3
How to Reduce Risk and the Uncertainty in Risk Estimates
Shielding
Recommendations for Research to Better Determine Shielding Requirements
When compared with concerns about other physiological issues and about vehicle reliability, there was initially minimal appreciation of the hazards of exposure to radiation in low Earth orbit. The lone exception involved the trapped radiation belts, which reached a maximum intensity in the South Atlantic Anomaly, but with judiciously chosen flight paths, even this exposure was kept to acceptable levels.
The relative risks posed by exposure to radiation will be substantially different for the establishment of a moon station or for a crewed mission to Mars. For instance, the protective effects of Earth's geomagnetic field will no longer apply, and consequently the spectra and abundance of particles composing the radiation source term will differ from that observed in low Earth orbit.
While minimal shield optimization was required in the initial low-Earth-orbit vehicles, optimization will and should be a major factor in the design of vehicles for prolonged solar system travel. Research areas that should be addressed are as follows:
- Characterization of the radiations in space and their uncertainties for galactic cosmic radiation and solar particle events (SPEs);
- Basic cross sections for primary particle interactions and formation of fragmentation products;
- Experimental validation of transport and fragmentation shielding computer modeling codes; and
- Complete reassessment of shielding materials and radiation risk models.
Knowledge Base Development
Current studies indicate that the particle distribution and energies that occur behind the various potential shield materials are critically dependent on the fragmentation and secondary particle production that occurs when spacecraft shielding is struck by radiation. Even cross sections for protons, which have been studied extensively both experimentally and theoretically in the most heavily supported computer modeling codes, show disagreements by a factor of 21 between the values calculated from models and measurements in energy regions for which there previously were no data. To reduce the uncertainties in any transport code (i.e., computer modeling programs used to calculate the transport of primary radiation and secondary particles through materials) being used for such calculations, precise measurements of interaction cross sections (relative probabilities) are required against which to benchmark the code.
The total amount of beam time purchased by NASA for research with ions heavier than protons is currently 100 hours per year. This includes the time not only for the physics experiments referred to above, but also for the biological irradiations and the dosimetry for biological experiments, which may take as much time as the biological irradiations themselves. This amount of beam time compares with about 400 hours per year previously available for similar research studies at the now-closed Berkeley BEVALAC. From the predicted cross sections for the secondary particles and the maximum count rates of the most sensitive detectors to detect these particles in the apparatus, it is possible to estimate typical time periods necessary to accumulate a sufficient number of counts at a specified beam rate, so that the random error in total counts is minimized. A reasonable estimate for measurements of secondary particle spectra is about 1 hour of beam on target for each data point, i.e., one incident particle type at one energy level for one target composition at one thickness. Each shield material would need to be tested with at least three particle types, not including protons. Each particle type would need to be accelerated to about five different levels of energy, and five shielding thicknesses should be tested at each energy. Including the time needed for setting up experiments and testing equipment (which may equal the time needed to accumulate data), the task group estimated that about 100 hours are needed for each shielding material examined with one particle type for data collected along the primary beam axis, with the beam time increasing geometrically with scattering angle. (A semipermanent or dedicated facility could drastically reduce set up times, since equipment may be left in place between experiments.) If collection of off-axis data is also considered, a conservative estimate of the time needed for obtaining data on particle types, numbers, and energies is about 300 hours for each particle for each shielding material, or about 900 hours for three materials. This amount of time would increase by a factor of 2 or more if data were collected at off-axis scattering angles and could correspond to about 1 or 2 equivalent chronological years of dedicated research at most DOE accelerators.
The composition of the GCR particle spectra dictates a focus on iron as the highest-atomic-number (Z) particle of critical interest. Studies with additional ions are required, however, to benchmark the theoretical cross section code against the Z range of interest. Because the uncertainty in the calculated cross sections is reflected in the uncertainty in the level of required shielding, reduction in the uncertainty of these data values will have major cost reduction implications.
Needed in addition to validation of the cross section calculation codes is validation of the transport code itself. Experimental measurements of particles emanating from a thick laminated shield need to be compared with theoretical calculations to benchmark the transport codes and reduce the uncertainty in the calculation of the amount of shielding needed. The laminate shields should be chosen carefully to reflect the full scope of materials that might be used; SiC, 6LiH, Al, regolith and other hydrogen-containing materials that show promise.
