This page in the original is blank. |
Net Energy Expenditure: A Method for Assessing the Environmental Impact of Technologies
Martin B. Hocking
Although it is easy to compare the relative environmental merits of bicycling and driving a car, it is more difficult to use life-cycle inventory methods to compare the merits of paper towels, cloth towels, and a hot-air hand dryer, or of paper plates and china used in a cafeteria. In other words, it is often difficult to select a valid approach to compare the environmental advantages of very different technologies, even when they are used to accomplish similar functions.
Various factors such as usefulness, convenience, aesthetics, or the production of waste could be used to weigh the value of parallel technologies; however, a comparison of the net energy expenditure may be the most useful measure for judging environmental performance. This is the approach that is explored in this paper.
Even significant differences in the emission characteristics of competing technologies can be minimized by the expenditure of some additional energy on an emission-control function. For example, 2 percent or more of the energy produced by a thermal power station is consumed by its emission-control activities. Similarly, in sewage treatment plants, the processes used are primarily for emission control and are also, generally, net consumers of energy. Thus, emissions reduction is one way the environmental merits of competing technologies can be improved. Energy conservation also can influence the choice of competing "green" technologies over time.
What is significant about the choice of energy consumption as the primary factor for comparing technologies? Why not compare the consumption of renewable resources (e.g., biomass) with that of nonrenewable resources (e.g., those of fossil origin), or the consumption of a large-reserve resource base (e.g., sand, iron, or salt) with that of a small-reserve resource base (e.g., copper, silver, or
gold), or the use of low-polluting-potential materials (e.g., iron, glass, or cement) with that of high-polluting-potential materials (e.g., lead, mercury, or thallium)? Energy consumption is a valid comparative factor because all of these other distinctions are based ultimately on energy expenditure. Oil, gas, and coal are nonrenewable only in the sense that the energy cost of producing them synthetically from biomass or other carbon sources is 3 to 10 times their current prices. Copper, silver, and gold are more expensive than iron primarily because it takes much more energy to isolate and recover them. Also, these materials are not consumed during use, although some uses may dissipate them. An ounce of gold finely distributed throughout 90 tons of gravel is not worth $400, although at this concentration (about 0.3 mg/kg) it may be worth processing. Until the energy has been expended to recover it, however, it is only worth a very small fraction of $400.
An Outline of the Method
Boundaries
To compare technologies using the energy assessment method, appropriate boundaries for the technologies must be defined. During the course of the assessment, additional factors may emerge that require adjusting the boundaries to maintain fairness in the inventory.
Data assembled by Chapman et al. (1974) for automotive transportation and home heating illustrate the importance of appropriate and fair selection of boundaries for a valid comparison of different technologies. For example, the gasoline-powered car has an engine transmission combined efficiency of about 0.2 (Figure 1). The electric car, with a 0.8 battery charge—discharge efficiency and a motor control system—transmission efficiency also of about 0.8 gives a net system efficiency of 0.64, apparently much higher than the gasoline-fueled car.
If the energy-producing facilities for each of these technologies are included in the calculation, however, a very different picture emerges (Figure 1, solid boundaries). Oil production, refining, and delivery systems are estimated to be 0.88 efficient at retaining the energy originally present in the crude oil. Multiplying this by the fractional efficiency of the gasoline-powered car produces an overall efficiency for this system of 0.17. The relatively low efficiency of thermal electricity generation coupled with losses in fuel production and delivery, and electricity lost during transmission, gives an overall efficiency for delivered electricity of about 0.24. Combining this information with the higher efficiency of the electric automobile drive system gives an overall efficiency of 0.15, quite comparable to the overall efficiency of 0.17 calculated for the gasoline-powered system.
