4
Capabilities of Enhanced Pump-and-Treat and Alternative Technologies
Given the limited capabilities of conventional pump-and-treat systems and the large number of contaminated sites, a substantial market exists for innovative ground water cleanup technologies. However, use of innovative technologies has not been as extensive as might be expected, considering the potential size of the market. For example, while conventional pump-and-treat systems were selected for use at 73 percent of Superfund sites with ground water contamination through fiscal year 1992, at the remaining 27 percent of sites the most common "remedies" were not innovative technologies but nontreatment measures such as providing alternative water supplies, aquifer use restrictions, and wellhead treatment (Kelly, 1994; K. Lovelace, Environmental Protection Agency, unpublished data, 1992). Furthermore, technologies that treat ground water in place rather than extracting it were specified as remedies at fewer than 2 percent of Superfund sites (Kelly, 1994).
This chapter evaluates the capabilities of innovative subsurface cleanup technologies and reviews why application of these technologies has been limited. Included in addition to reviews of technologies that treat ground water below the water table are reviews of technologies that treat soils above the water table, because ground water cleanup cannot be achieved if contaminants from the overlying soil continue to migrate downward. While the Environmental Protection Agency (EPA) defines innovative technologies as those for which limited or no cost and effi-
ciency data exist, this report groups all technologies other than conventional pump-and-treat systems under the heading "innovative." It is important to note that some of the technologies reviewed in this chapter are gaining wider use. However, the increasing use of newer technologies applies mainly to soil above the water table. The most striking example of this desired technical evolution is the increased use of soil vapor extraction systems, which have now become a leading cleanup technology for soil (Kovalick, 1993). For cleaning up petroleum hydrocarbons, in situ bioremediation is also becoming increasingly common. Despite the increasing use of these two technologies, application of innovative technologies for cleaning up ground water remains rare.
In this chapter, the committee has divided innovative technologies into two categories: enhanced pump-and-treat systems and alternative technologies. Enhanced pump-and-treat systems all involve, to some extent, the pumping of fluids such as water, water solutions, or air and thus will face some of the same difficulties as conventional pump-and-treat systems; the advantage of these enhanced systems is their potential to significantly increase the rate at which contaminant mass can be removed from the subsurface. Alternative technologies do not involve continuous pumping.
For each of these technologies, the importance of thorough site characterization and field tests prior to implementation and of process monitoring after implementation cannot be overstated. Because of the lack of performance data for most of the technologies reviewed here, the uncertainty associated with these methods is proportionately greater than the uncertainty associated with conventional pump-and-treat systems. Thorough characterization of the site's geologic and chemical characteristics, field tests of the remediation method, and continual monitoring of the full-scale system are all essential steps for minimizing uncertainties. For innovative technologies even more than for conventional pump-and-treat systems, an observational approach to remediation—in which the design of the system evolves as information on field performance is collected—is a requirement for effective cleanup.
ENHANCED PUMP-AND TREAT SYSTEMS
Conventional pump-and-treat systems extract relatively large volumes of water with relatively low contaminant concentrations. Because of geologic complexity and slow rates of contaminant desorption and dissolution, these systems must displace many pore volumes of aquifer water to flush out contaminants, as explained in Chapter 3. Conventional pump-and-treat systems thus are inherently inefficient for removing contaminants from the subsurface. Many technologies currently being
developed or tested are designed to enhance the efficiency of pump-and-treat systems. Some of these technologies reduce the ultimate burden on the pump-and-treat system by removing from the soil contaminants that would otherwise migrate to the ground water or by removing volatile contaminants from the soil and ground water. Other innovative technologies improve the efficiency of contaminant extraction by increasing the amount of contaminant removed with each volume of pumped water. Another group of innovative technologies pumps minimal amounts of fluids to stimulate treatment of contaminants in place, either biologically or chemically, rather than requiring contaminant extraction and surface treatment. All of these technologies have in common the requirement to pump fluids through the subsurface, meaning that to varying degrees the geologic and chemical conditions that impose limitations on conventional pump-and-treat systems also present problems for these innovations.
The committee has divided enhancements to pump-and-treat systems into two categories: demonstrated technologies and technologies in development.
Demonstrated Technologies
The following technologies are all close to being accepted or are already accepted for site cleanups. They have been tested in laboratory-scale batch and column studies, in controlled field experiments, and in large-scale site trials. Data collection and analysis are comprehensive.
Soil Vapor Extraction
Description
Soil vapor extraction (SVE) is one of the few innovative technologies that has gained wide use. The technology extracts organic contaminants (primarily from the unsaturated zone) by flushing with air. Air flow is induced by applying a vacuum at a sealed wellhead or with blowers. Where the air stream contacts contaminants—which may be present as a nonaqueous-phase liquid (NAPL), dissolved in water in the soil pores, or associated with the soil—mass transfer to the air can occur, with subsequent transport of the air and contaminants to the surface. An incidental effect of SVE is that by increasing the subsurface oxygen supply, it can promote biodegradation of contaminants by aerobic microbes, although standard SVE systems are not specifically designed for this purpose.1
As shown in Figure 4-1, an SVE system usually consists of one or more extraction wells, vacuum pumps or air blowers, and a treatment system for the extracted vapors. In some cases, the ground is covered with an impermeable cap to improve system performance by controlling
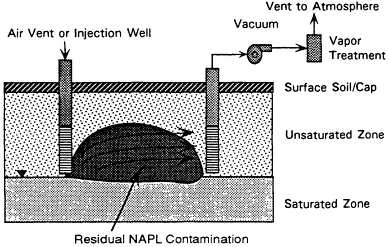
FIGURE 4-1 Process diagram for soil vapor extraction.
the direction of air flow and ensuring total capture of the extracted vapors. SVE also has been called soil venting, subsurface venting, in situ soil air stripping, and vacuum extraction. (For detailed descriptions of SVE systems, see Hutzler et al., 1989, and American Academy of Environmental Engineers, 1994.)
Application
SVE has proven effective for removing substantial quantifies of certain volatile organic contaminants from the unsaturated zone at a variety of sites in the United States and abroad. Numerous Records of Decision at Superfund sites have specified SVE as the technology of choice for unsaturated zone cleanup. The technique has also been extensively used for cleanups at gas stations and other sites where large quantities of volatile organic compounds have leaked from underground storage tanks. Although SVE also can remove contaminants from dewatered portions of the saturated zone, in which the water table has been purposely lowered through pumping, its use for this purpose has not been as extensive as for unsaturated zone treatment.
Conceptually, contaminant removal efficiency for SVE systems depends on the physical state of the contaminant. When present as a NAPL, contaminants will transfer from the pure liquid phase to the air via evaporation. If contaminants are adsorbed on or in the soil, then transfer must occur within the soil to the air-soil interface, with subsequent trans-
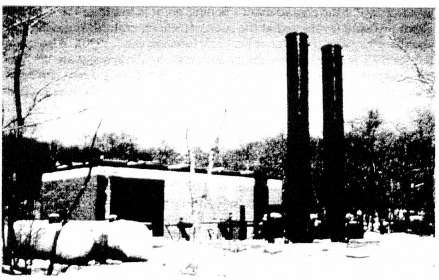
Vapor extraction facility that treats highly concentrated gasoline vapors from a large free-product plume. Courtesy of Peter Gerbasi, Roux Associates, Inc., Islandia, New York.
fer to the air stream. Finally, if the contaminants are dissolved in water in the soil pores, mass transfer must occur through the water-air interface. The rate of extraction thus depends in part on the efficiency of each of these molecular-scale mass transfer processes.
Based on this conceptual model, it is apparent that the efficiency of SVE depends strongly on contaminant and soil properties. Contaminant properties include vapor pressure, Henry's Law constant, hydrophobicity (usually quantified with the octanol-water partition coefficient), and diffusion characteristics. Soil properties include stratigraphy (for example, size distribution, permeability, and porosity), organic carbon content, mineralogy, and moisture.
The design of the SVE system also influences contaminant extraction efficiency. Principal design variables include the number of extraction wells, the rate of air flow (level of vacuum applied or rate of air injection), and the depth and length of the screened zone. Especially important to consider is the vapor flow path relative to the contaminant location. If the air stream bypasses zones of low permeability, the slow process of diffusion will dominate, making contaminant removal extremely slow.
As a rough rule of thumb for feasibility assessment, SVE is likely to
be successful if the contaminant's boiling point is less than 150°C or if its vapor pressure (evaluated at the subsurface temperature) is greater than about 5 × 10-4 atm (Hutzler et al., 1989; Johnson et al., 1990). Also essential for SVE is a soil permeability sufficient to allow adequate air flow. Typically, if the soil's permeability to air is less than 1 darcy (10-16 m2), flow rates may be too low to achieve successful removal in reasonable time frames. Because of the complex interrelationships among all the factors that influence SVE, the effectiveness of SVE should be evaluated carefully on a site-by-site basis.
Reviews of SVE systems in the United States show successful recovery of volatile organic compounds from the subsurface down to a depth of over 60 meters (see, for example, Hutzler et al., 1989; Buscheck and Peargin, 1991). Most of these systems addressed contaminant removal from the unsaturated zone rather than from dewatered portions of the saturated zone. Air flow rates ranged from 0.3 up to approximately 100 standard cubic meters per minute, with applied vacuums ranging from 0.0067 arm (5 mm of Hg) to 0.3 atm (230 mm of Hg). Unfortunately, the efficiency of the systems in terms of contaminant recovery was not reported. Typically, concentrations of volatile compounds in the extracted air stream decreased rapidly with time and approached asymptotic values similar to those seen in ground water pump-and-treat systems. Average removal rates for sites reviewed by Hutzler exhibited a narrow range: from 0.005 to 0.01 kg of contaminant per m3 of air extracted. Due to the apparent lack of detailed field investigations, a detailed assessment of SVE performance under controlled field conditions is needed.
Limitations
Flushing the subsurface with air, either injected or induced, is subject to the same limitations as flushing with water. The air stream is unlikely to flush zones of low permeability, which can contain significant quantities of contamination. In addition, SVE must overcome mass transfer limitations that inhibit the desorption of strongly adsorbed contaminants or contaminants that have penetrated the microstructure of the aquifer materials. Thus, all of the factors that inhibit release of contaminants during traditional pumping and treating also limit the performance of air flushing systems.
Advantages
A major advantage of air flushing versus water flushing is the higher fluid flow rates possible with air—provided that the soil permeability allows sufficient volumetric flow rates. Large numbers of pore volumes of air can be flushed through the subsurface in a short time, which permits recovery of a significant mass of released contaminants. Whether this increased flushing is sufficient to remove contaminants to acceptable levels is highly site specific.
SVE appears very promising for enhancing contaminant removal from dewatered sections of the saturated zone, although the degree to which this technology can remove contaminant sources has yet to be thoroughly evaluated. It is probable that SVE will be more successful at sites with light NAPLs (LNAPLs) than at sites with dense NAPLs (DNAPLs) because LNAPLs tend to remain above the water table, where they are more accessible, whereas DNAPLs tend to sink.
In Situ Bioremediation—Hydrocarbons
Description
In situ bioremediation systems stimulate subsurface microorganisms, primarily bacteria, to biodegrade contaminants. When given the proper stimuli, microorganisms can transform the contaminants to innocuous mineral end products, such as carbon dioxide and water. As explained in Chapter 2, the necessary stimuli for microbial growth in aquifers are oxygen or other electron acceptors (such as nitrate or sulfate) and nutrients (such as nitrogen and phosphorus). Typical in situ bioremediation systems therefore perfuse electron acceptors and nutrients through the contaminated region, as shown in Figure 4-2.
In situ bioremediation near the land surface can be achieved by using infiltration galleries that allow water amended with nutrients and electron acceptors to percolate through the soil. When contamination is deeper, in situ bioremediation systems inject the amended water through
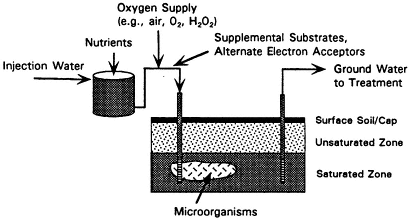
FIGURE 4-2 Process diagram for in situ bioremediation.
wells. As shown in Figure 4-2, some in situ bioremediation systems use extraction and injection wells in combination to control the flow of electron acceptors and nutrients and to hydraulically isolate the contaminated area.
The most common electron acceptor for the full-scale in situ bioremediation systems used today is oxygen, although in the future other electron acceptors (such as nitrate) may become more common. In situ bioremediation systems typically supply oxygen by bubbling air or pure oxygen into the injection water or by dosing the water with hydrogen peroxide. Alternately, they may supply oxygen by injecting air directly into the ground water, with nutrients added through injection wells or infiltration galleries.
Application
In situ bioremediation has been well established as a successful method for treating soil and ground water contaminated with certain types of hydrocarbons, primarily petroleum products and derivatives. In situ bioremediation was first successfully demonstrated for cleaning up subsurface petroleum hydrocarbons at a Sun Oil pipeline leak in Ambler, Pennsylvania, in 1972 (Lee and Ward, 1985). Since then, the technique has been used to clean up subsurface spills of refinery wastes, crude oil, and fuels. It has also been used to treat other easily biodegraded organic contaminants such as phenols, cresols, acetone, and cellulosic wastes. Although in situ bioremediation of other types of or-garlic contaminants, such as chlorinated solvents, is possible, the technology has not yet been demonstrated for these other applications.
Before an in situ bioremediation project is initiated, a specific microbial enhancement feasibility study and a general hydrogeologic site investigation are essential. The microbial study will help determine the types and amounts of substances required to stimulate optimum contaminant degradation. Site-specific geology and geochemistry also should be considered in project design. These parameters affect nutrient and electron acceptor availability, which may be hindered by sorption to the soils or reactions with naturally occurring subsurface chemicals.
Limitations
Like conventional pump-and-treat systems, in situ bioremediation systems are limited by geologic heterogeneities such as low-permeability zones, except the problem is reversed. For pump-and-treat systems, geologic heterogeneities limit the ability to extract contaminants, whereas for in situ bioremediation, geologic heterogeneities interfere with the ability to inject the necessary electron acceptors and nutrients. Adequate concentrations of electron acceptors and nutrients must be available to the bacteria throughout the contaminated zone to stimulate
growth; delivery of these growth-stimulating materials to zones of low permeability is difficult.
Mass transfer limitations that slow the dissolution of sorbed or NAPL contaminants and create problems for conventional pump-and-treat systems also interfere with in situ bioremediation. Microorganisms with the metabolic capability to degrade a contaminant will not do so if the contaminant is unavailable to the cell because it is contained in a NAPL or sorbed to subsurface particles. Slow dissolution from NAPLs and slow desorption from soils decrease the biodegradation rate, thereby increasing the cleanup time and the amount of chemicals that must be added to sustain microbial activity. Since there is currently no scientific consensus on what factors affect bioavailability or how bioavailability ultimately affects bioremediation, contaminant bioavailability must be considered on both a site- and a compound-specific basis.
Toxicity of contaminants to the microorganisms may also limit in situ bioremediation. Many contaminants are toxic to bacteria at high concentrations. For example, concentrations within a NAPL pool are likely to be toxic and restrict bioremediation to the periphery of the NAPL zone. Fortunately, the soluble concentrations of hydrocarbons normally observed at field sites are well below the toxic range.
Another limitation of in situ bioremediation is the requirement for a minimum contaminant concentration to maintain the microbial population and to induce the enzymes necessary for degradation. The existence of such a concentration threshold means that, theoretically, there is a minimum concentration below which no further bioremediation will occur. This minimum may exceed cleanup goals, particularly for heavier hydrocarbons. In studies of hydrocarbon biodegradation, minimum concentrations have ranged from 1 µg/liter to 1 mg/liter.
An additional limitation is the difficulty of delivering sufficient oxygen to the microorganisms because of oxygen's low water solubility. Injecting air directly into the ground water, rather than applying it in dissolved form in the nutrient-amended water, is one approach used to improve oxygen delivery.
