16
The Physiology of High-Altitude Exposure
Allen Cymerman1
INTRODUCTION
The problems inherent in traversing, fighting, and surviving at altitude are as true today as they were almost 2,000 years ago. This is evident by the quotation from Tookim, a Chinese official addressing the Generalissmo Wang Fung in 35 B.C. after crossing the Greater and Lesser Headache mountains near the Korakorams: ''…men's bodies become feverish, they lose colour, and are attacked with headache and vomiting: even the asses and cattle being all in like condition" (Ward et al., 1989, p. 26).
Despite the technological advances made in protecting humans from exposure to environmental extremes such as heat and cold, the problems at altitude are as true today as they were in Tookim's time. It must be remembered
that the only effective means for protection from the effects of hypoxia are the body's own adaptive physiological responses.
There are a host of factors found in the mountain environment that can affect a soldier's well-being. All of the factors shown in Figure 16-1 are important when troops are rapidly deployed to a high-terrestrial environment. Some are operational and can be controlled by trained soldiers; others are unavoidable. Many of the factors are interrelated and are often found in the presence of each other. Although many of these factors have been described and studied individually, they may not be independent of each other and probably act additively or synergistically.
FACTORS IN A MOUNTAIN ENVIRONMENT
Cold and Heat
Average ambient temperatures are reduced 2°C (3.6°F) for every 300-m (984-ft) rise in elevation. This means there is usually about an 8°C (14.4°F) difference between Colorado Springs, Colorado, at 1,829 m (6,000 ft) and the summit of Pikes Peak at 4,300 m (14,110 ft), a temperature and altitude change that is well within the limits of exposure that can be handled by the U.S. soldier of today. At any given hour, the "physiologic" temperature could drop 20°C (36°F) with changes in solar load and wind. In a relatively short time, a soldier could easily go from a sweating situation with several layers of
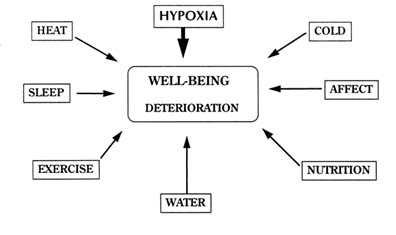
FIGURE 16-1 Interaction of various factors impinging on the well-being of individuals at high terrestrial elevations.
clothing to one in which there could be a freezing injury. Thus, a soldier could experience heat stress while overdressed and exercising in the sun and a short time later expose a hand to extreme wind chill because of a lost glove. This chapter does not review potential additive or synergistic effects of other environmental factors such as temperature but focuses on the effects of acute and long-term hypoxia per se on soldier well-being.
Affect
Studies of acute exposures to altitudes as low as 3,000 m (9,843 ft) show alterations in subjects' cognitive, motor, and affective modalities. After an initial period of euphoria, continued exposure to moderate and high altitudes may produce measurable degradations in personality and cognitive abilities. These degradations can be sufficiently severe to be classified as paranoia, obsessive-compulsive behavior, depression, and hostility. Although changes to this degree are rare, some changes in personality traits are noticed in most individuals (Nelson, 1982; Shukitt and Banderet, 1988; Tune, 1964).
Water
Water lost to the environment is characterized as both sensible and insensible. Sensible water losses include that lost through urination (1,500 ml/d), feces (100 to 200 ml/d), and perspiration (100 ml/d). Sensible perspiration can be as high as 2 liter/h or more depending on body temperature, exercise level and duration, and body weight. Insensible water loss is defined as losses due to vaporization through both the lungs and skin and can average about 700 to 1,000 ml/d (Noakes, 1993; Rhoades and Tanner, 1995).
Expired air is normally saturated with water at body temperature (47 mm Hg at 37°C [99°F]) regardless of the ventilatory rate and the altitude. Because relative humidity is generally lower and ventilatory rates higher with increasing altitude, the amount of insensible water loss can be large enough to cause dehydration and weight loss. Estimates of insensible water loss at high altitudes have been derived from sea-level measurements since no direct measurements have been made. Milledge (1992) estimates a water loss of about 1 liter/d for a ventilation rate of 40 liter/min in dry air at -15°C (5°F). The calculation is based on an equation developed by Ferrus et al. (1984) and assumes that expired air temperature is below normal body temperature and not fully saturated. This is comparable to the value of 750 ml/d predicted by Buskirk and Mendez (1967). Pugh (1964) estimated that to maintain a urine output of 1.5 liter/d, ingestion of 3 to 4 liters of fluid was required in men climbing for 7 hours. His direct measurements of water loss for the lungs
during exercise were about 2.9 g/100 liter of air (body temperature pressure saturated or BTPS2).
Another factor to consider with the increases in ventilation associated with altitude is increased respiratory heat loss. Given the same ventilatory rate at sea level and 4,572 m (15,000 ft) (i.e., a lower workload at altitude) and no decrease in expired air temperature, it is estimated that at the same ventilatory rate of 100 liter/min, the respiratory heat loss would be 1.6 times greater (Gonzalez et al., 1985).
It is generally believed that an altitude-induced diuresis normally occurs during the initial phases of altitude acclimatization when acute mountain sickness, high-altitude pulmonary edema, and high-altitude cerebral edema are not present. When significant symptomology of altitude illness is present, there is evidence of fluid retention and weight gain (Bärtsch et al., 1991; Hackett et al., 1981, 1982; Singh et al., 1969).
Exercise
Exercise is an inescapable consequence of traveling to high mountainous areas. The two factors associated with exercise that relate to weight loss are the increased water loss caused by high ventilatory rates and the increased energy output. The influence of exercise and energy expenditure on nutrition is covered by Schoene and by Hoyt and Honig (see Chapters 17 and 20 in this volume).
Sleep
The quality and quantity of sleep at altitude are important contributory factors that determine whether individuals acclimatize well or slowly deteriorate. Disturbances in sleep are related to the terrestrial elevation and the length of exposure. Many studies at very high and extreme altitudes confirm that sleep arousals of up to 30 to 40 per night can occur. These arousals are probably caused by increased episodes of periodic breathing. There is also a concurrent 50 percent reduction in total sleep time and a fivefold reduction in the REM (rapid eye movement) sleep stage. Less-disturbed sleep is observed at lower altitudes, but the phenomena may still last for weeks. Individuals commonly report "being awake half the night," "not being able to sleep," and having "frequent disturbing dreams." Problems of sleeping can contribute to mood changes during the day, loss of appetite, and daytime somnolence
(Anholm et al., 1992; Goldenberg et al., 1992; Normand et al., 1990; Sutton et al., 1979; Weil, 1985; White et al., 1987).