This example shows the considerations that enter into establishing boundaries for the conversion of energy into work and demonstrates the kinds of surprises that this exercise can reveal. An examination of appropriate boundaries for
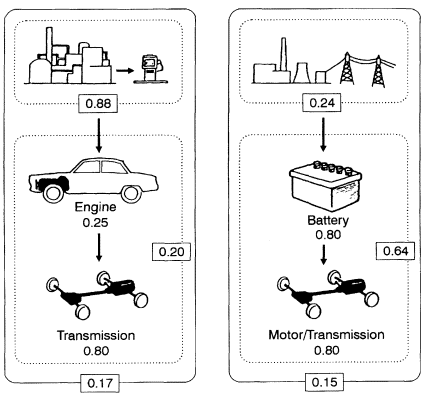
Figure 1
Significance of system boundaries on the perception of energy efficiency in automotive transport options (adapted from Chapman et al., 1974). Dotted-line boundaries encompass the fractional efficiencies restricted to automotive options, suggesting that the electric automobile, at 0.64 fractional efficiency, is more efficient than the gasoline-powered automobile. Solid-line boundaries include the efficiencies of the respective energy-delivery subsystems, demonstrating that, overall, the two systems have very similar efficiencies.
the conversion of various sources of energy into heat can also be instructive. Drawing a close boundary for a fossil-fueled boiler for home heating gives a fractional efficiency of about 0.75 (Figure 2). Repeating the process for electric heating gives a near-unity efficiency for the conversion of electrical energy to heat. With close boundaries, it is clear that electric heating is more efficient. If we extend the boundaries to include the respective energy delivery systems, however, the picture changes. The much higher efficiency of the fuel delivery system changes the fractional efficiency of the fueled boiler to 0.66 compared with 0.24 for the electrically heated system. Thus, burning a fuel in the place where the heat is required allows most of the thermal energy to be captured, whereas a large
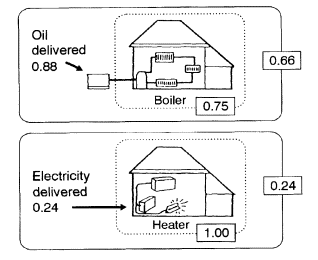
Figure 2
Significance of system boundaries on the perception of relative energy efficiencies of home heating options (adapted from Chapman et al., 1974). The relative efficiency within the dotted boundaries suggests that electricity, at 1.00 fractional efficiency, is more efficient than oil, at 0.75, for heating. However, assuming the larger solid boundaries, inclusive of the respective energy-delivery systems, results in a reversal of the perceived efficiencies: 0.24 for electricity and 0.66 for oil.
fraction of this heat is shed to the environment (i.e., wasted to air or water) during thermal electricity generation.
Information Sources
Data are available on the energy needed to produce many common materials. This information can be used as the starting point for an energy analysis. (See, for example, Berry et al., 1975; Boustead and Hancock, 1979; Gaines, 1981; Hocking, 1994a,b; Kindler and Nikles, 1979, 1980; Ringwald, 1982.) However, care must be taken to select the appropriate data. For the production of glassware, for example, published sources give energy requirements ranging from 9.1 to 79.1 J/g of glass, each correct for the particular boundaries and production conditions considered (Table 1). Researchers may present a range of data rather than a single number because of variations in the boundaries chosen (which may or may not be reported), the scale of production, or because more than one energy source is used. Thus, judicious selection of the most appropriate value is recommended, taking into account as many of the variables that reflect the particular situation as possible. Similarly, single energy-requirement values should be used
TABLE 1 Range of Energy Costs to Produce Glassware
kJ/g |
Remarks |
9.1 |
Glass melting only |
9.15 |
Cornelius furnace,a melting only |
9.18 |
Container glass, electricity, 3.06 GJ/mg, x 3b |
11.8 |
Container glass and containers |
12.6 |
Container glass, electricity x 3b |
10.5-17.8 |
Glass melting |
13.3 |
Container glassmaking, gas fired |
14.5 |
U.K. glass industry, overall, 1982 |
17.9 |
1 ton/day electric, from 1,500 kWh/ton,c x 3b |
18.0 |
Glass containers, 4,534 kWh/ton |
19.3 |
Glassware, melting only, 20 ton/day scale |
20.2 |
U.K. glass industry, overall, 1970 |
21.0-25.1 |
Total energy, from raw materials in the ground, generic glass |
25.0 |
Glass bottles, ca. 1980 data |
27.7 |
Glass tableware, includes raw material recovery, transport, plus 40 percent cullet |
46.5 |
Specialty glass, U.K., overall (includes tableware) |
79.1 |
Gas—air firing, melting only |
a Estimated primary fuel requirement from an electrical requirement of 1 kWh per 1.18 kg of glass. b Electrical energy required is multiplied by 3, assuming a 33 percent efficiency of thermal electricity generation (see text). SOURCE: Hocking (1994b). |
with care. Such values sometimes must be adjusted to better relate the conditions or boundaries of the cited data to the situation under analysis.
