transducing machine such as the bacteriorhodopsin “solar cell,” producing a light-powered machine for specialized transport of chemicals to a specific desired location.
Such work would require expertise in several different molecular machines, a combination of specialties rarely found within a single laboratory. This area is thus a good example of the need to encourage collaboration among groups of researchers involved in biomolecular materials research.
4
Needed Developments
Overview
Certain needs must be addressed to effectively take advantage of the important scientific and technological opportunities that this report and other studies42 have documented. The strong multidisciplinary skills required to make progress in the field, as well as the broad potential technological impact, present special challenges for research, infrastructure, and funding. The basic features of these challenges have also been documented in the studies referred to above. In this report the panel draws from these other studies, many of whose findings it shares, and provides additional input based on the panel's efforts.
Although the research needs documented below are particular to the area of biomolecular materials, the infrastructure and funding challenges contain many features common to other frontier areas that are emerging at the interfaces between established disciplines. Biomolecular materials is centered at the interface between materials science and biology, two fields that are themselves experiencing strong intellectual and technological progress. Materials science is itself highly multidisciplinary; it bridges the sciences and engineering. Thus, providing the needed infrastructure and funding that will successfully and effectively bring materials scientists and biologists together, and will supply them with the supporting infrastructure for research, education, technology transfer, and funding, represents a significant challenge to existing mechanisms.
In this section, after proposing broad research priorities and mid-term research goals for the field, the panel suggests some options with respect to infrastructure and funding mechanisms.
Research Priorities
This subsection discusses broad research priorities for the field. It gives specific examples of research areas that should be pursued over the next five to seven years. Success in accomplishing these research goals will provide a measure of the extent to which the possibilities outlined in Section 3, “Opportunities,” can be realized within a reasonable time.
Colloids
Because they are mechanically rigid (i.e., have a shear modulus) colloidal particles can serve as substrates for molecularly thin films of biopolymers or other surface-active agents. In applications in which film orientation is not important, coated colloidal particles provide an efficient means to pack these films. Extensive use has been made of silica and latex particles as substrates to which antibodies and antigens could be attached for assaying and in drug delivery applications. Lock-and-key protein
systems such as the biotin-avidin couple may be used as controllable strong adhesives. Colloidal dispersions have also been used as a solvent for self-assembling lamellar phases of surfactants. In particular, superparamagnetic grains have been used to allow orientation of the smectic liquid crystal phase in magnetic fields as low as a few gauss. The physics of the phase behavior of these amphicolloids is just now beginning to be studied. It is likely that there will be increasing activity in the study of colloid-surfactant interactions. Other studies, 43 mostly carried out in Europe, have investigated colloidal particles in polymer solutions. They have demonstrated that under appropriate conditions the particles may adsorb on the polymers, forming “pearl necklaces” that have the potential to combine the excellent mechanical properties of polymers with useful electronic or optical properties of the solids. In the future, we may expect biomolecular applications based on such “necklaces.”
Biosynthesis of Polymers
Although there has been impressive progress in the development of new polymeric materials by biosynthetic pathways, important challenges in synthesis remain. Artificial DNAs, especially those with highly repetitive sequences, can be so unstable in microbial hosts that they are rapidly deleted from the cellular population or are rearranged. Artificial messenger RNAs can be rapidly degraded or translated into protein with unacceptably low efficiency. Even after a protein product is formed, it may be toxic to the host cell or subject to rapid metabolic turnover. Bioprocess engineering for the separation and recovery of products from genetic engineering is, at present, a time-consuming and expensive step that is often rate-limiting. Further work on each of these problems will be required to make protein biosynthesis a tool of broad utility in materials science and technology.
Processing of Biosynthetic Polymers
As novel in vivo synthesized polymers become increasingly available, researchers need to address processing issues. The principles of self-assembly imply slow processing in order to achieve equilibrium. Practical situations may demand assisted assembly, however, and indeed the modification of self-assembly by external controls may in certain cases prove beneficial. Alternatively, the use of processing techniques that have worked well with synthetic polymers may prevent realization of the inherent properties present in precision-built biosynthetic materials. Processing of biosynthetic materials demands detailed investigation.
