compatibilized polymer alloys have been commercialized, and the area is experiencing a high rate of growth. A product based on poly(phenylene oxide), a polyamide, and an elastomer has been introduced for use in forming injection-molded automobile fenders and is currently being placed on several models of U.S. and European-made automobiles. The polyamide confers toughness and chemical resistance, the poly(phenylene oxide) contributes resistance to the harsh thermal environment of automotive paint ovens, while the elastomer provides toughening. Another automotive application is the formation of plastic bumpers by injection molding of ternary blends of polycarbonate, poly(butylene terephthalate), and a core shell emulsion-made elastomeric impact modifier (Figure 3.5). In this blend, the polycarbonate brings toughness, which is augmented at low temperatures by the impact modifier, while the poly(butylene terephthalate) brings the needed chemical resistance to survive contact with gasoline, oils, and greases. In the first example, the poly(phenylene oxide) and polyamide are very incompatible, and reactive coupling of the phases is required for morphology control and for interfacial strengthening. In the second example, the polycarbonate and polyester apparently interact well enough that no compatibilizer is needed.

FIGURE 3.5 High-performance alloys are now used extensively in the demanding exterior body applications of automobiles. For example, front fenders are molded from a PPO-nylon alloy that can withstand paint oven temperatures of 400°F and above. They are chosen for their class-A surface, dimensional stability, impact strength, and corrosion and chemical resistance. The side claddings on these vehicles are molded of a resin that is a polyester-polycarbonate alloy, chosen for its cold temperature impact strength, chemical resistance, and quality surface. For the front and rear bumper fascias, a copolyester elastomer is used, characterized by its cold temperature ductility, class-A surface, chemical resistance, and on-line paintability at temperatures exceeding 280°F. More than 60 pounds of engineering thermoplastics can be found on many of the vehicles. SOURCE: Photograph courtesy of GE Plastics, Southfield, Michigan.
Toughening by the addition of rubber was first practiced for commodity polymers, such as polystyrene, poly(vinyl chloride), polypropylene, and poly(methyl methacrylate) (PMMA). Widely different processes and product designs were required to achieve optimal products. Now this approach is being applied to engineering thermoplastics and thermosets in order to move these materials into applications that require stringent mechanical performance under demanding conditions. This ensures an excellent growth opportunity for a variety of toughening agents. Elastomers with low glass transition temperatures are needed to impart toughness at low use temperatures, while thermal and oxidative
resistance are needed to survive the high temperatures required for processing these materials. In addition, these elastomers must be dispersed within the matrix to an appropriate morphology (or size scale) and adequately coupled to the matrix. These two issues are often interrelated and specific to the particular matrix material. Continued efforts will be required to produce a better understanding of the various toughening mechanisms that are applicable to engineering polymers.
Numerous opportunities exist to achieve better understanding that would shorten the time to develop new blends and alloys. There is an interesting parallel between this field and alloying in metallurgy, and the polymer community may be able to learn from the long experience of metallurgists. Both fields involve a broad spectrum of issues including synthesis, processing, physical structure, interfaces, fracture mechanics, and lifetime prediction. The United States is currently in a position of technical leadership; however, companies and universities around the world are also aggressively pursuing research and development in this field.
Structural Composites
Polymer composites can provide the greatest strength-to-weight and stiffness-to-weight ratios available in any material, even the lightest, strongest metals. Hence, high-performance and fuel-economy-driven applications are prime uses of such composites. One of the most important attributes is the opportunity to design various critical properties to suit the intended application. Indeed, performance may be controlled by altering the constituents, their geometries and arrangement, and the interfaces between them in the composite systems. This makes it possible to "create" materials tailored to applications, the single greatest advantage and future promise of these material systems. Structural composites are of interest in aerospace applications and in numerous industrial and consumer uses in which light weight, high strength, long fatigue life, and enhanced corrosion resistance are critical. Much needs to be done to advance processibility and durability, to provide a more comprehensive database, and to improve the economics of these systems. A wide range of future needs encompasses synthesis, characterization, processing, testing, and modeling of important polymer matrix composite systems.
In general, the future of polymer matrix composites is bright. The engineering community is now in the second generation of applications of composites, and primary structures are now being designed with these materials. There is a growing confidence in the reliability and durability of polymer composites and a growing realization that they hold the promise of economic as well as engineering gain. Commercial programs such as high-speed civil transport will not succeed without the use of polymer composites. Integrated synthesis, processing, characterization, and modeling will allow the use of molecular concepts for the
design of the material system and to estimate the effect of how the materials are put together on the performance, economy, and reliability of the resulting component. A more precise understanding of the manufacturing, processing, and component design steps will greatly accelerate the acceptance of these advanced materials. New horizons for properties and performance, for example, in smart and intelligent materials, actuators, sensors, high-temperature organic materials, and multicomponent hybrid systems, will involve the potential of introducing a new age of economic success and technical excellence. It has been estimated that finished-product businesses of greater than $5B annually already exist for the aggregate of polymers, reinforcements, prepregs, tooling machinery, and other ancillary products (McDermott, 1993).
Advanced polymer matrix composites have been used for more than 20 years, for example, on the B-1 bomber and for many top-of-the-line Navy and Air Force jet fighters. For military purposes, the high performance and stealthiness of composites have often outweighed issues of durability and even safety. Building lighter, more maneuverable tanks, trucks, and armored vehicles might be an area for future military growth. However, as the Pentagon's budget shrinks, efforts to transform these materials into civilian uses are under way (Pasztor, 1992). Problems include the need to identify significant nondefense companies that will use advanced composites. For nearly 30 years, it has been suggested that aircraft designers around the world would rapidly utilize these new materials. Unfortunately, those predictions have not been realized, and U.S. plants making polymer matrix composites are now operating at less than 50 percent of capacity. For a number of reasons, there is continued reticence to employ these advanced materials in many areas, particularly in commercial aviation. Costs, processibility, and durability appear to be the major issues. To this point, this area has been considered a technical success but not a financial success. Nevertheless, aircraft in various stages of development have composites as some fraction of their structural weight. For example, 15 percent of the Boeing 777, 6 percent of the MD-11 Trijet, and 15 percent of the MD-12 are estimated to be composites. European aviation firms have begun flight-testing an all-composite tail rotor for a helicopter, and Japanese efforts are under way to develop a military helicopter that has a very high composite content.
It has been predicted that in the future, fiber-reinforced composites (FRCs) will partially replace conventional materials in civil engineering applications. These could include buildings, bridges, sewage and water treatment facilities, marine structures, parking garages, and many other examples of infrastructure components. Composite materials are also expected to help replace conventional materials such as steel and concrete in many future projects. A volume of $3T for fiber-reinforced composites in the rehabilitation of the country's infrastructure has been estimated (Barbero and Gangarao, 1991). The polymer matrix resin composites discussed above have already made inroads in areas such as antenna coverage and water treatment plants. Less expensive fiber-reinforced
plastics (FRPs), such as unsaturated polyester styrene matrix systems reinforced with glass fibers, have become important automobile and bathroom construction materials. Sheet molding compounds, which are used extensively in automobiles and housing, are not considered by many structural engineers to be suitable for infrastructure replacement owing to their relatively low strength. Advanced polymer composites, on the other hand, which often consist of continuously reinforced fiber materials, have superior strength and stiffness.
Liquid Crystalline Polymers
The liquid crystalline nature of stiff polymer molecules in solution was predicted by Onsager in 1947, further refined by Flory in 1956, and experimentally verified through aramid investigations at the Du Pont Company in the 1960s. Flory suggested that as the molecular chain becomes more rodlike, a critical aspect ratio is reached, above which the molecules necessarily line up to pack efficiently in three dimensions. Liquid crystal polymer concepts have been extended to encompass a vast number of homopolymer and copolymer compositions that exhibit either lyotropic or thermotropic behavior. Industrially, most of the effort has been focused on the main-chain nematic polymers. These polymers combine inherently high thermal and mechanical properties with processing ease and versatility. Processing ease originates from the facile way that molecular rods can slide by one another, the very high mechanical properties come from the "extended chain" morphology present in the solid state, and the thermal stability derives from the highly aromatic chain chemistry. Inherent in this structure is a high level of structural, and hence property, anisotropy (for example, the axial modulus is 1 to 2 orders of magnitude higher than the transverse modulus). The direction of molecular chain orientation is coincident with the direction of covalent bonding in the chain; normal to the orientation direction the bonding is secondary (van der Waals, hydrogen bonding, and so on). Low orientation in these materials means global but not local randomness, and properties within "domains" are highly anisotropic.