STUDY LOCATION
Before considering the requirements for nutrition in a high mountain environment, it is extremely important to remember that current knowledge of the effects of altitude exposure is provided by studies conducted over a wide range of locations and conditions. Data have been collected on climbing expeditions to extreme altitudes, on military field exercises, in mountain laboratories, during trekking excursions, and under simulated conditions in hypobaric chambers. Volunteers for these studies have ranged from average college students, Army soldiers, and Special Forces Mountain Teams to experienced mountain climbers. Many high-altitude researchers simply chose not to conduct nutritional studies in the field because of the difficulty of establishing appropriate controls, heterogeneity of populations, increased study time, and expense. The resultant confounding experimental circumstances can lead to potential complications in interpretation of results, especially where diet, nutrition, and energy balance are concerned.
Factors affecting studies conducted in various locations are shown in Table 16-1. Given the same "physiological" altitude, the stresses placed on the individual can vary greatly depending on the study location. Obviously, the more variables (e.g., temperature, humidity, food, exercise) are controlled and the more simulated the condition, the closer one is to studying the physiology of pure hypoxia.
BIOPHYSICS OF THE ATMOSPHERE
The earth is surrounded by an envelope of compressible gases comprised essentially of 20.95 percent oxygen and 78.08 percent nitrogen. This relationship remains constant up to an elevation of approximately 90 km. Because of the compressibility of gases, the pressure at sea level exerted by these gases is 14.7 lbs/in2 (760 mm Hg), and it decreases proportionately with ascent. Figure 16-2 displays several significant elevations relative to the summit of Mount Everest and illustrates the curvilinear relationship between barometric pressure and terrestrial elevation.
Assuming that cold is not a contributing factor, the problems of high-altitude exposure can be initially attributed to the reduction in the partial pressure of oxygen in the ambient atmosphere and the body's subsequent responses. The relative concentration of oxygen in the atmosphere remains constant at 20.95 percent up to approximately 110,000 m (360,892 ft), but because air is compressible, the pressure it exerts is highest at sea level and is
TABLE 16-1 Advantages and Disadvantages of Different Hypoxic Environments
Mountain |
Mountain Laboratory |
Hypobaric Chamber |
Real-life situational stresses |
Stable and controlled food and water supply |
Sophisticated measurement and study design |
Novel food; possible food contamination |
Protection from inclemency; stable temperature |
Stresses of confinement, noise, etc. |
Availability of potable water |
Sleep disturbances |
Sleep disturbances |
Adverse weather; low ambient temperature |
Infinite exposure time |
Controlled environmental conditions for temperature, pressure, and humidity |
Psychological effects; sleep disturbances |
Constant altitude |
Controlled nutrition and exercise |
Equipment and clothing problems |
Controlled exercise |
|
Abrupt, constant changes in elevation |
|
|
Frequent bouts of demanding exercise or long periods of inactivity |
|
|
|
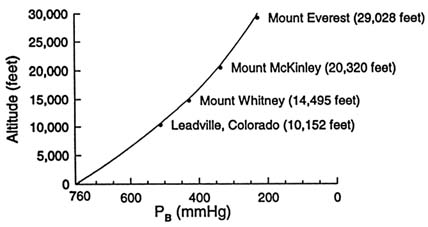
FIGURE 16-2 Relationship between altitude and barometric pressure (PB), with significant high-altitude locations in North America relative to Mount Everest highlighted (3,094; 4,418; 6,194; and 8,848 m [10,152; 14,495; 20,320; 29,028 ft]).
proportionately reduced with altitude. In a field situation, actual barometric pressure at any given elevation is also dependent on the latitude, whereas chamber studies usually utilize a standardized chart based on the ICAO (International Civil Aviation Organization) Standard Atmosphere (Anonymous, 1954).
The ICAO standard is used universally to equate atmospheric pressure and elevation in chambers and aircraft. However, in actual field situations, discrepancies in the ICAO pressure-elevation relationship occur because of temperature (season), latitude, and weather influences. These discrepancies become larger with increasing elevation.
The percent oxygen in levels of the atmosphere remains essentially the same as at sea level regardless of the elevation and latitude. The reduction in barometric pressure, however, does cause a concomitant, obligatory decrease in the partial pressure of oxygen (Po2), which is defined as the specific fractional composition of the mixed gas expressed as a pressure. For example, at sea level, the barometric pressure (PB) is 760 mm Hg, and Po2 is about 160 mm Hg (760 mm Hg × 0.21). At 5,500 m (18,045 ft, PB = 380 mm Hg), the Po2 is only 80 mm Hg (380 × 0.21). In the pulmonary alveoli, this reduction becomes significant because of the almost constant partial pressures of both water vapor (47 mm Hg at 37°C [99°F]) and carbon dioxide (CO2) (40 mm Hg with normal ventilation).
The decrease of ambient Po2 is the direct cause of many medical problems at altitudes above 2,438 m (8,000 ft). This decrease causes several interrelated physiologic responses that function to improve oxygen delivery to the tissues. Depending on the conditions of exposure and the characteristics of the individual, these responses can also be maladaptive, causing illnesses and dysfunction that could compromise performance and well-being (Cymerman and Rock, 1994).
ACUTE PHYSIOLOGICAL RESPONSES
The first consequence of a significant reduction in ambient Po2 is a decreased arterial Po2 that stimulates peripheral and central chemoreceptors. This stimulation occurs when the inspired Po2 is lowered to approximately 122 mm Hg or 1,524 m (5,000 ft) elevation. The sensitivity of peripheral chemoreceptors to hypoxemia (decreased blood oxygenation) is characteristic of an individual and is probably genetically determined. However, it can be modified by external factors such as respiratory depressants (alcohol, soporifics) and stimulants (caffeine, cocoa), but not physical fitness.
Chemoreceptor stimulation causes an increase in ventilation. This increase does not entirely compensate for the decrease in the inspired Po2. As a result, there is a sustained decrease in hemoglobin saturation and arterial Po2 that is
proportional to the altitude. Hyperventilation results in a reduction in arterial CO2 (respiratory alkalosis) that limits the increase in ventilation.