Surfactant-based Self-Assembly.
In order to realize the potential of self-assembled tubules for applications such as those mentioned in the “Status” section, the dimensions of the tubules need to be engineered for optimal performance for each application. Such an effort will require a better fundamental understanding of basic tubule phenomena. In addition, the cost of the tubule-based approach must be consistent with operational requirements and the competitive marketplace.
Multicomponent Self-Assembly
A high priority should be assigned to learning to control the processes by which multicomponent self-assembling systems evolve for homogeneous states.
Multicomponent self-assembly can yield structure at several length scales. The patterns that can be formed by a suitable quench from an initially homogeneous state can vary over several orders of magnitude in size. This variability arises from the many possible combinations of phase transition mechanisms (e.g., spinodal decomposition versus nucleation and growth), including phase transitions of one or more components. For example, the transition of one of the components from liquid to liquid
crystal, homogeneous liquid to microphase-separated liquid, or liquid to crystal can arrest the overall phase separation process at an intermediate stage. It is apparent that such multiple combinations of phase transitions give an extremely rich variety of possible structures, all of which should be accessible with a suitable choice of components and processes.
An illustrative example from the laboratory of Hashimoto44 demonstrates how a binary blend of isotactic polypropylene (PP, a crystalline polymer) and ethylene-propylene rubber (EPR, a random amorphous copolymer) can result in a novel morphology with two important length scales. A blend of PP and EPR was cooled from the high-temperature single-phase melt state to a temperature above the melting point of the PP but below the spinodal curve of the blend. After spinodal decomposition took place for some time, the blend was again quenched, now to below the melting point of the PP, whereupon crystallization of the PP effectively pinned the structure. This process history resulted in volume-filling PP spherulites approximately 100 mm in diameter with a fine-scale (0.1-mm) internal periodic bicontinuous structure of packets of PP lamellar crystals and amorphous EPR-rich regions from the initial spinodal decomposition. Thus the two-stage quench process produced and fixed a dual morphology consisting of spherulites and bicontinuous domain structures.
Several possibilities exist for pinning (fixing) an evolving system. These include crystallization, vitrification, and chemical cross-linking. Further morphological control can be added by imposing an electric, magnetic, or flow field during processing. For example, one can couple the shear deformation rate with the rate of chain relaxation, the rate of phase separation, and the crystallization rate to achieve structures with strong shape anisotropy that arises from the phase separation, phase elongation, and crystallization of flow-oriented molecules.
Establishing Design Rules for the Development of Advanced Molecular Materials
Improved understanding of the role that molecular structure and self-assembly have in determining molecular architectures leads researchers to be optimistic about the possibility of designing new materials with novel function starting at the level of the molecule, i.e., from the bottom up. Advances in computational techniques have led to a clearer understanding of protein folding. New insights in statistical physics have led to a better understanding of the role of chirality in determining the molecular architecture that leads to microstructure formation and novel properties in heteropolymers. Coupled with these recent theoretical and computational advances, the advent of new characterization tools that can successfully probe molecular architectures (e.g., atomic force microscopy (AFM), scanning tunneling microscopy (STM), and near-field microscopy) suggests that it will soon be possible to design advanced materials based on their engineering requirements by appropriately designing their molecular or polymeric structures.
Imaging on Mesoscopic Scales
A variety of techniques exist for examining the structure of materials on molecular and macroscopic length scales. Fewer methods exist, however, for mesoscopic length scales, i.e., 5 nm to 1 µm, the scale relevant for many of the systems discussed here. The development of scanning microscopies such as AFM, STM, and lateral force microscopy (LFM) has been a major advance, but these powerful methods have limited application.