A useful spin-off of the study of liquid crystal polymers was the recognition of the importance of mesophases in the development of structure in conventional polymers. Examples of this include the stiffening of polyimide backbones to reduce the expansion coefficient and improve processibility and the recognition of the importance of a pseudo-hexagonal (rotator, transient nematic) phase in the crystallization of oriented polymer melts. Increasing the end-to-end distance of conventional polymers through the application of either mechanical or electromagnetic fields can lead to the formation of structure equivalent to that achieved by the manipulation of molecularly stiff molecules.
Fibers from lyotropic para-aramid polymers (Figure 3.6) were initially commercialized by the Du Pont Company in 1970 under the Kevlar® trademark. The fibers are dry-jet wet spun from 100 percent sulfuric acid solution with sufficient

FIGURE 3.6 A lyotropic para-aramid liquid crystal polymer.
drawdown to orient the molecular chains, actually the liquid crystal domains, parallel to the fiber axis. An annealing step may be performed to improve structural perfection, resulting in an increase of fiber modulus. These fibers have very high modulus and tensile strengths as well as excellent thermal and environmental stability. Weaknesses include low compressive properties (endemic with all highly uniaxially oriented polymers) and a significant moisture regain. Worldwide fiber production capacity is about 70 million pounds (1991). Selling prices vary according to grade (i.e., modulus level) and market, ranging from as low as $8 per pound to over $50 per pound. Consumption worldwide in 1990 was about 50 million pounds, somewhat trailing capacity. Major markets include reinforcement for rubber and composites, protective apparel, ropes and cable, and asbestos replacement. The use of para-aramid fiber is projected to grow at greater than 10 percent per year worldwide over the next 5 years. The environmental issues involved in the handling and disposal of large quantities of sulfuric acid or other solvents may make thermotropic approaches more attractive in the future.
During the 1980s, thermotropic copolyesters were commercialized world-wide. More versatile than the lyotropic polymers, these nematic copolyesters (Figure 3.7) are amenable to uniaxial processing, such as fiber formation, and three-dimensional processing, such as injection molding, utilizing essentially conventional thermoplastic processing techniques. While fiber products exist, most of the commercial thermotropic copolyester is sold as glass-or mineral-filled molding resins, the majority into electrical and electronic markets. U.S. volume in 1991 was about 4.3 million pounds, at an average selling price of about $8 per pound. As in the case of the aramids, thermal and environmental stability is excellent. Advantages of these molding resins are the extremely low viscosity, allowing the filling of complex, thin-walled molds, excellent mold reproduction because of the low change in volume between liquid and solid, and fast cycle times. Weaknesses include property anisotropy and high cost.
The future growth of the main-chain nematogenic polymers will be dominated by two factors:
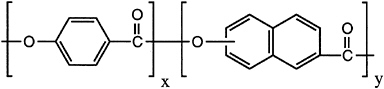
FIGURE 3.7 An aromatic polyester thermotropic liquid crystal polymer.
-
Polymer cost (ultimately dependent on monomer cost), and
-
Processing technology allowing cost-effective exploitation of properties, including orientation control in finished parts, as well as new forms (e.g., films, shaped extrudates, nonwovens, foams, and multilayers).
Two particularly intriguing properties of nematogenic polymers not yet important commercially are ductility under cryogenic conditions and very low permeabilities of small molecules through the solid-state structure (high barrier properties).
A potentially attractive route to both lower price and improved property control is the blending of liquid crystal polymers with conventional polymers. An extensive literature exists, and interesting concepts such as self-reinforcing composites and molecular composites have been developed to describe immiscible and miscible liquid crystal polymer-containing blends. Major problems encountered in this technology include:
-
Inherent immiscibility of mesogenic and conventional polymers, leading to large-scale phase separation;
-
Strong dependence of blend morphology (properties) on processing and polymer variables; and
-
Lack of adhesion between phases.
To date, commercial success for such blends has proved elusive. A related approach is the use of liquid crystal polymers in conventional composites, either as reinforcing fiber, matrix, or both. Penetration into conventional composite markets has been slow, the major problems being poor adhesion, poor compression (fibers), and the lack of design criteria for composite parts where both matrix and ply are anisotropic.
The potential of polymeric liquid crystals in device rather than structural applications has been recognized in both industry and academia, but no commercially viable products have yet emerged. The combination of inherent order, environmental stability, and ease of processing has led to interest in the use of polymeric liquid crystalline textures in applications as diverse as nonlinear optics, optical data storage, and "orienting carriers" for conducting polymers. With structural parameters of secondary importance, all textures are under active investigation. Both main-chain and side-chain approaches are of interest, the goal
being to tailor orientational and transitional states to the specific end use desired. Emerging problems include achieving sufficient density of active species to produce materials with competitive figures of merit (i.e., dipole concentration for nonlinear optical applications) and balancing mesogenicity effects with high and stable use temperatures. Clearly, the introduction of mesogenicity into polymers opens vast possibilities for molecular design, which may ultimately lead to the creation of materials with highly specific and unique property sets.
Films, Membranes, and Coatings
Polymers are used in many applications in which their main function is to regulate the migration of small molecules or ions from one region to another. Examples include containers whose walls must keep oxygen outside or carbon dioxide and water inside; coatings that protect substrates from water, oxygen, and salts; packaging films to protect foodstuffs from contamination, oxidation, or dehydration; so-called "smart packages," which allow vegetables to respire by balancing both oxygen and carbon dioxide transmission so that they remain fresh for long storage or shipping times; thin films for controlled delivery of drugs, fertilizers, herbicides, and so on; and ultrathin membranes for separation of fluid mixtures. These diverse functions can be achieved partly because the permeability to small molecules via a solution-diffusion mechanism can be varied over enormous ranges by manipulation of the molecular and physical structure of the polymer. The polymer that has the lowest known permeability to gases is bonedry poly(vinyl alcohol), while the recently discovered poly(trimethylsilyl propyne) is the most permeable polymer known to date. The span between these limits for oxygen gas is a factor of 1010. A variety of factors, including free volume, intermolecular forces, chain stiffness, and mobility, act together to cause this enormous range of transport behavior. Recent experimental work has provided a great deal of insight, while attempts to simulate the diffusional process using molecular mechanics are at a very primitive stage. There is clearly a need for guidance in molecular design of polymers for each of the types of applications described in more detail below. In addition, innovations in processing are needed.
Barrier Polymers
As shown earlier, packaging applications currently consume roughly one-third of the production of thermoplastic polymers for fabrication of a wide array of rigid and flexible package designs (see Figure 3.2). These packages must have a variety of attributes, but one of the most important is to keep contaminants, especially oxygen, out, while critical contents such as carbon dioxide, flavors, and moisture are kept inside. Metals and glass are usually almost perfect barriers, whereas polymers always have a finite permeability, which can limit
the shelf-life of the products they protect. In spite of this deficiency, the light weight, low cost, ease of fabrication, toughness, and clarity of polymers have driven producers to convert from metal and glass to polymeric packaging. Polymers often provide considerable savings in raw materials, fabrication, and transportation, as well as improved safety for the consumer relative to glass; however, these advantages must be weighed against complex life-cycle issues now being addressed. The following discussion illustrates the current state of this technology, its problems, and future opportunities.
There are certain polymer molecular structures that provide good barrier properties; however, these structural features seem invariably to lead to other problems. For example, the polar structures of poly(vinyl alcohol), polyacrylonitrile, and poly(vinylidene chloride) make these materials extremely good barriers to oxygen or carbon dioxide under certain conditions, but each material is very difficult to melt fabricate for the same reason. The good barrier properties stem from the strong interchain forces caused by polarity that make diffusional jumps of penetrant molecules very difficult. To overcome these same forces by heating, so that the polymer chains can move in relation to one another in a melt, requires temperatures that cause these reactive materials to degrade chemically by various mechanisms. Thus neither poly(vinyl alcohol) nor polyacrylonitrile can be melt processed in its pure form. Resorting to solvent processing of these materials or using them to make copolymers compromises their value. Poly(vinyl alcohol), by virtue of its hydrogen bonding capability, is very hygroscopic, to the point of being water soluble, and this property prevents its use as a barrier material in the pure form even if it could be melt processed. In general, polarity favors good oxygen barrier properties but leads to poor water barrier properties. This is true for aliphatic polyamides (nylon). On the other hand, very nonpolar materials, such as polyethylene and polypropylene, are excellent barriers to water but not oxygen. This property-processibility trade-off has led to an interest in composite structures. The ''composites" can be at the molecular level (copolymers), microlevel (blends), or macrolevel (multilayers).
