3
Evolution and the Nature of Science
Science is a particular way of knowing about the world. In science, explanations are restricted to those that can be inferred from confirmable data—the results obtained through observations and experiments that can be substantiated by other scientists. Anything that can be observed or measured is amenable to scientific investigation. Explanations that cannot be based on empirical evidence are not a part of science.
The history of life on earth is a fascinating subject that can be studied through observations made today, and these observations have led to compelling accounts of how organisms have changed over time. The best available evidence suggests that life on earth began more than three and a half billion years ago. For more than two billion years after that, life was housed in the bodies of many kinds of tiny, single-celled organisms, some of which produced the oxygen that now makes up more than a fifth of the earth's atmosphere. Less than a billion years ago, much more complex organisms appeared. By about half a billion years ago, evolution had resulted in a wide variety of multicellular animals and plants living in the sea that are the clear ancestors of many of the major types of organisms that continue to live to this day. Somewhat more than 400 million years ago, some marine plants and animals began one of the greatest of all innovations in evolution—they invaded dry land. For our own phylum, the Chordata, this move away from the nurturing sea led to the appearance of amphibians, reptiles, birds, and mammals—the latter including, of course, our own species, Homo sapiens.
This chapter looks at how science works in the context of our overall understanding of how biological evolution occurred. It begins, however, by discussing another scientific development that challenged long-held understandings and beliefs: the discovery of heliocentricism.
Heliocentricism and the Nature of Science
Surely one of the first major natural phenomena to be understood was the cause of night and day. Some of the earliest surviving human records left on clay tablets relate to the movements of the sun and other celestial bodies. The obvious cause of day and night is the rising and setting of the sun. This is an observation that can be made today by anyone and, seemingly, requires no further explanation.
Archaeological evidence and early records make it clear that our ancestors realized that not only does the sun appear to rise and set, but so do the moon and stars. The movements of the moon and stars, however, are not precisely synchronized with
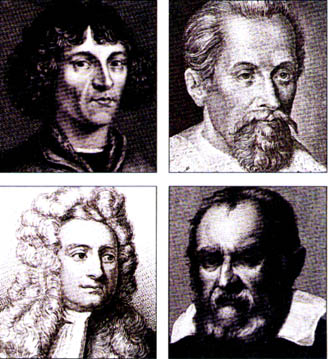
Clockwise from top left, Nicolaus Copernicus (1473-1543), Johannes Kepler (1571-1630), Galileo Galilei (1564-1642), and Isaac Newton (1642-1727) led the way to a new understanding of the relationship between the earth and the sun and initiated an age of scientific progress that continues today.
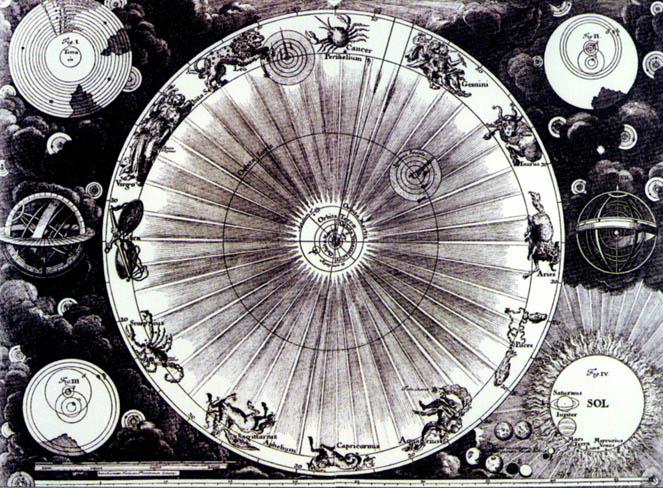
Illustration from the 18th century depicts the Ptolemaic system in the upper left corner and the Copernican system in other corners and center.
those of the sun. The moon is slower by about one hour per day. The stars remain almost the same on successive nights, but slowly it becomes obvious that they, too, are slowed in their movements compared to the sun. Thus, the stars of summer are different from those visible in the winter. In fact, it takes a full year for the stars to return to their previous position, an interval of time that defines our year.