When no published data for the product of interest can be located, or when detailed verification is necessary for appropriate selection from a published range, then a detailed inventory of production inputs and outputs is necessary. Doing an inventory is similar to compiling the materials component of a life-cycle inventory (Vigon et al., 1992). The energy components that result from this process can be used to calculate the net energy requirement for that commodity. (See, for example, Hocking, 1991a,b, 1994a,b.) Boundaries must be clearly defined, and any energy conversion factors and equivalencies used in the analysis should be specified so that others may understand the process used and, if necessary, reproduce the results of the analysis.
If a significant proportion of the energy consumed to produce a commodity is from electricity, then the efficiency of conversion of the primary energy source used to generate the electricity has to be considered. Modern fossil-fueled thermal power stations are close to 33 percent efficient; nuclear power generation is
slightly more efficient. By comparison, hydroelectric power generation is about 70 percent efficient (Boustead and Hancock, 1979). Thus, with the extensive use of power grids for developed nations, it is possible to estimate a nation's electricity-generating efficiency based on the weighted average of the generating sources used. Grid-generating efficiencies calculated in this way are, for Canada, 57.3 percent; for Norway, 69.9 percent; for the United Kingdom, 35.2 percent; and for the United States, 38.0 percent (International Energy Agency, 1993; United Nations, 1990). Although electricity generation is probably subject to the widest range of production efficiencies, similar considerations apply to the production and delivery of other energy commodities, such as coal, oil, or natural gas (Boustead and Hancock, 1979; Hocking, 1994b).
An Example Using This Method
A recently completed energy analysis of reusable and disposable cups illustrates the method outlined and the kinds of information that can be obtained. Three reusable cup types—ceramic, glass, and reusable polystyrene—and two disposable cup types—uncoated paper and molded polystyrene foam—are considered.
For the plastic cup types, the boundaries for evaluation included the total energy required—from the extraction of crude oil to production of the final product; for the paper cup, they included the total energy required to produce a finished cup from a standing forest; and for the glass and ceramic cups, they included the energy required to process the raw materials and to produce the finished cups. On the output side, the energy consumed during each cup's life-cycle was evaluated up to the point of discard. For the reusable cups, the total operating energy of various commercial dishwashers was calculated. No account was made of the energy or materials required to make a commercial dishwasher, because this energy component per cup-use cycle over the life of the dishwasher will be small relative to the operating energy component.
The energy parameters of interest for each type of cup were compared using equations developed specifically for this purpose (Hocking, 1994b). The published energy requirements for each of the five cup technologies were then collected without regard to their relevance to cups or to whether the reported value was for part of the process or for the whole process (Hocking, 1994b). Detailed examination of these sources permitted selection of appropriate energy data that took into account the specified boundaries (Table 2). Operating details were also obtained for several commercial dishwashers with particular reference to their water, energy, detergent, sanitizer, and rinse agent requirements (Hocking, 1994b). The published values were then converted to common units, kilojoules per gram of material processed, which enabled comparisons within and among technologies.
TABLE 2 Selected Energy Requirements to Make Typical Hot-Drink Cups
|
|
|
Energy Requirement |
|
Cup Type |
Mass Range (g) |
Selected Cup (g) |
kJ/g (source) |
kJ/cup |
Ceramic |
227-337 |
292.3 |
48.2 (van Eijk et al., 1992) |
14,088 |
Heat-proof glass |
166-255 |
198.6 |
27.7 (Fenton, 1992) |
5,501 |
Reusable polystyrene |
27-109 |
59.1 |
106.6 (Fenton, 1992) |
6,300 |
Uncoated paper |
6.3-10.2 |
8.3 |
66.2 (Hocking, 1991b) |
549 |
Molded polystyrene foam |
1.4-2.4 |
1.9 |
104.3 (Hocking, 1991b) |
198 |
SOURCE: Hocking (1994b). |
Energy of Manufacture
The published energy values for each technology selected for Table 2 represent the best available for the purposes of this study. These values are consistent with the temporal trends in the energy requirements for these technologies established by Fenton (1992), and they are at the upper end of the tabulated range. None are extreme values, however. The highest energy requirement for the fabrication material is for the two types of polystyrene: 104.3 kJ/g for the foamed material and 106.6 kJ/g for the reusable polystyrene varieties. Paper (66.2 kJ/g) and ceramic (48.2 kJ/g) materials required much less energy. Glass required only about one-quarter the energy, or 27.7 kJ/g, needed to make polystyrene.