The attractive barrier characteristics of poly(vinyl alcohol) have been captured via copolymers, and this achievement has led to some important commercial products using clever molecular engineering and processes that minimize its shortcomings of water uptake and lack of melt processibility. Copolymers containing units of ethylene and vinyl alcohol are made commercially by starting with ethylene and vinyl acetate copolymers and then hydrolyzing them. By critically balancing the structure of these materials, melt processible products that are relatively good barriers with reduced moisture sensitivity can be achieved. These copolymers are incorporated into multilayer structures by coextrusion processes. For example, blow-molded bottles with five to seven layers in the side wall are in commercial use for marketing very sensitive foodstuffs. Lightweight, squeezable, fracture-resistant bottles for ketchup are now on the market. Layers of ethylene/vinyl alcohol copolymer provide the oxygen barrier
to prevent spoilage of the sensitive tomato base, while layers of polypropylene provide the water barrier that prevents dehydration on storage. Interlayers are often needed to adhere the functional layers to one another when the two differ greatly in chemical structure. Sometimes a mixed layer is included to accommodate recycled material from the process. The barrier function can also be provided by metal foil or by coatings of other polymers or inorganic layers onto containers. Of course, composite structures are inherently more difficult to recycle. Layers based on halogen-based polymers generate acid gases upon incineration. Reconciling these issues will be a major preoccupation during the next decade.
One of the major developments over the past two decades has been the replacement of glass with plastics in bottles for soft drink merchandising. The driving forces for this conversion were issues of cost, weight, safety, and total energy considerations. The commercialization of this technology using poly(ethylene terephthalate), or PET, involved innovative developments in processing for increasing molecular weight (solid-state reaction) and for fabrication (injection-blow molding) to achieve a highly oriented and transparent bottle. The carbon dioxide permeability of PET provides just enough shelf-life for very successful marketing of large 2-liter products; however, smaller bottles, such as the half liter, with a higher surface-to-volume ratio, have a shorter shelf-life. PET is also easily recycled, and considerable progress is being made in this area. PET, however, has not been able to succeed so far in the beer packaging market, owing to marginal oxygen barrier characteristics among other issues. Polyesters with much better properties are known, such as poly(ethylene naphthalene-2, 6-dicarboxylate), but these have not yet become commercial because economical processes for raw material production have not been developed.
Current areas of focus include the development of packages that can be directly microwaved, such as packages for soups in single-serving sizes, and controlled atmosphere packaging, which is capable of keeping fruits and vegetables fresh for weeks. Successes in the latter area could revolutionize the agriculture and food industries of the world in terms of where produce is grown, how it is distributed, and who has access to it. There are some clear fundamental challenges for development of new barrier materials that are economical, melt processible, and environmentally friendly, but significantly better than current ones in terms of permeability to oxygen, water, and oil.
Membranes
Membrane-based processes that provide many useful functions for society, usually at lower cost, particularly in terms of energy, have achieved substantial commercial importance relatively recently. The majority of the membranes used are made from polymers. It has been estimated that the worldwide sales of membranes in 1990 was of the order of $2B (ancillary hardware associated with membrane systems raises this value severalfold) and that this industry is growing
at 12 to 15 percent per year (Strathman, 1991). The United States is clearly in the lead position, but Europe and Japan are gaining rapidly. There is interest in other materials, such as ceramics, but it is clear that polymers will dominate in most uses. For the most part, the major limitation of membrane technology is the performance of the membrane itself; hence, sustained growth demands new developments in membrane materials and membrane fabrication.
Membranes are used to produce potable water from the sea and brackish waters, to treat industrial effluents, to recover hydrogen from off-gases, to produce nitrogen and oxygen-enriched air from air, to upgrade fuel gases, and to purify molecular solutions in the chemical and pharmaceutical industries. They are the key elements in artificial kidneys and controlled drug delivery systems. Basically, membranes may function in one of two general ways, depending on the separation to be performed and the structure of the membrane.
Some membranes act as passive filters, albeit usually on a very small scale. These membranes have pores through which fluid flows, but the pores retain larger particles, colloids, or macromolecules (e.g., proteins). Depending on the scale of the pores and the solute or particles, the operations are subdivided into ultrafiltration, microfiltration, and macrofiltration. The material dictates the manner in which the membrane can be formed and especially the size and distribution of the pores. Porous polymer-based membranes are made by solution processes, mechanical stretching, extraction, or ion bombardment processes. The nature of the membrane material is a key factor in resistance to damage and fouling and in compatibility with the fluid phase (e.g., blood).
When the membrane is nonporous, the polymer is a more direct participant in the transport process. Permeation across the membrane involves dissolution of the penetrant into the polymer and then its diffusion to the other surface, that is, a solution-diffusion mechanism. The thermodynamic solubility and kinetic diffusion coefficients of penetrants in polymers depend critically on the molecular structures of the penetrant and the polymer and their interactions. This is the mechanism by which reverse osmosis, gas separation, and pervaporation membranes function.
In order to have usefully high rates of production in membrane processes, it is generally necessary to have membranes that are very thin and to have a very large membrane area packaged in small volumes. Ingenious approaches have been developed to achieve both. Membranes may be in the form of a flat sheet wrapped into a spiral for packaging into modules or in the form of very fine hollow fibers. In either case the membrane has a dense skin that is very thin (0.01 to 2 micrometers [µm]), which is the selective rate-controlling element that overlays a much thicker porous substructure to provide mechanical support or integrity at minimal permeation resistance. The skin and the substructure may be integral, made of the same material. The method to fabricate such asymmetric membranes was discovered in the 1960s and was first applied to make reverse osmosis membranes and later to make gas separation membranes. This
was the pivotal invention that has made most of modern membrane technology possible. A variety of composite membrane concepts were developed later that have the advantage that the skin and porous support are not integral and can, in fact, be made of different materials. This is especially useful when the active skin material is very expensive. Reverse osmosis and gas separation membranes of both types are in current use.
There is growth in almost all sectors of the membrane industry; however, the opportunities for future impact by new polymer technology appear somewhat uneven. For example, one of the major limitations to the use of ultrafiltration-type processes in the growing biotechnology arena is the tendency for surface fouling by protein and related macromolecules. The discovery of new membrane materials or surface treatments that solve this problem would be of major importance.
Intense polymer research related to reverse osmosis during the 1960s and 1970s led to commercial installation of desalinization plants around the world. Membranes in use are made of cellulose acetate and polyamides. Future demands for fresh water from the sea could stimulate renewed research interest in this area. Currently most of the efforts are devoted to developing reverse osmosis membranes and processes for removal of organic pollutants, rather than salt, from water.
Gas separation is clearly one of the most active and promising areas of membrane technology for polymer science and engineering (Figure 3.8). The first commercial membranes introduced in the late 1970s were hollow fibers formed from polysulfone by using a unique technology to remove minute surface defects. Since then, other polymers have been introduced in the United States, including cellulose acetate, polydimethylsiloxane (PDMS), ethyl cellulose, brominated polycarbonate, and polyimides. The first materials selected for this purpose were simply available commercial polymers that had adequate properties. New generations of materials especially tailored for gas separation are being sought to open new business opportunities. The key issues involve certain trade-offs. The polymer must be soluble enough to be fabricated into a membrane, but it needs resistance to chemicals that may be in the feed streams to be separated. The membrane should have a high intrinsic permeability to gases in order to achieve high productivity, but the permeation should be selective; that is, one gas, for example, O2, must permeate much faster than another, for example, N2. New polymers whose permeability and selectivity are higher than those of current membrane materials are being developed via synthesis of novel structures that prevent dense molecular packing, thus yielding high permeability, while restraining chain motions that decrease selectivity.