The ancient observers realized that not all stars move in unison. Although most move in majestic unity, a few others are "wanderers"—appearing now with one group of stars and a week later somewhere else. The majority were called "fixed stars," the wanderers were called "planets."
During the late Middle Ages, and especially in the Renaissance, beautiful brass models known as orreries were made to show the relative positions and movements of the sun, planets, and moon as they circled the earth. As the center of the universe, the earth was a sphere in the center of the orrery. The other celestial bodies were positioned on rings of metal, each moving by clockwork at its own rate. The fixed stars required a simple solution—they could be considered stuck in an outermost shell, also moved by clockwork.
The problem with orreries—and with the theories of the cosmos then prevailing—was that they had to become successively more complex as more became known. Careful observations of the movements of the stars and planets greatly complicated the hypotheses used to account for those movements. This growing complexity stimulated some of the leading astronomers of the 16th and 17th centuries, including Copernicus, Kepler, and Galileo, to make even more precise observations of the movements of the heavenly bodies. Astronomers used these measurements to demonstrate that the age-old human explanations of the heavens were incomplete. In the process, they replaced a complex and confusing explanation with a simple one: the sun, rather than the earth, is at the center of a "solar system," and the earth revolves around it. That simple step—a bold departure from past thinking due mainly to the insights of Copernicus (1473 to 1543)—dramatically changed the picture of the then known universe.
This concept of heliocentricism initially ran counter to the positions of religious authorities. The view of Christianity over most of its history, based on a literal interpretation of the Bible, was that the earth is the center of the universe around which the celestial bodies revolve. Copernicus dedicated his book describing the theory of heliocentricism, De revolutionibus orbium coelestium, to Pope Paul III and promptly died. That saved him the troubles that were to beset Galileo (1564 to 1642), whose astronomical observations confirmed the views of Copernicus. Galileo was told to abandon his beliefs, and he later was tried by the Inquisition and sentenced to the equivalent of house arrest. The Church held that his views were dangerous to faith.
Continued study and ever more careful measurements of the movements of the planets and sun continued to support the heliocentric hypothesis. Then, in the latter half of the 17th century, Isaac Newton (1642 to 1727) showed that the force of gravity—as measured on the earth—could account for the movements of the planets given the laws of motion that Newton derived. As a result of the steady accumulation of evidence, the theological interpretation of celestial movements gave way to the naturalistic explanation, and it is now accepted that night and day are the consequences of the rotation of the earth on its axis. Today, we can see for ourselves the rotation of the earth from satellites orbiting the planet.
Like biological evolution, the theory of heliocentricism brought order and new understanding to an otherwise chaotic and confusing aspect of nature. It also had great practical applications, in that the exploration of the world by European seafarers used the more accurate understanding of celestial mechanics to assist in navigation.
Looking at the night sky remains a powerful experience. But that experience is now informed not only by the beauty and majesty of the heavens, but by a deeper understanding of nature and by an appreciation of the power of the human intellect.
This triumph of the human mind says a great deal about the nature of science. First, science is not the same as common sense. Common sense indicates that the sun does rise and set. Nevertheless, there can be other explanations of that phenomenon, and one of them, the rotation of the earth on its axis, is responsible for day and night. A concept based on observation proved to need extensive modification as new observations accumulated.
Second, the statements of science should never be accepted as "final truth." Instead, over time they generally form a sequence of increasingly more accurate statements. Nevertheless, in the case of heliocentricism as in evolution, the data are so convincing that the accuracy of the theory is no longer questioned in science.
Third, scientific progress depends on individuals, but the contributions of one individual could be made by others. If Copernicus had kept his ideas to himself, the discovery of heliocentricism would have been postponed, but it would not have been blocked, since other astronomers eventually would have come to the same conclusion.