Advantages
In situ bioremediation has four unique advantages over conventional pump-and-treat systems. First, while pump-and-treat systems extract contaminants to the surface for disposal or treatment elsewhere, in situ bioremediation treats contaminants in place and can convert them to innocuous products (such as carbon dioxide and water). As a result, in situ bioremediation reduces the requirement for surface treatment and disposal of the recovered water and minimizes the contaminant exposure hazard. Second, pumping requirements are likely to be lower for in situ bioremediation than for conventional pump-and-treat systems. The wa-
ter circulation requirements for delivering growth-stimulating materials to the subsurface are much lower than the requirements for attempting to flush out contaminants with a pump-and-treat system. Third, in situ bioremediation may be faster than conventional methods. Bioremediation at the periphery of a NAPL pool or on surfaces where contaminants are sorbed decreases the contaminant concentration near these remaining sources, increasing the dissolution rate. In addition, microbially mediated chemical transformations are generally faster than the same reactions in the absence of microorganisms. Fourth, certain microorganisms are able to move toward regions of higher contaminant concentration through a process known as chemotaxis, helping to expand the zone of biodegradation and eventually achieve complete treatment (Bosma et al., 1988)
Bioventing
Description
Bioventing is in situ bioremediation of the unsaturated zone. Like soil vapor extraction, bioventing involves inducing air movement through the unsaturated soil. However, the main purpose of bioventing is not to extract volatile contaminants but to enhance aerobic biodegradation of contaminants by supplying oxygen to soil microbes. Air flow requirements are therefore much lower for bioventing systems than for soil vapor extraction systems. Inorganic nutrients also may be added, if necessary.
As shown in Figure 4-3, the components of a bioventing system resemble those of a soil vapor extraction system, with the addition of a mechanism for nutrient delivery. Bioventing systems use air recovery wells either alone or with air injection wells. Since they are designed to promote biodegradation rather than physical removal of vapors, air recovery wells are located at the periphery of the contaminated area, and air flow rates are kept at the minimum rate required to deliver oxygen.
Application
Bioventing is used primarily for petroleum hydrocarbons and some chlorinated solvents. The technology is particularly useful in cases where excavation of the site is impractical, such as under buildings, where underground utilities are present, or where the contaminated soils are deep. Because bioventing requires air flow, it is more easily applied to permeable soils such as sand than to clays. Soil moisture levels are also an important parameter. Although biodegradation rates improve with high moisture levels, high soil moisture inhibits air movement.
Limitations
Where the natural nutrient supply is insufficient, nutrient addition can be problematic, especially at capped sites with low-perme-
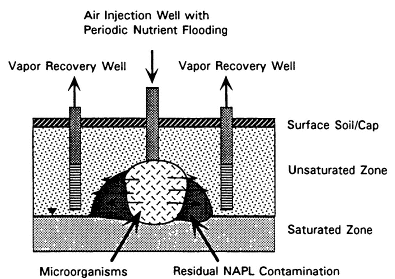
FIGURE 4-3 Process diagram for bioventing.
ability soils. Bioventing systems add nutrients in aqueous solution. The added liquid affects soil moisture content and, consequently, may inhibit air movement. The change in soil moisture can also affect the load-bearing capacity of the soil—an important consideration when treating soil under or near a building. In addition, flushing nutrients through the soil may transport contaminants from the unsaturated into the saturated zone. Researchers have investigated the possibility of using gaseous ammonia as a nitrogen source to eliminate these problems, but this method has not been very successful. To avoid causing unintended contaminant migration, before nutrients are added, the air circulation system can be operated for a period of time to biodegrade and/or physically remove the most mobile contaminants.
Another limitation of bioventing systems is that they may cause air quality problems if large quantities of volatile contaminants are vented to the atmosphere. Off-gas treatment may be necessary to meet local regulatory discharge limits.
Bioventing systems are also limited by the same factors mentioned for SVE. Significant masses of contaminants may remain in zones of low
permeability or in the microstructure of aquifer materials, even after bioventing.
Advantages
As for SVE, an advantage of bioventing is the greater ease of circulating air compared to circulating water. Soil permeability to air is two to three orders of magnitude greater than its permeability to water (Wilson and Ward, 1987). Furthermore, oxygen can be transported more easily in air than in water: at 15°C, air can transport 280 mg O2/liter, versus only 10 mg O2/liter for water sparged with air and 40 mg O2/liter for water sparged with pure oxygen. It is therefore possible to move relatively large amounts of oxygen with a bioventing system, even through soils with moderate to low permeabilities.
Technologies in Development
The following technologies are in the investigation and development stage. They have been tested in the laboratory, in controlled field experiments, and in some cases at a limited number of sites. However, they lack adequately documented cost and performance data. More controlled field studies and large-scale site trials are necessary to generate reliable performance data.
Pulsed and Variable Pumping
Description
The simplest enhancements to conventional pump-and-treat systems are pulsed and variable pumping. These methods intermittently slow or stop pumping to allow the contaminant concentration to build up, with the goal of increasing the mass of contaminant removed per unit volume of water pumped. Variable pumping differs from pulsed pump-Lug in that the pumping rate cannot be diminished to zero because hydraulic control of the plume is required.
As discussed in Chapter 3, pump-and-treat systems treat only dissolved contaminants. In conventional systems, the subsurface flow rates induced by pumping may be too rapid to allow enough time for sorbed or trapped chemicals to enter the bulk ground water for extraction. Pulsed and variable pumping allow more contact time between the moving water and residual contaminants that are sorbed, contained in NAPLs, or trapped in low-permeability zones. The increased contact time permits the system to approach chemical equilibrium, increasing desorption, dissolution, and diffusion.
Application
Pulsed and variable pumping have application for sites with large areas of residual contamination spread through the saturated zone
and for sites with pools of separate-phase organic liquids. The key design criteria are the duration of the cycle of maximum pumping and the duration of the cycle during which the pumping rate will be slowed or stopped. Like conventional systems, pulsed systems are most effective in homogeneous zones with high hydraulic conductivities (greater than about 10-5 cm/s). Pulsed pumping can work in heterogeneous, less permeable rocks, but with far longer projected cleanup times.
Limitations
Like conventional pump-and-treat systems, pulsed pumping systems are effective only in zones with sufficient permeability to sustain pumping. In addition, cleanup times may be long—presumably longer than for conventional pump-and-treat systems—because of the reduced extraction rate. Therefore, pulsed pumping may decrease the time efficiency of cleanup.
Advantages
Pulsed pumping may reduce total pumping requirements and costs, although its effectiveness is currently being debated and needs to be evaluated on a case-by-case basis.
In Situ Bioremediation—Chlorinated Solvents
Description
The physical components of in situ bioremediation systems for chlorinated solvent removal are the same as those for hydrocarbon removal: these systems use pumps, wells, and injection galleries to circulate materials through the subsurface to stimulate bacterial growth (see Figure 4-2). However, the metabolic processes for chlorinated solvent degradation are more complex than those for hydrocarbon degradation. Therefore, in situ bioremediation of chlorinated solvents often requires circulation not only of electron acceptors and elemental nutrients, but also of other growth-stimulating materials specific to the metabolic process by which the contaminants are degraded.
Application
Laboratory- and pilot-scale studies, along with a limited number of field tests, have documented two metabolic pathways for chlorinated solvent destruction. Each of these pathways requires different environmental conditions to proceed.
The first possible metabolic pathway is biodegradation by bacteria known as methanotrophs under aerobic conditions (i.e., in the presence of oxygen). Transformation by methanotrophs does not appear effective for compounds such as carbon tetrachloride and perchloroethylene that are fully substituted with chlorine atoms. However, research has shown that methanotrophic bacteria can transform chlorinated compounds such as trichloroethylene while using methane as their primary energy source
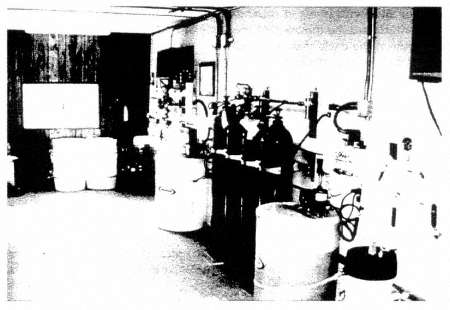
Equipment used to deliver nutrients and electron acceptors in an in situ bioremediation system. Courtesy of Ground Water Services, Houston, Texas.
(Haber et al., 1983; Wilson and Wilson, 1985). In the process of consuming the methane, the bacteria produce an enzyme, methane-monooxygenase, that incidentally transforms the chlorinated compound. Therefore, stimulating methanotrophic bacteria to transform chlorinated solvents requires adding methane to the site, in addition to oxygen and nutrients.
Methanotrophs have been tested for controlling chlorinated solvents at a limited number of field research sites. Semprini et al. (1990) alternately injected about 20 mg/liter methane and 32 mg/liter oxygen into a test plot at the Moffett Naval Air Station in Mountain View, California, to stimulate methanotrophs and evenly distribute their growth in the aquifer. They observed the following amounts of biodegradation by the active methanotrophs with fluid residence times of one to two days: trichloroethylene, 20 to 30 percent; cis-1,2-dichloroethylene, 45 to 55 percent; trans-1,2-dichloroethylene, 80 to 90 percent; and vinyl chloride, 90 to 95 percent. The same approach—injecting methane for in situ stimulation of methanotrophs to degrade chlorinated solvents—was employed in St. Joseph, Michigan, with equally promising results (McCarty et al., 1991).
The second possible metabolic pathway for chlorinated solvent destruction is biodegradation by a consortium of bacteria under anaerobic conditions (i.e., in the absence of oxygen). Anaerobic biotransformation
of chlorinated solvents has been widely observed in field studies (Roberts et al., 1982), in laboratory experiments using continuous-flow fixed-film reactors (Bouwer and McCarty, 1983; Vogel and McCarty, 1987; Bouwer and Wright, 1988), and in aquifer microcosms (Wilson et al., 1986). The initial step in the anaerobic biotransformation is generally reductive dechlorination. In reductive dechlorination, the chlorinated compound becomes an electron acceptor, and microbially catalyzed reactions replace a chlorine atom on the compound with a hydrogen atom. For reductive dechlorination to proceed, an electron donor, such as a low-molecular-weight organic compound (lactate, acetate, methanol, glucose, or toluene) or hydrogen (H2), must be available to provide reducing equivalents. Consequently, in situ bioremediation using reductive dechlorination requires the addition of an electron donor, in addition to nutrients.
Sometimes, reductive dechlorination is incomplete, and compounds that are less chlorinated than the original contaminant but still hazardous accumulate in the system. Commonly observed intermediates include vinyl chloride, chloroform, and various isomers of dichloroethene and dichloroethane. However, although some observers have reported the buildup of such intermediates, recent work has demonstrated that complete reductive dechlorination of trichloroethylene and perchloroethylene to ethene or ethane is possible under anaerobic conditions (DiStefano et al., 1991; De Bruin et al., 1992). Ethene and ethane are innocuous and are easily degraded by aerobic microbes. Consequently, complete detoxification of chlorinated solvents appears possible under certain anaerobic conditions.
Limitations
All of the limitations that apply to in situ bioremediation of hydrocarbons also apply to in situ bioremediation of chlorinated solvents. In situ bioremediation of chlorinated solvents also has limitations that are not factors in hydrocarbon bioremediation. Probably the major obstacle to using anaerobic processes is the possibility that hazardous intermediates will accumulate. A second limitation is the possibility of undesirable water quality changes. As conditions switch from oxic to anoxic, iron and manganese may dissolve. These metals cause taste and odor problems and stain pipes, bathroom and kitchen fixtures, and clothes. In addition, anaerobic organisms excrete metabolites that increase the concentration of organic matter. The organic matter may react with disinfectants used for drinking water purification to form hazardous byproducts such as trihalomethanes. The microbial metabolites may also dissolve cadmium, copper, lead, and zinc oxides, facilitating their passage into the drinking water distribution systems (Francis and Dodge, 1988).
The need for large chemical inputs to stimulate the organisms is a third limitation, applicable to both aerobic and anaerobic pathways. Both
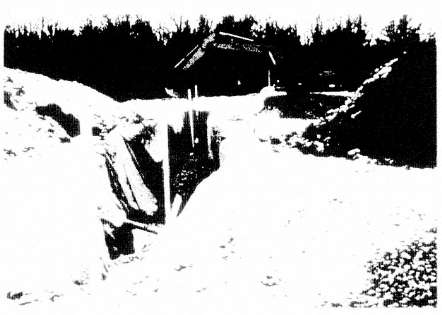
Construction of an infiltration trench for delivering fluids in an in situ bioremediation system. Courtesy of U.S. Environmental Protection Agency, R. S. Kerr Environmental Research Laboratory.
aerobic and anaerobic processes require the input of electron donors to provide the primary energy sources for the bacteria. Often, the mass of electron donor to the mass of chlorinated solvent biotransformed is extremely large, ranging from 100:1 to 1,000:1. Delivering these large quantities of chemicals to the proper locations is difficult at geologically complex sites and sites where the locations of contaminants are unknown. In addition, injecting large quantities of electron donors results in the buildup of large amounts of end products such as carbon dioxide, methane, and biomass—much more than in bioremediation of hydrocarbons under aerobic conditions. How to control these byproducts is an important question yet to be resolved. Biomass growth is likely to fill up the pore space, markedly reducing the formation's permeability. Plugging will in turn interfere with proper delivery of the required chemicals.
An additional limitation is that if appropriate microorganisms for carrying out the desired contaminant transformation are not present, then introduction of a specific population may be required. While most subsurface environments are inhabited by diverse microbial communities that can eventually adapt to the contaminants, for recalcitrant contaminants such as chlorinated solvents adaptation may require a long time,
and addition of nonindigenous microbes may be necessary to speed the process. However, introducing new microorganisms to the contaminated zone is difficult because the subsurface is an efficient filter medium that generally restricts microbial transport. In addition, the viability of introduced organisms—whether they will survive once injected in the subsurface—is poorly understood.
As for bioremediation of hydrocarbons, the extent to which this process can lower concentrations of chlorinated solvents is limited by the requirement for a minimum concentration threshold to stimulate microbial activity. In field trials, residual concentrations of chlorinated hydrocarbons have remained above typical health-based standards of 1 to 5 µg/liter (Major et al., 1991; Semprini et al., 1991). However, residual concentrations below 1 µg/liter have been observed in laboratory microcosms (Wilson et al., 1986). Consequently, it may be possible for optimized biotransformation systems to meet relevant regulatory end points.
A final potential limitation of anaerobic reductive dechlorination is the time required for the metabolic reactions to proceed. Aerobic cometabolism of chlorinated solvents by methanotrophs is rapid, with half-lives ranging from hours to days (Semprini et al., 1990). However, anaerobic reductive dechlorination rates appear to be slow, with half-lives on the order of weeks to months in acclimated laboratory systems (Bouwer and Wright, 1988). Extrapolation of optimal rates presently observed in the laboratory suggests cleanup times of years in the field. Such slow rates may be problematic with regulatory timetables.
Advantages
Like in situ bioremediation of hydrocarbons, in situ bioremediation of chlorinated solvents is advantageous because it has the potential to completely convert the contaminants to innocuous products, instead of simply extracting them for disposal elsewhere. In addition, the pumping rates for delivering growth-stimulating materials to promote bioremediation are lower than pumping rates for contaminant extraction. Finally, while in situ bioremediation using reductive dechlorination may be slow, in situ bioremediation using methanotrophs is relatively rapid.
In Situ Bioremediation—Metals
Description
The physical systems used to promote in situ bioremediation of metals are like those for bioremediation of hydrocarbons and chlorinated solvents (see Figure 4-2). However, while bacteria can destroy hydrocarbons and chlorinated solvents, they can change the form of metals but cannot destroy them. Therefore, in situ bioremediation of metals relies on manipulating bacteria to either dissolve the metals, which mobi-
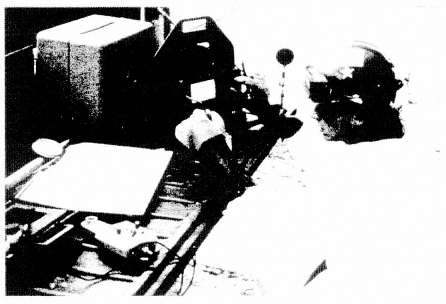
Measuring dissolved oxygen levels at a site being treated by in situ bioremediation. Courtesy of Ground Water Services, Houston, Texas.
lizes the metals for extraction with a pump-and-treat system, or to precipitate the metals, which immobilizes them to prevent their further migration.
Microbial activity against metals occurs primarily under anaerobic conditions. Anaerobic microorganisms can affect metal dissolution and precipitation by one or more of the following mechanisms: (1) direct enzymatic reduction of the metal, (2) biochemical alteration of conditions that influence the oxidation state of the metal, (3) excretion of microbial metabolites or decomposition products that can chelate or sequester the metal, and (4) bioaccumulation and release of metals elsewhere in the subsurface. At metal-contaminated sites, it may be possible to stimulate anaerobic microbial activity and control these mechanisms to influence the state of the metals.