Pervaporation is a process in which a liquid is fed to a membrane process and a vapor is removed. The difference in composition between the two streams is governed by permeation kinetics rather than by vapor-liquid equilibrium as in simple evaporation. Thus, pervaporation is useful for breaking azeotropes and is
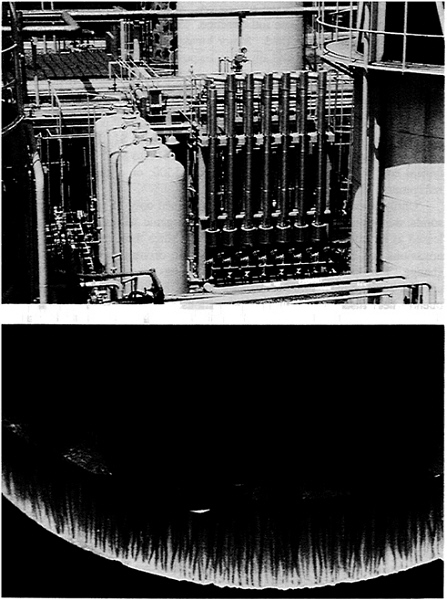
FIGURE 3.8 An industrial installation (top) of a membrane separator used in conjunction with a pressure-swing adsorption system to recover 90 percent of the hydrogen gas that normally would be lost in a waste stream. Also shown (bottom) is a cut-off section of a bundle of thousands of tiny hollow fibers made of polysulfone embedded in an epoxy tube sheet that fits into each tubular module shown. SOURCE: Photographs courtesy of Permea Inc., St. Louis.
generally used for alcohol-water separations, which become more important as biotechnology processes come into practice. Europe and Japan seems to be the leaders of research in this field. Major breakthroughs in membrane materials and fabrication are needed and appear to be possible.
Coatings
The U.S. coatings (paint) industry uses about 2.6 × 109 pounds of polymers per year and converts these to coatings having about $11B in sales. These coatings are applied onto goods valued at about $1T to $2T. For example, the typical cost of the paint on a house is about $500; on a car, $200; and on a refrigerator, $50.
Until the early 1970s most coatings contained only 15 to 30 percent paint solids, the remaining 70 to 85 percent being organic solvents, which were released as air pollutants when the films dried. Since then, reduction of solvent emissions has been the most important single driving force for technology change. Two kinds of 100 percent solids coatings are now being sold for specialized applications. Powder coatings are electrostatically applied and subsequently heat fused. Radiation-cured coatings are based on solventless liquid oligomers responding to ultraviolet or electronbeam cure. Solvent-borne high-solids coatings play an ever-increasing role. These are often based on oligomers containing hydroxyl groups as cross-linkable sites. Cross-linking is accomplished by formaldehyde-based methylolated or alkoxy-methylolated nitrogenous (amino) compounds. Acid-catalyzed heat cure causes the formation of multiple ether-based cross-links. Alternately, hydroxyl functional oligomers are cross-linked with isocyanates to form urethanes. Other common high-solids coatings have drying oil functionality and are cross-linked by air oxidation. Such high-solids coatings now contain about 20 to 50 percent volatile organic compounds (VOCs). The VOCs include solvents, by-products of the cross-linking reaction, and amines used to block catalysts. The role of aqueous coatings in reducing emissions of VOCs has increased greatly in the past few years. These coatings are based mostly on high-molecular-weight latex polymers and still contain some organic solvents to help film formation and wetting. Alternately, aqueous coatings are based on lower-molecular-weight hydrophilic polymers, which, unlike latexes, are synthesized not in water but in organic solvents and are subsequently dispersed into water. The ratio of VOCs to paint solids in aqueous coatings is now about 1:1 to 2:1. The environmental concerns leading to a reduction in VOCs were met by newly developed coatings based on new polymer chemistry that often provide better performance than the traditional coatings. In particular, resistance to weathering and to corrosive environments (including acid rain) was improved, not only for heat-cured but also for ambient-cured coatings. Lowering the cure temperature has allowed the application of
coatings to a variety of structural plastics, which are starting to replace metals in many products.
The structure-property relationships for the great variety of new polymers are poorly understood. In fact, the fast empirical development of the new coatings has outstripped our scientific understanding. A few examples illustrate this. The most sophisticated coating resin system cannot be described solely by the overall monomer composition. Latexes are now being synthesized so that the monomer composition varies stepwise or gradually from the center to the surface of the particles. For example, a high-Tg (glass transition temperature) core and a low-Tg skin in the latex particles provide relatively hard coatings (related to high Tg) with ease of film formation (related to low Tg). Because the latex particles are very small, typically 50 to 300 nanometers (nm), it is practical to blend into them thermodynamically incompatible polymers. Layering, as described above, is one example. Acrylic monomers can be polymerized into urethane latexes, leading to separation into microphases within the latex particles or to formation of interpenetrating polymer networks. Alternately, latexes of differing compositions are synthesized separately and then blended. Also, latexes can be blended with separately synthesized low-molecular-weight water-soluble polymers. A single coating can be based on several polymers varying greatly in composition: polar and nonpolar vinyl polymers, urethanes, epoxies, polyesters, and alkyds. This way, the rheology, wetting properties, reactivity, and chemical resistance of the coatings can be optimized beyond what can be obtained by single polymer systems.
Apart from reduction of VOCs, another driving force for change is elimination of toxic ingredients from coatings. Some of the several sources of toxicity are cross-linkers for hydroxy-functional polymers: isocyanates or formaldehyde in amino cross-linkers. New cross-linking mechanisms are being sought. Promising early results involve reactions of carboxyl groups with epoxies or with nitrogenous heterocycles. The Michael reaction of acrylic double bonds with amines or activated methylene groups looks promising. Ketones or α,β-diketones are reacted with amines or carbohydrazides. Silane-functional polymers cross-link when exposed to atmospheric moisture. Some of these new systems provide chemically resistant cross-links even at ambient temperature, a great advantage for applications where high-temperature cure is not possible, such as car refinishes, aviation, implements, plastics, wood furniture, and architecture. New, very inert cross-linked resins in the coatings often provide good corrosion protection, allowing the use of nontoxic chrome-free corrosion inhibitors. Also, some new polymers provide improved interaction with difficult-to-disperse pigments so that toxic lead-or chrome-based pigments can be conveniently replaced by all-organic pigments. The greater variety of resins now available for coatings allows the elimination of toxic aromatic and ethylene-oxide-based solvents from high-solids or aqueous coatings.
Because the United States began to impose legislation to control the environment
earlier than other countries, the U.S. coating industry gained early international technical leadership in nonpolluting coatings. These new technologies are now internationally available. However, the potential for further reducing VOCs and toxic ingredients and for further improving coating performance is huge.
For further progress, better understanding of solid-and liquid-state rheology and thermodynamics of complex resin systems is required. New chemical approaches for precision synthesis and for cross-linking of polymers and oligomers are also needed. Aqueous polymer systems are particularly fertile ground for new research. The preparation of complex acrylic latexes, urethane latexes, epoxy dispersions, solubilized polyesters, alkyds, and vinyl resins is still an empirical art.
Inorganic Polymers
The materials described above are made up primarily of polymer molecules based on covalently bonded chains in which carbon is the principal element. Polyethylene, polystyrene, poly(methyl methacrylate), and poly(vinyl chloride) are all based on carbon chains with differing side groups. Nitrogen and oxygen can also be incorporated into polymer chains, as in polyamides (nylons) and polyesters, but carbon atoms separate the hetero-atoms. Considering the importance and variety of organic polymers, natural and synthetic, it is remarkable that the chemistry of carbon is so unique and so dominant.
Other polymers exist in which carbon is less dominant. Polypeptides and proteins have one nitrogen for every two carbons, and the great variety is derived entirely from differences in groups attached to the alpha carbon atom. All of these materials are, however, entirely "organic" in character. Polyoxymethylene is a polymer with alternating oxygen and carbon along the main chain. It is a partially crystalline molding compound. Poly(ethylene oxide), with two carbons for each oxygen in the chain, has been of particular interest because of its water solubility.