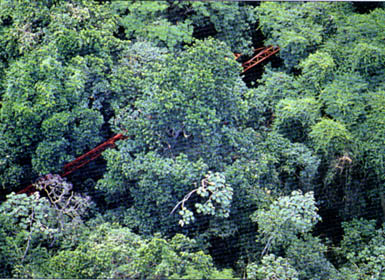
Biologists have used construction cranes to study the many newly discovered species that live in the canopies of tropical forests, as in this research project in Panama.
Similarly, had Darwin and Wallace not published their hypotheses, the concept of biological evolution would nevertheless have emerged as the accepted explanation for the history of life on earth. The same cannot be said in other areas of human endeavor; for example, had Shakespeare never published, we would most assuredly never have had his plays. The publications of scientists, unlike those of playwrights, are a means to an end—they are not the end itself.
Science Requires Careful Description
What are the scientific methods that have led to our current understanding of the history of life over vast eons of time? They begin with careful descriptions of the material being studied.
The material for the study of biological evolution is life itself. One basic aspect of life is that individuals can be grouped as similar kinds, or species. Another important observation is that many species seem to be closely related to each other. The scientific classification of species and their arrangement into groups began with the publication in 1758 of Systema Naturae, or system of nature, by the Swedish naturalist Carolus Linnaeus (1707 to 1778). For example, Linnaeus knew seven dog-like species, and he gave each a double name. Subsequently many more species were discovered and some of the names were changed—and continue to be changed as more information is obtained. The domestic dog is Canis familiaris; the coyote of North America is Canis latrans ; the Australian dingo is Canis dingo; and the wolf of the northern hemisphere is Canis lupus. Thus Canis is the name of the genus of dog-like animals, and the distinctive second name is the species name.
Generations of scientists have discovered new species, described them, and
arranged them into the system first suggested by Linnaeus. Whereas Linnaeus recognized about 9,000 species, systematists now have recognized about 1.5 million. The task of categorizing and describing species is still far from complete. Most species of smaller invertebrates, and many bacteria and other microscopic organisms, remain to be discovered. The plant kingdom is also incompletely known. Though the flowering plants of many areas, such as Europe and North America, are fairly well described, many other regions have not been nearly as well explored by botanists.
Recent investigations in the exceptionally diverse rainforests of South America have caused biologists to raise their estimates of the number of undescribed species. For example, a very high proportion of insects collected from the forest canopy are "new" species to science. It is now believed that the number of different species of plants and animals in the world may be ten million, or even more.
The scientific methods used in classifying organisms have been greatly improved over time. The process begins with the intensive field work in which the animals, plants, and microorganisms are collected and carefully examined. Most will be known to a specialist, but there might be some unusual examples. However, none is likely to be a complete stranger, since the specialist will probably recognize that any puzzling specimen is similar to some familiar species. Next the specialist must check all that has been published on the group of organisms that contains the similar species. If, after an exhaustive search, there is no record of a described species that corresponds to the one being examined, the specimen is probably a new species. The specialist will then prepare a careful description of the new species and publish it in a scientific journal. There is a permanent reward for being the describer of a new species: thereafter monographs that deal with the classification of the group to which the new species belongs will add the describer's name at the end of the scientific
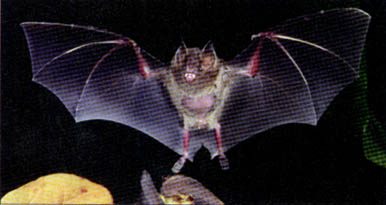
Despite their similarities with birds, bats are mammals that evolved from flightless ancestors.
name. Thus, for example, "Homo sapiens Linnaeus" is our own proper identification, because Linnaeus was the first to give us our scientific name.
This example makes it clear that not all scientific data are derived as the result of experimentation. The conventional classification of species into seemingly natural groups involved the careful observation of a variety of different species, followed by the use of selected characteristics in an attempt to define groups of species thought to be related. But the groupings are not always obvious. For example, it might have seemed reasonable to classify bats with birds, since the most conspicuous characteristic of each is the ability to fly. But bats are mammals. Like all mammals, their bodies are covered with hair and their young are born alive (instead of hatching from eggs) and are nourished by milk from the mother's mammary glands.