Within several days, the kidneys compensate for the respiratory alkalosis by increasing excretion of bicarbonate. Resting ventilation continues to rise slowly, reaching a maximum in about 1 week. With continued ascent, the process is repeated to a smaller degree with further increases in ventilation. Increased ventilation is the principal characteristic of acclimatization (the adaptation to altitude exposure) and is the primary mechanism responsible for improving the oxygen availability at the cellular level.
Figure 16-3 illustrates the relative changes in ventilation, end-tidal CO2 partial pressure, and blood oxygen saturation over the course of 20 days at the summit of Pikes Peak (4,300 m [14,110 ft]). Resting minute ventilation of approximately 5 to 7 liter/min at sea level can increase to almost 15 liter/min at this elevation. The increase observed in blood oxygen saturation is not entirely independent of ventilatory acclimatization. Some of the increase can be attributed to changes in lung parenchyma, circulation, erythrocytes, and metabolism (Bender et al., 1989).
Figure 16-4 shows that the initial increase in cardiac output is caused principally by the increase in heart rate with little or no change in stroke volume.
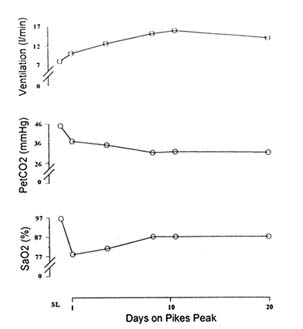
FIGURE 16-3 Resting respiratory responses during acclimatization to 4,300 m (14,110 ft) altitude. SaO2, arterial oxygen saturation; PetCO2, end tidal partial pressure of carbon dioxide. SOURCE: Adapted from Bender et al. (1989).
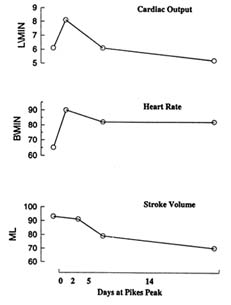
FIGURE 16-4 Relationship between cardiac output, heart rate, and stroke volume during 14 days of acclimatization to 4,300 m (14,110 ft). ML, milliliters; B/MIN, beats per minute; L/MIN, liters per minute. SOURCE: Adapted from Hannon and Vogel (1977).
With continued exposure, stroke volume falls as a result of the reduced plasma volume and decreased venous return. After 1 week of exposure, cardiac outputs are essentially normal or only slightly reduced (Hannon and Vogel, 1977).
Stimulation of the sympathetic nervous system, principally secretion of norepinephrine, as a response to altitude exposure has been confirmed in many studies (Brooks et al., 1991a; Cruz et al., 1976; Cunningham et al., 1965; Hoon et al., 1976; Kotchen et al., 1973; Mazzeo et al., 1991; Moncloa et al., 1965; Reeves et al., 1992a; Young et al., 1989). The typical response of an increase in norepinephrine without a corresponding increase in epinephrine is depicted in Figure 16-5. This increase is probably responsible for many of the cardiovascular and metabolic changes seen with acclimatization, such as augmentation of metabolic rate, lactate accumulation during exercise, and increased heart rate. Norepinephrine increases may also be correlated with alterations in myocardial contractility, atrial natriuretic factor, angiotensin, and aldosterone (Reeves et al., 1992b).
Exact indices of acclimatization are difficult to determine and are altitude-and time dependent. Some of the physiological variables used to delineate successful acclimatization include the plateauing of the rise in ventilation, the
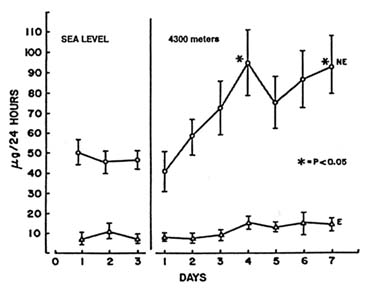
FIGURE 16-5 Differential response of urinary norepinephrine (NE) and epinephrine (E) during 7 days of exposure to 4,300 m (14,110 ft). SOURCE: Adapted from Burse et al. (1987).
compensated respiratory alkalosis, the increased real hematocrit, the restoration of normal blood flow distribution, and altered metabolic pathways.
The degree of adaptability of the human organism to very high terrestrial elevations is best exemplified by the natives of the Andes mountains. From present-day observations and historical records of South American Indians, there is evidence that oxygen concentrations as low as 10.5 percent (equivalent to 5,500 m or 18,045 ft) can be tolerated to do heavy physical work as long as the natives sleep at a lower altitude. High-altitude natives can apparently maintain a routine of working in mines at 5,500 m (18,045 ft) and sleeping at lower elevations for extended periods of time without marked deterioration.
For sojourners to high altitudes without any acclimatization, this level of exposure would result in marked deterioration and illness. The ability for newcomers to acclimatize adequately to a moderate altitude is dependent on the rate of ascent and the time allowed for acclimatization. Figure 16-6 illustrates the relative time, magnitude, and direction of changes at the respiratory, circulatory, and cellular levels with respect to their relationship to general health and performance at 4,267 to 4,572 m (14,000 to 15,000 ft).
Several illnesses can occur with ascent to altitude. Of these, only two are of real concern for the purposes of this book. A brief discussion of these illnesses is warranted because they affect nutrition. These illnesses are termed acute mountain sickness (AMS) and high-altitude cerebral edema (HACE).
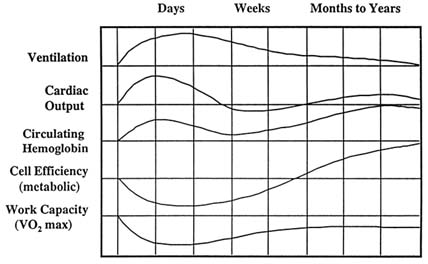
FIGURE 16-6 Approximate size, direction, and temporal changes that occur during acclimatization to 4,267 to 4,572 m (14,000 to 15,000 ft). SOURCE: Adapted from Houston (1982).
AMS is the most common manifestation of the altitude illnesses. It is associated with rapid exposure of the unacclimatized individual to altitudes above 3,048 m (10,000 ft). Symptoms usually start after several hours of exposure. The illness is self-limiting, remitting over the course of 3 to 7 days, depending on staging (temporary residence at an intermediate altitude) and any changes in elevation.