Recent developments in soft x-ray optics, such as multilayer mirrors and high-resolution zone plates, have stimulated work on a new class of microscopes: x-ray high-fidelity optical microscopes and high-resolution and lower-fidelity electron microscopes. These will provide images of soft materials at high-resolution in their natural environment. A reflection imaging soft x-ray microscope is based on the fact that surfaces of different materials have reflection coefficients for soft x-ray radiation near 10 to 20 nm
that differ by up to several percent. At these wavelengths the resolution of such a microscope can be an order of magnitude better than that of an optical microscope. The reflection coefficient is strongly dependent on the material and the angle of incidence of the radiation, and thus provides sufficient contrast for imaging microscopy. The soft x-ray reflection microscope, when fully developed, will make it possible to view the surface and near-surface structure of soft biomolecular materials at a relatively high-resolution—not as high as that obtained with a scanning electron microscope, but achievable in a natural state without staining. The technique will add significant new information to data obtained with the atomic force microscope and the near-field scanning microscope, especially for those cases in which subsurface structural information is important.
Vesicle Adhesion and Fusion
Many of the interactions between membranes leading to vesicle adhesion are reasonably well understood and are similar to the Derjaguin-Landau-Verwey-Overbeek (DLVO) interactions between simple colloidal particles. As a result, vesicle stabilization methods based on classical colloidal schemes have been successful, including steric stabilization by end-grafting polymers to lipid molecules in the bilayer,45 or electrostatic stabilization, as in the case of equilibrium vesicles made from cationic-anionic surfactant mixtures.46 However, there exist interactions unique to membranes that are less well understood, such as repulsion due to membrane undulations or protrusions, which depend sensitively and in unknown ways on membrane composition, lipid properties, and other factors. Additional experimental and theoretical work needs to address the dependence of membrane elasticity on membrane composition and how that dependence affects membrane adhesion.
The mechanism of fusion is still elusive from both an experimental and theoretical viewpoint. Many proteins and ions are known to enhance fusion, but the detailed pathway of fusion is not yet known. On the experimental side, new light-sensitive calcium chelators47 might be used to examine the early steps of calcium-induced fusion in membranes. Biochemical and biophysical studies are necessary to examine the effects of cell fusion proteins and their mechanisms.48
Membrane Proteins
Membrane-associated proteins offer exciting opportunities for the development of advanced materials. Membrane proteins serve as active components and as such facilitate some of the most important cellular processes, including nerve conduction, energy conversion, active ion and molecular transport, and cell-cell adhesion.
A future goal is the development of new types of materials that incorporate the functional activity of membrane-associated proteins. For example, functionalized biomolecular interfaces that incorporate receptor proteins could form the basis for developing advanced materials that serve as chemical and biological sensors, or materials with controlled interfacial properties (e.g., adhesion and lubrication). Membrane proteins with photoactivity could be used to develop a new class of optoelectronic materials in the nascent field of molecular electronics.
Bioactive gels based on membrane proteins incorporated in lipids (Figure 12) are expected to become increasingly important. The bioactive gel would combine (1) the optimal mechanical integrity and processability properties of the host polymer together with (2) the particular intrinsic activity of the protein macromolecule, leading to a new class of gels suitable for a variety of purposes including chemical and biological sensors. For example, many biotechnology companies are anxious to improve
skin-healing gels that are used on burn patients. One technique that has been developed is the incorporation of a peptide in the disordered gels that acts as a glue for the incoming white blood cells that are needed for the initial scavenging of dead cells. These bioactive gels have been found to significantly shorten skin healing times. Membrane-based bioactive gels would have the advantage (over disordered gels) of having a natural membrane environment for the needed peptide or protein to attach to via an anchor.
Opportunities for the development of new biomolecular materials based on membrane proteins also stem from the natural tendency of some membrane proteins, such as bacteriorhodopsin and gap junctions, and proteins constituting the outermost wall of bacterial surface layers (S-layers), to self-assemble into two-dimensional lattices. For example, given their molecular sieving ability derived from their microscopic pore structures, ranging typically between 2.0 to 5.0 nm for different strains, specifically processed multilayer stacks of S-layers will result in a tortuous molecular path with potential for use in separations technology.