Chains containing no carbon (C) exist, and virtually limitless compositional and structural diversity is accessible through utilization of more of the periodic table. Silicon, which is in the same group as carbon in the periodic table, is one such example. The vignette "Silicones" explores the properties and uses. At this time, however, the polysiloxane family is by far the most important. These materials have been available for several decades in the form of liquids, gels, greases, and elastomers that exhibit good stability and properties. They are the most thoroughly studied and highly commercialized class of inorganic polymers. Although the chain is entirely inorganic—with alternating single silicon (Si) and oxygen (O) atoms—organic side groups (usually methyl or phenyl) are attached to the silicon atoms. Many applications of polysiloxanes derive from the extraordinary flexibility of the siloxane backbone. The Si–O bond is significantly
SILICONES Beginning in 1942, B-17 bombers were flown from factories in the United States to airbases in Great Britain. But before the Flying Fortresses could cross the Atlantic—much less raid German factories—a critical problem had to be solved. The thin air at cruising altitudes can be ionized by the high-voltage electricity of an aircraft ignition system. The effect is not large in the relatively dry air over land, but the water vapor in damp oceanic air is highly susceptible. The moist air would work its way into tiny pores in the rubber wires insulating the ignition system. Soon the pilot would notice a blue glow around the leading edge of an engine nacelle—a corona of electricity arcing from spark plug to cylinder, shorting out the plug. The engine would start misfiring, eventually dying altogether as more pistons quit. If more than one engine went south, the crew might have to ditch, and the "Fort" would be lost at sea. A silicone polymer developed for waterproofing electrical equipment aboard submarines proved to be the answer. Applied liberally to the spark plug wires and boots, the silicone grease kept the wires dry and the bombers airborne—it was, in fact applied to all U.S. bombers throughout the war. Silicones are polymers whose backbones are long, flexible chains of alternating silicon and oxygen atoms. Dangling from the backbone like charms from a bracelet are side chains, usually small, carbon-based units, and the choice of these side chains gives silicones a remarkable range of properties. The water-repellent grease has oily, nonpolar side chains such as methyl groups. The nonpolar side chains and the polar water molecules do not mix, repelling the water from the silicone. Another application of silicones depends on a careful balance between polar and nonpolar side chains. Small amounts of silicone foaming agents control the bubble size in polyurethane foams. A high proportion of polar side chains makes the foam foamier. The bubbles become bigger, forming open pores and producing the soft foams found in car seats and furniture cushions. Reduce the number of polar side chains, and the bubbles remain small. These tiny bubbles do not open up to form pores, and the foam is a much stiffer solid used for insulation. Silicones have other, seemingly contradictory properties. A silicone resin coating the bread pans in a bakery keeps fresh-baked bread from sticking in the pan, and a liquid silicone polymer on the molds in tire factories does the same thing for newly made tires. But adding a "tackifying resin" makes the silicone sticky and produces the drug-permeable contact adhesive used on those skin patches containing nicotine (for smokers who are trying to quit) or scopolamine (for seasickness sufferers who are trying not to lose it). Silicon and oxygen are the two most abundant elements on Earth, and they combine naturally to form silicates, including glass and such minerals as quartz and granite. These two elements were first combined synthetically—as silicones—in the United States in the 1930s. They were originally expensive and unhandy to make, but the discovery of a cheaper, easier method of producing them, coinciding with the interest in their novel properties sparked by World War II, started an avalanche of research into new uses for these versatile polymers that continues unabated today. |
longer than the C–C bond, the oxygen atoms are unencumbered by side groups, and the Si–O–Si bond angle of 143 degrees is much more open than the usual tetrahedral angle of 110 degrees. These combined structural features increase the dynamic and equilibrium flexibility of the chain, causing, for example, the glass transition temperature of polydimethylsiloxane to be -125°C. Important applications include high-performance elastomers, membranes, electrical insulators, water-repellent sealants, adhesives, protective coatings, and hydraulic, heat-transfer, and dielectric fluids. The polysiloxanes also exhibit high oxygen permeability and good chemical inertness, which lead to a number of medical applications, such as soft contact lenses, artificial skin, drug delivery systems, and various prostheses.
Another family of inorganic polymers—the polyphosphazenes—is based on a chain of alternating phosphorus (P) and nitrogen (N) atoms. Over 300 different polymers have been synthesized, mainly by variation of the pendant groups. The pendant groups may be organic, inorganic, or organometallic ligands. The nature of the pendant groups affects the skeletal flexibility, solubility, refractive index, chemical stability, hydrophobicity, electronic conductivity, nonlinear optical activity, and biological behavior. Thus by choice of the appropriate side group, polyphosphazenes can be tailored for a variety of applications. These materials are prepared by methods that give little control over stereoregularity and, hence, mixed-substituent polyphosphazenes are amorphous. Glass transition temperatures as low as -100°C have been achieved, and elastomeric performance over a wide temperature range is characteristic of this family. Fluoroalkoxy substituents yield hydrocarbon-resistant materials that could be useful as fuel lines, o-rings, and gaskets in demanding environments. Ether side groups coordinate lithiumions, which leads to possible applications as polymeric electrolytes for high-technology batteries. The ease of side group substitution has also led to new applications in biomedical materials. Hydrophobic polymers such as poly[bis(trifluoroethoxy) phosphazene] minimize the "foreign body" interactions that normally occur when nonliving materials are implanted in contact with living tissues, such as blood. Hydrophobic polyphosphazenes are therefore good candidates for use in cardiovascular replacements or as coatings for pacemakers and other implantable devices. Hydrophilic or mixtures of hydrophilic and hydrophobic groups can be substituted to produce hydrophilic or amphiphilic polymers deliberately designed to stimulate tissue adhesion or infiltration or to generate a biochemical response. Unfortunately, while polyphosphazenes are an interesting class of materials that have physical and chemical characteristics that suggest many applications, they are costly to produce, and commercial success has consequently been modest.
Polysilanes (also called polysilylenes) have been the subject of research interest within the last decade. These all-silicon chains, with alkyl or phenyl side groups, are analogous to vinyl polymers, but they are made from silyldichlorides rather than from the analogue of ethylene. Linear and cross-linked
structures can be made, leading to materials that are glassy, elastomeric, or partially crystalline. The chains of silicon atoms are flexible. Applications that have been proposed for polysilanes include ceramic precursors (for silicon carbide), photoresists, photoinitiators, and nonlinear optical materials. The use of these inorganic polymers as ceramic precursors is important because the precursor can be spun into a fiber that yields fibrous ceramics following processing. In fact, polysilanes are the basis of a process commercialized by Nippon Carbon Company to produce continuous NICALON® silicon carbide fibers for use in such applications as fiber-reinforced ceramic composites. Poly(dimethyl silane) or the cyclic oligomer are heated to 450°C to produce a polycarbosilane that can be spun into fibers. The fibers are heated first in air to create a silica skin that prevents melting of the fiber during subsequent pyrolysis in N2 to produce silicon carbide (SiC).
The polyphosphates are inorganic polymers of interest in their own right, but their most important role is that they serve as part of the repeat unit in polynucleotides. The phosphate sequence is, therefore, being extensively studied with regard to biopolymer functions, for example in replication. Polysilazanes, with alternating silicon and nitrogen in the main chain, have also been prepared. Although these materials have not been as extensively studied as the polysilanes, it has been demonstrated that they can be used as precursors for silicon nitride ceramics.
Hybrid systems that combine organic ligands, polymers, or networks with inorganic polymers or networks, often referred to as CERAMERS or ORMOCERS (organically modified ceramics), represent some of the most structurally diverse and compositionally variable polymeric materials. The organic substituents can be designed as pendant groups that modify the inorganic polymer or network as in poly(organo siloxanes), as oligomers or polymers covalently bonded to the inorganic network, as interpenetrating networks, or as combinations of two or more of these functions. Hybrid systems are most often synthesized by low-temperature sol-gel hydrolysis using combinations of metal alkoxides and alkyl substituted and organofunctional alkoxysilanes: M(OR)n + R2Si(OR)2 + YR'Si(OR)3, where R is an alkyl group, R' is an alkylene, and Y is an organofunctional group such as vinyl, epoxy, or methacrylate.
Hybrid systems can potentially combine the advantageous properties of both organic and inorganic polymers. For example, epoxy-derived titanosiloxane polymers can be deposited at room temperature and cured at 90°C to produce optical quality, abrasion-resistant thin films as protective coatings on eyeglass lenses (and other optical elements) constructed of plastics such as poly(methyl methacrylate). The organic network provides resiliency and toughness, while the inorganic network provides hardness. The incorporation of elastomers such as polydimethylsiloxane and poly(tetraethylene oxide) into inorganic networks imparts flexibility to otherwise brittle materials, allowing large complex shapes to be processed rapidly without cracking. Independent free-radical and hydrolysis
condensation reactions have been used to design hybrid gels that do not shrink upon drying.
The isolation of organic dye molecules, liquid crystals, or biologically active species in inorganic or hybrid matrices has led to a vast array of composite optical materials currently being developed as lasers, sensors, displays, photo-chromic switches, and nonlinear optical devices. These materials are superior to organic matrix composites because the inorganic matrix (normally silica) exhibits greater transmittance and is less susceptible to photodegradation. Organic molecules embedded in inorganic matrices can also serve as templates for the creation of porosity. Removal of the templates by thermolysis, photolysis, or hydrolysis creates pores with well-defined sizes and shapes. Inorganic materials with tailored porosities are currently of interest for membranes, sensors, catalysts, and chromatography.