Although most of the species we know today were described after the time of Linnaeus, we continue to use his basic system of hierarchical classification. For example, similar genera are united in families, similar families in orders, similar orders in classes, and similar classes in phyla. The dog-like species listed above (the genus Canis ), plus a number of similar but more distant dog-like animals, are grouped as the family Canidae. This family plus the families of cats, bears, seals, and weasels form the order Carnivora. The carnivores and all other animals with hair are combined as the class Mammalia. Mammals are combined with the birds,
reptiles, amphibians, fishes, plus some small marine animals in the phylum Chordata. Today, many systematists group organisms according to a system known as cladistics. By determining which traits of a species evolved earlier and which evolved later, this system seeks to classify organisms according to their evolutionary history.
Science as Explanation
In the quest for understanding, science involves a great deal of careful observation that eventually produces an elaborate written description of the natural world. This description is communicated to scientists in scientific journals or at scientific meetings, so that others can build on pre-existing work. In this way, the accuracy and sophistication of the description tends to increase with time, as subsequent generations of scientists correct and extend the observations
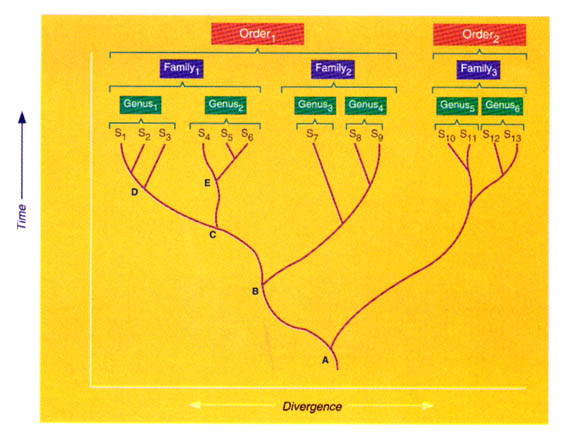
Evolutionary relationships often are depicted in diagrams that resemble the branches of trees. Closely related species (denoted S1, S2, etc.) are grouped into genera, genera into families, and so on. The result is a hierarchical diagram showing how different species evolved from common ancestor species (represented in this diagram by the letters A through E).
of their predecessors. Because the total sum of scientific knowledge increases relentlessly, scientific progress is something that all scientists take for granted.
But science is not just description. Even as observations are being made, the human mind attempts to sort, or organize, the observations in a way that reveals some underlying order in the objects or phenomena being observed. This sorting process, which involves a great deal of trial and error, seems to be driven by a fundamental human urge to make sense of our world.
The sorting process also suggests new observations that might otherwise not be made. For example, the suggestion that bats should be grouped with mammals led to an intensified examination of the similarities between bats and rodents—first at the anatomical level, and later with respect to the genes and protein molecules that form their cells. In this case, new evidence was obtained that confirmed the suggested relationship. In other cases, the further observations inspired by a tentative grouping have caused the rejection of a new idea.
The realization that species can be arranged in a hierarchy of groups of seemingly similar forms raised an obvious question: What accounts for the relatedness of different groups of organisms? The mechanism that was proposed by Darwin directly addressed this question. It suggested that all animals classified as belonging to the same group had a common ancestor species. That is, dogs, wolves, coyotes and all members of the genus Canis are descended from a common ancestor species that lived in the remote past. In a similar manner all species in a family, an order, a class, or a phylum share a common inheritance.
How could one possibly test such a hypothesis? In the decades before Darwin proposed his hypothesis, geologists realized that the sedimentary rocks of the earth's crust contain a running diary of earth's history. This record of past events comes about because the earth's crust is in a constant state of change. This observation might not be obvious in the lifetime of an individual, but it is dramatic over thousands of years. Relatively flat surfaces are uplifted to form mountains, and then the mountains slowly erode to form flatlands. Storms produce powerful waves that erode cliffs at the seashore. These phenomena have the common feature of moving solid materials, and the subsequent settling out of these materials makes possible the formation of a special form of rock that contains a record of the earth's past.