Membrane protein self-assembly is expected to play an increasingly important role in obtaining increased temperature stability for the development of heat-proof proteins and enzymes. Proteins that are stable at high temperatures will find utility in numerous present-day and future biotechnology applications, such as biosensors (e.g., toxin detectors), bioreactors (for purifying genetically engineered proteins with high-temperature sieves), and catalytic applications (e.g., current polymerase chain reaction (PCR) machines, which use enzymes at high temperatures).
The recent finding that the membrane protein bacteriorhodopsin may be stabilized to 140°C through higher-order self-assembly in dry films49 suggests the generalization of the technique for temperature stabilization, through higher-order self-assembly, of a variety of functional proteins. A promising approach50 to higher-order self-assembly is via schemes involving the specific binding of macromolecules to functionalized interfaces, e.g., by using the biotin-avidin scheme pioneered by Uzgiris and Kornberg.51
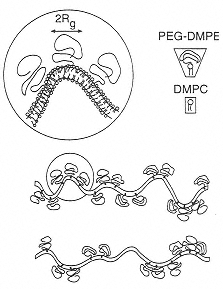
Figure 12
Two undulating fluid membranes [composed of dimyrithristoyl phosphatidyl choline (DMPC) and cosurfactant pentanol (single chain)] with poly(ethylene glycol)-derived polymer (PEG-lipid) hydrophobically anchored but freely diffusing within the membrane. This system forms a gel. (Reprinted, by permission, from H.E. Warriner, S.H.J. Idziak, N.L. Slack, P. Davidson, and C.R. Safinya, Science 271:969–973 (1996). Copyright © 1996 by the American Association for the Advancement of Science.)
Gene Therapeutics
Genetic engineering, gene therapy, and gene therapeutics depend on the transfer of different size nucleic acids to the cytoplasm of bacteria or within the cytoplasm or nucleus of eukaryotic cells. Retroviral vectors are currently the most common method of gene delivery in gene therapy because of their efficient and stable integration of nucleic acid into the host genome.52 The conventional non-viral
transfer methodologies, which have transfection rates significantly lower than viral transfection rates, include anionic liposomes that encapsulate nucleic acid, calcium-phosphate precipitation, use of polycationic reagents (DEAE-dextran or polylysine), and electroporation. An important recent development involves the use of cationic liposomes as non-viral vectors of nucleic acids and recombinant DNA molecules. While the transfection rates and reproducibility in many cells have been found to be significantly enhanced as compared to the other chemical methods involving non-viral vectors, the mechanism of transfection via cationic liposomes remains unknown.
An important future development in gene therapy and therapeutics will come about through an increased understanding at the molecular level of the mechanisms of transfection via chemical methods such as those involving liposomes.
Biological Computation
Discovery of the polymerase chain reaction (PCR) as a method for amplifying very small amounts of DNA has ushered in a wide variety of possible new applications. Although used primarily for genetic engineering and forensics, the PCR reaction has also been used in a “biological computation,” in which DNA recognition was the basis for solving the Hamiltonian path problem, an important mathematical problem in combinatorics that involves finding a path between vertices of a graph.53 The path was encoded in strands of DNA. The first half of a particular DNA strand encoded the starting vertex of a link in the graph, and the second half encoded the target vertex. A complete solution was deduced by using PCR primers for the initial and final vertices.
Sensor Development
Mid-term research goals for biosensors include the development of better methods for interfacing biomolecule-sensing units with the device. Achieving this goal will require a better understanding of surface chemistry and surface interactions, and perhaps the development of better transduction mechanisms.
Biological molecules are often non-robust, and one of the challenges in biomaterials research for sensors is to understand what factors contribute to thermal and oxidative stability of biomolecules. Furthermore, the response of a biosensing device is often quite slow, and research is needed to improve response times.
Processing Using Templates.