Prominent manifestations of the disorder are listed in Table 16-2. Sleep disorders characterized by periodic breathing are not uncommon. A factor not
TABLE 16-2 Symptoms of Acute Mountain Sickness (AMS) and High-Altitude Cerebral Edema (HACE)
AMS |
HACE |
Headache |
Severe headache |
Nausea |
Nausea |
Vomiting |
Vomiting |
Weakness |
Extreme lassitude |
Lassitude |
Truncal ataxia |
General malaise |
Altered mental status |
Decreased coordination |
Ataxic gait |
Dizziness |
Generalized neurologic abnormalities |
Oliguria |
|
related to AMS per se, but one that probably affects food consumption, is gas expansion in the gastrointestinal tract. The problem obviously persists with continued ascent due to the continued reduction in barometric pressure.
HACE is thought to be a progressive form of AMS with similar symptoms as shown in Table 16-2, albeit on a more severe scale. The incidence of HACE is extremely low but requires immediate attention and medical treatment. From a nutritional standpoint, it should not be a concern for troops at high terrestrial elevations.
WEIGHT LOSS AT ALTITUDE
Altitude exposure usually leads to significant weight losses in nonacclimatized individuals. Table 16-3 summarizes the weight losses of subjects from several selected studies conducted on high-altitude expeditions, in high-altitude field laboratories, and in hypobaric chambers. This weight loss has been attributed to the novelty of the environment, the effects of altitude-related illnesses, and to the factors shown in Figure 16-7. These factors may or may not be related to altitude-induced illness. Weight loss appears to occur in those whose travel commences at sea level and stabilizes with acclimatization. It does not occur to any appreciable extent in high-altitude natives.
The degree of weight loss is apparently dependent on the altitude and the duration of stay at high altitudes. The direct relationship between the severity of hypoxia and body weight loss has not been demonstrated decisively for humans, but has been demonstrated in animals (Schnakenberg et al., 1971). With acclimatization, weight loss may not be observed unless the altitude is extreme, that is, above 5,000 m (16,404 ft). In fact, Pugh (1962) observed an increase in body weight in one subject on the 1960–1961 Silver Hut Expedition when that subject had a respite of 20 days at 4,500 m (14,764 ft) after descent from 5,800 m (19,029 ft). Even in mountain laboratories at moderate altitudes where diet was controlled, there may not be evidence of an obligatory loss of weight (Butterfield et al., 1992; Kayser et al., 1993).
All of the factors shown in Figure 16-7 can contribute to an overall loss in body weight in deployed soldiers who may not have the benefit of time for acclimatization that is afforded to mountain trekkers and climbers.
Diuresis and Hypodipsia
Hypoxia-induced diuresis and subliminal thirst (hypodipsia) are usual occurrences in healthy subjects acutely exposed to altitudes similar to the summit of Pikes Peak (4,300 m [14,110 ft]). With individuals having symptoms of AMS, fluid retention that is independent of fluid intake has been observed. These individuals are characterized by a gain in weight. Bärtch et
TABLE 16-3 Reductions in Body Weights from Selected Expeditions, Field Laboratories, and Hypobaric Chamber Studies
Study |
Site |
Elevation (m [ft]) |
Sample Size |
Durations (days) |
Δ Weight (kg) |
Loss (%) |
Surks et al., 1966 |
Pikes Peak |
4,300 (14,110) |
5 |
8 |
-2.4 |
3.4 |
Siri et al., 1969 |
Mount Everest |
5,400-7,000 (17,717-22,966) |
10 |
63 |
-10.4 |
12.9 |
Grover et al., 1976 |
Chamber |
4,300 |
4 |
5 5 |
-3.4 +2.0 |
- - |
Boyer and Blume, 1984 |
Mount Everest |
5,400 |
8 8 |
32 47 |
-1.2 -6.5 |
1.6 8.8 |
Rose et al., 1988 |
Chamber |
8,848† (29,030) |
6 |
40 |
-7.4 |
8.9 |
Boutellier et al., 1990 |
Mount Lhotse |
8,398 (27,550) |
6 |
58 |
-1.5 |
2.2 |
Bärtsch et al., 1991 |
Capanna Margherita |
4,559 (14,960) |
15‡ |
5 |
-2.25 |
- |
Edwards et al., 1991 |
Potici, Bolivia |
3,500 (11,483) |
20 |
16 |
-3.0 |
4.0 |
Butterfield et al., 1992 |
Pikes Peak |
4,300 |
7 |
21§ |
-2.0 |
- |
Kayser et al., 1993 |
Italian Research Lab, Nepal |
5,050 (16,568) |
8 |
7 14 28 |
-0.3 -2.7 -2.6 |
0.4 3.5 3.4 |
* Subjects received supplemental CO2 to prevent respiratory alkalosis. † Simulated ascent of Mount Everest; inspired Po2 to 43 torr ‡ Subjects who had a weight gain became ill. § No evidence of weight loss with adjusted energy intake after 7 days. |
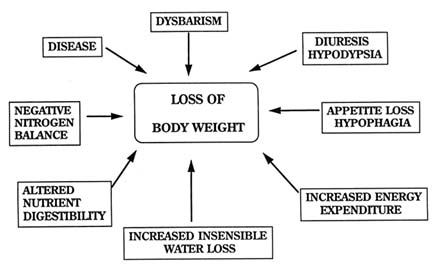
FIGURE 16-7 Factors affecting weight loss at altitude that do not involve altitude-specific illnesses.
al. (1988) found reduced diuresis associated with severe AMS, as have several other investigators (Hackett and Rennie, 1979; Hackett et al., 1976, 1981; Singh et al., 1969). Headache, the most prominent symptom of AMS, can be sufficiently severe to have a negative impact on food intake. It is estimated that food intakes are decreased by as much as 25 to 50 percent during the first 3 days of acute exposures to 4,300 m (14,110 ft), the period when illness symptoms are at their peak (Consolazio et al., 1966; Janoski et al., 1969; Surks et al., 1966).
Dysbarism
Dysbarism is a symptom complex resulting from exposure to decreased or changing barometric pressure unaffected by hypoxia. Although decreased energy intake per se can be associated with gastrointestinal upset, gas expansion above 3,353 m (11,000 ft) intensifies the degree of distress. The expansion of intraluminal gas, which can be caused by both gas expansion and a high-fiber mountain diet, may be very disconcerting and even painful (Hultgren, 1983). If diet and constantly changing altitudes are continued for any length of time, there can be adverse effects on food consumption.