Inorganic, organometallic, and hybrid polymers and networks represent a potentially huge class of materials with virtually unlimited synthesis and processing challenges. It is envisioned that future research will continue to explore the periodic table in search of new combinations of materials, new molecular structures, and improved properties. Hybrid systems appear especially rich for research in the area of multifunctional materials, that is, smart materials that perform several optical, chemical, electronic, or physical functions simultaneously. The development of hybrid materials that exhibit some of the extraordinary strengths and fracture toughness of natural materials such as shell and bone is also anticipated. The remarkable versatility of polyphosphazenes and polysiloxanes will continue to be exploited for biomedical applications such as drug delivery and organ and soft tissue replacement as well as advanced elastomers, coatings, and membranes.
The future of preceramic polymers and sol-gel systems appears bright. A major challenge is to develop synthetic routes to pure, stoichiometric nonoxide ceramics, especially SiC, that exhibit spinnability and high ceramic yields. New synthetic routes such as ''molecular building block" approaches to multicomponent ceramics will be explored to prepare superconductor, ferroelectric, nonlinear optical, and ionic conducting phases, primarily in thin film form. The use of sol-gel processing to prepare "tailored" porous materials for applications in sensors, membranes, catalysts, adsorbents, and chromatography is an especially attractive area of research and development.
POLYMER PROCESSING
The growth of polymers both in volume and in number of uses, as described above, is in part related to their ease in processing. Contrary to popular perception, plastics are often more expensive than steel—that is, on a per-pound basis—but they are also much lighter than steel, glass, or aluminum. The great advantage to polymers lies in the many ways that they can be processed for
functional uses such as coatings for surface protection, films for a wide variety of uses, fibers for fabrics and carpeting, and an enormous variety of molded shapes.
Melt Processing
Melt processing is the most widely used and generally the preferred processing method. It is used for polymers that become liquid at elevated temperatures so that they can be extruded into fibers, films, tubes, or other linear shapes or molded into parts of complex shape. Such processes involve much more than simply changing the physical shape of the polymer; they also influence phase morphology, molecular conformations, and so on and ultimately have an important role in the performance of the product.
Molding
A mold is a hollow form that imparts to the material its final shape in the finished article. The term "molding" is employed for processes involving thermosets and thermoplastics and includes injection, transfer, compression, and blow molding. The injection molding process is the most common method of making plastic parts. In that process, thermoplastic pellets are melted and pumped toward a melt reservoir by a rotating screw. When enough molten plastic has accumulated, the screw plunges forward to push the melt into a steel mold. The plastic solidifies on cooling, and the mold is opened for removal of the part. Injection molding cycle times vary from a few seconds to minutes, depending on the plastic and the part size. Molding machines have become very sophisticated, and they are capable of turning out large numbers of molded articles with little or no operator attention. The heated plastic conforms intimately to the polished mold surface, which may be of complex shape, and the part produced usually requires little or no further machining or polishing. The mold and the machine that delivers plastic to the mold can be quite costly; therefore, the technology is suited only to parts needed in large numbers. Even so, injection molding is a process capable of exceptionally low cost in comparison with production processes for metal or ceramic parts.
Some of the current challenges in polymer processing include developing new materials, achieving greater precision, pursuing process modeling and development, and recycling. Some examples of new materials are special blends of existing polymers, polymer composites with fiber reinforcement, and liquid crystalline polymers. Some of these new materials are expensive and may be difficult to form into desired shapes; however, they are of value to the defense and aerospace industries in applications in which weight and performance are more important than cost and processibility. In contrast, automotive and appliance industries use materials that are less expensive, readily molded, and dimensionally
accurate. Yet there are limitations to what can be done, even with common materials. Molten plastics are viscous, and making thin parts may require high pressures. Further, the plastic shrinks as it cools, and this tendency must be compensated for by using oversized mold cavities. Molds are expensive, from several thousands to millions of dollars each.
Plastic materials are rheologically complex, and as a result many factors can affect the properties and dimensional accuracy of parts made from them. There are variations in operation of the molding machine, small temperature fluctuations, and differences in molecular orientation caused by flow into the mold. However, injection molding has been brought to levels that allow tolerances on small parts in the micrometer range. Among the high-performance plastics that have been introduced to meet the demands of the high-precision market are the thermotropic liquid crystalline polymers and low-viscosity versions of high-temperature materials such as polyetherimides and polyaryl sulfones.
Advances in processing are occurring at a rapid rate as on-line sensing, computing, and process feedback allow control and optimization of the molding process that were undreamed of only a few years ago. Parameters of importance include injection speed, peak pressure, hold pressure, and mold temperature, along with less obvious factors such as "cushion length" and position-or pressure-dependent cutoff. As the processing industry learns to take advantage of the capabilities of the new machines and materials, precision injection molding can be expected to make further inroads into the domain of machined metal parts.
Process dynamics and the properties of the finished article are critically dependent on the conditions of flow and solidification, down to the molecular level. As the mold is filling, the molten polymer solidifies first along the walls. The material that is farthest from the wall flows more rapidly, leading to a shearing and molecular elongation in the wall area. After the flow front has passed and the mold is full, the central regions solidify under conditions in which shear elongation is not a major factor. This solidification process leads to a morphology characterized by a skin of highly oriented polymer around a core of less oriented material. The two layers are mechanically and optically distinct. Control of these components through polymer composition and processing technology is a central issue in the production of precision, high-performance parts. Skin effects are most obvious in parts made from polymers that crystallize. Amorphous polymers are much less influenced. These morphological factors have important consequences for the failure mode and fracture mechanics of the finished part.
Additional processing techniques, such as gas-assisted molding and injection-compression molding, are gaining industrial acceptance. Materials suppliers are developing new plastics with enhanced flow characteristics and better
physical properties. Compact disks can be made because of high flow grades of polycarbonate and the injection-compression molding process.
The importance of injection molding, and of precision injection molding in particular, can hardly be overstated. The economies that can be realized in the production of mechanically complex parts can contribute to the feasibility of large-scale manufactured products. For example, communications systems of the future, such as fiber optics to the home providing broad-band information, depend on many manufactured details that must be reduced in cost if the concepts are to succeed. Polymer-based solutions are essential to the realization of the promise of progress in diverse areas.
Extensive progress has also been made recently in blow molding, especially to form bottles for various packaging applications. Special grades of polymers with uniquely tailored rheological properties, via broad molecular weight distribution and chain branching, have been developed for this market. Stretch blow molding processes allow control of the development of chain orientation and crystalline structure for materials such as poly(ethylene terephthalate) to gain better barrier properties.
Extrusion
Melt extrusion processes are usually the most convenient, economical, and environmentally favorable for film and sheet manufacturing. Screw extruders, in which a rotating screw transports material through a heated barrel and a shape-forming die, are the heart of such processes. Extruder screws, which can be very sophisticated, are designed with the help of extensive computer-assisted modeling. Frequently, mixing, compounding, and devolatilization are also involved to process formulations that include special additives, such as antioxidants, plasticizers, flame retardants, lubricants, pigments, fillers, and other polymers. Films are formed through film blowing of thin-walled tubes or drawing and tentering of cast films. Optimization of the process requires fundamental understanding of material properties and processing characteristics.
The properties of the fabricated product are strongly dictated by the details of the fabrication process. Influential variables include uniaxial or biaxial orientation, degree of crystallinity, morphology of amorphous and/or crystalline regions, internal stress, and dimensional control. The complete system—from material design, synthesis, and formulation to product design and processing—must be addressed to achieve optimal material selection for a specific application. All elements of the system are important. Materials scientists and engineers, who understand structure-property relationships and can manipulate molecular design, work closely with process engineers and product designers in a systems approach to meet the growing demand for extrudable polymers that have specific characteristics.
Solid-State Forming
Solid-state forming is one of the newest polymer processing methods and is especially useful for increasing strength and modulus of polymeric materials. The latter involves achieving morphologies with well-aligned, extended, and closely packed chains, which can be done by synthesizing rigid rodlike polymers containing parasubstituted aromatic structures in the chain backbone or by processing conventional flexible chain polymers in ways that lead to similar results.
In this processing approach, a highly oriented and extended chain conformation with substantially increased tensile modulus may be achieved by solid-state deformation of thermoplastics using the extrusion or alternate drawing techniques, such as extrusion of supercooled melts, and by drawing from gel or dilute flowing solutions. High-density polyethylene (HDPE) has been widely studied, in order to understand the morphological transformations and because it can now be drawn into one of the highest specific tensile moduli and strengths in both fiber and film form.