Spatial and temporal in vivo characterization of biomineralization growth processes is providing insight into biomolecular templating of inorganic phase structure, phase transformations, crystallographic orientation, and metamorphosis through growth stages. Studies of this type are leading to the “bioinspired” synthesis of composite materials that are structurally designed at nano-, meso- and micro-length scales by templating inorganic growth with surfactants and vesicular arrays. An example is the condensation of calcium hydroxyapatite phases with mesoporosity induced by liquid crystal-like surfactant array templating to generate mesoporosity and a hierarchical longer-range structure generated through a second vesicular-like templating component. Multicomponent cooperative assembly of this type, involving organic and inorganic phases, can be expected to lead to polyphasic materials with nano-structured regions that are spatially well-defined and, if desired, chemically accessible for modification during and after the initial synthesis processing.
Infrastructure
Two previous studies have recognized the challenge that multidisciplinary research presents to the existing infrastructure. The 1990 National Science Foundation (NSF) study of biomolecular materials reached the following conclusion:54
Research in biomolecular materials requires expertise in both biological and materials sciences. Researchers must be capable of working across a variety of disciplines. Furthermore, organizational structures have to be created within industry, government, and universities that facilitate synergistic interactions among scientists, each of whom may have outstanding individual skills.
In the National Research Council study on interdisciplinary research, a similar theme was presented but with a more focused target:55
The conclusions and recommendations on policy strategies are all directed toward advancing the following goals:…
- forging new links between disciplines through educational programs and institutions and through professional activities,…
- ensuring that the most productive and beneficial areas of interdisciplinary research are well supported, and
- identifying factors that create respectable and productive environments for interdisciplinary research collaboration.
Both studies also recognized the need to couple research in biomolecular materials to technology that could benefit the economy and quality of life:
National competitiveness in a number of areas will, to a large extent, hinge on the support for education, research and knowledge transfer in this research area.56
The creation of effective mechanisms for the flow of knowledge and invention between academic institutions and industry is becoming an increasingly important factor in funding for university research and in improving the competitiveness of U.S. business in biotechnology, medical devices, and pharmaceuticals.57
The studies found further that academic-industrial interaction has become a significant stimulus for interdisciplinary research.
The panel's deliberations led it to agree with the above conclusions, with the added emphasis that as time passes, and as the record of significant results grows and the potential economic impact becomes more apparent, the need for providing the new infrastructure is becoming even more acute. Put simply: The existing infrastructure is not keeping pace with scientific and technological opportunities in the field of biomolecular materials and in other fields that are emerging at the interfaces between established disciplines.
The panel considered at length what course it should suggest to overcome these well-documented barriers to interdisciplinary biomolecular materials research. The outcome of its deliberations was greatly influenced by the successful approach that led to the establishment of university programs in materials science and engineering over the last decade. The situation for materials science and engineering a few decades ago was similar to that for the field of biomolecular materials today. Namely, the field was clearly recognized as important for the United States, but capturing its potential required bringing physical scientists and engineers together. Two parallel and related approaches were taken that managed to successfully overcome the barriers that existed at that time: (1) specific organizations and funding programs directly targeted at materials science and engineering were established by the relevant federal agencies, and (2) centers were created at major research universities at a scale that was sufficient to influence the university culture to establish academic programs in the field. By all accounts, these efforts have been very successful in providing the United States with the expertise, trained students, and knowledge base that have been so essential to progress in both the scientific and the technological aspects of materials science and engineering.
As documented throughout this report, there now exists a need to extend this approach to include the biological sciences, as well as engineering and the physical sciences, by creating organizations and funding mechanisms that are directly targeted at biomolecular materials research.
In 1995 a first step was taken toward addressing this need when the NSF Directorate for Mathematical and Physical Sciences created its Office of Multidisciplinary Activities. This is a very positive development, but in the panel's opinion it should be extended to other agencies, particularly the National Institutes of Health (NIH). Furthermore, to have a significant impact specifically on the field of biomolecular materials there need to be directly targeted funding mechanisms that can create on a smaller scale the critical mass of activity that has been created over the last decade in materials science and engineering. Only then can we be sure that in the 21st century the United States will have the experience and knowledge needed to capture the scientific and technological opportunities that this report describes.