Anorexia
In addition to a possible direct effect on brain centers controlling appetite, changes in menu inherent in military mountain field studies usually result in reduced dietary nutrient intake. Although palatability of food may have been a factor with early dietary studies, a caloric deficit secondary to appetite suppression (anorexia) may still occur when the best possible food is available.
Edwards et al. (1994) studied nutritional intakes and the effects of carbohydrate supplementation of 67 soldiers performing moderate amounts of physical work at altitudes of 3,200 to 3,500 m (10,499 to 11,483 ft) for 13 days. They concluded that despite the availability of ample, well-cooked food, soldiers with high rates of energy expenditure failed to maintain their body weights and lost approximately 1.7 kg. The effect of solid carbohydrate supplementation was not significant. Even when the diet may not be as palatable as in the Edwards study, Butterfield et al. (1992) showed that in seven young men exposed to 4,300 m (14,110 ft) for 21 days and given a supplemented Ensure diet©, weight loss appeared to be halted after the energy intake was purposefully increased to compensate for measured increases in basal metabolic rate during the second week of exposure.
In a short review of nutrition and altitude exposure, Kayser et al. (1992) also concluded that with maintenance of adequate intake, body weight losses attributable to water, fat loss, and muscle wasting could be avoided. The implication from the latter two studies is that there is no dysfunction of nutrient absorption at altitudes up to 5,000 m (16,404 ft); rather, energy intakes are voluntarily reduced for some unexplained reason. The result is that with continued residence at a constant, moderate altitude such as 4,300 m (14,110 ft) and no illness symptomatology, there may be progressive loss of body weight in a military population performing tasks requiring a high level of exertion.
When military operations require ''living off the land," the problems associated with ingestion of indigenous foods are common and well known. Often the basic principles of sanitary food preparation are dismissed when time is limited and basic supplies are in short supply or rationed. Proper sterilization of water is also often neglected when the supply is thought to be clean because of remoteness. This type of negligence usually results in a wide range of gastrointestinal problems such as parasitic infection, anorexia, and diarrhea.
Imbalance Between Energy Intake and Expenditure
The discordance in energy intake and expenditure is evident in military field situations irrespective of the terrestrial elevation. It is not unusual for daily energy expenditures to be on the order of 4,000 to 6,000 kcal, with contributions of approximately 1,200 to 2,800 kcal/d from body energy stores.
This expenditure could produce energy deficits as high as 31 percent (Moore et al., 1992). In two different studies of soldiers on field training exercises at moderate terrestrial elevations (2,200 to 3,100 m [7,218 to 10,171 ft]), Hoyt et al. (1991, 1994) using the doubly labeled isotope method found mean total energy expenditures of 4,919 kcal/d for 11 days and 4,558 kcal/d for 5 days. This level of energy expenditure resulted in body weight losses of 2.5 and 1.3 kg, respectively. It appears that body weight losses, especially at altitude, are inevitable in field studies involving high levels of strenuous exertion, regardless of the availability of food. A more definitive discussion of energy expenditure is provided by Hoyt and Honig (see Chapter 20 in this volume).
Loss of Body Water
Losses in sensible and insensible body water due to working in a high, dry environment can have serious consequences on the ability to function and survive. Insensible water losses are discussed in the section on water. Losses via both avenues, however, will occur at altitude under conditions that are high-hot and high-cold and that result in voluntary dehydration—the difference between water intake and water losses. Especially when exertion is involved, voluntary dehydration will be a universal phenomenon at altitude. The condition occurs soon after exposure and probably lasts for an indefinite period of time. The loss of total body water combined with fluid shifts within the extravascular compartment can interfere with the standard measurements of energy expenditure and body composition (Fulco et al., 1985). Relative hypodypsia—decreased water intake—can be as much as one-half of the fluid loss with active periods in a hot, dry environment (Adolph et al., 1947). It is anticipated that it could be higher under high-altitude conditions.
Nutrient Digestibility
Some studies have suggested a malabsorption of nutrients from the gastrointestinal tract at altitude. Most of these studies have involved mountaineering expeditions and altitudes in excess of 5,000 m (16,404 ft). For example, Pugh (1962) found visual evidence of steatorrhea (excess fat in the stools) at 4,570 m (14,993 ft), and Boyer and Blume (1984) found increased fecal fat content at 5,400 m (17,718 ft). However, the consensus of findings is that fat digestibility is normal up to altitudes of 5,000 m (16,404 ft). The same conclusion has been drawn for carbohydrate absorption and protein (Chesner et al., 1987; Imray et al., 1991; Kayser, 1992; Kayser et al. 1992; Rai et al., 1975; Sridharan et al., 1982). Both Butterfield et al. (1992) and Kayser et al. (1993) conclude that there is no dysfunction of absorption but rather a
dysfunction of intake and that loss of weight can be avoided by matching energy input to expenditure.
When analyzing the results of nutrition studies at altitude, it may be difficult to compare and interpret the conclusions. Boyer and Blume (1984) noted that weight loss on expeditions may be due to the fact that climbers will "bulk up" before expeditions in anticipation of increased energy expenditure and that different genetic and cultural factors may be operative. Sherpas, members of the Tibetan people living on the high southern slopes of the Himalayas, do not undergo the same body composition changes as do Caucasians above 5,400 m (17,718 ft). Statistical problems are also encountered when subject numbers are so low that including or excluding one aberrant subject is sufficient to place the significance of changes in doubt.
In conclusion, at moderate altitudes there appears to be no problem in the digestion and absorption of nutrients from the gastrointestinal tract. Palatability, however, may be altered. Most climbers prefer a high-carbohydrate, low-fat diet, with fatty foods actually becoming distasteful.
Studies bearing on the composition of a "mountain" diet indicate that rations used at sea level would be adequate at moderate altitudes. It is generally believed that high-carbohydrate diets may be beneficial at high altitudes with regard to both amelioration of high-altitude illness symptoms and physical performance (Dramise et al., 1975; Hansen et al., 1972; Strange et al., 1990). In fact, a greater reliance on glucose as an energy source has been demonstrated in several studies of acute and long-term altitude exposure (Brooks et al., 1991b; Dramise et al., 1975; Johnson et al., 1974).
Protein Metabolism
With even a minimal loss of weight at high altitudes, there will be a loss of lean body mass. However, care should be used when interpreting results based on skinfold measurements alone. Skinfold measurements may not be accurate in a high-altitude environment. Skinfold measurements used in equations for estimating fat mass and fat-free mass tend to underestimate the former and overestimate the latter (Fulco et al., 1992).