Application
Of all the applications of in situ bioremediation, cleanup of metals is the least tested, with few published studies available. A nitrogen-fixing bacterial species isolated from coal-cleaning waste was capable of dissolving several metal oxides under anaerobic conditions (Francis and Dodge, 1988). This species dissolved iron (Fe2O3) and manganese (MnO2) oxides by direct enzymatic reduction. The species dissolved ox-
ides of cadmium (CdO), copper (CuO), lead (PbO), and zinc (ZnO) by indirect action from the production of metabolites and the lowering of the pH in the growth medium. Recently, researchers discovered anaerobic bacteria that rapidly reduce uranium(VI), which is soluble, to uranium(IV), which precipitates (Lovley and Phillips, 1992). Thus, it may be possible to immobilize uranium and other radioactive metal contaminants, such as plutonium and technetium, by stimulating microbial reduction of the metal in the subsurface (Lovley et al., 1991).
Limitations
All of the difficulties associated with introducing chemicals for stimulating microbial activity that apply to in situ bioremediation of hydrocarbons and chlorinated solvents also apply to in situ bioremediation of metals. In addition, the movement of metal contaminants mobilized by microbial activity must be carefully controlled to prevent detrimental migration. Finally, the long-term integrity of using microorganisms to immobilize metal contaminants is not well known.
Advantages
In situ bioremediation is particularly superior to ex situ methods because it eliminates the requirement for extracting the metal and treating it at the surface. It is advantageous over chemical treatment methods because microbial reactions with metals are often specific to the metal, whereas chemicals added to treat the metal may react with a variety of subsurface compounds. In addition, for some metals the microbial reactions are more efficient than equivalent chemical reactions at low contaminant concentrations.
Air Sparging
Description
Air sparging, also known as in situ aeration, uses circulated air to remove volatile contaminants. The principles upon which air sparging is based are similar to those for soil vapor extraction, except air sparging applies to the saturated zone while soil vapor extraction is primarily for the unsaturated zone. Air sparging systems inject air either directly into the aquifer formation or into specially designed extraction wells. Air is injected into the formation under pressure, where it displaces pore water and rises through the saturated zone into the vadose zone. The air stream must then be captured by a properly designed soil vapor extraction system and treated prior to release. Figure 4-4 shows a schematic diagram of one configuration for air sparging.
Conceptually, air sparging promotes contaminant removal by both physical and biological mechanisms. Dissolved volatile compounds partition into the air following Henry's Law. Volatile compounds present as NAPLs will also volatilize into the air stream. Adsorbed compounds
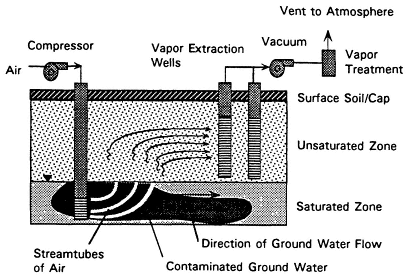
FIGURE 4-4 Process diagram for air sparging.
desorb directly into the passing air stream (a more rapid process than desorption into water). Air sparging also can enhance aerobic biodegradation of certain contaminants by increasing the oxygen content of the saturated zone.
Application
Air sparging has been tested in laboratories and at numerous field sites. A recent evaluation of air sparging that reviewed 21 published case studies concluded that air sparging is effective for removing substantial quantities of volatile aromatic and chlorinated hydrocarbons in a variety of geologic settings (Loden and Fan, 1992). In the case studies, air sparging reportedly reduced contaminant concentrations below 1 mg/liter within a matter of months. Another recent report of several case studies showed that air sparging can reduce benzene concentrations below regulatory levels (0.5 µg/liter) (Marley, 1992). Laboratory-scale tests using high volumes of air sparged vertically through small columns have demonstrated nearly complete removal of gasoline present as a NAPL and as a residual product in sand with an average diameter of 0.9 mm (Baehr et al., 1989).
As for soil vapor extraction, the effectiveness of air sparging depends
on the geologic characteristics of the site. The saturated zone must be sufficiently permeable to permit air flow. Empirical observations suggest that aquifers having hydraulic conductivities in either the horizontal or vertical directions of less than 10-5 cm/s will not be amenable to this technology (Brown, 1992). Others suggest a more stringent limit of greater than 10-3 cm/s as a prerequisite for sufficient subsurface air flow (Middleton and Hiller, 1990).
The soil grain size is also important. Typically, if the size of the porous medium exceeds 1 mm, air bubbles will migrate to the surface. For very large grain sizes, such as in gravel, the air bubbles will migrate to the surface so readily that the radius of influence of the air sparging wells will be very small, but this is an uncommon situation. On the other hand, for grain sizes less than 1 mm, air channels will form (Johnson et al., 1992). These air channels will bypass the less permeable zones in the aquifer, leaving substantial zones of residual contamination. It is probable that air channels will form in most applications of this technology because of the frequent presence of fine-grained (diameter less than 0.5 ram) soil in most aquifers.
The effectiveness of air sparging, like that of soil vapor extraction, also depends on contaminant chemistry. For dissolved contaminants, sparging appears to be effective when the Henry's Law constant is greater than 10-5 atm-m3/mole and the aqueous solubility is less than 20,000 rag/liter (Brown, 1992). For successful volatilization of contaminants present as NAPLs, experience suggests that the vapor pressure should be greater than 1 mm Hg (Angell, 1992).
Finally, performance depends on system design. Key design parameters include the injection air pressure, the number and spacing of injection wells, the volume of air to be injected, the depth of air injection, and the size of the screened section of the well. Most published data on installed systems reflect experience in relatively shallow contaminated aquifers (generally at depths less than 15 meters), with the exception of field tests conducted at the Department of Energy's (DOE's) Savannah River site (at which wells extend to depths of 45 meters) (Schroeder et al., 1992). Principal constraints on the use of air sparging at great depths are the uncertain flow path of the air stream and poor penetration of low-permeability layers within more permeable soils.
Other Configurations
An alternative to injecting air through vertical wells is to inject it through perforated pipes installed horizontally in the subsurface. Figure 4-5 shows a schematic of a horizontal well. Injected air flows upward through the saturated zone, volatilizing contaminants and carrying them into the vadose zone, where they are captured by a vapor extraction system (Angell, 1992). Horizontal wells appear to improve
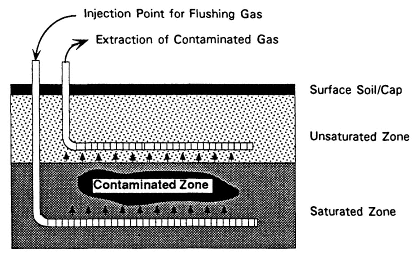
FIGURE 4-5 Process diagram for horizontal wells.
vertical transport of air bubbles through the aquifer, enhancing contact with the contaminants. The design parameters for horizontal wells are similar to those for standard vertical well air sparging systems.
A field trial conducted at the Savannah River site indicated that two horizontal wells were effective at removing a mixture of volatile chlorinated solvents (Looney et al., 1991). One well (90 meters long and 45 meters deep) was placed below the water table in a contaminant plume and was used to inject air and strip the contaminants from the ground water. The second horizontal well (50 meters long and 20 meters deep) was placed in the vadose zone to capture contaminants stripped from the ground water and to extract residual contaminants from the vadose zone. The system removed about 7,250 kg of contaminants during the 139-day test. The extraction efficiency with the two horizontal wells was five times higher than the performance of a vertical vacuum extraction well operated at the same test site. Significantly, the horizontal well system lowered costs by about 48 percent compared to a conventional pump-and-treat system with soil vapor extraction (Schroeder et al., 1992).
An alternative to injecting air into the aquifer is using in-well aeration. With this technology, air is injected into the well casing, where it strips volatile contaminants from the water as the water is extracted
through the well. The air is then captured at the ground surface and treated as required. Several technologies of this nature have been or are under development. In Germany, ground water remediation has been achieved using the so-called vacuum vaporizer wells (or UVB, the German acronym for this technology). The system consists of a unique well design coupled with an air injection system. The well contains two well screen sections, one near the bottom of the well and another just above the water table. Air is injected into the well casing beneath the water table, causing the water to rise and establishing a vertical circulation system with a radius of influence dependent on aquifer parameters, ground water flow, and rate of air injection (see Herrling et al., 1990, 1991a,b). Contaminants are stripped from the water in the casing, and the air stream is captured at the surface and treated. This technology is reportedly widely used in Germany, and several installations now exist in the United States. However, no thorough evaluation of the performance of the technology has been reported.
A second technology under development uses an air injection system in the well casing (Gvirtzman and Gorelick, 1992). Air is pumped into the well casing below the water table, which causes the water to flow upward. At the same time, rising air bubbles collect volatile contaminants from the water. The stripped water rises up the casing and is then deflected into a distribution channel, where it infiltrates back into the vadose zone. Theoretical calculations indicate that this technology will work, and a field test is imminent.
The major advantage of in-well technologies compared to other air sparging systems is that stripping occurs in the well, thus avoiding channeling problems. However, these technologies also must overcome the geologic and mass transfer barriers that interfere with the other types of treatment systems. Further development is expected.
Limitations
Although air sparging shows promise, particularly when the contaminants are volatile and biodegradable, significant questions remain about the ability of this technology to achieve health-based cleanup levels throughout the saturated zone. While laboratory studies of these technologies have been published, no peer-reviewed articles exist that demonstrate their efficacy in the field. Most of the claims of success have come from equipment vendors or engineering companies with a commercial interest in demonstrating success.
In addition to uncertainties stemming from a lack of peer-reviewed research, the technology has significant technical limitations. As with conventional pump-and-treat systems, diffusion-limited processes can slow cleanup. Contaminants trapped in low-permeability zones and dead-end pores will generally control the cleanup time.
Air sparging also has other limitations unique to the technology. Extracting multicomponent mixtures can be problematic. For example, a gasoline mixture will at first respond favorably to air injection, but the extraction efficiency will decline over time as the mixture's boiling point increases with the loss of the more volatile fractions. Use of air sparging at depths greater than approximately 10 meters below ground surface may also be problematic because the air flow path may be difficult to predict. If not properly controlled, air sparging can cause unanticipated contaminant spreading due to unexpected air flow paths. Finally, air sparging can cause dissolved ferrous iron to precipitate, resulting in clogged well screens and system failure.
Advantages
Air sparging can be advantageous over conventional pump-and-treat systems for removing volatile components of NAPLs because transfer of these contaminants to air is faster than transfer to water. In addition, pumping air is more efficient and less costly than pumping water because of the lower viscosity of air and the lower head loss incurred when pumping air. Air sparging also increases the likelihood of extracting contaminants by promoting mixing in the subsurface. An additional advantage of air sparging is the stimulation of aerobic bioremediation.
Soil Flushing
Description
Soil flushing enhances contaminant recovery in conventional pump-and-treat systems by increasing the quantity of contaminants transported with the pumped water. Soil flushing systems use injection wells, drains, or infiltration basins to flood the contaminated zone with chemical agents. The injected agents mobilize contaminants by increasing hydraulic gradients, reducing interfacial tension between NAPLs and water, increasing contaminant solubility, and/or reducing NAPL viscosity. Once the contaminants are mobilized, the system sweeps them to recovery wells or drains. At the conclusion of the flood, the flushing solution can be displaced to the recovery system by injecting water via the delivery system. Figure 4-6 shows a schematic of such a system.
Application
Soil flushing can be used to enhance recovery of contaminants with low water solubility, pools and discontinuous ganglia or globules of NAPLs, and sorbed contaminants. Two types of chemical agents can enhance contaminant recovery: cosolvents and surfactants.
Cosolvents are substances that, when mixed with water, enhance the solubility of some organic compounds. Examples are alcohols, such as methanol, and ketones, such as acetone. For example, in one set of tests
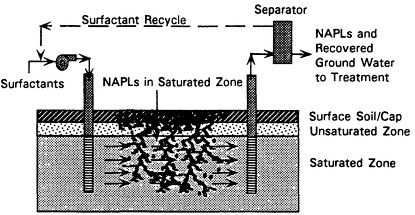
FIGURE 4-6 Process diagram for soil flushing.
the solubility of naphthalene in methanol-water mixtures increased by three orders of magnitude as the fraction of methanol increased from 0 to 1 (Fu and Luthy, 1986a). The injection of water-miscible cosolvents may therefore substantially increase the solubility and decrease the retardation factors of NAPLs, facilitating their extraction (Fu and Luthy, 1986b). Cosolvents that microbes can use as substrates may have the added advantage of promoting contaminant degradation by cometabolism if the cosolvents are used at nontoxic levels.
Surfactants are molecules with two structural units: one with an affinity for water (the hydrophilic portion) and one with an aversion for water (the hydrophobic portion). Hydrophobic contaminants may partition into the hydrophobic core of a surfactant, increasing their mobility in the water (Edwards et al., 1991). Surfactants are especially useful for dissolving NAPLs and enhancing NAPL mobility by lowering the interfacial tension between the NAPL and water. Surfactant solutions may also enhance recovery of sorbed contaminants.
The amount of organic contaminant mobilized during soil flushing depends on the chemical structure of the cosolvent or surfactant, the cosolvent or surfactant concentration, geochemical conditions, the chemical structure of the contaminant, and temperature. Cosolvent concentrations must typically be greater than 20 percent to cause effective mobilization. Surfactants require much lower concentrations—an advantage over cosolvents. At low concentrations, the surfactant exists predominantly in monomeric form. As more surfactant is added, a concentration range is reached, termed the critical micelle concentration (CMC), at which mono-
mers begin to assemble in ordered, colloidal aggregates known as micelles. At surfactant concentrations greater than the CMC, additional micelles form to incorporate the excess amount of surfactant added. Hydrophobic contaminants dissolve in the micelles (Rosen, 1989). If the micelles move with the water, they increase the ability to extract contaminants with the water. Surfactant flushing has been the subject of several laboratory investigations for removal of anthracene and biphenyls (Vignon and Rubin, 1989), petroleum hydrocarbons (Ellis et al., 1985), automatic transmission fluid (Abdul et al., 1990), and polychlorinated biphenyls (Abdul and Gibson, 1991).
Some researchers have suggested that surfactants may improve bioremediation by increasing the accessibility of the contaminant to microorganisms. However, surfactant-contaminant interactions must be considered to determine whether the surfactant solutions are toxic to the microorganisms. In one laboratory study, surfactants inhibited phenanthrene mineralization at surfactant concentrations in excess of the CMC (Laha and Luthy, 1991). The mechanism for this inhibition remains unclear. The inhibition may have resulted from surfactant molecules interfering with the microorganism's biochemistry or from limited bioavailability due to low contaminant exit rates from the micelies.
Limitations
As with conventional pump-and-treat systems, geologic conditions can limit the performance of soil flushing systems. Soil flushing systems, like conventional pump-and-treat systems, are most effective in permeable, uniform media. Heterogeneous and low-permeability soils with mixtures of contaminants will generally result in reduced sweep efficiency, longer project duration, and less successful recovery. As a result, complete mobilization and removal of NAPLs has not been observed in the field with normal working concentrations of the chemical amendments, even though ongoing laboratory studies with pure solvents and controlled surfactant additions have demonstrated that near complete mobilization is possible.
Soil flushing systems also have other limitations. Fluids containing the large amount of cosolvent required for NAPL mobilization have densities and viscosities that differ substantially from those of water, complicating prediction of transport behavior. Further, the movement of contaminants mobilized by the cosolvent or surfactant must be carefully controlled. Controlling contaminant movement generally requires pumping a larger volume of ground water than the soil flushing system injects; this excess volume of extracted water must be treated and discharged elsewhere. Finally, other potential adverse reactions caused by the solution, such as permeability reduction, coating of aquifer solids, and water
quality changes due to residual cosolvent or surfactant in the ground water, are also important to consider.
Advantages
Soil flushing systems may significantly increase the mobility of NAPLs, improving their removal rate. Because they increase the quantity of contaminants extracted with the water, these systems may reduce the cleanup time and total volume of water that must be extracted to achieve cleanup goals.
In Situ Chemical Treatment
Description
In situ chemical treatment uses chemicals to transform contaminants in place in the subsurface, as shown in Figure 4-7. Added chemicals can oxidize or reduce contaminants, converting them to nontoxic forms or immobilizing them to minimize contaminant migration. Possible oxidizing agents include hydrogen peroxide, Fenton's reagent, ozone, and potassium permanganate (Cho and Bowers, 1991). (Chlorine, while a strong oxidant, is not recommended because of the likely formation of chlorinated byproducts that pose a risk to human health.) Possible reducing agents include sulfur dioxide; sulfite salts (sodium bisulfite, sodium metabisulfate, or sodium hydrosulfite), ferrous sulfate, metallic iron and zinc, and sodium borohydride.
Application
Evidence on the application of in situ chemical treatment is very limited. Based on the existing evidence, it appears that this technology may be promising for treating the unsaturated carbon-carbon bonds in perchloroethylene (PCE) and trichloroethylene (TCE). For example, Farquhar et al. (1992) demonstrated that addition of potassium permanganate to PCE residual in soil completely destroyed the contaminant.