Solution Processing
Not all polymers can be fabricated by the convenient and economical melt processing techniques; nor is this desirable in some instances. Certain polymers have such strong interchain bonding that they do not melt or flow when heated until they reach temperatures at which chemical degradation occurs. These intractable, nonmoldable polymers are often fabricated, typically into fibers or films, by solution methods. Some well-known examples include certain cellulosics and polyacrylonitrile materials, plus specialty polymers for high-performance fibers like Nomex®, Kevlar®, and polybenzimidazoles (see the vignette "Polymers Stronger Than Steel"). Often such materials are soluble only in aggressive solvents such as sulfuric acid. In the usual case, the polymer is dissolved to relatively high concentrations, and these solutions are extruded into the form of fibers or film, or solvent cast into films. The polymer is solidified by removal of the solvent by evaporation through optimized drying regimes (dry spinning) or by coagulation and extraction with a nonsolvent (wet spinning). Cellulose is not generally soluble without chemical modification, and so rayon fiber manufacture involves a series of reactions, first to make the polymer soluble and then to harden it by regeneration of the original cellulosic structure. These types of solution processes are less well understood than melt processing operations, owing to their added complexity and the relatively little attention paid to this area because of the small quantity of products produced in this way. Because of the necessary solvent recovery steps, these processes tend to be expensive. The products produced by them often have complex morphological structures associated with the manner in which the solvent is removed that can leave residual porosity or other structural features not typical of melt-fabricated materials. In
POLYMERS STRONGER THAN STEEL One often thinks of polymers as soft plastic. However, when certain polymers are spun into fibers, the resulting materials are truly amazing. All polymers are long, chain-like molecules made up of many smaller molecules linked together. In flexible fibers, such as polyester, the chains are partially relaxed; that is, as we follow a polymer chain along a fiber, it passes alternately through regions that are well ordered and rigid and regions that are disordered and softer. However, the chains in these new rigid polymers are fully extended and lie parallel to each other, like a fistful of uncooked spaghetti. These fibers are unexpectedly strong for their weight. One such fiber, poly(paraphenylene terephthalamide), was found to have a tensile strength higher than that of a steel fiber of the same dimensions, yet it weighs one-fifth as much. The commercial development of this product was a long and very expensive process. More than 12 capital-intensive steps are required to convert the basic aromatic feedstocks into a strong polymer. The key to realizing the outstanding inherent strength of these materials is the process by which the polymer is spun into fibers. The first attempts using traditional techniques involving injecting a stream of the polymer directly into a cooling bath did not result in an unusual material. However, it was found that raising the spinner head above the cooling water bath that the still-molten spun fiber falls into imparted unprecedented strength to the fiber. As the molten fiber falls toward the cooling bath, the polymer molecules become stretched and aligned. The oriented polymers can then form hydrogen bonds between molecules in adjoining chains, further strengthening the fiber. Because the molecules are fully aligned along their axis, forces on the fiber are absorbed by strong chemical bonds, not by the weak, loosely intertwined chains in the flexible polymers. One of the aramid polymers, Kevlar®, has saved the lives of many soldiers and police officers. It has such high impact resistance that an aramid vest thin enough to be worn comfortably under the shirt will stop a bullet as effectively as steel plate. The helmets worn during Operation Desert Storm were also made of this remarkable material. This strong, lightweight material is now taking the place of steel belts in radial tires and is being exploited in a myriad of other applications that require a high strength-to-weight ratio or resistance to corrosive environments, such as the cables that anchor oil-drilling platforms at sea. The molecular structure of a polymer is important, but, as shown above, the orientation of polymer molecules in relation to each other also plays a major role in determining the properties of the final product. The molecules' orientation can be greatly affected by the chemical engineers' choice of the way(s) in which the material is processed, allowing many more ways to customize polymers for their applications than are possible through chemistry alone. |
addition, the potential solvent emissions and exposure of workers raise numerous environmental and health concerns. Nevertheless, such fabrication methods are the only option available for certain materials of critical importance. Interest in polymers with very high heat resistance and other special attributes is likely to increase the need for solution processing technology, because by the nature of their properties such materials cannot be melt fabricated.
In other cases, solution processing is required because of the nature of the product being fabricated rather than the nature of the polymer. Application of certain types of paint or coatings is most conveniently done by making the polymer fluid by dissolving it in a solvent and hardening by solvent removal. Melt fabrication cannot be used to economically perform certain operations like tablet coating (for controlled delivery) in the pharmaceutical industry. Formation of polymer membranes with complex structures consisting of extremely thin skins overlaying a porous support layer generally involves a variety of solution-processing protocols that can hardly be accomplished in any other way. Prepregs, used to make continuous-fiber-reinforced composites, often involve a solution processing step. "Molecular composites" based on rigid rod polymers dispersed in a matrix of a random coil chain polymer have attracted enthusiasm recently, and most techniques for forming them will invariably involve solution technology.
The state of scientific knowledge about the thermodynamics, rheology, diffusion, and morphology development in multicomponent polymer-solvent systems will need to be advanced in order to place these types of processes on a solid technical footing. For example, recent attempts to mathematically model the formation of asymmetric membranes have failed to give some of the insight critically needed simply because the fundamental elements of such models are not currently well-enough understood.
Environmental and health concerns associated with solution processing must be dealt with at several levels. There are opportunities for innovation in both the processes and the materials used. One avenue of interest is systems that use more benign solvents. Ongoing work in the area of supercritical fluid technology appears promising for some applications.
Dispersion Processing
Dispersion processing—the generation of particulate forms of polymers and their conversion into products—is a key technique that is likely to grow in importance, driven by both environmental and materials considerations. The polymer may be in the form of a dry powder, an aqueous dispersion (e.g., a latex), or a nonaqueous dispersion (NAD). A number of examples illustrate the current state of this technology and future directions.
Polytetrafluoroethylene, or Teflon®, is a powder after the polymerization process, and its melting point is so high that melt fabrication is usually not practical. However, by combinations of heat and pressure it is possible to fabricate solid forms in much the same way as used in powder metallurgy. Powder coatings are applied to various substrates by a number of techniques, including fluidized beds and flame heating. Powdered liquid crystalline polymers can be "extruded" below their melting points in much the same way as metal powders
are formed. Technology is being developed to convert polymer powders into three-dimensional prototype parts by using a computer-driven laser-sintering technique. There is much to be learned about the physics of sintering polymer powders.
Emulsion polymerization is widely practiced because it is a convenient process for producing high-molecular-weight polymers using free radical mechanisms at high rates while controlling the exothermic nature of the reaction. In some cases, the resulting aqueous dispersion of the very fine polymer particles (called a latex) can be used directly for convenient fabrication of products. For example, the ubiquitous rubber gloves employed nowadays to protect against the spread of AIDS are made by dipping forms into a rubber latex followed by vulcanization. The popular water-based latex paints are made by emulsion polymerization. The paint film is formed by the evaporation of water, as opposed to organic solvents for oil-based paints. Painters also like cleaning up using water rather than paint thinner or solvents. The tiny emulsion polymer particles fuse into a continuous film driven by surface tension forces as the water evaporates. The rheological characteristics of the polymer must be designed such that fusion can occur without macroscopic flow of the coating. Environmental considerations strongly favor formation of coatings using latexes rather than solutions that "cure" by evaporation of organic solvents.
Nonaqueous dispersion technology has emerged as a means of applying high-performance coatings (e.g., automotive paints) while minimizing solvent emissions. These are sophisticated materials in which fine polymer particles are formed by dispersion polymerization in a nonaqueous environment in which dispersant polymer chains prevent coalescence of the particles through steric stabilization. By this route, high-solids fluids of acceptable viscosity can be rapidly applied to metal substrates to form high-performance coatings with reduced emission of organic solvents.
Inverse emulsion homo-or co-polymerization of water-soluble monomers such as acrylamide or acrylamide with co-monomers, such as cationic quaternary acrylates, represents a new commercially practiced method for the generation of materials that are suitable as flocculants. The materials as generated are extremely effective for purifying water, even at a level of 500 parts per million. When activated in water via modification of the surfactant or stabilizer, they can induce large flocs to be formed, which can be centrifuged from waste systems like sewage. The resulting flocs can be certrifuged, dried, and used as fertilizers. The resulting water is, of course, in a much purer state than before this treatment. Such operations are already in wide commercial use in many of the large cities of Europe and North America and are an example of how polymers can help clean the environment. Producing such polymers is already a multimillion dollar business.