When intake of food is limited for whatever reason, the body does not appear to use fat stores preferentially over carbohydrate and protein. In the best of diets aimed at reducing fat stores, there is still a 25 percent loss of lean body mass. Of the weight loss observed with long-term exposure, a sizable fraction is due to the reduction in muscle size. Feretti et al. (1990) and Rose et al. (1988) found decreases of up to 14 percent in vastus lateralis cross section. Although detraining (inability to maintain sea-level workloads and exercise durations) of elite climbers may be a possibility to explain the reduced muscle mass, there are indications that smaller fiber sizes may be beneficial in that capillary density is increased and diffusion distances are decreased.
Interestingly, when endurance training is performed in the presence of hypoxia, there is an increase in capillarity and muscle fiber size (Hoppeler and Desplanches, 1992).
There is still evidence that protein metabolism may be altered with moderate hypoxia, at least acutely. Rennie et al. (1988) found significant effects on leucine metabolism in the forearm, and Surks (1966) demonstrated a significant increase in albumin degradation during the first 3 days at 4,300 m (14,110 ft).
AUTHOR'S CONCLUSIONS AND RECOMMENDATIONS
The following conclusions are made regarding the physiology of high-altitude exposure:
- Initial weight loss with altitude exposure may be unavoidable due to increased water losses and decreased energy intake relative to expenditure.
- Weight loss is due to an initial loss of water followed by loss of fat and muscle.
- Increasing food intake can prevent loss of body weight, with energy intake matching expenditure as much as possible.
- There is no problem of nutrient digestibility at moderate altitudes.
- Sea-level rations are well tolerated for several weeks at moderate altitudes.
- A high-carbohydrate, low-fat diet, with a possible liquid carbohydrate supplement, may be the preferred altitude ration.
- Confounding effects of altitude-related illness, uncontrolled physical exertion, and differences in ultimate altitudes complicate interpretations between studies.
Recommendations for future research include controlled studies designed to answer the following questions:
- Can altitude-induced weight losses be reduced? Can weight losses be reduced, stopped, or reversed at any point in the exposure by dietary manipulation? Is the weight loss detrimental?
- Would additional carbohydrates in the diet, especially in liquid form, be efficacious in ameliorating illness and improving physical performance?
- Are the combined effects of altitude and cold or hot conditions additive? How much more energy is required under these conditions to perform a standard amount of physical work? How much more insensible and sensible water is lost?
- Is muscle function altered as a result of loss of muscle mass?
REFERENCES
Adolph, E.F., and associates 1947 Physiology of Man in the Desert. New York: Interscience Publishers, Inc.
Anholm, J.D., A.C. Powles, R. Downey, C.S. Houston, J.R. Sutton, M.H. Bonnet, and A. Cymerman 1992 Operation Everest II: Arterial oxygen saturation and sleep at extreme simulated altitude. Am. Rev. Respir. Dis. 145:817–826.
Anonymous 1954 Manuel de l'atmosphè type OACI. Montreal: Civil Aviation Organization.
Bärtsch, P., S. Shaw, M. Franciolli, and M.P. Gnadinger 1988 Atrial natriuretic peptide in acute mountain sickness. J. Appl. Physiol. 65:1929–1937.
Bärtsch, P., N. Pfluger, M. Audétat, S. Shaw, P. Weidmann, P. Vock, W. Vetter, D. Rennie, and O. Oelz 1991 Effects of slow ascent to 4559 m on fluid homeostasis. Aviat. Space Environ. Med. 62:105–110.
Bender, P.R., R.E. McCullough, R.G. McCullough, S.Y. Huang, P.D. Wagner, A. Cymerman, A.J. Hamilton, and J.T. Reeves 1989 Increased exercise SaO2 independent of ventilatory acclimatization at 4,300 m. J. Appl. Physiol. 66:2733–2738.
Boutellier, U., O. Deriaz, P.E. Di Prampero, and P. Cerretelli 1990 Aerobic performance at altitude: Effects of acclimatization and hematocrit with reference to training. Int. J. Sports Med. 11:S21–S26.
Boyer, S.J., and F.D. Blume 1984 Weight loss and changes in body composition at high altitude. J. Appl. Physiol. 57:1580–1585.
Brooks, G.A., G.E. Butterfield, R.R. Wolfe, B.M. Groves, R.S. Mazzeo, J.R. Sutton, E.E. Wolfel, and J.T. Reeves 1991a Decreased reliance on lactate during exercise after acclimatization to 4,300 m. J. Appl. Physiol. 71:333–341.
1991b Increased dependence on blood glucose after acclimatization to 4,300 m. J. Appl. Physiol. 70:919–927.
Burse, R.L., A. Cymerman, and A.J. Young 1987 Respiratory response and muscle function during isometric handgrip exercise at high altitude. Aviat. Space Environ. Med. 58:39–46.
Buskirk, E.R., and J. Mendez 1967 Nutrition, environment and work performance with special reference to altitude. Fed. Proc. 26:1760–1767.
Butterfield, G.E., J. Gates, S. Fleming, G.A. Brooks, J.R. Sutton, and J.T. Reeves 1992 Increased energy intake minimizes weight loss in men at high altitude. J. Appl. Physiol. 72:1741–1748.
Chesner, I.M., N.A. Small, and P.W. Dykes 1987 Intestinal absorption at high altitude. Postgrad. Med. J. 63:173–175.
Consolazio, C.F., R.A. Nelson, L.O. Matoush, and J.E. Hansen 1966 Energy metabolism at high altitude (3,475 m). J. Appl. Physiol. 21:1732–1740.
Cruz, J.C., R.F. Grover, J.T. Reeves, J.T. Maher, A. Cymerman, and J.C. Denniston 1976 Sustained venoconstriction in man supplemented with CO2 at high altitude. J. Appl. Physiol. 40:96–100.
Cunningham, W.L., E.J. Becker, and F. Kreuzer 1965 Catecholamines in plasma and urine at high altitude. J. Appl. Physiol. 20:607–610.
Cymerman, A., and P.B. Rock 1994 Medical problems in high mountain environments: A handbook for medical officers. Technical Report TN 94-2. U.S. Natick, Mass.: U.S. Army Research Institute of Environmental Medicine.