Chemical treatment also could enhance in situ bioremediation. Preoxidation of refractory organics with ozone or hydrogen peroxide can increase their biodegradability. One way to implement this approach is to use a vacuum extraction system with air or oxygen containing ozone. Ozonation of an organic compound usually creates compounds that are more polar than the parent compound (Hoigné, 1988) and thus often increases the compound's aqueous solubility and improves its bioavailability. Additional effects of ozonation include formation of hydroxyl, carbonyl, and carboxyl groups, loss of double bonds and aromaticity, and a shift to compounds with lower molecular weight. An advantage of coupling chemical and biological reactions is a reduction in the amount of oxidant required to destroy the compound.
Limitations
In situ chemical treatment has several potential limitations.
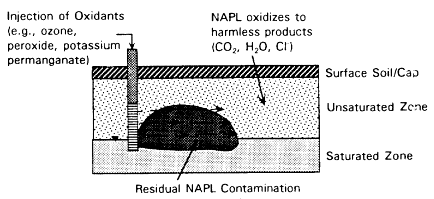
FIGURE 4-7 Process diagram for in situ chemical treatment.
First, like in situ bioremediation, this method is limited by geologic complexities that complicate delivery of chemicals to the contaminants. Second, the chemical reactions are often nonspecific, and the oxidant is capable of reacting with Fe(II), Mn(II), H2S, and other inorganic and organic reductants in the soil, in addition to reacting with the contaminant. As a consequence, chemicals must be added in amounts that far exceed the amount necessary to eliminate the contaminant. Third, chemical additions are likely to alter redox conditions and pH locally within the aquifer and cause undesirable side reactions. For example, chemical addition may cause precipitation of metals that may clog the aquifer and injection and extraction wells. Alternatively, chemical additions designed to immobilize a target metal may mobilize other metals, which then must be handled with another treatment process. Fourth, the reaction rates are often strongly dependent on the pH, and possible shifts in pH due to chemical treatment may cause a drastic reduction in contaminant removal rates. Finally, although theoretical reaction rates are fast, destruction efficiencies are in most cases insufficient to achieve health-based cleanup levels. A great deal more development work is needed to identify possible chemical reactions and their application in subsurface systems.
Advantages
Like in situ bioremediation, in situ chemical treatment reduces pumping requirements and worker exposure to contaminants by treating contaminants in place. In addition, chemical treatment can transform contaminants that resist biodegradation. Finally, because chemical oxidants and reducing agents are nonspecific, they may be suitable for treating mixtures of contaminants.
In Situ Thermal Technologies
Description
All of the physical, chemical, and biological phenomena that can be used to remove contaminants from the subsurface depend on temperature. In contrast to most remediation technologies, which operate at site-specific temperatures, in situ thermal technologies are designed to increase the temperature of either the unsaturated or saturated subsurface soil, thereby accelerating the various removal mechanisms. The added heat can either mobilize or transform contaminants to accelerate removal or, at very high temperatures (greater than 1000°C), permanently immobilize contaminants (a process known as in situ vitrification). Since rates of many physical and chemical properties increase with increasing temperatures, heat addition promises enhancements in both the rate and effectiveness of contaminant removal. Organic contaminants will mobilize more rapidly due to enhanced volatilization (if present as residual organic liquids), enhanced desorption from the soil, increased water solubility, and increased fluid flow rates (due to the reduced viscosity at higher temperatures). Higher temperatures can also accelerate biodegradation (within certain physiological temperature limits of the microorganisms) and certain abiotic transformation or decomposition reactions.
Application
Several technical alternatives exist for adding heat to the subsurface. Heat can be introduced by injection of heated fluids such as air, water, or steam. Alternatively, heated fluids can be pumped through buried pipes, with conductive heat transfer being the primary mechanism of heat addition. Other means of heat addition include installation of vertical electrodes that provide resistance heating by passing electrical current through the soil between the electrodes, with the amount of heat controlled by the amount of electrical current. Finally, heat can be added by installation of modified radio transmitters either vertically or horizontally into the formation, a process known as radio frequency heating. Such heating, analogous to microwave cooking, can raise the soil temperature well above the boiling point of water.
Of the numerous technical options, steam injection, radio frequency heating, and in situ vitrification have shown the greatest promise for subsurface remediation and have been tested at the field scale. Only steam injection and radio frequency heating are applicable to the saturated zone, however, and radio frequency heating would require dewatering of the contaminated zone. The capabilities, advantages, and disadvantages of these two technologies are described below. A useful review of these technologies can be found in Smith and Hinchee (1993).
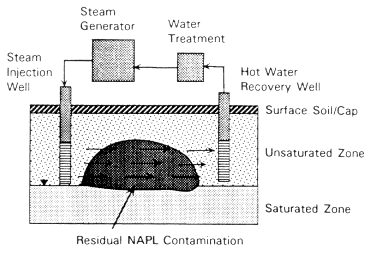
FIGURE 4-8 Process diagram for steam-enhanced extraction.
Steam-Enhanced Extraction
Description
Steam injection has been proposed as a technique to enhance the extraction of a variety of contaminants that are difficult or impossible to remove by water flushing. Steam injection is widely used in the petroleum industry to enhance petroleum extraction from deep formations. Extension of this technology to contaminant removal from the subsurface is a recent development, with active research at the laboratory and field scales under way at several universities and other institutions in the United States. In addition, several companies currently offer patented or patent pending techniques for steam injection and recovery for subsurface remediation (see, for example, EPA, 1991).
Conceptually, this technology uses the heat of steam to enhance contaminant mobilization. Steam is injected through vertical wells at appropriate depths, rates, and pressures (see Figure 4-8). As the steam passes through the subsurface, a steam front with three distinct zones forms. In the first zone, temperatures are near 100°C, and all compounds with vapor pressures greater than 1 atm at 100°C volatilize completely. Compounds with lower vapor pressures at this temperature will also volatilize at rates far higher than would be observed at normal subsurface temperatures but in proportion to their vapor pressures (Stewart and Udell, 1988; Udell and Stewart, 1990). In the second zone, steam and organic vapors condense due to heat transfer to the soil and fluids present
in the pore space. In this zone, a complex mixture of condensed steam, organic liquids, and the original water in the pores moves in the direction of the steam front. In the third zone, contaminants are present in their original distribution, awaiting the arrival of the moving steam front.
A significant practical issue associated with steam injection is recovery of the mixture of injected fluids and mobilized contaminants. Recovery can be accomplished with extraction trenches or extraction wells or through the use of vacuum extraction technology. Vacuum techniques are preferable because they prevent unintended contaminant spreading.
Application
This technology has been tested in laboratory columns and at a few field sites. Hunt et al. (1988a,b) report on nearly complete removal of several DNAPLs and LNAPLs by injection of steam into laboratory-scale columns. Lord et al. (1987) completely removed kerosene from sand columns using 126 pore volumes of steam injected at a temperature near 100°C. Hunt et al. (1988a,b) presented results of successful mobilization of NAPLs from laboratory-scale models of porous media.
While laboratory tests have shown nearly complete removal of volatile and semivolatile organic contaminants with steam injection, field tests have shown promise but have not resulted in complete contaminant removal, probably due to the presence of low-permeability zones through which the steam will not flow. Udell carried out a detailed assessment of steam technology to remove LNAPLs at a gasoline spill site (K. S. Udell, University of California, Berkeley, personal communication, 1993). Of the estimated 4,500 kg of gasoline in the subsurface, up to 40 percent could be recovered with steam injection. Udell and Stewart (1989) tested steam injection for removing volatile organic compounds (VOCs) from the saturated zone at a former solvent recycling facility; they reported recovery of substantial quantities (in the range of 100 kg) of VOCs, which were present in the dissolved, adsorbed, and organic liquid phases. Ghassemi (1988) reported reducing total petroleum hydrocarbon (TPH) levels in the unsaturated zone from over 1,000 mg TPH/kg of soil to the required level of less than 100 mg/kg. However, this report did not include a mass balance to determine the overall efficiency of the process.
Because steam injection is still in a developmental stage, only limited information is available on system design parameters. According to Udell and Stewart (1990), up to 350 pore volumes of steam may be needed to displace 1 pore volume of contaminated water. Reported steam injection pressures range from gauge pressures of 0.7 to 4 kg/cm2 (10 to 60 psi). Udell (personal communication, 1993) recommends that maximum steam injection pressure not exceed a gauge value of 0.1 kg/cm2 per meter of depth (0.5 psi per foot of depth) to the top of the injection interval in the well to avoid possible fracturing of the subsurface and the formation of
channels that would cause the steam front to short circuit the contaminated zone. Wilson and Clarke (1992) present a simplified model of the steam injection process that can be used to determine the optimum number and placement of injection wells. Information on the efficiency and design of this process is also available through the EPA's Superfund Innovative Technology Evaluation (SITE) program. Through this program, the EPA evaluated a patented steam injection system known as Toxic Treatment, which applies steam through an in situ soil drilling and mixing system. The evaluation indicated that treatment costs for this system may be less than for ex situ soil treatment.
Limitations
Although this technology should significantly enhance recovery of contaminants from the subsurface, provided that the mobilized contaminants can be effectively captured, limitations on achievable removal efficiencies can be anticipated. In the case of NAPL removal in a source area, some residual saturation is expected even in the permeable zones where significant steam flow can be achieved due to entrapment of the NAPL in small volumes of low-permeability soils. Steam extraction will also be limited by subsurface heterogeneities and the presence of contaminants in low-permeability zones. If such zones contain a significant fraction of the contaminant mass in the vadose or saturated zones, extensive remediation will be severely restricted because only a small fraction of the steam flow may pass through some of these areas, depending on the relative permeabilities of the zones. Other concerns regarding the efficiency of this technology include possible mobilization and loss of NAPLs into unanticipated channels and vapor loss through the surface. It is likely that this technology will also face a depth limitation controlled primarily by the difficulties in recovering the mobilized material at significant depths (greater than 30 meters).
Advantages
The increase in temperature provided by steam injection enhances contaminant recovery in several ways. Organic compounds with low boiling points (such as TCE and other common solvents) will vaporize, and semivolatile compounds (such as naphthalene and other polycyclic aromatic hydrocarbons) will partially evaporate. (For example, the vapor pressure of naphthalene increases from 1.4 mm Hg at room temperature to 389 mm Hg at 100°C.) The steam front will displace and mobilize low-viscosity fluids. The desorption rate for compounds attached to the soil particles will significantly increase, as will the rate of abiotic transformation processes. Steam can also enhance extraction of contaminants from low-permeability zones through conduction heating. All of these processes have the potential to significantly decrease cleanup time.
Radio Frequency Heating
Description
Radio frequency heating involves injecting heat into the subsurface through electromagnetic radiation generated at frequencies ranging from 45 Hz to 10 GHz, a frequency band allocated to the industrial, scientific, and medical sectors. Modified radio transmitters serve as the power source, with transmission through vertical tubular electrodes installed in the subsurface or horizontal electrodes positioned on the soil surface. Soil temperatures well above 80°C can be easily achieved. Water in the soil vaporizes, producing steam. Organic contaminants then vaporize, desorb, or decompose, with released contaminant gases captured through soil vapor extraction systems or with the use of a surface vapor barrier and appropriate appurtenances for gas management and treatment. A typical system consists of the radio frequency energy deposition array (electrodes), a radio frequency power generation source, vapor control, and a gas and liquid condensate handling and treatment system.
Application
Radio frequency heating is primarily applicable for removing contaminants with boiling points above 80°C from the unsaturated zone or from dewatered portions of the saturated zone. Examples include the longer-chain aliphatic and aromatic hydrocarbons, found in gasoline and jet fuel, and chlorinated solvents, such as tetrachloroethane, hexachlorobenzene, and tetrachloromethane. The technology has been under development since the early 1980s, principally at the Illinois Institute of Technology (see, for example, Dev et al., 1984). Currently, at least one patent on this process has been granted, and the technology is licensed by at least one remediation company. Bench, pilot, and field tests of the technology have been completed (Dev et al., 1987, 1988), with results published through the EPA's SITE program (Dev et al., 1989). Results have been reported for laboratory and field tests for removal of numerous organic contaminants, including hexachlorobenzene, tetrachloroethene, and JP-4 and JP-8 jet fuels.
Test results are too limited to provide detailed guidance on design, expected removal efficiencies, and estimated unit treatment costs. The key design parameter is the operating frequency of the radio frequency generator, determined on the basis of soil properties, contaminant properties, soil moisture, and speed of cleanup desired. Bench and pilot tests have reportedly achieved removal efficiencies above 90 percent. High removal efficiencies have also been reported in the field (Dev et al., 1989), but overall mass balances are difficult to make under these circumstances, and thus actual efficiency under real field conditions is still uncertain.
Limitations
Using radio frequency heating to treat dewatered zones may be problematic because of the residual water saturation. Field tests are limited, and application to heterogeneous subsurface environments may be difficult. As for other technologies, contaminants must still diffuse out of low-permeability zones to reach the gas capture system, and supplemental gas flow through soil vapor extraction may be required. Other limitations include the potentially negative impact of high soil temperature on the soil microbial population, effectively minimizing or eliminating biodegradation. Costs are uncertain, as is the durability of equipment under diverse field conditions. Further testing of the technology under controlled field conditions is necessary.
Advantages
Like steam-enhanced extraction, radio frequency heating may increase the contaminant removal rate by speeding contaminant volatilization and desorption.
ALTERNATIVE TECHNOLOGIES
Pump-and-treat systems require a continuous energy input for pumping fluids. These systems thus require continuous site management and maintenance, which are generally expensive processes. Non-pumping approaches that do not require a continuous energy input are being developed. The approaches under investigation rely on the aquifer's intrinsically favorable conditions for biodegradation, contain the contamination, or use hydraulic barriers to direct ground water flow through a reactive medium. While these methods show promise, they are in the development stage and have not yet been demonstrated.
Intrinsic Bioremediation
Description
Intrinsic bioremediation is essentially in situ bioremediation without human intervention. In this approach, the native microbes transform contaminants without stimulation from added electron acceptors, nutrients, or other materials (although these materials must be present naturally for biodegradation to occur). The biodegradation processes in intrinsic bioremediation are the same as those occurring in the engineered bioremediation systems discussed earlier in this chapter, but nothing is used to accelerate these natural processes. In most cases, such intrinsic bioremediation supplements conventional remediation techniques. For example, prior to use of intrinsic bioremediation, removal of the contaminant source may be necessary to prevent toxic inhibition of microbial activity. Similarly, conventional pump-and-treat systems may be used to
lower the contaminant concentration, with intrinsic biotransformation allowed to complete the remediation after the pumps are turned off.
Although intrinsic bioremediation does not involve active site manipulation, it requires construction and maintenance of a monitoring system. The monitoring system should include interior wells to monitor the plume distribution and indicator parameters of biodegradation, such as dissolved oxygen concentration, changes in redox potential, pH, and availability of nutrients. It should also include wells outside the contaminated area to monitor potential off-site migration and determine if additional remedial measures are required.
Application
Many recent studies have demonstrated that diverse microbial populations indigenous to subsurface environments can degrade important classes of organic contaminants. However, whether intrinsic bioremediation is sufficient to eliminate the contamination is highly site specific. The extent of intrinsic bioremediation depends on the biodegradability of the contaminant and on the site's hydrogeologic and chemical characteristics.
A number of studies have demonstrated that plumes of dissolved hydrocarbon contaminants may attenuate without human intervention. Creosote biodegradation was observed in contaminated ground water at a former wood preserving facility in Conroe, Texas. Contaminant removals correlated with the availability of dissolved oxygen (Wilson et al., 1985). Field studies at a contaminated aquifer in St. Louis Park, Minnesota, showed that methane production was occurring in zones within the aquifer that had been contaminated with creosote, indicating biodegradation by methane-producing organisms (Godsy et al., 1983). Similar work at an abandoned creosote plant in Pensacola, Florida, demonstrated that a wide variety of organic compounds present in the aquifer was undergoing biodegradation by methane-producing organisms. Transport distances in the aquifer correlated with biodegradation rates derived from laboratory batch experiments (Goerlitz et al., 1985). At a gasoline plant in northern Michigan, investigators have observed intrinsic biodegradation of benzene, toluene, and xylene (Chiang et al., 1989). The biodegradation rate at this site closely matches the rate that researchers predicted in modeling studies.