Arbitrary samples of each cup type (8-9-ounce nominal capacity) manufactured in Canada, China, the United States, and the United Kingdom were weighed, and the median weight of each type was used to calculate the energy of manufacture. On this basis, the very low mass of the molded polystyrene foam cup required the least total energy to produce, 198 kJ/cup, and the ceramic cup the most, at 14,088 kJ/cup.
Energy of Reuse
Most of the electrical energy required for dish washing goes to heat the water, which must be hot for effective cleaning. The electricity required per cycle for the more energy-efficient commercial dishwashers was similar, in the range of 70-83 kJ/cup (Hocking, 1994b). The energy requirements of some models were offset to an extent by reuse of the hot rinse water as the wash water for the next cycle; even so, two of the machines that use this strategy required about 130 kJ/cup per wash. However, electricity is a secondary energy source. To compare dish washing energy with the energy needed to manufacture each cup type, the calculation must include the additional expenditure of primary energy used to generate electricity, as already explained.
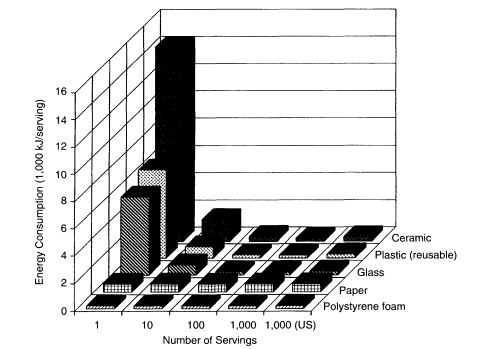
Figure 3
Change in energy consumption per serving for three types of reusable cups used only once before washing and two types of disposable cups used only once before discarding. Canadian electricity-generating efficiency was used for the 1-, 10-, 100-, and the first 1,000-serving column. American electricity-generating efficiency was used for the 1,000-serving column labeled US.
Figure 3 illustrates the energy consumption in kilojoules per serving for each of the reusable cup types used only once before washing, calculated using a 184 kJ/cup primary wash energy requirement, an intermediate value representing Canadian generating efficiency. For the disposable cup types used only once before discard, the energy consumption per use is the energy required to manufacture the cup. For a single use, all three types of reusable cups consume more than 10 times the energy per use than do either of the disposable cups. Energy consumption per use of the reusable cups drops to less than that of the paper cup only after about 100 servings. At some point between 100 and 1,000 servings, the energy consumption per use of the reusable cups finally falls to less than that of polystyrene foam disposables.
Another interesting feature emerges from this exercise if one uses the same energy data for an economical commercial dishwasher in combination with the lower average generating efficiency for the United States. In this situation, the median primary energy required to wash a reusable cup is 278 kJ. This is some-
what more than the 198 kJ of primary energy required to make a polystyrene foam cup. In other words, for a single use of both cup types in a country with a low average electrical-generating efficiency, there will be no point at which a reusable cup consumes less energy per use than one use of a polystyrene foam cup (Figure 4). Only if the reusable cup were used twice between washes and the disposable cup used only once before being discarded (not a ''level playing field") could any of the reusable cups be a better energy value.
The 278 kJ required to wash a reusable cup with an efficient dishwasher in the United States is less than half of the energy needed to make a paper cup. This means that, compared with a paper cup, a glass cup would use less energy per use after 15 uses, reusable polystyrene after 17 uses, and ceramic after 39 uses. Considering dishwashers of lower energy efficiency, the 340-360 kJ of primary energy per cup used by two of the high-temperature dishwashers in the United States is more than half of the 549 kJ required to make a paper cup. The 549 kJ/cup represents the expenditure of 433 kJ in wood energy, 82 kJ in fossil fuel energy, and 34 kJ in chemicals and processing energy (Hocking, 1994b). Therefore, the
per-cup energy use by any dishwasher exceeds the 82 kJ fossil fuel fabrication component (for an 8.3-g cup) of energy required by a paper cup. This means that, using the criterion of fossil fuel consumption, a single use of a paper cup consumes less than a single use of any of the other cup types examined.
