1
Introduction
In future years, gas-turbine engines of all types will have the potential to reach new heights of efficiency and service life. But to keep pace, engineers will have to design coatings concurrently with the intended substrate. Indeed, for some proposed designs, the coating and substrate form a continuum, literally blurring the boundary between the surface deposit and the material it coats. In selecting a coating for hot structures, the engineer will also have to keep in mind two primary criteria: the ability of the coating to protect the component from degradation and the ability of the coating to retain, over the long term, its protective properties in the harsh environment of the engine.
For decades, improvements in gas-turbine efficiency, whether for land-based power generation, marine applications, or aircraft propulsion, have been primarily driven by significant increases in gas temperatures within the engine. Of particular note have been the dramatic increases in the gas-path operating temperature within the combustor and high-pressure turbine sections of the engine. For example, turbine inlet operating temperatures have increased by hundreds of degrees, and the specific thrust has nearly doubled during the five decades of the existence of gas-turbine engines.
These large increases in gas temperature have been enabled by the development of highly engineered cooling schemes for the substrate and high-performance materials technologies. Advances in cooling technology involve sophisticated convection, impingement, and film cooling designs for turbine hardware. In many cases, these developments were facilitated by parallel advances in materials processing technologies, such as precision casting, machining, and drilling operations. Cooling has arguably accounted for more than 75 percent of the increased temperature capability and is still the ''first line of defense" in new turbine designs.
The introduction of new materials and processes has also heralded a series of advances in engine technology. For example, the transition from wrought to cast alloys provided a step improvement in high-temperature strength, a capability further enhanced by the introduction of vacuum investment casting. Within the range of cast superalloys, further incremental improvements occurred as directionally solidified and single-crystal alloys were developed to tolerate severe, cyclic thermal gradients. Future improvements will occur as intermetallic compounds, ceramics, composite materials, and refractory metal alloys are eventually incorporated in advanced engines, although significant technical barriers must still be overcome before these new families of materials are incorporated in critical engine components.
Because seemingly modest increases in upper temperature limits translate into huge benefits for engine performance, the large development costs associated with the introduction of new turbine blade alloys have historically been readily justified for even modest increases in temperature capability.1 The cycle time associated with the introduction of a new alloy has resulted in an average improvement in temperature capability of approximately 5°C (9°F) per year.
As highlighted in this report, advances in superalloy materials have gone hand-in-hand with continual improvements in the coatings used to protect the components from environmental degradation mechanisms, particularly high-temperature oxidation and hot corrosion. The coatings used to date have not increased the inherent temperature capability of the substrate material, but they have protected superalloys from increasingly aggressive service environments and appreciably extended the service lives of critical, costly components. This coating/substrate combination has provided such improved component capability that, along with advanced cooling technology, turbine gas-path operating temperatures in localized regions exceed the melting point of the engine's structural materials without causing catastrophic failure.
Recently, ceramic thermal barrier coatings (TBCs) deposited onto turbine alloys have been shown to have two major benefits. First, for the same gas-path operating temperatures, the cooling air requirements can be greatly reduced. Second, for the same cooling air flow, the hot gas temperature can be raised significantly. In practice, the designer can choose the operating conditions between these two alternative ranges. Turbines can be operated at higher temperatures while maintaining an acceptable temperature range within the substrate
material (i.e., TBCs are capable of providing at least 110°C [200°F] of increased capability). This latter benefit will allow TBCs to effectively fill the gap in materials capability for advanced engines between the current family of nickel-base superalloys (which are generally regarded as being at or close to their ultimate service temperature) and the advanced structural materials, such as intermetallics and ceramics.
As the gas-turbine industry becomes more dependent on coatings, the scatter (variation) in coating properties is becoming increasingly important. Figure 1-1 illustrates the scatter in performance of a given property of a coating, together with the property values and variation required to meet a set of advanced engine requirements. A wide bell-shaped distribution in a property such as coating adherence is tolerable—although less than optimal—when the coating is only to be used for extending the life of the base metal. This is particularly true if the failure mode is graceful, as is the case when a coating is protecting a superalloy that possesses inherent (but insufficient) environmental resistance.
If the coating must be relied on to last for a guaranteed time between overhauls, however, the performance of the system will be compromised if the coating cannot provide protection on a continued, reliable basis. If the coating system was further required to extend the temperature capability of the component system (as in the case of TBCs), scatter and unpredictability of the coating properties become critical. In either case, a coating with too great a variation in critical properties would not be implemented. For example, TBC life can be modeled as a broad statistical distribution, such as seen in figure 1-1. As a result, TBCs have generally not been used for critical applications such as for high-performance turbine airfoils. A current design criterion requires that a component not fail prior to the next scheduled inspection after local TBC spalling (Soechting, 1994). This limits the incremental thermal advantage of TBCs to approximately 40°C (70°F) over an uncoated part. This gain is nevertheless a significant benefit, approximating the gains typically achieved by a generation or two of alloy development. The full potential of TBCs can only be realized, however, if these coatings can be relied on to provide thermal protection of the substrate throughout the service life of the component. Thus, the coating must remain adherent and functional for the extended periods between coating refurbishment.
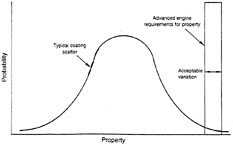
Figure 1-1 Typical ranges of a coating property compared with advanced engine requirements for a property.
The dependence on the reliability of coatings to improve performance and extend service life places an entirely new set of requirements on coating application processes and on coating system design. Coatings are becoming an integral part of the materials system needed to satisfy the application requirements and component design. Critical objectives of coating technologists, therefore, are to reduce the scatter in the property distribution curve (i.e., to enhance reliability) and to move the minimum predicted value into the regime required by the advanced engine requirements. This theme provides the basis for much of the discussion in this report and is a fundamental consideration of coating development and implementation on current and future high-temperature structural materials.