In general, four types of hydrogeologic and geochemical characteristics determine whether relying on intrinsic biodegradation instead of an engineered cleanup system may be possible (National Research Council, 1993). First, the ground water flow direction should be predictable and consistent throughout the seasons. Predictability of flow is necessary to analyze whether the native microbes will degrade the contaminant more quickly than it spreads. Second, the site must have a concentration of
electron acceptors—such as oxygen, nitrate, sulfate, or ferric iron—sufficient to support increased microbial growth. Third, the site should have adequate capacity to buffer against pH changes that may occur with the increased microbial activity. Carbonate minerals such as limestone, dolomite, and shell material can provide buffering capacity. Finally, the site must have a natural supply of the elemental nutrients (especially nitrogen and phosphorus) necessary for microbial metabolism.
Limitations
At present, very little operating history exists to judge the effectiveness of intrinsic bioremediation. While at many low-priority sites regulators may have assumed that intrinsic biodegradation would adequately control contaminant migration, very few of these sites have been monitored sufficiently to determine if this approach is actually effective or to identify factors that influence the efficiency of intrinsic bioremediation. Use of intrinsic bioremediation involves somewhat greater risk of failure than engineered bioremediation because active measures are not used to control plume migration. Reliable methods need to be developed for predicting the effectiveness of intrinsic biodegradation (National Research Council, 1993).
Use of intrinsic bioremediation is also subject to institutional limitations. Regulators, environmental groups, and the public may be unwilling to accept this approach, which they may perceive as equivalent to doing nothing. In addition, a contaminant plume left in place to biodegrade intrinsically may migrate under an adjoining property. Adjoining property owners are likely to have strong concerns about the potential risks and effects on property values posed by plume migration.
Advantages
Intrinsic bioremediation minimizes treatment costs by requiring little or no energy input. In addition, at sites where contaminants have low mobility, it eliminates the chance of remobilizing contaminants or causing additional contamination by pumping.
Containment Technologies
Description
Because of the limitations of conventional pump-and-treat systems, interest in physical containment technologies has increased, both for isolating contaminant source zones and containing contaminant plumes. Contaminated soil and ground water can be physically isolated with low-permeability barriers such as caps, liners, and cutoff walls. Contaminated soil can also be contained by solidifying it in place with either chemical fixatives or extreme heating (a process known as vitrification). Containment systems for ground water frequently include low-flow
pump-and-treat systems to help control ground water flow and prevent contaminated water from escaping the confined area.
Application
Soil and ground water can be contained with barriers made of a variety of materials. The key requirement is that the material have a lower permeability than the aquifer. Typical materials include compacted clay, synthetic plastics (known as geomembranes and used as liners), soil and bentonite mixtures, cement and bentonite mixtures, and sheet piling. A barrier that completely encircles a contaminated region will provide better containment than nonencircling walls. Straight walls have limited effectiveness because ground water can flow around the ends of the walls.
New developments in the technology for constructing sheet pile cutoff walls have significantly reduced the potential for leakage through these walls (Starr and Cherry, 1992). Sheet piles can be made of interlocking steel, precast concrete, or wood sections. Sheet pile cutoff walls are constructed by driving individual sections into the ground using pile drivers. The interlocks can be grouted to seal the joints between sheets. This configuration can completely prevent advection of contaminated ground water through the cutoff wall.
As a possible alternative to installing physical barriers, soil can be solidified in place by mixing it with cementing agents. Possible cementing agents include pozzolan-portland cement, lime-flyash pozzolan, and asphalt. Complete mixing of the cementing agents with the contaminated soil is necessary for successful containment. Soil can also be solidified by heating it into a molten mass that solidifies upon cooling, a process known as in situ vitrification. During in situ vitrification, an electrical input heats the subsurface to between 1600 and 2000°C. The high temperatures pyrolyze organic contaminants, and vapors can be captured at the land surface for treatment. Inorganic contaminants and ash remain in the solid material formed from the molten mass. Solidification and in situ vitrification are most effective for contamination at shallow depths.
Limitations
The long-term performance of physical barriers is uncertain. Construction difficulties are common. For example, sheet piling is difficult to install in rocky soils, and questions remain about the ability to key into the confining layers below the aquifer without creating leaks in the formation. The layer underlying the aquifer must be reasonably free of flow channels to maintain the integrity of the barrier.
The long-term stability and leaching characteristics of contaminated materials that have been solidified or vitrified are unknown. In addition, vitrification may cause volatilization, mobilization, and migration of contaminants.
Advantages
Cutoff wall enclosures may be useful for sites where present technologies cannot clean up contaminant source areas. They can be used for pilot-scale evaluations of in situ remedial techniques and can improve the effectiveness of enhanced pump-and-treat methods. By isolating a portion of the subsurface, the enclosure can prevent off-site migration of treatment fluids and contaminants mobilized by the remedial process. The enclosure also can improve the effectiveness of the remedial process by minimizing dilution of the treatment fluids and by facilitating control of flow directions.
In Situ Reactive Barriers
Description
In situ reactive barriers treat the contaminant plume as it passes through permeable reactive zones or walls within the aquifer. As natural or induced hydraulic gradients move the water through the reactive zone, materials in the reactive zone remove or degrade the contaminants, leaving uncontaminated water to emerge from the downstream side. Figure 4-9shows a schematic of one possible configuration for an in situ reactive barrier, the ''funnel and gate.''
Application
Several approaches for installing the reactive wall or treatment zone are possible (Gilham and Burris, 1992). One approach, limited to shallow depths, is to excavate and backfill a trench with the reactive material. A second approach is to use slurry wall construction technology to create a deeper and larger permeable curtain. In this approach, a polymer mixed with reactive materials replaces subsurface materials as
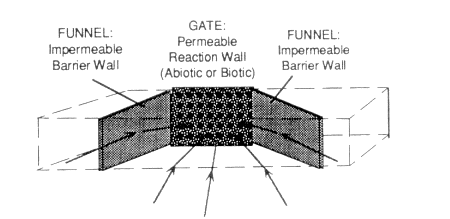
FIGURE 4-9 Process diagram for in situ reactive barriers.
excavation proceeds. When excavation is complete, the polymer is removed by pumping and biodegradation, leaving a permeable wall containing the reactive material. A third approach is to temporarily install sealable sheet piling to allow dewatering and construction of a reactive zone. For these three approaches, costs are likely to be high if a continuous wall is installed across zones of contaminated water. A promising alternative is to use sealable sheet piling to funnel the natural ground water flow to narrow openings in the wall in which the reactive material has been installed, as shown in Figure 4-9. This method provides greater control of the treatment zone and facilitates replacement or removal of the reactive material. Principles of reactor engineering can be applied to design the appropriate reaction zone length.
The reactive zone can use a combination of physical, chemical, and biological processes. One possible physical method uses granular activated carbon to adsorb organic contaminants. Other possible physical/chemical methods use redox controls to precipitate metals and metal catalysts to degrade halogenated organic compounds (Gilham and O'Hannesin, 1991). For example, in one set of laboratory studies a calcium apatite barrier system precipitated lead (Pb2+) as lead phosphate via phosphate dissolution from the apatite (Xu and Schwartz, 1992). In another study, iron-bearing solids in a laboratory soil column removed chromate (CrO42-) by reducing chromium(VI) to chromium(III).
Another possibility is to add nutrients and/or bacteria to the reaction zone to enhance contaminant biodegradation. Researchers are currently investigating several possible methods for creating biological reaction zones. For example, some researchers have tested biological removal of nitrate from ground water by periodically injecting a readily biodegradable organic compound to stimulate denitrifying bacteria, which convert nitrate to nitrogen gas (Gilham and Burris, 1992). Other researchers have used denitrifying bacteria to oxidize aromatic compounds while using nitrate as an electron acceptor (Hutchins et al., 1991). In tests at a commercial site in North Carolina, researchers have used a series of wells packed with briquettes that slowly release oxygen to stimulate aerobic bacteria that degrade gasoline components (Borden et al., in press). Researchers at Lawrence Livermore National Laboratory are testing ways to inject methanotrophs and methane to create an in situ microbial filter for passive decontamination (Taylor et al., 1992).
When barriers use exhaustible material such as granular activated carbon or chemical reactants, they must be installed as modules to enable periodic replacement.
Limitations
The technology of reactive barriers is in the conceptual and development stages, with the principles being studied on a small scale.
Two engineering challenges for the use of permeable reaction walls are installation of the reactive materials and provision of suitable amounts of these reactive materials. Using reactive barriers without source cleanup also raises institutional concerns because the barriers do not completely remediate the site.
Advantages
Like intrinsic bioremediation, reactive barriers lower costs by requiring little or no energy input once installed. Yet, because they capture the contaminant plume instead of allowing it to follow its natural course, they have the potential to be safer and more reliable than intrinsic bioremediation. In addition, because the reaction zone is limited in area, reactive barriers may be easier to design, monitor, maintain, and control than other systems.
IMPORTANCE OF COMBINING PROCESSES
Waste sites are rarely characterized by a single contaminant. Mixtures of contaminants with varying physical and chemical properties, such as chlorinated solvents and polycyclic aromatic hydrocarbons, chlorinated solvents and phenols, gasolines and acetone, and any organic contaminant with one or more heavy metals, are much more likely to be present. Because a single remedial process may be effective at removing only a subset of the compounds in a waste mixture, a combination or sequence of processes is likely to be necessary. From this perspective, each remedial technology should be treated as part of a treatment train— analogous to the sequence of processes used to treat drinking water or wastewater. More work is needed to develop combinations of treatment processes for addressing contaminant mixtures.
One simple example of combining processes is the use of bioventing. Bioventing uses vacuum extraction together with in situ biodegradation to remove contaminants, as shown in Figure 4-3. Vacuum extraction removes separate-phase globules of the contaminants and the bulk of the volatile contaminants, while biodegradation handles residual concentrations. Box 4-1 describes another example of combining processes. At the site described in the box, the remedial goal was achieved by a combination of free product recovery, in situ biodegradation, and venting/air stripping.
RELATIVE EFFECTIVENESS OF ENHANCEMENTS AND ALTERNATIVES
As for conventional pump-and-treat systems, the effectiveness of a specific alternative treatment technology is strongly related to site condi-
BOX 4-1 TREATMENT TRAIN FOR GASOLINE CLEANUP—LONG ISLAND, NEW YORK This example illustrates how the most effective strategy for cleaning up subsurface contamination often employs multiple technologies. At this site, free-product recovery, soil vapor extraction, in situ bioremediation, and pumping and treating were all combined to remove spilled gasoline from a Long Island, New York, aquifer. (For details, see Lee and Raymond, 1991.) The gasoline at this site originated from a slow leak in an underground storage tank at a filling station. An estimated 106,000 kg leaked, creating a plume that reached a residential subdivision and released fumes into the basements of local homes. When the spill was discovered, the owners of the station initiated a response program and extracted more than 82,000 kg of the gasoline—more than 77 percent of what had spilled—using free-product recovery methods that skimmed the gasoline from the water table. Samples after the free product was recovered showed that approximately 24,600 kg of gasoline remained in the subsurface in a 2.3-meter vertical section above and below the water table. In the next stage of cleanup, a soil vapor extraction system was installed to remove volatile contaminants from the soil and in situ bioremediation and pump-and-treat systems were installed to remove ground water contaminants. The in situ bioremediation system supplied oxygen via hydrogen peroxide and nutrients via a proprietary solution to stimulate indigenous organisms to degrade the gasoline. Monitoring showed a dramatic increase in the number of gasoline-degrading organisms corresponding with the oxygen additions and the decrease in gasoline concentrations. The ground water extraction system removed remaining volatile contaminants by air stripping. Over an eight-year treatment period, the combined system removed nearly 99 percent of the 24,600 kg of residual gasoline. The remaining 270 kg of gasoline were confined to a 0.61-meter vertical zone occupying a small area. Levels of gasoline in soil and ground water outside this zone were undetectable. Investigators at the site estimated that the air stripper used to treat the extracted water removed 6,240 kg, or 25 percent, of the gasoline. The soil vapor extraction system removed an estimated 450 kg, or 1.8 percent, of the gasoline. The majority—17,640 kg, or 72 percent—of residual gasoline was removed by in situ bioremediation. REFERENCE: Lee and Raymond, 1991. |
tions and contaminant chemistry. Table 4-1 gives the relative effectiveness of the enhancements and alternatives described in this chapter as a function of contaminant chemistry. The conditions ranked 1 represent those for which the technology is most effective, while those ranked 4 represent conditions for which the technology is least effective. The rankings are based largely on theoretical potential effectiveness, with some input from field experience where applicable. With contaminants that are volatile or biodegradable (columns 1 and 3), a relatively high degree
TABLE 4-1 Relative Effectiveness of Alternative Treatment Technologies as a Function of Contaminant Chemistry for Average Geology
|
Contaminant Chemistry |
|||||
Alternative Technology |
Mobile, Dissolved (degrades/ volatilizes) |
Mobile, Dissolved (nonreactive/ nonvolatile) |
Sorbed, Dissolved (degrades/ volatilizes) |
Sorbed, Dissolved (nonreactive/ nonvolatile) |
Separate Phase LNAPL |
Separate Phase DNAPL |
Conventional pump and treat |
1a |
1 |
2 |
2 |
2-3 |
4 |
Vacuum extraction and bioventing |
NAb |
NA |
2 |
NA |
2 |
3 |
Air sparging (vertical or horizontal wells) |
2 |
NA |
2 |
NA |
2 |
3 |
In situ bioremediation—hydrocarbons |
1 |
NA |
2 |
NA |
2-3 |
3-4 |
of effectiveness (ranking 1 to 2) is expected using vacuum extraction, air sparging, in situ bioremediation, and steam stripping. Treatment is typically less effective (ranking 3 to 4) for sorbed contaminants or contaminants present as separate phases because of the slow rates of desorption and dissolution for such contaminants.
In Table 4-1, the hydrogeology corresponds to homogeneous multiple-layer formations or heterogeneous single-layer formations analogous to the middle rows in Table 3-2. The table includes technologies for cleaning up the unsaturated zone and technologies for cleaning up the saturated zone (some technologies apply to both). Technologies not applicable for certain contaminants are marked "NA." For example, bioremediation would not be employed for contaminants that do not degrade, and processes relying on volatilization, such as vacuum extraction, air sparging, and steam stripping, would not be employed for nonvolatile contaminants.
Table 4-2 summarizes the relative performance of the various treatment alternatives for cleaning up contaminant plumes, and Table 4-3 shows relative performance for cleaning up contaminant source areas. In these tables, plume remediation refers to treatment of dissolved contaminants emanating from contaminant source areas. Source remediation refers to treatment in the immediate vicinity of where the contaminant entered the subsurface, which typically contains separate-phase organic liquids and/or high concentrations of metals; it also refers to treatment of pools of NAPLs and other large accumulations of undissolved contaminants along the contaminant flow path. The tables give expected performance in terms of the residual contaminant concentration in the ground water, the residual contaminant concentration sorbed to solids in the aquifer or source area, and cleanup time. The relative number of peer-reviewed publications is an indicator of the maturity of the technology.
In Tables 4-2 and 4-3, the technologies listed as leaving "low" residual contaminant concentrations and as having "short" cleanup times perform much more effectively than those leaving ''high" residual concentrations and requiring "long" cleanup times. However, depending on the site characteristics and contaminants, the performance of a specific technology can vary widely. Consequently, the tables show considerable overlap and similarity in the performance designations for the different technologies.