Shielding Approaches
As indicated above, radiation has not been considered a serious hazard in low-Earth-orbit missions of relatively short duration at low inclinations, given that the doses within spacecraft have been well within NCRP guidelines.2 For the mission to Mars, however, loss of the shielding benefits of Earth's magnetic field and the longer time periods over which dose is accumulated require that the shielding design be reevaluated. The concept of “just add a little more aluminum” is not a satisfactory solution.
Two options can be considered for shielding in space: active or passive shielding. The passive approach of bulk shielding was chosen for the NASA low-Earth-orbit missions and resulted in a reliable system at reasonable cost. An active system would require the use of very large magnetic field strengths to deflect charged particles away from the spacecraft. Minimizing energy requirements would entail the use of superconducting systems and would involve the associated complexity of such systems. Since cosmic radiation is essentially isotropic, a fully encompassing magnetic shield would be desirable but from a practical point of view would be very difficult to achieve. Thus, the construction of a satisfactory active shield is questionable. NASA has chosen to not pursue design of an active shielding system primarily because of doubts about its reliability and this report focuses on the requirements for a passive shielding system.
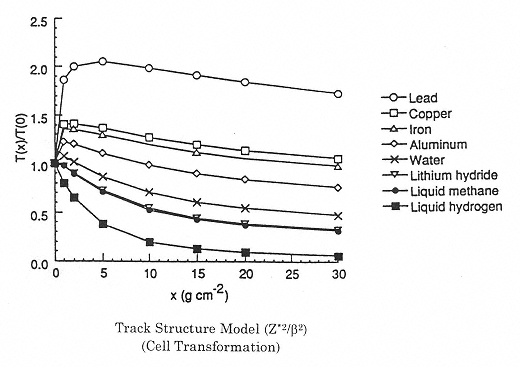
Figure 3.1
Calculated effect of shield material on cell transformation using the program NUCFRG2. Attenuation of cell transformation in one-year exposure behind several shield materials. T(x)/T(0) is the relative radiation-induced transformation as a function of shield thickness x. T(x) is the number of transformations at depth (x). T(0) is the number of transformations at the surface. SOURCE: Wilson, J.W., Kim, M., Schimmerling, W., Badavi, F.F., Thibeault, S.A., Cucinotta, F.A., Shinn, J.L., and Kiefer, R. 1995. Issues in space radiation protection: Galactic cosmic rays. Health Phys. 68:50–58. Reproduced from the journal Health Physics with permission from the Health Physics Society.
At a recent space shielding workshop, liquid hydrogen was identified as the optimum shield material.3 Accepting this fact, metal hydrides—i.e., lithium hydride—would appear to be prime candidates for consideration as potential shield materials.
Current calculations with the HZETRN/NUCFRG2, a transport code utilized by Langley Research Center investigators, indicate that the production of fragments and secondary particles is such that increasing the thickness of aluminum shielding has conflicting effects depending on the risk model in use. When evaluated by the dose-equivalent model (see Figure 2.5) or the model for transformation of cells in vitro (Figure 3.1), a substantial buildup in radiation damage occurs with increasing aluminum thickness. However, when evaluated using the conventional quality-factor LET model, a smaller increase is observed. When the results of the two models are compared using lithium hydride as the shield material, an increase in risk with increasing shield thickness is not predicted by either model. Clearly, the risk model chosen will have a substantial impact on material selection and the design of the spacecraft and thus must be determined with care and “frozen” early in the time line of the project.
Some data4 exist for questioning the use of the terrestrially developed quality factor as a function of the LET model (discussed in Chapter 1), currently incorporated in radiation safety standards, for evaluating the hazards of exposure to radiation in space. Of note is the variation in cross section for transformation with particles at a constant LET of about 18 keV/µm. A theory of doubly restricted stopping power has been proposed by Grusell5 that suggests such a variation but does not accurately predict the observed magnitude of the difference. Additional effort is needed to determine the viability of the doubly restricted stopping power theory. This work should be complemented by biological studies in a series of cell types to confirm the variation in biological damage with particle mass at a constant LET.
Cost of Research vs. Cost of Excess Shielding.