If we compare the energy costs per use for the scenario in which the reusable cups are used twice between washes and the disposables are used twice before discard, the picture changes. The reusables must be used between 30 and 2,000 times (per 15-1,000 washes) before they reach break-even per-use energy consumption relative to the disposable cups.
Net energy expenditure of the disposable and reusable polystyrene cup types could be further reduced by recycling or through energy recovery, instead of relying on landfill disposal. The energy reduction achieved would be equivalent to the intrinsic energy content of the material from which they are made, less the energy required to collect these materials for recycling. This would, of course, also shift the energy break-even value. A discarded ceramic cup has no recycle value except as fill, and the energy required to reuse the material in the glass cup as cullet is almost as much as is required in the initial manufacture of glass from raw ingredients (Berry and Makino, 1974; Boustead and Hancock, 1979; Miller, 1983). Thus, no energy is recoverable from ceramic cups, and negligible energy is recoverable from glass cups. But the intrinsic energy recoverable by recycling the reusable polystyrene, the polystyrene foam, and the paper cup types is about 2,364 kJ/cup (59.1 g/cup x 40 kJ/g), 76 kJ/cup (1.9 g/cup x 40 kJ/g), and 166 kJ/ cup (8.3 g/cup x 20 kJ/g), respectively (Hocking, 1991b), minus the energy required to collect these materials for recycling.
Trash collection and plastics recycling operations have been estimated to have energy costs of 0.28-0.40 kJ/g and 26.7 kJ/g, respectively (Berry and Makino, 1974). If there is a similar energy cost to recycle paper, recycling the material of the three cup types back into cups results in net energy costs of 1,599 kJ/cup [(59.1 g/cup x 0.36 kJ/g collection) + (59.1 g/cup x 26.7 kJ/g reprocessing)] for the reusable polystyrene cup, 51 kJ/cup [(1.9 g/cup x 0.36 kJ/g) + (1.9 g/ cup x 26.7 kJ/g)] for the polystyrene foam cup, and 222 kJ/cup [(8.3 g/cup x 0.36 kJ/g) + (8.3 g/cup x 26.7 kJ/g)] for the paper cup. For this hypothetical scenario, then, recycling these materials entails energy costs equal to about one-fourth those involved in using virgin material for both plastic cup types and two-fifths of that required if virgin material is used for the paper cup. The larger fractional benefit of recycling plastic cups is due solely to the larger intrinsic energy content of polystyrene. This illustrates an important general relationship between materials and recycling: The higher the intrinsic energy content of the material, the higher the potential energy savings that is obtained by recycling rather than using virgin material. It should be pointed out, however, that direct recycling of materials such as these back into food service is not permitted by law, for public safety reasons, without at least a layer of virgin material placed on the food-contact side of the container. In this example, if energy costs of recycling (collection/sorting,
transporting, reprocessing) can be kept low and the grade of end use of the recycled material kept high to maintain the high intrinsic energy content of these materials, then in terms of energy consumption, recycling is an attractive option. Otherwise, recycling is a less attractive option, and an energy recovery strategy is preferable.
Commentary on the Example
The range of correct values for some of the energy inputs considered in this analysis was particularly broad for the manufacture of ceramic and glass cups. But the analysis revealed that the break-even points are not as sensitive to changes in this parameter (the fabrication energy of the reusable cup types) as they are to the energy required for washing and sanitizing the reusable cups or fabricating the disposable cups. This analysis also demonstrated that the high fabrication energy required for the reusable cups became unimportant over enough uses, say 500 or more, compared with the energy required to wash and sanitize them for reuse. It also revealed that the wash energy alone is as much or more than that required to make a polystyrene foam cup in the United States and more than half that required to make a paper cup. Therefore, from an energy standpoint, use of disposable cups is appropriate, especially in situations where the return and reuse rate of the reusable cups is likely to be low.