BARRIERS TO IMPLEMENTATION OF INNOVATIVE TECHNOLOGIES
Although the innovative technologies described in this chapter show promise for improving the effectiveness and reducing the costs of haz-
TABLE 4-2 Relative Performance of Alternative Technologies for Plume Remediation
Alternative Technology |
Residual Ground Water Concentration |
Residual Sorbed Concentration in Aquifer |
Cleanup Timea |
Number of Peer-Reviewed Publicationsb |
Conventional pump and treat |
Low |
Medium to high |
Long |
Many |
Air sparging (vertical or horizontal wells) |
Low to medium |
Low to medium |
Medium to long |
Limited |
In situ bioremediation— hydrocarbons |
Low to medium |
Low to high |
Medium to long |
Some |
In situ bioremediation— chlorinated solvents |
Low to medium |
Low to high |
Medium to long |
Some |
In situ bioremediation—metals |
Low to medium |
Low to high |
Medium to high |
Limited |
In situ reactive barriers |
Low |
NA |
Long |
Limited |
Intrinsic bioremediation |
Low to medium |
Low to medium |
Long |
Limited |
NOTE: A "low" residual concentration and "medium" cleanup time reflect relatively good performance, while a "high" residual concentration and "long" cleanup time reflect much less effective performance. "NA'' denotes that the technology is not applicable to this situation. a Because few cases of achieving cleanup goals have been reported, these qualitative assessments reflect the judgment of the committee. b "Limited" indicates that very little information about this technology is available in peer-reviewed publications, while "some" indicates a greater availability of information. |
TABLE 4-3 Relative Performance of Alternative Technologies for Source Remediation
Alternative Technology |
Residual Ground Water Concentration |
Residual Sorbed Concentration in Source Area |
Cleanup Timea |
Number of Peer-Reviewed Publicationsb |
Conventional pump and treat |
Low to medium |
Medium to high |
Long |
Some |
Vacuum extraction and bioventing |
NA |
Low to medium |
Short |
Some |
Air sparging (vertical or horizontal wells) |
Low to medium |
Low to medium |
Short to medium |
Limited |
In situ bioremediation— hydrocarbons |
Low to medium |
Low to high |
Short to medium |
Some |
In situ bioremediation— chlorinated solvents |
Low to medium |
Low to high |
Medium to long |
Some |
Alternative Technology |
Residual Ground Water Concentration |
Residual Sorbed Concentration in Source Area |
Cleanup Timea |
Number of Peer-Reviewed Publicationsb |
Cosolvent and surfactant flushing |
Low to medium |
Low to medium |
Short to medium |
Some |
Steam stripping |
Low to medium |
Low to medium |
Short |
Some |
In situ thermal desorption |
Low to medium |
Low to medium |
Short |
Some |
In situ chemical oxidation |
Medium (?) |
Medium to high (?) |
Medium (?) |
Limited |
In situ bioremediation—metals |
Low to medium |
Low to high |
Medium to high |
Limited |
Intrinsic bioremediation |
Low to medium |
Low to high |
Long |
Limited |
NOTE: A "low" residual concentration and a "short" cleanup time reflect relatively good performance, while a "high" residual concentration and a "long" cleanup time reflect much less effective performance. ''NA" denotes that the technology is not applicable to this situation. (?) denotes a high degree of uncertainty regarding the performance of this technology. a Because few cases of achieving cleanup goals have been reported, these qualitative assessments reflect the judgment of the committee. b "Limited" indicates that very little information about this technology is available in peer-reviewed publications, while "some" indicates a greater availability of information. |
ardous waste site cleanups, it has been widely recognized that numerous barriers have obstructed the implementation of these innovations, and significant efforts are under way by federal agencies and others to accelerate their use. Nevertheless, barriers remain in all three key phases in the life cycle of an innovative technology: the technology development phase, the selection phase, and the procurement or implementation phase.
Technology Development Phase
The first key phase in the life cycle of an innovative technology is the development of ideas conceived in the laboratory to the point where they can be commercialized. Technology development has been undertaken by numerous stakeholders, including entrepreneurs, university research laboratories, national federal laboratories, and private companies in the environmental technology business with sufficient financial resources. All of these potential developers make investment decisions based on their understanding of the potential market for such technologies. This market, in turn, depends on the potential purchasers or buyers of these future technologies. Potential purchasers of innovative technologies include federal agencies such as the EPA, the Department of Defense (DOD), and the DOE; private industries designated as potentially responsible parties; and other government entities such as state or local governments and publicly owned utilities. Each of these prospective buyers influences the potential size of and the timing of the demand for innovative technologies.
During this first phase in the life cycle of an innovative technology, numerous barriers may inhibit the translation of creative ideas into a potentially viable technology. These barriers can be classified as technical, institutional, and economic.
Technical Barriers
As discussed frequently in this report, ground water cleanup is subject to significant uncertainties arising from the heterogeneous nature of the subsurface and the usual lack of information on the size and timing of contaminant releases. These uncertainties increase the risk of failure for both conventional and innovative technologies. Site characterization activities attempt to minimize these uncertainties, but because of the heterogeneous nature of the distribution of contaminants and the physical and chemical characteristics of sites, significant unknowns are likely to persist. Consequently, it is difficult for potential technology developers to define a "standard market" for their products. Furthermore, this lack of
a standard market makes it difficult to raise investment capital to commercialize a new technology.
Another significant barrier to the development of innovative technologies is the issue of scale-up. In most areas of process technology development, bench- or pilot-scale testing is a necessary precursor to implementation of the technology on a larger scale. However, innovative technologies for subsurface cleanup tested on the bench or small pilot scale often cannot be scaled up for field application due to the heterogeneous nature of the problem. Thus, at the early stages of technology development, full-scale demonstration of the technology is needed at each new site. As a technology becomes more widely used under a variety of conditions, such demonstrations may no longer be needed.
Institutional Barriers
Innovative technology development is further limited by institutional barriers that have a direct impact on the willingness of entrepreneurs and private investors to invest in new technologies. One significant institutional barrier is the lack of facilities for testing new technologies under controlled conditions. For example, the Safe Drinking Water Act bans the injection of hazardous wastes into most aquifers, unless the injection is part of a Resource Conservation and Recovery Act (RCRA) or Super-fund remedial action. In part because of this regulatory barrier, at the time this report was prepared injection of contaminants into the subsurface for the purpose of testing new technologies was permitted at only one site, the Moffett Air Force Base in California. As a result, many U.S. researchers rely on results from Canada, where researchers at the Waterloo Centre for Ground Water Research have been permitted to inject NAPLs and other contaminants under controlled conditions to test alternative technologies.
A second institutional barrier is the slow development of new technologies being researched in government laboratories, due either to changing budget priorities or to a lack of efficient technology transfer from the national laboratories to commercialization. A third institutional barrier is the uncertainty about whether the public will accept innovative technologies at sites where public comment is either encouraged or statutorily required. Although public groups may be strongly in favor of some innovative technologies that have the potential to reduce the toxicity and mobility of contaminants or to provide permanent solutions, acceptance by public groups is another uncertainty that can inhibit investor interest in innovative technologies.
Economic Barriers
The primary economic barrier faced by developers of new technologies is difficulties in raising sufficient capital for successful commercialization. Although the demand for new technologies appears large based on the magnitude of the contamination problem (EPA, 1993), predicting the timing of that demand has proven elusive. For example, projections by both the federal government and private organizations on the rate of expenditure for waste site remediation have been significantly inaccurate. Many companies formed in response to such projections only to find that the actual timing of the demand was delayed substantially, from two to five years. Some of these companies have had an early demise in the marketplace. There has been a general consolidation of the remediation industry, with the number of firms and investor interest declining. (Environmental stocks were one of the worst-performing sectors in 1993.) These economic uncertainties limit the ability of private parties to obtain sufficient venture capital to undertake development of remediation technologies, particularly in situ technologies. (For ex situ technologies, the degree of uncertainty in success is considerably lower, and therefore obtaining venture capital is easier.) Generally speaking, the amount of federal or state funds available for investment in new technologies is also limited, due to the inherent suspicion on the part of many government agencies as to the future outcome of such investments and debates over who benefits and who should benefit.
An additional economic barrier is the inability to define accurately the potential rates of return on investment in new technologies. Under this high degree of uncertainty, the enthusiasm of entrepreneurs and other entities for investing in environmental technologies is limited.
Technology Selection Phase
The second key phase in the life cycle of an innovative technology is selection of the appropriate technological combinations required for site cleanup. The process of selecting technologies for hazardous waste site remediation is well defined under both RCRA and the Superfund act and has been presented in detail in various EPA guidance documents. Either a feasibility study or corrective measure study, under the Superfund act or RCRA, respectively, provides the background for screening and selecting innovative technologies. If an innovative technology is found to be suitable for use at a particular site, it can then be identified in the Superfund Record of Decision or in the RCRA Corrective Measure Implementation Plan. The final step in the selection of technologies is public review and acceptance or rejection.
The selection process involves numerous stakeholders, including environmental consultants, engineering and construction contractors, the regulated community (primarily the potentially responsible parties), and the public, which by statute and regulations is asked to comment on the selection of technologies at numerous milestones during the project. As was the case in the technology development phase, significant technical, institutional, and economic barriers to innovative technologies exist in the technology selection phase.
Technical Barriers
The major technical barriers to the selection of innovative technologies are uncertainties due to site complexity and the inadequacy of site characterization techniques for minimizing these uncertainties. Technological uncertainties due to site complexity pose difficult dilemmas for consultants and regulators, who may be either unfamiliar with the potential effectiveness of an innovative technology or unable to obtain information on the potential applicability of that technology. On the one hand, innovative technologies may promise cost and efficiency advantages for site cleanup compared to conventional technologies. On the other hand, the cost and efficiency data taken at one site, or under very controlled conditions, may not be easily extrapolated to another site. Generally, the lack of information on the efficiency, cost, and suitability of an innovative technology under various site conditions, combined with significant technical uncertainty regarding site characterization, often causes consultants, owners and regulators to reject an innovative technology. This unwillingness to take risks characterizes the technology selection process at hazardous waste sites in the United States.
Another significant technical barrier to innovative technology selection is the setting of cleanup levels that are unlikely to be achieved by any technology, including an innovative one. This barrier is especially important for very complex sites such as those in category 4 in Table 3-2. There is little incentive to select an innovative technology if cleanup levels cannot be achieved in technically practical time frames.
Institutional Barriers
Technical uncertainty, in turn, raises institutional barriers to innovative technology selection. One institutional barrier is lack of adequate technical expertise on the part of consultants and regulators, leading to conservative decisionmaking. A second barrier is the potential for liability if the technology fails. There is a pervasive unwillingness to share risks of selecting innovative technologies between the regulator, the con-
sultant, and the technology vendor or construction contractor. The lack or shortage of insurance coverage for the selection of innovative technologies amplifies the liability problems; the consultant, or the engineering contractor, must bear the brunt of the risk, limiting the number of firms willing to take such financial risks to major companies that are financially stable and able to self insure. Also contributing to the liability problem is the unwillingness of many federal agencies or potentially responsible parties to indemnify consultants and engineering contractors when innovative technologies are recommended or selected.
A key difference in the selection of technologies for Superfund sites compared to selection of technologies at non-Superfund sites is the need for public approval of the remedy. In some cases, public groups will strongly support innovative technologies. Because public groups tend to be risk averse, however, there is the potential for rejection of some innovative technologies, such as those involving use of thermal treatment methods or genetically engineered microorganisms. In balance, however, the posture of public groups may in fact be a positive support for the development and selection of innovative technologies. The impact of public involvement in remedy selection—whether positive or negative— has not been evaluated.
Economic Barriers
The major economic barrier to innovative technology selection is cost uncertainty. The lack of cost history available for new technologies exacerbates the level of uncertainty facing the engineer or the regulator and makes private parties responsible for cleanup hesitant to select new technologies. Contributing to cost uncertainty is the likely increase in costs due to project delays, a result of institutional barriers that must be overcome by testing the technology. Another component of the cost problem is unwillingness to fund the treatability studies and other developmental tests required to demonstrate that an innovative technology may be successful at a particular site. The selection of innovative technologies may also be inhibited by fears of patent infringement and unknown licensing fees for innovative technologies that are still in the development stage. All of these factors represent barriers to the selection of innovative technologies, particularly under conditions in which there is an urgency to arrive at a remedy selection. Many of these factors, however, are similar to barriers facing the implementation of any new technology or scientific advance in society. The two unique factors associated with ground water remediation are the continuing uncertainty in the success of any technology because of uncertainty in site characterization and the litigious nature of many site remediation projects.
Technology Implementation/Procurement Phase
The final phase of the life cycle of an innovative technology is the procurement, construction, and operation of the technology. The transition from the Record of Decision, remedial action plan, or corrective measure implementation plan to the final implementation of a remedial action is complex, with the details dictated by the ultimate owner of the technology. Various procurement options are used, including several different contractual mechanisms, depending on whether the remediation is being overseen by a government agency or a private party. The most common are the fixed-price or lump-sum contract, the unit pricing contract (often preferred by private parties), and the time and materials contract. The latter has been used less frequently than the fixed-price and unit-price contracts. At this stage in the life cycle of an innovative technology, as in the two prior stages, technical, institutional, and economic barriers exist.
Technical Barriers
During the procurement stage, site complexities and the heterogeneities decrease the willingness of contractors to guarantee the effectiveness of their technology. This is particularly true for innovative technologies for which only limited data are available. The general unwillingness of contractors to guarantee the effectiveness of an innovative technology may cause site owners to reject that technology. The issue at hand is who should take the risk of failure: the responsible party/owner or the owner/developer of the technology. Another technical barrier to procurement is the likely need for a combination of technologies to achieve desired cleanup levels. If more than one innovative technology is included, the project becomes even more complex and more difficult to implement.
Institutional Barriers
Many of the institutional barriers complicating the selection process for innovative technologies are also relevant to the procurement stage. As noted above, technical uncertainties result in a major institutional barrier: the unresolved debate over who should bear the brunt of the risk for the possible failure of the technology. For example, the EPA recently altered its position on indemnification of remedial action contractors operating at Superfund sites. The previous policy was to provide unlimited indemnification. The new policy, approved during the final days of the Bush administration, provides indemnification only in the event that
there are insufficient bids during the procurement process and that the insufficiency is due to the lack of government indemnification. The indemnification limits have been set at $75 million for EPA contractors and $50 million for all other remedial action contractors (HMIR, 1993). This new policy may reduce the number of sureties willing to provide bonding coverage for remedial action contractors and cause further delays in the overall Superfund remedial action program. Presumably, similar problems will occur under the RCRA corrective action program.
Other institutional barriers involve the nature of the federal procurement process. For example, a contractor who undertakes treatability studies cannot compete for construction of the innovative technology at full scale because the Federal Acquisition Regulations specify that any contractor involved in the selection or testing of a technology is not eligible for construction. Consequently, the developer of that technology will be reluctant to support such essential studies. Another barrier is excessive bonding requirements, which may reduce the number of contractors willing to compete.
Economic Barriers
The principal economic barrier to procurement of innovative technologies, like selection of innovative technologies, is uncertainty over costs of both the innovative technology and total site remediation. For organizations like the Army Corps of Engineers that prefer lump-sum or fixed-price contracts, a major barrier arises. Such contracting mechanisms are not suitable for sites where a high degree of uncertainty exists, either with respect to the extent and nature of the contamination or with respect to the applicability of the technology to the particular site. An alternative to the lump-sum contract is the indefinite delivery contract, in which each task of a project is negotiated in sequence rather than bidding the entire project at once. However, some federal procurement agencies perceive that such contracts may lead to higher total costs (including construction costs) for the remediation project. Such potential cost increases would be justifiable if an innovative technology could provide substantial efficiency and life cycle cost savings. However, such cost savings are often difficult to predict with a level of accuracy that overcomes the reluctance of agencies to take the risk on larger short-term costs.
Adequacy of Action to Address Barriers
Despite these barriers, use of innovative technologies to clean up waste sites has increased substantially. For example, in 1984, only one innovative technology was selected for use at Superfund sites. In 1992,
59 were specified (Kelly, 1994). However, nearly half of these involve soil vapor extraction, which is suitable primarily for soils, not ground water. Significant efforts to expand the use of innovative technologies are under way through government programs and laboratories, cooperative research agreements between government agencies and private organizations, and various educational initiatives.
Overcoming Technical Barriers
The federal government is making significant investments in development of new waste site characterization and cleanup technologies through the EPA, DOE, and DOD. The major EPA program for promoting development and demonstration of new technologies is the SITE program, which evaluates and compiles data on new technologies. The EPA also sponsors five hazardous substance research centers, authorized under 1986 amendments to the Superfund law, and four university research centers whose research missions address issues related to hazardous waste site remediation. In addition to these EPA programs, the DOE and DOD are investing significant amounts in development of new technologies that could be used to clean up defense and weapons manufacturing facilities. For example, in its 1995 budget request the DOE allocated $426 million—about 7 percent of its $6 billion environmental management budget—for technology development, some of which will be used to develop new ground water and soil cleanup technologies (Department of Energy, 1994). The DOD's 1995 budget request calls for $30 million for technology demonstrations for the DOD environmental cleanup program and $15 million for an environmental security technology certification program, which will assist in transferring promising technologies tested in the laboratory to the field (Department of Defense, 1994).
Other initiatives are under way to identify federal facilities that government agencies and private industries can use as test sites for innovative technology evaluation. The mechanism for implementing such agreements is the Cooperative Research and Development Agreement (CRADA), which specifies the terms and conditions to be established between federal agencies and other entities to undertake research at federal facilities. A recent CRADA signed by the EPA, the Air Force, Clean Sites, Inc., and several private companies involves the use of McClellan Air Force Base in California as an innovative technology test site (Clean Sites, Inc., 1992). Another major initiative currently in progress involves a CRADA between the Western Governors Association and numerous federal agencies, including the EPA and the Departments of Defense, the Interior, and Energy. Under the memorandum of understanding between these agencies, the Western Governors Association has created task forces
to guide development, testing, and implementation of innovative technologies for cleaning up federal facilities located in the western United States.