The process of designing a shielded environment for prolonged space travel is a difficult task even when given accurate known information on the space radiation source spectra and accurate nuclear and atomic reaction models. However, the current state of knowledge is such that accurate source spectra, interaction cross sections, fragmentation cross sections, and biological response functions to radiation exposure are not available. Much of this information can be obtained through a focused, primarily ground-based, research program. The costs of such a program, in time and money, have to be compared with the costs of using currently incomplete and inaccurate databases and radiation shielding models and then allowing for excess shielding in the spacecraft design to compensate for the maximum uncertainty associated with the poorly known parameters. Using the Apollo mission experience as a guide, Wilson has estimated that at an overall uncertainty factor of 3 for the risk posed by exposure to HZE particles, the increased costs of compensating for that uncertainty by using excess shielding in the spacecraft for the Mars mission are about $10 billion; at an overall uncertainty factor of 6, the value is close to $30 billion. 6 These figures far exceed NASA's annual research budget for particle physics and biology research of approximately $4.5 million per year. 7
Accepting increased costs as being associated with increased uncertainty, the question then arises: What are the sources of uncertainty in the current shielding design information? By one estimate the uncertainty factor for HZE ion transport is 2 to 38 and for biological response to HZE particles is 5 to 10,9 corresponding to an overall uncertainty factor as large as 10 to 30. The disparity between the cost of the excess shielding required to compensate for these large uncertainties, and current NASA expenditures cited above, indicate the need for a significant increase in the research budget for space radiation physics and biology if NASA intends to pursue deep-space missions with human crews. This would be true even if the research determined that the actual risks were somewhat higher than currently estimated. The task group concluded that the savings to be obtained by reducing the current level of uncertainty in estimating the risks of exposure to radiation in space far outweigh the cost of obtaining the necessary information through an enhanced ground-based NASA research effort.
Radioprotective and Chemoprotective Drugs and Diet
It is well known that nausea and vomiting are common after exposure to moderate to high doses of radiation, and the use of antiemetic agents is a clinically accepted practice in radiation medicine. However, it is unlikely that radiation-induced nausea will be a major concern for the Mars mission. Rather, the development of nausea is likely to be a concern only in the case of a crew member working outside a vehicle during an SPE. In anticipation of such an occurrence, an antiemetic might be taken as a routine precaution prior to participation in any extravehicular activity.
The development of drugs that can be used to protect personnel from adverse health effects of radiation is one possible approach to reducing acute effects following exposure to radiation from SPEs that might be encountered outside the spacecraft. This is the only scenario for which strategies employing radioprotective agents are warranted, as day-to-day exposure inside a spacecraft is unlikely to be sufficient to result in such acute effects. A large battery of radioprotective compounds developed as part of a program at the Walter Reed Army Institute of Research includes WR-2721, a compound that has been shown in experimental studies to be effective in reducing a number of deterministic effects associated with exposure to radiation.10 Considering the potential risks of such effects associated with exposure to radiation during SPEs, the availability during a mission of such radioprotective compounds would be highly desirable. Unfortunately, WR-2721 requires the administration of an oral dose that produces side effects not desirable during spaceflight. These include vomiting and vasodilatation, which results in hypotension. Other compounds need to be examined to determine their efficacy as radioprotective agents at doses that have fewer toxic side effects.
More recently, a series of studies by Grdina and his colleagues have suggested that low doses of WR-2721 (which are substantially below toxic levels) and its active metabolite, WR-1065, given up to 3 hours after exposure can reduce the mutagenic and carcinogenic effects of radiation by means of a mechanism independent of its protective effects against cytotoxicity.11–13 It is not known whether this compound would be effective in preventing effects produced by protons and heavy ions; its proposed mechanism of action suggests that its effects might be applicable for both high-and low-LET radiation. Therefore, it is quite
possible that this drug would be useful following exposure to radiation encountered in space as well. Unfortunately, daily use of this compound is not possible since multiple low doses eventually result in toxic effects similar to those seen with higher single doses. This makes the use of such a compound inappropriate for modifying the effects of daily doses of radiation encountered during spaceflight. On the other hand, the use of this drug or similar drugs on a short-term basis following exposure to radiation associated with SPEs might be reasonably considered. Clearly, more research is required before this strategy could be incorporated into an overall radiation protection program.
Diet is known to be an important influence on cancer development. A full discussion of dietary factors and cancer is beyond the scope of this report and the expertise of this task group. However, it is recommended, in planning a mission to Mars, that careful consideration be given to the inclusion of dietary supplements, including vitamins and antioxidants that have been shown to have the potential for modifying oxidative damage and for reducing the lifetime risk of contracting cancer.