Dramise, J.G., C.M. Inouye, B.M. Christensen, R.D. Fults, J.E. Canham, and C.F. Consolazio 1975 Effects of a glucose meal on human pulmonary function at 1600-m and 4300-m altitudes. Aviat. Space Environ. Med. 46:365–368.
Edwards, J.S.A., E.W. Askew, N. King, C.S. Fulco, R.W. Hoyt, and J.P. DeLany 1991 An assessment of the nutritional intake and energy expenditure of unacclimatized U.S. Army soldiers living and working at high altitude. Technical Report T10-91. Natick, Mass.: U.S. Army Research Institute of Environmental Medicine.
Edwards, J.S.A., E.W. Askew, N. King, and C.S. Fulco 1994 Nutritional intake and carbohydrate supplementation at high altitude. J. Wilderness Med. 5:20–33.
Ferretti, G., H. Hauser, and P.E. Di Prampero 1990 Maximal muscular power before and after exposure to chronic hypoxia. Int. J. Sports Med. 11:S31–S34.
Ferrus, L., D. Commenges, J. Gire, and P. Varene 1984 Respiratory water loss as a function of ventilatory or environmental factors. Respir. Physiol. 56:11–20.
Fulco, C.S., A. Cymerman, N.A. Pimental, A.J. Young, and J.T. Maher 1985 Anthropometric changes at high altitude. Aviat. Space Environ. Med. 56:220–224.
Fulco, C.S., R.W. Hoyt, C.J. Baker-Fulco, J. Gonzalez, and A. Cymerman 1992 Use of bioelectrical impedance to assess body composition changes at high altitude. J. Appl. Physiol. 72:2181–2187.
Goldenberg, F., J.P. Richalet, I. Onnen, and A.M. Antezana 1992 Sleep apneas and high altitude newcomers. Int. J. Sports Med. 13(suppl. 1):S34–S36.
Gonzalez, R.R., L.A. Stephenson, W.L. Holden, and M.A. Kolka 1985 Respiratory heat loss at altitude: Effect of o2 peak. Physiologist 27:230.
Grover, R.F., J.T. Reeves, J.T. Maher, R.E. McCullough, J.C. Cruz, J.C. Denniston, and A. Cymerman 1976 Maintained stroke volume but impaired arterial oxygenation in man at high altitude with supplemental CO2. Circ. Res. 38:391–396.
Hackett, P.H., and D. Rennie 1979 Rales, peripheral edema, retinal hemorrhage and acute mountain sickness. Am. J. Med. 67:214–218.
Hackett, P.H., D. Rennie, and H.D. Levine 1976 The incidence, importance, and prophylaxis of acute mountain sickness. Lancet 2(7996):1149-1155.
Hackett, P.H., D. Rennie, R.F. Grover, and J.T. Reeves 1981 Acute mountain sickness and the edemas of high altitude: A common pathogenesis? Respiration Physiol. 46:383–390.
Hackett, P.H., D. Rennie, S.E. Hofmeister, R.F. Grover, E.B. Grover, and J.T. Reeves 1982 Fluid retention and relative hypoventilation in acute mountain sickness. Respiration 43:321–329.
Hannon, J.P., and J.A. Vogel 1977 Oxygen transport during early altitude acclimatization: A perspective study. Eur. J. Physiol. 36:285–297.
Hansen, J.E., L.H. Hartley, and R.P.I. Hogan 1972 Arterial oxygen increase by high-carbohydrate diet at altitude. J. Appl. Physiol. 33:441–445.
Hoon, R.S., S.C. Sharma, V. Balasubramanian, K.S. Chadha, and O.P. Mathew 1976 Urinary catecholamine excretion on acute induction to high altitude (3,658 m). J. Appl. Physiol. 41:631–633.
Hoppeler, H., and D. Desplanches 1992 Muscle structural modifications in hypoxia. Int. J. Sports Med. 13(suppl. 1):S166–S168.
Houston, C.S. 1982 Acclimatization. Pp. 158–160 in Hypoxia: Man at Altitude, J.R. Sutton, N.L. Jones, and C.S. Houston, eds. New York: Thieme-Stratton Inc.
Hoyt, R.W., T.E. Jones, T.P. Stein, G.W. McAninch, H.R. Lieberman, E.W. Askew, and A. Cymerman 1991 Doubly labeled water measurement of human energy expenditure during strenuous exercise. J. Appl. Physiol. 71:16–22.
Hoyt, R.W., T.E. Jones, C.J. Baker-Fulco, D.A. Schoeller, R.B. Schoene, R.S. Schwartz, E.W. Askew, and A. Cymerman 1994 Doubly labeled water measurement for human energy expenditure during exercise at high altitude. Am. J. Physiol. 266:R966–R971.
Hultgren, H.N. 1983 High-altitude illness. Pp. 1–26 in Management of Wilderness and Environmental Emergencies, P.S. Auerbach and E.C. Geehr, eds. New York: Macmillan.
Imray, C.H.E., I. Chesner, A. Wright, J.P. Neoptolemos, and A.R. Bradwell 1991 Fat absorption at altitude: A reappraisal. P. 306 in Hypoxia and Mountain Medicine, J.R. Sutton, G. Coates, and C.S. Houston. eds. Burlington, Vt.: Queen City Publishers, Inc.
Janoski, A.H., B.K. Whitten, J.L. Shields, and J.P. Hannon 1969 Electrolyte patterns and regulation in man during acute exposure to high altitude. Fed. Proc. 28:1185–1189.
Johnson, H.L., C.F. Consolazio, R.F. Burk, and T.A. Daws 1974 Glucose-14 C-UL metabolism in man after abrupt altitude exposure (4,300 m). Aerosp. Med. 45:849–854.
Kayser, B. 1992 Nutrition and high altitude exposure. Int. J. Sports Med. 13(suppl. 1):S129–S132.
Kayser, B., K. Acheson, J. Decombaz, E. Fern, and P. Cerretelli 1992 Protein absorption and energy digestibility at high altitude. J. Appl. Physiol. 73:2425–2431.
Kayser, B., M. Narici, S. Milesi, B. Grassi, and P. Cerretelli 1993 Body composition and maximum alactic anaerobic performance during a one month stay at high altitude. Int. J. Sports Med. 14:244–247.
Kotchen, T.A., R.P. Hogan, A.E. Boyd, T.K. Li, H.C. Sing, and J.W. Mason 1973 Renin, noradrenaline and adrenaline responses to simulated altitude. Clin. Sci. 44:243–251.