Action Options
The panel has identified four options that could help to stimulate progress in the field:
- Interdisciplinary collaborations could be encouraged by a new mode of research through which small numbers of scientists would come together to work on a specific problem, such as the ones identified in this report. This mechanism would encourage new collaborations while keeping their size small to help ensure accountability.
- Consortia in biomolecular materials could be developed, i.e., groups of investigators that are focused on a specific theme or a specific instrumental capability. Such groups could involve scientists at a particular site such as a university campus or a government laboratory, or they could be consortia involving several sites. They would vary in size but would each have a well-defined focus: specific instruments, particular scientific problems, or a defined technological goal. Pre-existing collaborations with established track records of interdisciplinary activity should be favored in establishing these groups. Some of the groups could be built into existing structures such as the Materials Research Laboratories (MRLs), Science and Technology Centers (STCs), and government laboratories. Groups that have special facilities should be open to external scientists. Geographical dispersion could be a component in the selection criteria. Incorporation of the government laboratories into the groups should be strongly encouraged since the government laboratories house a broad spectrum of instruments, experience in instrument development, and relevant expertise in such areas as synchrotron radiation, neutrons, imaging
- (electron microscopy, scanning tunneling microscopy, atomic field microscopy, and x-ray microscopy), and chemical and biological synthesis and characterization.
- Academic programs could be established at universities to encourage curriculum development and training in biomolecular materials. These programs would bridge biology, materials science, and the physical sciences. The multidisciplinary character of biomolecular materials research, though in many ways a great strength, can be a barrier for students pursuing an education in the field. New academic programs and curriculum development could help to overcome this problem. It is important that students are trained in one of the disciplines in depth, however, obtaining interdisciplinary breadth during the research phase of their graduate careers. One way to support such training could be the provision of special training grants like those that NIH has recently provided in areas related to biomaterials. Any such grants should include requirements for additional courses as well as for a program of research. The panel believes that the effectiveness of such a grant program would be enhanced if institutions receiving grants were encouraged to strengthen their ties with government and industrial laboratories. For example, they could make arrangements for outside laboratories to provide summer jobs for their graduate students, and the participating government and industrial researchers could host visitor programs and serve as guest lecturers at the universities receiving the grants.
- A national Biomolecular Materials Institute (BMI) could be established, located at a university or a government laboratory or another site with an appropriate intellectual environment. Like options 1 and 2 above, this option is motivated by the panel's consensus that interdisciplinary collaboration requires special support and encouragement. For example, in the study of many aspects of biomolecular materials, such as those described above for molecular machines, close interaction between researchers is both difficult and very important. In addition, a national institute would broaden access to instruments and research facilities, facilitate contacts between the academic community and private industry, and enhance the visibility of the field in a way that would encourage the creation of university programs in biomolecular materials research and education.
A national BMI would act as an umbrella organization for the field. It would have four main tasks:
- To examine research directions through workshops, meetings, and studies, giving particular attention to proposed novel initiatives;
- To encourage interdisciplinary collaborations by bringing together scientists and engineers from different backgrounds, e.g., different disciplines or affiliations;
- To provide instrumental facilities that would encourage interactions between experimental groups; and
- To provide industry with a single contact point for obtaining information about biomolecular research activities and for obtaining assistance in making connections with those activities.
Structurally, the BMI might resemble the NSF-sponsored Institute for Theoretical Physics in Santa Barbara. For example, it would have quasi-independent status and be overseen by a broad-based advisory board. It would consist of a small cadre of permanent scientists, plus staff commensurate with the above-listed tasks, such as experts to assist visiting scientists in using the instruments and laboratories. Funding should if possible be provided in at least five-year increments, either by a single agency or preferably by a consortium of agencies such as NSF, NIH, the Department of Energy, and the Department of Defense. Funding should also include substantial industrial support if at all possible, probably at about the 25% level.
Although this option may be difficult to achieve in the current funding environment, the panel believes it is an important goal for the future.