A few states have also undertaken programs to assist in the development and implementation of innovative technologies. The state of California, for example, has an Office of Appropriate Technologies, which has used cost-sharing approaches to encourage innovative technology development.
Overcoming Institutional Barriers
Several initiatives are under way to reduce institutional barriers to innovative technologies. The EPA, through the Technology Innovation Office (TIO), has made major strides in developing data bases to provide information on the efficacy and cost of both conventional and innovative cleanup methods (EPA, 1992a,b,c,d). The TIO has developed a vendor data base (the Vendor Information System for Innovative Treatment Technologies, or VISITT) that provides relevant information on vendors of remediation technologies and is updated annually. Several of the DOE's national laboratories have instituted an extensive program of technology transfer to accelerate the translation of research concepts into new technologies. This effort is planned as a major focus of the national laboratory network as it makes a transition from weapons production to civilian missions. Significant support is now anticipated from the regulatory community for the testing of innovative technologies, particularly in the context of the CRADAs being initiated across the country.
Deficiencies in the training of personnel who have the responsibility to select and implement technologies for site cleanups have led to the proliferation of training programs addressing a wide range of topics. These workshops, short courses, conferences, and satellite video conferences are sponsored by the private sector, federal and state agencies, universities, and professional societies. Additionally, the TIO, in conjunction with a number of professional organizations, has launched the Waste Tech Initiative, which will culminate in the publication of eight monographs on innovative technologies appropriate for cleanup of contaminated soil and ground water.
Overcoming Economic Barriers
A variety of federal efforts are under way to reduce economic barriers to use of innovative technologies. For example, the EPA and other federal agencies have undertaken major efforts to develop a contracting approach that is more suitable for engineering under the level of uncer-
tainty characteristic of waste site remediation (EPA, 1992b,c,d). (One example is the EPA's use of level-of-effort contracts, which specify the approximate number of hours or people that may be required for the duration of the contract, with hours negotiated on a task-by-task basis.) The DOE and DOD, through the Small Business Administration's Small Business Technology Transfer Program, are funding small businesses to carry out research and pilot studies of innovative cleanup technologies (SBA, 1994). In addition, the EPA's Superfund Accelerated Cleanup Model, currently in a trial mode at one site in each EPA region, urges early evaluation of innovative technologies. Finally, the EPA has issued guidance documents on the use of innovative technologies and, through the efforts of the TIO, continues to attack the problem of the lack of cost and efficacy data for innovative technologies. The list of vendors prepared by the TIO suggests that competitive pressure does exist for some innovative technologies and that costs of innovative technologies will likely decrease in the future.
In summary, although considerable efforts have been and are being invested in the development and implementation of innovative technologies, there is insufficient information available to objectively assess the success of these efforts. Such a task would not be straightforward. It would be analogous to a private company trying to show that its investments in new technologies have provided significant return to the owners. In the private sector, the marketplace provides an answer to this question. In the public sector, the ''investments'' are being made by a number of federal and state agencies, and the "benefits" are difficult—if not impossible—to quantify. The committee recommends that the EPA, DOE, DOD, and other federal agencies supporting the development of innovative technologies either collectively or individually assess, on a regular basis, the effectiveness of the programs to promote new environmental technologies for hazardous waste site remediation. The efforts of government agencies should also be compared to investments in the private sector to determine what, if any, lessons can be learned regarding the success or failure of different policy initiatives.
RESEARCH NEEDS FOR ADVANCING THE DEVELOPMENT OF INNOVATIVE CLEANUP TECHNOLOGIES
This chapter has described a range of potential enhancements and alternatives to pump-and-treat systems. However, the majority of these technologies are in the development stage, with few quantitative data sets for predicting how well they might perform at a given site. For the majority of technologies discussed in this chapter, field testing is needed
to establish the reliability of the technology, to provide data for preparing design manuals, and to identify the inherent limitations for users. These field tests should evaluate performance for a range of chemical contaminants and contaminant mixtures (such as petroleum hydrocarbons, chlorinated solvents, polychlorinated biphenyls, and metals) and site conditions (such as shallow and deep aquifers and homogeneous and heterogeneous geology). They should be designed to allow a mass balance analysis comparing the amount of contamination present in the subsurface with the amount removed. Data from the field tests should undergo scientific peer review.
In addition to field tests, focused research is needed to answer more fundamental questions about the technologies. The committee believes that focused research could lead to especially promising advances for engineered in situ bioremediation, intrinsic bioremediation, soil vapor extraction, air sparging, containment methods, and inorganic contaminant treatment methods.
Engineered And Intrinsic In Situ Bioremediation
Engineered and intrinsic in situ bioremediation are promising cleanup methods because they treat contaminants in place instead of requiring extraction, can convert contaminants to innocuous products, and minimize or eliminate pumping requirements. However, the microbial processes underlying bioremediation and how to optimize these processes are still not fully understood. Especially important is research to address the following questions:
-
Under what chemical conditions are contaminants susceptible to biodegradation?
-
What genetic characteristics and biochemical mechanisms control microbial degradation of particular types of contaminants?
-
What are the ecological relationships between the various microbial communities involved in biodegrading contaminants?
-
How can subsurface microbial populations be selected and manipulated to carry out specific biotransformations?
In addition to advanced understanding of microbial processes, improvements are needed in the engineering systems used to promote bioremediation. The key engineering challenges are delivering adequate growth-stimulating materials to the microorganisms and ensuring adequate contact between the organisms and the contaminants. Research addressing the following questions would advance the design of bioremediation systems:
-
How can systems for delivering oxygen and other growth-stimulating materials be optimized to provide sufficient quantities of the necessary materials, especially in low-permeability and heterogeneous soils? How can these delivery systems be improved to ensure mixing throughout the contaminated zone and prevent excessive microbial growth near the injection point?
-
What is the efficiency of methods such as surfactant and solvent flushing in enhancing contact between the microorganisms and the contaminants?
-
What is the ultimate fate in the subsurface of surfactants and solvents designed to promote microbial contact with contaminants?
-
How can protocols for monitoring and evaluating the progress of bioremediation be improved?
Soil Vapor Extraction and Air Sparging
Soil vapor extraction and air sparging have the potential to rapidly remove large quantities of volatile organic contaminants from shallow zones, as documented in this chapter. However, accurately predicting mass removal rates and operating times for these systems is difficult because of limited process-level understanding. Research addressing the following questions would advance the state of the art for soil vapor extraction and air sparging:
-
What is the relationship between air velocity and attainment of local equilibrium?
-
What is the importance of diffusion-limited processes, such as movement of contaminants from low-permeability zones and dead-end pores, in the performance of vapor extraction and air sparging systems?
-
What are the implications of assuming laminar flow versus assuming turbulent flow in design calculations?
-
What is the response of multicomponent contaminant mixtures, such as gasoline, to removal by volatilization? How does the extraction efficiency decrease over time with loss of the more volatile fractions?
-
How can the optimal well spacing be determined?
-
What are the appropriate models for describing two-phase hydro-dynamic conditions generated by air flow in the saturated zone?
-
How does the elevated air supply influence chemical and biological reactions?
-
What is the most cost-effective balance between designing air delivery systems to promote contaminant volatilization and designing them to promote contaminant biodegradation?
Containment Technologies
Construction difficulties and questions about long-term reliability have limited the application of containment technologies in the past. Research addressing the following questions would improve the ability to design effective containment systems:
-
How can methods for detecting defects in containment systems be improved?
-
How can the bottoms of vertical walls be effectively sealed?
-
What is the long-term reliability of different materials used for containment?
-
How significant is diffusive transport of contaminants across barriers over long time scales?
Treatment Methods For Inorganic Compounds
Most of the enhancements and alternative technologies reviewed in this chapter are for treating organic contaminants. However, as described in Chapter 1, metal contaminants such as lead and chromium are present at hundreds of Superfund sites as well as at the many other types of waste sites. One example of a metal removal technique currently being researched is electro-osmotic purging (Acar, 1992), in which electrodes are inserted in the soil to enhance the diffusion of metals and facilitate their extraction from low-permeability soils. Other suggested methods for treating metals involve using chemicals or microbes to either dissolve the metals and improve their recovery or immobilize the metals for long-term containment. In general, however, the techniques for removing metals are much less developed than those for removing organic compounds. Therefore, research is needed to develop existing metal recovery methods and to explore possible new techniques.
EDUCATIONAL NEEDS
As discussed in this chapter, an important barrier to the use of innovative technologies is lack of technical expertise on the many possible innovative cleanup methods. Advancing use of these technologies will require improved education, especially of the people in direct decisionmaking positions. The committee recommends three types of educational programs:
-
Formal interdisciplinary programs: The nation's formal academic educational programs need to be updated and the interdisciplinary op-
-
portunities within these programs expanded to train future generations of technical personnel.
-
Technical training courses: Training courses are needed to improve the knowledge of existing technical personnel.
-
Opportunities for representatives of industry, researchers, regulators, consultants, and contractors to exchange ideas and experiences: Opportunities are needed to discuss successes and failures, barriers to using more efficient treatment methods, and steps that could be taken to increase the diversity of available technologies.
CONCLUSIONS
Based on technical reports about innovative subsurface cleanup technologies and an assessment of the application of these technologies at contaminated sites, the committee reached the following conclusions:
-
Enhancements to conventional pump-and-treat systems can significantly increase the mass of contaminants removed from the subsurface and the rate at which they are removed. Pulsed pumping can improve cleanup cost efficiency by allowing contaminants to desorb and diffuse into mobile fluid zones, increasing the mass of contaminant extracted with each volume of pumped water. In situ bioremediation and in situ chemical treatment can improve cleanup efficiency by promoting contaminant destruction in place, eliminating or minimizing the need to extract the contaminants. Soil vapor extraction, air sparging, and horizontal wells can improve cleanup efficiency by removing volatile contaminants via air, a more effective transport medium than water. Soil flushing and in situ thermal technologies can improve cleanup efficiency by enhancing contaminant removal from soil above the water table, preventing contaminant migration into the ground water.
-
Although enhancements can substantially increase the efficiency of pump-and-treat systems, they are subject to similar limitations as conventional systems because they involve pumping fluids such as water, water solutions, and/or air. Geologic complexities can interfere with delivery of the fluids to zones where contaminants may be lodged. Contaminant sorption to solid materials and the presence of NAPLs can limit the availability of contaminants for capture or treatment by the circulating fluids. Sorption and NAPLs can also limit contaminant contact with microorganisms, which is required for in situ bioremediation.
-
In situ nonpumping alternatives to pump-and-treat systems may reduce cleanup costs, but they have important limitations. Nonpumping alternatives reduce costs by eliminating the need to circulate fluids through the subsurface, which can have a high energy cost. Nonpump-
-
ing approaches can contain the contamination, route the dissolved contamination through a stationary reactive medium, or use the capability of indigenous microbes to degrade the contamination without human intervention. However, the long-term integrity of containment systems is unproven, stationary barriers for treating contamination have been tested only in small-scale laboratory experiments, and very little operating history exists to judge the effectiveness of intrinsic biodegradation. In addition, members of the public may be unwilling to accept these approaches, which they may perceive as equivalent to no action or as resulting in incomplete site remediation.
-
No known technology can overcome all of the limitations to ground water cleanup. For innovative cleanup technologies as for conventional pump-and-treat systems, the geologic conditions at the site and the chemical nature of the contaminants can prevent restoration of ground water to health-based standards. Nevertheless, the use of innovative technologies should be encouraged even if the technologies cannot currently reach health-based cleanup goals, because new technologies may outperform conventional systems and because wider use of the technologies may lead to discoveries that improve their performance.
-
For enhanced pump-and-treat systems and nonpumping alternatives, thorough site characterization, pilot testing, and monitoring are critical to effective performance. An observational approach to remediation, in which the treatment system is adjusted as new information becomes available, is even more important for innovative technologies than for conventional pump-and-treat systems because of the greater uncertainties associated with the innovations.
-
A combination or sequence of remedial technologies is likely to be more effective—and necessary—at most sites. The majority of waste sites contain mixtures of contaminants with varying physical and chemical properties. A single remedial process is typically effective at removing only a subset of the compounds in a waste mixture. Treatment trains that couple remedial techniques may be necessary to treat the different types of contaminants that may be present at the site.
-
Despite the potential for innovations to increase the efficiency and reduce the costs of ground water cleanup, significant barriers have obstructed the development and application of these innovations . A combination of technical, institutional, and economic barriers discourages those involved in cleanups from assuming the risks associated with using technologies that lack proven track records. Options need to be explored for developing mechanisms that allow risk sharing when innovative technologies are used, so that neither the site owner nor the technology developer must assume the full burden of risk if the technology fails.
-
Federal agencies supporting innovative technology development should assess the effectiveness of current technology development efforts. Cooperative research and development agreements among government agencies, the DOE's research at its national laboratories, and the EPA's efforts under the SITE program, through the TIO, and through the hazardous substance research centers are important steps in expanding use and development of innovative technologies. However, whether these programs are sufficient to overcome the many barriers to technology development is unknown. Given the size and cost of the ground water cleanup problem, the importance of ensuring that adequate resources are directed toward technology development cannot be overstated.
NOTE
1. |
Technologies that circulate air specifically to promote biodegradation are known as bioventing systems and are described later in this chapter. |
REFERENCES
Abdul, A. S., and T. L. Gibson. 1991. Laboratory studies of surfactant enhanced washing of polychlorinated biphenyl from sandy material. Environ. Sci. Technol. 25(4):665-671.
Abdul, A. S., T. L. Gibson, and D. N. Rai. 1990. Selection of surfactants for the removal of petroleum products from shallow sandy aquifers. Ground Water 28(6):920-926.
Acar, Y. D. 1992. Electrokinetic cleanups. Civil Eng. 62(10):58-60.
American Academy of Environmental Engineers. 1994. Vacuum Vapor Extraction. Annapolis, Md.: American Academy of Environmental Engineers.
Angell, K. G. 1992. In situ remedial methods: air sparging. Natl. Environ. J. 2(1):20-23.
Baehr, A. L., G. E. Hoag, and M. C. Marley. 1989. Removing volatile contaminants from the unsaturated zone by inducing advective air-phase transport. J. Contam. Hydrol. 4(1):2-6.
Borden, R. C., C. Gomez, and M. Becker. In press. Natural bioremediation of a gasoline spill. In Proceedings of the Second International Symposium on In Situ and On-Site Bioremediation, San Diego, April 5-8, 1994. Chelsea, Mich.: Lewis Publishers.
Bosma, T. N. P., J. L. Schnoor, G. Schram, and A. J. B. Zehnder. 1988. Simulation model for biotransformation of xenobiotics and chemotaxis in soil columns. J. Contam. Hydrol. 2:225-236.
Bouwer, E. J., and P. L. McCarty. 1983. Transformations of halogenated organic compounds under denitrification conditions. Appl. Environ. Microbiol. 45(4):1295-1299.
Bouwer, E. J., and J. P. Wright. 1988. Transformations of trace halogenated aliphatics in anoxic biofilm columns. J. Contain. Hydrol. 2:155-160.
Brown, R. 1992. Air Sparging: A Primer for Application and Design. Trenton, N.J.: Groundwater Technology, Inc.
Buscheck, T., and R. Peargin. 1991. Summary of a nationwide vapor extraction system performance study. In Proceedings of Petroleum Hydrocarbons and Organic Chemicals in Ground Water. Dublin, Ohio: National Ground Water Association.
Chiang, C. Y., I. P. Salanitro, E. Y. Chai, J. D. Colthart, and C. L. Klein. 1989. Aerobic biodegradation of benzene, toluene, and xylene in a sandy aquifer: data analysis and computer modeling. Ground Water 27(6):823-834.
Cho, S. H., and A. R. Bowers. 1991. Treatment of toxic or refractory aromatic compounds by chemical oxidants. Pp. 273-292 in On-Site Bioreclamation Processes for Xenobiotic and Hydrocarbon Treatment, R. E. Hinchee and R. F. Olfenbuttel, eds. Boston: Butterworth-Heinemann.
Clean Sites, Inc. 1992. Proposal for Joint Industry-Government Evaluation of Innovative Technologies and Treatment Trains at Federal Facility Sites. Alexandria, Va.: Clean Sites, Inc.
De Bruin, W. P., M. J. J. Kotterman, M. A. Posthumus, G. Schraa, and A. J. B. Zehnder. 1992. Complete biological reductive transformation of tetrachloroethene to ethane. Appl. Environ. Microbiol. 58(6):1996-2000.
Dev, H., J. Bridges, and G. Sresty. 1984. Decontamination of hazardous waste substances from spills and uncontrolled waste sites by radio frequency in-situ heating. In Proceedings from Hazardous Materials Spills Conference, April 1984. Rockville, Md.: Government Institutes, Inc.