Many people may prefer to use a ceramic, glass, or reusable polystyrene cup rather than a disposable cup. It is difficult to determine how much weight should be given to this "personal-preference" factor. However, this comparison of energy consumption demonstrates that it is environmentally reasonable to use the disposable cup types when the return rate of reusable cups is likely to be low, or for situations of one-time use such as for large parties, because the energy required for manufacture of the disposable cup types is less than, or very close to, the energy needed to clean a reusable cup.
Finally, this analysis confirms that there is environmental merit in having a diversity of cup types available depending on type of use. In certain circumstances, disposable cups may actually use fewer resources and cause less environmental impact than ceramic, china, or glass cups.
Concluding Notes
In an energy-based analysis of relative ecological merit, it is crucial to define clearly the boundaries to be used. The analyst must also be prepared to adjust these boundaries as the data are gathered if the initial limits to the system appear inappropriate.
Energy costs of raw materials will vary with location, so the area where the technology is being studied has to be specified. Correction factors may have to be used to adjust the energy components used in the analysis, but they should be
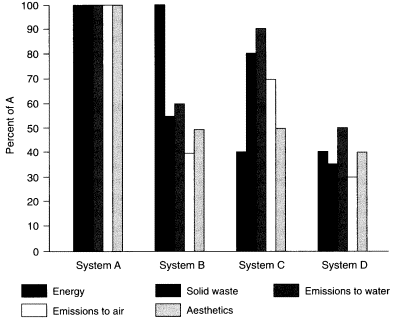
Figure 5
Representations of energy consumption, waste-stream discharges, and aesthetic preferences for four hypothetical technology systems.
specified and the rationale for their selection explained. Spelling out the specifications of an analysis makes it possible for others to repeat it, which lends validity and credibility to the results.
It is possible to apply sensitivity tests to an energy analysis by decreasing or increasing key energy terms and observing the effect on the results. (See, for example, Hocking, 1994b.) In this way, it is possible to identify the factors most important for decreasing the energy consumption of a technology. In the cup example, sensitivity tests demonstrated that the significant factors were the energy requirement for washing reusable cups and the fabrication energy for the disposable cups.
Although energy analysis can be used to compare widely differing technologies and is a useful tool for the quantitative determination of resource expenditure, it contributes little information on the relative emission characteristics of alternate technologies. The primary energy sources, whether coal-fired or gasfired boilers, or hydroelectric, will have different emission characteristics, but the energy assessment process itself does not give this information.
Inventory versus Analysis
A comprehensive picture of a technology's environmental impact requires both an energy assessment and a life-cycle inventory. The analyst must catalog the available energy consumption data and assemble the data relating to solid-waste and water and air emissions. Even with this information, it may be difficult to assess the relative merits of two or more sets of inventory data. Four hypothetical scenarios demonstrate this difficulty (Figure 5). System B has the same energy consumption as System A but produces significantly lower quantities of all three wastes. To compare System B with System C, which has higher volumes of all three waste streams but much lower energy consumption, requires a judgment call or an "eco-points" rating system of some kind. Does the fact that System C has a lower energy consumption offset the higher emission rates? System D, which has both the lowest energy consumption and the lowest emission rates, is most readily rated the best of the four. System B or C could be considered better than A and worse than D, but some kind of aesthetic, ecological, or resource rating system is needed in order to decide.
The scenarios presented in Figure 5 are relatively simple. Even so, the products are difficult to rank. If we increase the level of detail in each of the waste streams to the point of quantifying the particular components of the solid-waste streams (e.g., suspended solids, dissolved solids, biochemical oxygen demand [BOD], for emissions to water; particulate matter, sulfur dioxide, carbon monoxide for emissions to air) the difficulty of ranking technologies increases enormously. How does a technology with high sulfur dioxide emissions and low suspended solids and BOD rate relative to one in which negligible sulfur dioxide is discharged and the aqueous waste stream is high in suspended solids and BOD? Is there a way to assess or weigh equivalent impacts of an air emission and a water emission? For air emissions, such assessments can be based on human toxicity or photochemical-smog-forming potential (Hocking, 1985), but ratings of pollutant categories again require judgment calls (i.e., become subjective).