Consider the case of a river with a source in the mountains. As the water moves downstream, it erodes the slopes of the mountains. Tiny grains produced by the erosion, called silt, are relatively easy to move. When the river reaches the flatlands, a lake, or the ocean, the solid material being carried by the water is deposited—often reaching great thicknesses over long periods of time. Then the pressure of the sediments on top can cause the sediments beneath to harden into "sedimentary rocks."
The river may carry things other than
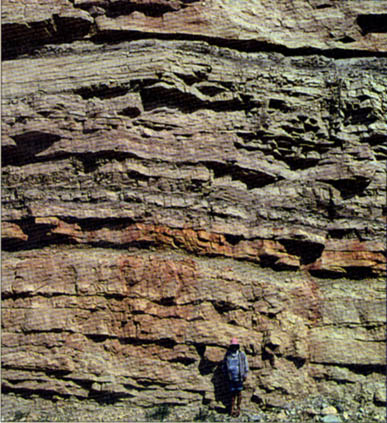
Sedimentary rocks are formed when solid materials carried by wind or water accumulate in layers and then are compressed by overlying deposits. Sedimentary rocks sometimes contain fossils formed from the parts of organisms deposited along with other solid materials.
silt, sand, and rocks. Hard structures of organisms such as the bones and teeth of animals may be carried along as well. These, too, will be deposited with the silt, sand, and rocks. Under certain circumstances, these remains of organisms undergo a chemical change in which the original material is replaced by molecules that form stone. In this way, the organic remains of living things are fossilized (changed into stone), creating the evidence of ancient life studied by scientists.
Because of the order in which the sediments are deposited, the most recent layer of rocks normally will be on top and the oldest layer will be on the bottom (though sometimes sediments are flipped upside down by the geologic folding of rock layers). Also, the fossils in each layer usually will be of those organisms that lived at the time the layer was formed. Thus, the fossils in the lower layers will represent species that lived earlier than those found in the upper layers.
The relative position of fossils tells only which are older and which younger. One
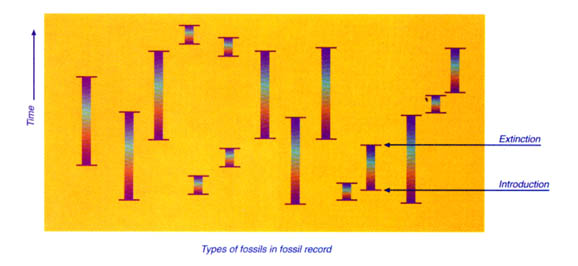
In the fossil record, most species are characterized by a specific appearance, a duration over time, and extinction. The evolutionary origins of species are inferred from the morphological relations among fossils.
can estimate the difference in the ages of the two fossils by noting the thickness of the rock that separates them. If the difference is only one foot, one might guess the interval of time is less than if two fossils are separated by 50 feet of rock layers. Today, however, far more accurate methods of dating fossils are available, as described on the next page. Because these methods are based on the known rates of radioactive decay, they provide valuable measures of absolute time.
The scientific study of fossils is called paleontology, and the methods used for their identification and classification are similar to those used for living species. But in some respects the task of the paleontologist is far more difficult. Many species lack hard parts such as bones and shells, and such organisms almost always decay without becoming fossilized. This is the case for many groups of soft-bodied invertebrates—such as worms of many kinds, jellyfish, and protozoans. Even for such species as mammals, birds, reptiles, and amphibians, death is usually followed by the skeleton being dismembered and the bones scattered. For this reason, whereas isolated bones are often fossilized, it is exceptionally rare for an intact skeleton to be found.