Mazzeo, R.S., P.R. Bender, G.A. Brooks, G.E. Butterfield, B.M. Groves, J.R. Sutton, E.E. Wolfel, and J.T. Reeves 1991 Arterial catecholamine responses during exercise with acute and chronic high-altitude exposure. Am. J. Physiol. 261:E419–E424.
Milledge, J.S. 1992 Respiratory water loss at altitude. Newslett. Int. Soc. Mountain Med. 2:5–7.
Moncola, F., M. Gomez, and H. Anda 1965 Plasma catecholamines at high altitudes. J. Appl. Physiol. 20:1329–1331.
Moore, R.J., K.E. Friedl, T.R. Kramer, L.E. Martinez-Lopez, R.W. Hoyt, R.E. Tulley, J.P. DeLany, E.W. Askew, and J.A. Vogel 1992 Changes in soldier nutritional status and immune function during the Ranger training course. Technical Report T13-92. Natick, Mass.: U.S. Army Research Institute of Environmental Medicine.
Nelson, M. 1982 Psychological testing at high altitudes. Aviat. Space Environ. Med. 53:122–126.
Noakes, T.D. 1993 Fluid replacement during exercise. Exerc. Sports Sci. Rev. 21:297–330.
Normand, H., M. Barragan, O. Benoit, O. Bailliart, and J. Raynaud 1990 Periodic breathing and O2 saturation in relation to sleep stages at high altitude. Aviat. Space Environ. Med. 61:229–235.
Pugh, L.G.C.E. 1962 Physiological and medical aspects of the Himalayan scientific and mountaineering expedition, 1960–61. Br. Med. J. 2:621–627.
1964 Animals in high altitudes: Man above 5,000 meters—mountain exploration. Pp. 861–868 in Handbook of Physiology, Adaptation to the Environment, D.B. Dill, E.F. Adolph, and C.G. Wilber, eds. Washington, D.C.: American Physiological Society.
Rai, R.M., M.S. Malhotra, G.P. Din, and T. Sampathkumar 1975 Utilization of different quantities of fat at high altitude. Am. J. Clin. Nutr. 28:242–245.
Reeves, J.T., R.S. Mazzeo, E.E. Wolfel, and A.J. Young 1992a Increased arterial pressure after acclimatization to 4300 m: Possible role of norepinephrine. Int. J. Sports Med. 13(suppl. 1):S18–S21.
Reeves, J.T., L.G. Moore, E.E. Wolfel, R.S. Mazzeo, A. Cymerman, and A.J. Young 1992b Activation of the sympatho-adrenal system at high altitude. Pp. 10–23 in High Altitude Medicine, G. Ueda, ed. Matsumoto, Japan: Shinshu University.
Rennie, M.J., P. Babij, J.R. Sutton, J.J. Tonkins, W.W. Read, C. Ford, and D. Halliday 1988 Effects of acute hypoxia on forearm leucine metabolism. Prog. Clin. Biol. Res. 136:317–323.
Rhoades, R.A., and G.A. Tanner 1995 Medical Physiology. Boston: Little Brown and Co.
Rose, M.S., C.S. Houston, C.S. Fulco, G. Coates, J.R. Sutton, and A. Cymerman 1988 Operation Everest II: Nutrition and body composition. J. Appl. Physiol. 65:2545–2551.
Schnakenberg, D.D., L.F. Krabill, and P.C. Weiser 1971 The anorexic effect of high altitude on weight gain, nitrogen retention and body composition of rats. J. Nutrition 101:787–796.
Shukitt, B.L., and L.E. Banderet 1988 Mood states at 1600 and 4300 meters terrestrial altitude. Aviat. Space Environ. Med. 59:530–532.
Singh, I., P.K. Khanna, M.C. Srivastava, M. Lal. S.B. Roy, and C.S.V. Subramanyam 1969 Acute mountain sickness. N. Engl. J. Med. 280:175–184.
Siri, W.E., A.S. Cleveland, and P. Blanche 1969 Adrenal gland activity in Mt. Everest climbers. Fed. Proc. 28:1251–1256.
Sridharan, K., M.S. Malhotra, T.N. Upadhayay, S.K. Grover, and G.L. Dua 1982 Changes in gastrointestinal function in humans at an altitude of 3,500 m. Eur. J. Appl. Physiol. 50:145–152.
Strange, S., L.B. Rowell, N.J. Christensen, and B. Saltin 1990 Cardiovascular responses to carotid sinus baroreceptor stimulation during moderate to severe exercise in man. Acta Physiol. Scand. 138:145–153.
Surks, M.I. 1966 Metabolism of human serum albumin in man during acute exposure to high altitude (14,100 feet). J. Clin. Invest. 45:1442–1451.
Surks, M.I., K.S. Chinn, and L.R. Matoush 1966 Alterations in body composition in man after acute exposure to high altitude. J. Appl. Physiol. 21:1741–1746.
Sutton, J.R., C.S. Houston, A.L. Mansell, M.D. McFadden, P.M. Hackett, J.R.A. Rigg, and A.C.P. Powles 1979 Effect of acetazolamide on hypoxemia during sleep at high altitude. N. Engl. J. Med. 301:1329–1331.
Tune, G.S. 1964 Psychological effects of hypoxia: Review of certain literature from the period 1950 to 1963. Percept. Mot. Skills 19:551–562.
Ward, M.P., J.S. Milledge, and J.B. West 1989 High Altitude and Medicine, p. 26. Philadelphia: University of Pennsylvania Press. Quoting A. Wylie, Notes on the Western regions, translated from the 'Tseen Han Shoo Book 96, Part 1, Volume 10, J.R. Anthropology Institute (1881):20–73.
Weil, J.V. 1985 Sleep at high altitude. Clin. Chest Med. 6:615–621.
White, D.P., K. Gleeson, C.K. Pickett, A.M. Rannels, A. Cymerman, and J.V. Weil 1987 Altitude acclimatization: Influence on periodic breathing and chemoresponsiveness during sleep. J. Appl. Physiol. 63:401–412.
Young P.M., M.S. Rose, J.R. Sutton, H.J. Green, A. Cymerman, and C.S. Houston 1989 Operation Everest II: Plasma lipid and hormonal responses during a simulated ascent of Mt. Everest. J. Appl. Physiol. 66:1430–1435.
This page in the original is blank. |