Crew Selection
The Chapter 2 sections titled “Variation in Susceptibility to Radiation Across Subject Types” and “DNA Repair” provide examples of genotypes that could predispose individuals to adverse health effects from exposure to ionizing radiation. In general, the genotypes that produce large increases in sensitivity and/or susceptibility are rare in the population and frequently are phenotypically obvious. The probability of an individual with such a genotype being enrolled in a crewed spaceflight program is very low. Thus, the normal process for crew selection should generally be adequate for screening unusually susceptible individuals. However, as noted above, there is the potential for some genotypes (particularly heterozygous ones, such as that for AT) to confer a small increase in sensitivity to radiation for cell killing, induction of mutations, and even cancer. At this time it is either not possible or not feasible to type individuals genetically for the purpose of identifying the status of a range of “sensitivity genes.” Many of the genes have not been identified or have not been characterized; if they have been cloned, they are generally too large for routine individual characterization. If the G2 radiosensitivity assay of Jones et al.14 turns out to be able to identify a range of sensitive individuals who are cancer prone, it might be feasible to use this method as a screening assay to at least allow the range of sensitivities to be incorporated into a risk estimate or to guide investigators to estimate dose limits for individuals with different sensitivities.
Optimal Time for Flight
Because of the long period of the solar cycle (typically 11 years), it may be possible with a judicious choice of starting date to significantly reduce the radiation dose equivalent received by space crew members. During the solar maximum, there are increased numbers of SPEs with a concurrent reduction in fluence rate for the GCR, in comparison with the rate during solar minimum. During the solar minimum, there is a significant decrease in the probability of solar events and a corresponding increase in GCR fluence rates of particles in some energy ranges by as much as a factor of 10. Based on previous studies, it is the general consensus of the task group that the optimal time for a planetary mission is probably during solar minimum, despite the increased total fluence and dose equivalent associated with the increased galactic fluence.15 This consensus is in part based on the total estimated dose equivalents for each flight scenario, but also is based on the variability of the doses expected from solar events. Moreover, the potential for acute effects arising from exposure at high levels is greater from solar events, although such large exposures are unlikely even during solar maximum except for specific cases such as a large SPE exposing personnel engaged in extravehicular activities.
The optimal launch date would still appear to be one that takes full advantage of the solar minimum, but this conclusion is partially dependent on RBE values for the primary galactic HZE particles relative to those for primary photons, the materials chosen for spacecraft construction and shielding, and the distribution of secondary particles arising from the HZE particles and protons traversing these materials. The task group concluded that the choice of a launch date should be reexamined periodically as better information is acquired.
Solar Particle Event Warning System
Flares
Clearly the most prominent signal we have of a flare occurrence is the bursts of electromagnetic radiation that originate in the flare event and travel in a straight-line path to Earth, thus reaching Earth in about 8 minutes, compared with flare-generated solar energetic particles (SPEs), which can arrive as soon as 18 minutes after the flare.
Our ability to predict the occurrence of SPEs due to flares is imprecise at best, and the accuracy of the prediction varies inversely with the length of the advance warning desired. In addition, the probability of flare SPEs reaching Earth depends on the location of the flare on the sun's surface. The magnetic field line that connects Earth to the sun has its origin on the sun at location W55. Thus the closer the flare event is to this location, the shorter the time it takes the solar flare particles to reach Earth. With increasing distances from location W55 on the sun, the protons take longer to reach Earth or may not be connected by magnetic field lines to Earth; thus such SPEs would not be observed on Earth.
Coronal Mass Ejections/Interplanetary Shocks.
Coronal mass ejections are large eruptions of coronal material that produce interplanetary shocks when they move at high speeds from the sun through the solar wind. These shocks produce SPEs by accelerating a small fraction of the solar wind particles during their passage through interplanetary space. It is now appreciated that not all coronal ejections that produce SPEs have related flares, and the contribution of nonflare events to the SPE population has only recently been appreciated. Such events are detectable in soft x-ray images obtainable above Earth's absorbing atmosphere. However, it is not yet possible to predict from such images whether the disturbance launched toward Earth is fast enough to produce a shock and thus SPEs.
Conclusions
Assuming the goal of allowing time for crew members, in space or on another planetary body, to reach shielded locations, current monitoring systems using visual observations for predicting SPEs are not acceptable. The fact that a space vehicle during its travel may be connected to a flare on the sun by magnetic field lines that originate on the far side creates a blind spot for Earth-based monitoring stations. A series of satellite platforms for monitoring the sun's activity could keep the sun's surface and corona under continual surveillance, but such a system is complex and costly. Thus there is a need for new and innovative ideas in this area. The spacecraft itself may be equipped with the necessary instrumentation to allow crew members to participate in the monitoring process. However, this system would, like Earth-based stations, have blind spots and should be viewed as a complement to any series of space platform monitoring stations.