Tiny fossils first reveal the existence of bacteria 3.5 to 3.8 billion years ago, and animals composed of more than a single cell are known from about 670 million years ago. But the organisms that lived between these two dates lacked hard parts and, hence, were rarely preserved as fossils. Then, about 570 million years ago, a dramatic change took place. At the beginning of the Cambrian period, animals evolved that had calcified shells and other types of body coverings that had a far better chance of becoming fossilized. These fossils demonstrate that Cambrian seas were populated with a variety of invertebrates. The earliest vertebrate fossils date from about 500 million years ago. Thereafter early amphibians and reptiles appeared. Birds and mammals appear in the fossil record only about 200 million years ago, while dinosaurs first appear about 225 million years ago and disappear suddenly about 160 million years later.
In the 1830s, when Darwin began his studies, the essential features of the fossil record were known (although absolute dates had not yet been determined). Many thousands of living species had been described, and it was clearly recognized that they could be organized into various groups—suggesting that they are somehow relatives. In addition, analysis of the fossil record revealed that the organisms on the ancient earth had undergone major changes over time—with whole groups of animals appearing, persisting for long periods of time, and then disappearing.
Darwin was an unusually keen observer. But he was not content to catalogue facts
and observations. Instead, the natural world to him was a gigantic, very challenging puzzle that demanded an explanation for its otherwise bewildering complexity. Why are different organisms so similar? Why has there been a succession of different kinds of species throughout geologic time?
Certain observations seemed particularly important. For example:
-
In South America, the only continent where living armadillos were found, Darwin discovered fossil evidence for the prior existence of ancient species that had many of the unique features of living armadillos, yet were clearly different. Such fossils were found nowhere else in the world. Why were both living and ancient armadillo-like species confined to the same geographical region?
-
On the Galapagos Islands, 600 miles off the coast of Ecuador, Darwin observed many distinct living species of birds and reptiles that closely resembled each other—yet were different on each tiny island. Why, for example, should the beak size of the
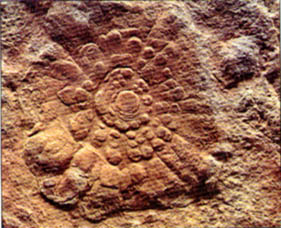
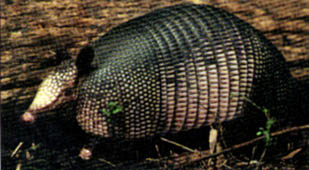
In South American, Darwin found fossil species that were clearly related to modern armadillos, yet neither the fossils nor the living animals were found anywhere else in the world. In The Origin of Species, he explained that ''the inhabitants of each quarter of the world will obviously tend to leave in that quarter closely allied though modified descendants."
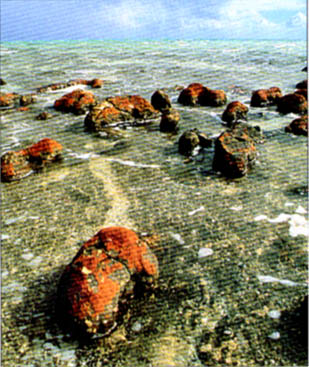
Before the start of the Cambrian period about 550 million years ago, multicellular organisms lacked hard parts like shells and bones and rarely left fossils. However, a few pre-Cambrian organisms left traces of their existence. Some ancient rocks contain stromatolites—the remnants of bacteria that grew in columns like stacked pancakes (right). top, a fossil just predating the Cambrian shows the outlines of a marine invertebrate that might have resembled a jellyfish.