Finally, there is the difficulty of weighing the significance of the aesthetic, or the personal-preference, factor in technology choices. In Figure 5, System A, which could represent one of the reusable cups, has a high aesthetic rating but also high energy costs and emission loadings. System D, which could represent a disposable cup, has a low aesthetic rating but ecologically favorable low energy costs and low emission loadings. Again, it is a difficult choice to make. Perhaps the best solution is to continue to provide a diversity of cup technologies for the diverse applications to be met. In this way, a reusable cup that begins to become energy competitive after enough uses can fulfill both a reasonable resource expenditure and aesthetic needs.
Alternatively, in situations where little or no reuse is likely, selecting the appropriate disposable cup and accommodating consumer preferences (i.e., insulated for hot drinks or noninsulated/clear for cold drinks) would be the best choice.
Acknowledgments
The author is grateful to the University of Victoria for support of this work and to the many individuals in companies whose readiness to provide information made the reported analyses possible. They are acknowledged wherever feasible in the published full accounts of this work.
References
Berry, R. S., and H. Makino. 1974. Energy thrift in packaging and marketing. Technology Review 76(4):32-43.
Berry, R. S., T. V. Long II, and H. Makino. 1975. Energy budgets: 5. An international comparison of polymers and their alternatives. Energy Policy 3(2): 144-155.
Boustead, I., and G. F. Hancock. 1979. Handbook of Industrial Energy Analysis. New York: John Wiley & Sons.
Chapman, P. F., G. Leach, and M. Slesser. 1974. The energy cost of fuels. Energy Policy 2(3):231-243.
Fenton, R. 1992. The Winnipeg Packaging Project: Report No. 2. Comparison of Coffee Cups. Winnipeg, Manitoba: The University of Winnipeg.
Gaines, L. L. 1981. Energy and Materials Use in the Production and Recycling of Consumer Goods Packaging. Report ANL/CNSV-TM-58. Argonne, Ill.: Argonne National Laboratories.
Hocking, M. B. 1985. Modern Chemical Technology and Emission Control. New York: Springer-Verlag.
Hocking, M. B. 1991a. Paper versus polystyrene: A complex choice. Science 251:504-505.
Hocking, M. B. 1991b. Relative merits of polystyrene foam and paper in hot drink cups: Implications for packaging. Environmental Management 15:731-747.
Hocking, M. B. 1994a. Disposable cups have eco merit. Nature 369:107.
Hocking, M. B. 1994b. Reusable and disposable cups: An energy-based evaluation. Environmental Management 18:889-899.
International Energy Agency, Organization for Economic Co-operation and Development (OECD). 1993. Energy Statistics of OECD Countries, 1990-1991. Paris: OECD.
Kindler, H., and A. Nikles. 1979. Energiebedarf bei der Herstellung und Verarbeitung von Kunststoffen. Chemie-Ingenieur-Technik 51(11): 1125-1127.
Kindler, H., and A. Nikles. 1980. Energieufwand zur Herstellung von Werkstoffen: Berechnungsgrundsätze und Energieäquivalenzwerte von Kunststoffen. Kunstoffe 70 (12):802-807.
Miller, R. K., ed. 1983. Energy Conservation and Utilization in the Glass Industry. Atlanta, Ga.: Fairmont Press Inc.
Ringwald, R. M. 1982. Energy and the chemical industry. Chemical & Industry 9:281-286.
United Nations. 1990. World Resources, 1990-1991, A Report by the World Resources Institute and the United Nations Environment Programme. Oxford, England: Oxford University Press.
van Eijk, J., J. W. Nieuwenhuis, C. W. Post, and J. H. de Zeeuw. 1992. Reusable versus Disposable. A Comparison of the Environmental Impact of Polystyrene, Paper/Cardboard, and Porcelain Crockery. Zoetermeer, Netherlands: Ministry of Housing, Physical Planning and Environment.
Vigon, B. W., D. A. Tolle, W. Cornaby, H. C. Latham, C. L. Harrison, T. L. Boguski, R. G. Hunt, and J. D. Sellers. 1992. Product Life-Cycle Assessment: Inventory Guidelines and Principles. Cincinnati, Ohio: U.S. Environmental Protection Agency.