The required advance warning time will be dictated by the mission program. Under the assumption that crew members will return to a base camp on Mars for rest at the end of each day, an advance warning of 8 hours would be desirable. Shorter warning periods may curtail the scope of a mission if the level of safety is to be maintained.
The current state of the science is that Earth-based monitoring stations can only partially support a space vehicle in predicting the occurrence of SPEs at the vehicle. A system that monitors the global surface of the sun and corona should be developed and knowledge improved on how to interpret information acquired by monitoring the sun. Research is necessary to provide an understanding of the basic mechanisms that trigger solar events, the precursor signals that can be detected from terrestrial or satellite observation, and the ability to determine the location of flare occurrence.
References
1. Dicello, J.F., Schillaci, M.E., and Liu, L. 1990. Cross sections for pion, neutron, proton, and heavy-ion production from 800-MeV protons incident upon aluminum and silicon. Nucl. Instrum. Methods B45: 135–138.
2. National Council on Radiation Protection and Measurements (NCRP). 1989. Guidance on Radiation Received in Space Activities. Recommendations of the National Council on Radiation Protection and Measurements. NCRP Report No. 98. National Council on Radiation Protection and Measurements, Bethesda, Md.
3. Wilson, J.W., Cucinotta, F.A., Thibeault, S.A., Kim, M.H., Shinn, J.L., and Badavi, F.F. 1997. Radiation Shielding Design Issues. Chapter 7 in: Shielding Strategies for Human Space Exploration: A Workshop (John W. Wilson, Jack Miller, and Andrei Konradi, eds.). NASA, Washington, D.C., forthcoming.
4. International Commission on Radiological Protection (ICRP). 1991. 1990 Recommendations of the International Commission on Radiological Protection. ICRP Publication 60. Annals of the ICRP 21. Pergamon Press, Elmsford, N.Y.
5. Grusell, Erik. 1996. Doubly restricted stopping powers. Physics Med. Biol. (submitted for publication).
6. Wilson, J.W., Cucinotta, F.A., Shinn, J.L., Kim, M.H., and Badavi, F.F. 1997. Shielding Strategies for Human Space Exploration: Introduction. Chapter 1 in: Shielding Strategies for Human Space Exploration: A Workshop (John W. Wilson, Jack Miller, and Andrei Konradi, eds.). NASA, Washington, D.C., forthcoming.
7. Schimmerling, Walter. Presentation to workshop on Shielding Strategies for Human Space Exploration, Dec. 6–8, 1995, NASA Johnson Space Center, Houston, Texas.
8. Wilson, J.W., Kim, M., Schimmerling, W., Badavi, F.F., Thibeault, S.A., Cucinotta, F.A., Shinn, J.L., and Kiefer, R. 1995. Issues in space radiation protection: Galactic cosmic rays. Health Phys. 68: 50–58.
9. Wilson et al., 1997, Shielding Strategies for Human Space Exploration.
10. NCRP, 1989, Guidance on Radiation Received in Space Activities.
11. Hill, C.K., Nagy, B., Peraino, C., and Grdina, D.J. 1986. 2[(aminopropyl)amino]ethanethiol (WR-1065) is anti-neoplastic and anti-mutagenic when given during 60Co gamma-ray irradiation. Carcinogenesis 7: 665–668.
12. Grdina, D.J., Kataoka, Y., Basic, I., and Perrin, J. 1992. The radioprotector WR-2721 reduces neutron-induced mutations at the hypoxanthine-guanine phosphoribosyl transferase locus in mouse splenocytes when administered prior to or following irradiation. Carcinogenesis 13: 811–814.
13. Grdina, D.J., Shigematsu, N., Dale, P., Newton, G.C., Aguilera, J.A., and Fahey, R.C. 1995. Thiol and disulfide metabolites of the radiation protector and potential chemopreventive agent WR-2721 are linked to both its anti-cytotoxic and anti-mutagenic mechanisms of action. Carcinogenesis 16: 767–774.
14. Jones, L.A., Scott, D., Cowan, R., and Roberts, S.A. 1995. Abnormal radiosensitivity of lymphocyte from breast cancer patients with excessive normal tissue damage after radiotherapy: Chromosome aberrations after low dose-rate irradiation . Int. J. Radiat. Biol. 67: 519–528.
15. National Council on Radiation Protection and Measurements (NCRP). 1993. Limitation of Exposure to Ionizing Radiation. NCRP Report No. 113. National Council on Radiation Protection and Measurements, Bethesda, Md.