Process Models
Extensive efforts have been devoted to the development of mathematical modeling or simulation of polymer processes to develop sophisticated manufacturing methods; however, much remains to be done. Most of these efforts have been applied to polymerization processes or to fabrication processes. The most essential ingredient of the former has been kinetic modeling of the reactions involved, while rheological behavior of the fluid polymer is usually the center-piece of fabrication models. Each type may be coupled with mass and energy balances, along with accounting for heat, mass, and momentum transfer in varying levels of detail. Major issues in all cases are the level of sophistication of the models used and the accuracy of the input data. In the past, the sophistication or level of detail was limited by the computational power that could be brought to bear on the problem; however, this no longer seems to be the limitation. Future work in this area is expected to be extensive and more limited by the nature of the physical models available as the trend moves from simple process questions that can be answered by simple phenomenological models to complex product questions that require more detailed molecular models.
The most common driving force for developing process models is to aid in design. This function is often referred to as computer-aided design (CAD). For example, at the most elementary level a model of a polymerization process must be able to size the reactor and ancillary hardware and, in fact, to aid in making choices about the type of reactor system that would be most advantageous from a process point of view. Once a process configuration has been selected, computer-based models are generally able to predict temperature, pressure, and conversion profiles in time or space. More sophisticated versions are able to predict certain product qualities, such as molecular weight averages, copolymer composition, and branching frequencies. Models of this kind are valuable for modern computer-based process control schemes. They can also be of great value for evaluation of process safety issues. The future lies in going several steps beyond this to predict intrinsic polymer properties such as processing and end-use performance behavior. Such computer-based methods can be of value in the formulation, at least as a first estimate, and setting of process conditions to produce new grades of existing products.
Extensive efforts have been devoted to modeling fabrication operations such as extrusion, mixing, and molding operations. The sophistication employed depends on the objective to be achieved. Again, the most common motivation for model development is to aid design. For example, because molds are expensive to build, it is valuable to have models that are able to calculate whether a particular design will function as intended (e.g., fill up in the available time) for possible operating variables.
Most companies currently use externally or internally available software, combined with phenomenological rheological and heat transfer characterization
of the polymer, to assess a proposed design before cutting metal. In spite of their computational complexity, such models answer only fairly simple process questions. Molecular-based models of flow behavior are emerging, which if properly combined with a process model are likely to be able to answer more subtle questions about both the process and the product. For example, in addition to simply determining whether the mold will fill properly, it would be useful to predict the crystallinity and molecular orientation (and thus the mechanical properties) of the product.
A good example of the progress being made in the development of molecular theories is in the field of rheology, which is one of the basic sciences needed for the understanding of polymer processing. Rheology involves macroscopic shape changes of a polymeric fluid in complex transient stress and temperature fields. In rheological studies, well-defined stresses or strains are applied in order to measure and to predict the mechanical behavior of complex materials. The most important recent advances in polymer rheology came about in the 1970s, when P.G. de Gennes proposed a model for macromolecular motion that was then explored by M. Doi and S.F. Edwards to derive a rheological constitutive equation for the stress in macromolecular fluids. Many rheological phenomena have since been explained in terms of molecular parameters such as molecular weight, branching, chain stiffness, surface interaction, and polydispersity. Many of the current efforts in rheology focus on the basic understanding of the effects of phase transitions and anisotropy. Other major efforts concentrate on new continuum models that expand and fine-tune the predictions of Doi and Edwards. These initiatives are supported by advances in experimental rheology that give direction to the development of its theory.
CONCLUSIONS
-
Chemical products, in general, represent one of the few areas of the U.S. economy where the value of exports exceeds that of imports. Polymers contribute substantially to this positive balance of trade in chemicals. For example, plastics in both primary and nonprimary forms contributed $6.0B, or 37 percent, to the net positive trade balance in chemicals during 1992 (Chemical & Engineering News, 1993).
-
Plastics manufacturing is an important part of the national economy. While the commodity polymers represent a maturing industry, significant process and product innovations continue to be introduced and appear likely to lead to healthy growth rates for the foreseeable future.
-
A great deal of the current research and development on polymeric materials and associated processes is being driven by environmental considerations.
-
Research on the recycling of polymers has been ongoing for more than two decades, and considerable progress has been made in some areas. A great deal of effort has been devoted to developing automated processes for segregation
-
according to polymer type, and a uniform identification system has been agreed to by the industry to aid segregation by the consumer. Reprocessing of comingled waste leads to poor physical properties. Compatibilization may be technically possible, but it is quite expensive in relation to the value of the products produced. On the other hand, some plastic products appear to be easily recycled. For example, poly(ethylene terephthalate), which is used for soft drink bottles, is easily identified and is usually relatively free of contamination. A number of ways of reusing these materials have been identified, and some are in use. Perhaps the most significant are those that cause the polymer to revert chemically to oligomeric species that can be repolymerized into poly(ethylene terephthalate) or other polymeric products.
-
While innovations in polymer recycling are needed, other options must also be pursued. Opportunities include source reduction and design of products with recycling in mind. The potential value of biodegradable polymers as part of the solution to the solid waste and litter problem needs to be better understood. Such materials are likely to be much more expensive than the relatively inexpensive polymers currently used, and their performance may not be as good. It is not clear that any materials biodegrade in landfills. In any case, the release of the low-molecular-weight degradation products into the environment could lead to more serious air or water contamination concerns. Incineration for fuel value is another, and perhaps the ultimate, form of recycling of polymers. Most polymers are derived from oil, and about 95 percent of all the oil produced is burned for its energy value; thus oil converted into polymers is simply being borrowed for a while to be used as a material prior to returning to its ultimate fate of being burned for its energy. Of course, concerns about the impact of incineration on health and the environment need to be resolved.
-
Polymer interfaces are key to the performance of composites, blends or alloys, lubricants, adhesives, coatings, and thin films. Advances in the fundamental understanding of these interfaces and methods to engineer desired performance of these surfaces will no doubt lead to improved products and a competitive edge.
-
New engineering plastics, including some blends or alloys, with ever-increasing performance characteristics continue to be introduced and in many cases are being used for structural applications traditionally dominated by other materials, mainly metals. Ease of fabrication into dimensionally precise parts with high-quality surface finish is one driving force.
-
For polymer-based materials being used in highly critical structural applications, there is need for a better understanding of the mechanical, chemical, and environmental factors that affect their useful lifetimes and for methodologies to predict lifetimes in complex situations. Fracture mechanics techniques are not yet used to the same extent for polymers as for other structural materials.
-
There are opportunities for new polymer systems with controlled permeability properties for use in food packaging, medicine, clothing, agriculture, industrial
-
manufacturing, pollution control, water purification, and other applications. Innovative synthesis, knowledge of structure-property relations, and fabrication technologies will need to be integrated to achieve functional products that are cost-effective.
-
Instrumentation is emerging that makes possible real-time determination of chemical and physical structures during processing, allowing instantaneous comparison with process simulation and control models and quality assurance.
-
Rapid progress in the development of production processes is being made through implementation of a ''systems approach" wherein the materials, their composition, and the process are all considered variables in an iterative process. The systems approach, in comparison with the traditional "compartmentalized" approach, facilitates rapid identification of the critical material and processing parameters and aids manufacturing procedures.
-
The relationship between processing and properties, particularly for complex polymer systems such as blends, composites, and liquid crystalline polymers, is not fully developed. The role of rheology in the development of structure is at a particularly rudimentary stage, and it appears that significant advances could be possible. The development of real-time probes of structure during processing would be of immense value. Of course, a linkage must be made between the structure generated during processing and the performance of the product.
REFERENCES
Barbero., E., and H.V.F. Gangarao. 1991. "Structural Applications of Composites in Infrastructure." SAMPE Journal 27 (No. 6, Nov./Dec.):9.
Chemical & Engineering News. 1991. Vol. 69, No. 23, June 10, p. 39.
Chemical & Engineering News. 1992. "Facts & Figures for the Chemical Industry." Vol. 70, No. 26, June 29, pp. 62-63.
Chemical & Engineering News. 1993. "Facts & Figures for the Chemical Industry." Vol. 71, No. 26, June 28, pp. 38-83.
McDermott, J. 1993. Advanced Composites: 1993 Blue Book. Cleveland, Ohio: Advanstar Communications.
Modern Plastics. 1982. "Materials 1982: A Modern Plastics Special Report." Vol. 59, No. 1, January, pp. 55-87.
Modern Plastics. 1992. "Resins 1992: Supply Patterns Are Changing." Vol. 69, No. 1, January, pp. 53-96.
Pasztor, A. 1992. "Composites Makers Take Aim at Nondefense Markets; As Pentagon Budget Shrinks, Firms Seek to Dispel Cost, Safety Concerns." Wall Street Journal. August 26, pp. B3-B4.
Strathman, H. 1991. Effective Industrial Membrane Processes: Benefits and Opportunities, M.K. Turner, ed. New York: Elsevier.