Dev, H., P. Condorelli, J. Bridges, C. Rogers, and D. Downey. 1987. In-Situ Radio Frequency Heating Process for Decontamination of Soil. Symposium Series 338. Washington, D.C.: American Chemical Society.
Dev, H., G. Sresty, J. Bridges, and D. Downey. 1988. Field test of the RF in-situ soil decontamination process. Contain. Soil Treatment (November):498.
Dev, H., G. Sresty, J. Bridges, J. Enk, N. Mshaiel, and M. Love. 1989. Radio Frequency Enhanced Decontamination of Soils Contaminated with Halogenated Hydrocarbons. EPA/600/S-2-89/008. Washington, D.C.: EPA.
DiStefano, T. D., J. M. Gossett, and S. H. Zinder. 1991. Reductive dechlorination of high concentrations of tetrachloroethene to ethene by an anaerobic enrichment culture in the absence of methanogenesis. Appl. Environ. Microbiol. 57(8):2287-2292.
DOD (Department of Defense). 1994. Office of the Secretary of Defense Operation and Maintenance Overview: Fiscal Year 1995 Budget Estimates. Washington, D.C.: DOD.
DOE (Department of Energy). 1994. Environmental Management 1994: Progress and Plans of the Environmental Restoration and Waste Management Program. Washington, D.C.: Center for Environmental Management.
Edwards, D. A., R. G. Luthy, and Z. Liu. 1991. Solubilization of polycyclic aromatic hydrocarbons in micellar nonionic surfactant solutions. Environ. Sci. Technol., 25(1):127-133.
Ellis, W. D., J. R. Payne and G. D. McNabb. 1985. Treatment of Contaminated Soils with Aqueous Surfactants. EPA/600/2-85/129. Cincinnati, Ohio: EPA, Risk Reduction Engineering Laboratory.
Environmental Protection Agency (EPA). 1991. Toxic Treatments: In Situ Steam Hot-Air Stripping Technology—Application Analysis Report. EPA 540/A5-90/008. Washington, D.C.: EPA.
EPA. 1992a. In Situ Treatment of Contaminated Ground Water: An Inventory of Research and Field Demonstrations and Strategies for Improving Ground-Water Remediation Technologies . Washington, D.C.: EPA, Technology Innovation Office.
EPA. 1992b. One Year Later: What Has Been Done to Reduce Impediments to the Use of Innovative Technology? Washington, D.C.: EPA, Technology Innovation Office.
EPA. 1992c. Procuring Innovative Treatment Technologies at Remedial Sites: Regional Experiences and Process Improvements. EPA 542/R-92/002. Washington, D.C.: EPA, Office of Solid Waste and Emergency Response.
EPA. 1992d. Strategy to Increase the Use of Innovative In Situ Treatment of Technologies for Contaminated Ground Water. Washington, D.C.: EPA, Technology Innovation Office.
EPA. 1993. Cleaning Up the Nation's Waste Sites: Markets and Technology Trends. EPA
542-R-92-012. Washington, D.C.: EPA, Office of Solid Waste and Emergency Response.
Farquhar, G. J., M. J. Schnarr and T. Gonullu. 1992. Enhanced chemical attenuation: destruction of the oily phase, in situ chemical oxidation. Pp. 63-65 in Proceedings from Subsurface Restoration Conference, Dallas, Texas, June 21-24. Houston: Rice University, Department of Environmental Science and Engineering.
Francis, A. J., and C. J. Dodge. 1988. Anaerobic microbial dissolution of transition and heavy metal oxides. Appl. Environ. Microbiol. 54(4):1009-1014.
Fu, J. K., and R. G. Luthy. 1986a. Aromatic compound solubility in solvent/water mixtures. ASCE J. Environ. Eng. 112(2):328-345.
Fu, J. K., and R. G. Luthy. 1986b. Effect of organic solvent on sorption of aromatic solutes onto soils. ASCE J. Environ. Eng. 112(2): 346-366.
Ghassemi, M. 1988. Innovative in-situ treatment technologies for cleanup of contaminated sites. J. Hazardous Mater. 17:189.
Gilham, R. W., and D. R. Burris. 1992. In situ treatment walls—chemical dehalogenation, denitrification, and bioaugmentation. Pp. 66-68 in Proceedings from Subsurface Restoration Conference, Dallas, Texas, June 21-24. Houston: Rice University, Department of Environmental Science and Engineering.
Gilham, R. W., and S. F. O'Hannesin. 1991. Metal catalyzed abiotic degradation of halogenated organic compounds. Ground Water 29(5):752.
Godsy, E. M., D. F. Goerlitz, and G. G. Ehrlich. 1983. Methanogenesis of phenolic compounds by a bacterial consortium from a contaminated aquifer in St. Louis Park, Minnesota. Bull. Environ. Contam. Toxicol. 30:261-268.
Goerlitz, D. F., D. E. Troutman, E. M. Godsy, and B. J. Franks. 1985. Migration of wood preserving chemicals in contaminated ground water in a sand aquifer at Pensacola, Florida. Environ. Sci. Technol. 19:955-961.
Gvirtzman, H., and S. M. Gorelick. 1992. The concept of in-situ vapor stripping for removing VOCs from groundwater. Transport in Porous Media 8:71-92.
Haber, C., L. Allen, S. Zhao, and R. Hanson. 1983. Methylotrophic bacteria: biochemical diversity and genetics. Science 221:1147-1153.
Herrling, B., W. Buermann, and J. Stamm. 1990. In-situ remediation of volatile contaminants in groundwater by a new system of "under-pressure-vaporizer wells." In Proceedings of the Conference on Subsurface Contamination by Immiscible Fluids, K. U. Weyer, ed. Rotterdam: A. A. Balkema.
Herrling, B., J. Stamm, E. J. Alesi, P. Brinnel, F. Hirschberger, and M. R. Sick. 1991. In situ ground water remediation of strippable contaminants by vacuum vaporizer wells (UVB): operation of the well and report about cleaned industrial sites. Presented at the Third Forum on Innovative Hazardous Waste Treatment Technologies: Domestic and International, June 11-13,1991, Dallas.
Herrling, B., J. Stamm, and W. Buermann. 1991. Hydraulic circulation system for in situ bioreclamation and/or in situ remediation of strippable contamination. In Proceedings of In-Situ and On-Site Bioreclamation International Symposium, March 19-21, 1991, San Diego. Grand Haven, Mich.: Type-Right.
Hoigné, J. 1988. The Chemistry of Ozone in Water—Process Technologies for Water Treatment. New York: Plenum Publishing Corporation.
HMIR (Hazardous Material Intelligence Report). 1993. Feb. 5. P. 4.
Hunt, J. R., N. Sitar, and K. S. Udell. 1988a. Nonaqueous phase liquid transport and cleanup, 1: analysis of mechanisms. Water Resources Res. 24(8):1247-1258.
Hunt, J. R., N. Sitar, and K. S. Udell. 1988b. Nonaqueous phase liquid transport and cleanup II: experimental studies. Water Resources Res. 24(8):1259-1269.
Hutchins, S. R., G. W. Sewell, D. A. Kovacs, and G. A. Smith. 1991. Biodegradation of
aromatic hydrocarbons by aquifer microorganisms under denitrifying conditions. Environ. Sci. Technol. 25(1):68-76.
Hutzler, N., B. Murphy, and J. Gierke. 1989. State of Technology Review: Soil Vapor Extraction Systems. EPA/600/2-89/024. Cincinnati: EPA Risk Reduction Engineering Laboratory.
Johnson, P., C. Stanley, M. Kemblowski, D. Byers, and J. Corthart. 1990. A practical approach to the design, operation, and monitoring of in situ soil-venting systems. Ground Water Monit. Rev. (Spring):159.
Johnson, R. L., W. Bagby, M. Perrott, and C. Chen. 1992. Experimental examination of integrated soil vapor extraction techniques. In Proceedings of Petroleum Hydrocarbons and Organic Chemicals in Ground Water: Prevention, Detection, and Restoration. Dublin, Ohio: National Ground Water Association.
Kelly, M. M. 1994. Applying innovative technologies to site contamination: historical trends and future demand. Presented at HazMat South '94, February 16, 1994, Orlando, Fla.
Kovalick, W. 1993. Testimony before the U.S. House of Representatives Committee on Science, Space, and Technology, Subcommittee on Investigations and Oversight, Washington, D.C., April 28-29, 1993.
Laha, S., and R. G. Luthy. 1991. Inhibition of phenanthrene mineralization by nonionic surfactants in soil-water systems. Environ. Sci. Technol. 25(11):1920-1930.
Lee, M. D., and R. L. Raymond, St. 1991. Case history of the application of hydrogen peroxide as an oxygen source for in situ bioreclamation. Pp. 429-436 in In Situ Bioreclamation: Applications and Investigations for Hydrocarbon and Contaminated Site Remediation, R. E. Hinchee and R. F. Olfenbuttel, eds. Boston: Butterworth-Heinemann.
Lee, M. D., and C. H. Ward. 1985. Biological methods for the restoration of contaminated aquifers. J. Environ. Toxicol. Chem. 4:743.
Loden, M. E., and C. Fan. 1992. Air sparging technology evaluation. In Proceedings of Research and Development, '92. National Research and Development Conference on the Control of Hazardous Materials: San Francisco, Calif., Feb. 4-6, 1992. Greenbelt, Md.: Hazardous Materials Control Resources Institute.
Looney, B. B., T. C. Hazen, D. S. Kaback, and C. A. Eddy. 1991. Full Scale Field Test of the In Situ Air Stripping Process at the Savannah River Integrated Demonstration Test Site. Report No. WSRC-RD-91-22. Aiken, S.C.: Department of Energy and Westinghouse Savannah River Company.
Lord, A. E., R. M. Koerner, V. P. Murphy, and J. E. Brugger. 1987. Vacuum-assisted in-situ steam stripping to remove pollutants from contaminated soil. In Proceedings of the 13th Annual Research Symposium, EPA Conference on Land Disposal, Remedial Action, Incineration and Treatment of Hazardous Waste, Cincinnati, Ohio, May 6, 1987. EPA/600/9-87/015. Cincinnati, Ohio: EPA, Center for Environmental Research Information.
Lovley, D., and E. J. P. Phillips. 1992. Reduction of uranium by Desulfovibrio desulfuricans. Appl. Environ. Microbiol. 58(3):850-856.
Lovley, D., E. J. P. Phillips, Y. A. Gorby, and E. R. Landa. 1991. Microbial reduction of uranium. Nature 350:413-416.
Major, D. W., E. W. Hodgins, and B. J. Butler. 1991. Field and laboratory evidence of in situ biotransformation of tetrachloroethene to ethene and ethane at a chemical transfer facility in north Toronto. Pp. 147-171 in On-Site Bioreclamation Processes for Xenobiotic and Hydrocarbon Treatment, R. E. Hinchee and R. F. Olfenbuttel, eds. Boston: Butterworth-Heinemann.
Marley, M. C. 1992. Removing gasoline from soil and groundwater through air sparging. Remediation 2(2):121-131.
McCarty, P. L., L. Semprini, M. E. Dolan, T. C. Harmon, C. Tiedeman, and S. M. Gorelick. 1991. In situ methanotrophic bioremediation for contaminated ground water at St. Joseph, Michigan. Pp. 16-40 in On-Site Bioreclamation Processes for Xenobiotic and Hydrocarbon Treatment, R. E. Hinchee and R. F. Olfenbuttel, eds. Boston: Butterworth-Heinemann.
Middleton, A., and D. Hiller. 1990. In situ aeration of ground water—a technical overview. In Proceedings of Prevention and Treatment of Soil and Ground Water Contamination in the Petroleum Refining and Distribution Industry, Montreal, October 16-17, 1990. Ottawa, Canada: Environment Canada.
National Research Council. 1993. In Situ Bioremediation: When Does It Work? Washington, D.C.:National Academy Press.
Roberts, P. V., J. Schreiner, and G. D. Hopkins. 1982. Field study of organic water quality changes during groundwater recharge in the Palo Alto Baylands. Water Resources 16(6):1025-1035.
Rosen, M. J. 1989. Surfactants and Interfacial Phenomena, 2nd ed. New York: John Wiley and Sons.
SBA (Small Business Administration). 1994. Small Business Technology Transfer Program: STTR Pre-Solicitation Announcement. Washington, D.C.: SBA, Office of Innovation Research and Technology.
Schroeder, J. D., N. D. Rosenberg, E. P. Barnes-Smith, and S. R. Booth. 1992. In Situ Air Stripping: Cost Effectiveness of a Remediation Technology, Field Tested at rite Savannah River Integrated Demonstration Site. Report No. LA-UR-92-1927. Los Alamos National Laboratory.
Semprini, L., P. V. Roberts, G. D. Hopkins, and P. L. McCarty. 1990. A field evaluation of in situ biodegradation of chlorinated ethanes: part 2—results of biostimulation and biotransformation experiments. Ground Water 28(5):715-727.
Semprini, L., G. D. Hopkins, P. V. Roberts, and P. L. McCarty. 1991. In situ biotransformation of carbon tetrachloride, Freon-113, Freon-11, and 1,1,1-TCA under anoxic conditions. Pp. 41-58 in On-Site Bioreclamation Processes for Xenobiotic and Hydrocarbon Treatment, R. E. Hinchee and R. F. Olfenbuttel, eds. Boston: Butterworth-Heinemann.
Smith, L., and R. Hinchee. 1993. In-Situ Thermal Technologies for Site Remediation. Ann Arbor, Mich.: Lewis Publishers.
Starr, R. C., and J. A. Cherry. 1992. Applications of low permeability cutoff walls for ground water pollution control. Presented at Forty-fifth Canadian Geotechnical Conference, Toronto, Canada, October 26-28, 1992.
Stewart, L. D., and K. S. Udell. 1988. Mechanisms of residual oil displacement by steam injection. SPE Reservoir Eng. 3:1233-1242.
Taylor, R. T., W. B. Durham, A. G. Duba, K. J. Jackson, R. B. Knapp, J. P. Knezovich, A. M. Wijesinghe, D. J. Bishop, M. L. Hanna, M. C. Jovanovich, and D. R. Shannard. 1992. In situ microbial filters. Pp. 66-67 in Proceedings of In Situ Bioremediation Symposium '92, Niagara-on-the-Lake, Ontario, Canada, September 20-24, 1992. Burlington, Ontario, Canada: Wastewater Technology Centre.
Udell, K. S., and L. D. Stewart. 1989. Field Study of In Situ Steam Injection and Vacuum Extraction for Recovery of Volatile Organic Solvents . UCB-SEEHRL Report No. 89-2. Berkeley: University of California, Environmental Health Research Laboratory.
Udell, K. S., and L. D. Stewart. 1990. Combined steam injection and vacuum extraction for aquifer cleanup. Paper presented at rite International Association of Hydrologists Conference, Calgary, Alberta, Canada, April 1990.
Vignon, B. W., and A. J. Rubin. 1989. Practical considerations in rite surfactant-aided
mobilization of contaminants in aquifers. J. Water Pollut. Control Fed. 61(7):1233-1240.
Vogel T. M., and P. L. McCarty. 1987. Abiotic and biotic transformations of 1,1,1-trichloroethane under methanogenic conditions. Environ. Sci. Technol. 21:1208-1213.
Wilson, B. H., G. B. Smith, and J. F. Rees. 1986. Biotransformation of selected alkylbenzenes and halogenated aliphatic hydrocarbons in methanogenic aquifer material: a microcosm study. Environ. Sci. Technol. 28(6):997-1002.
Wilson, D. J., and A. N. Clarke. 1992. Removal of semivolatiles from soils by steam stripping I: a local equilibrium model. Separation Science and Technology 27(11):1337.
Wilson, J. T., J. F. McNabb, J. W. Cochran, T. H. Wang, M. B. Tomson, and P. B. Bedient. 1985. Influence of microbial adaptation on the fate of organic pollutants in ground water. Environ. Toxicol. Chem. 4:721-726.
Wilson, J. T., and C. H. Ward. 1987. Opportunities for bioreclamation of aquifers contaminated with petroleum hydrocarbons. J. Ind. Microbiol. 27:109.
Wilson, J. T., and B. H. Wilson. 1985. Biotransformation of trichloroethylene in soil. Appl. Environ. Microbiol. 49(1):242-243.
Xu, Y., and F. W. Schwartz. 1992. Immobilization of lead in ground water with a reactive barrier system. Pp. 204-206 in Proceedings of Subsurface Restoration Conference, Dallas, Texas, June 21-24, 1992. Houston: Rice University, Department of Environmental Science and Engineering.