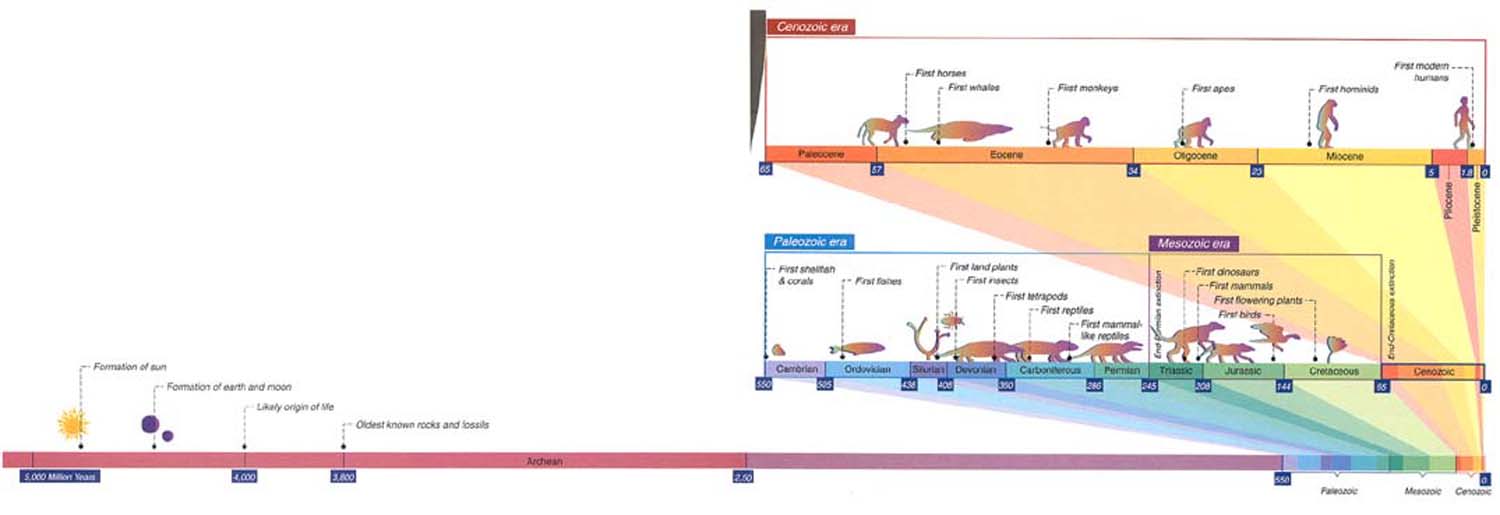
A timeline of evolution demonstrates the tremendous expanse of geologic time compared to the period since humans evolved. Each higher scale details part of the scale beneath it. While the estimated times of various evolutionary events continue to change as new fossils are discovered and dating methods are refined, the overall sequence demonstrates both the scope and grandeur of evolutionary change.
-
mockingbirds on one island be different from that of a closely related mockingbird on an island only 30 miles away? And why were the various types of animals on these islands related, but distinct from, the animals in Ecuador, whereas those on the otherwise very similar islands off the coast of Africa were related to the animals in Africa instead?
Darwin could not see how these observations could be explained by the prevailing view of his time: that each species had been independently created, with the species that were best suited to each location on the earth being created at each particular site. It looked instead as though species could evolve from one into another over time, with each being confined to the particular geographical region where its ancestors happened to be—particularly if isolated by major barriers to migration, such as vast expanses of ocean.
But how could one species turn into another over the course of time? In constructing his hypothesis of how this occurred, Darwin was struck by several other observations that he and others before him had made.
-
People who bred domesticated animals and plants for commercial or recreational use had found and exploited a great deal of variation among the progeny of their crosses. Pigeon breeders, for example, had observed wide differences in colors, beaks, necks, feet, and tails of the offspring from a single mating pair. They routinely enhanced their stocks for desired traits—for example, selectively breeding those animals that shared a particular type of beak. Through such artificial selection, pigeon fanciers had been able to create many different-looking pigeons, known as breeds. A similar type of artificial selection
-
for mating pairs of dogs had likewise created the whole variety of shapes and sizes of these common pets—ranging from a Great Dane to a dachshund.
-
Animals living in the wild can face a tremendous struggle for survival. For some birds, for example, fewer than one in 100 animals born in one year will survive over a harsh winter into a second year. Those with characteristics best suited for a particular environment—for example, those individual birds who are best able to find scarce food in the winter while avoiding becoming food for a larger animal—tend to have better chances of surviving. Darwin called this process natural selection to distinguish it from the artificial selection used by dog and pigeon breeders to determine which animals to mate to produce offspring.
At least 20 years elapsed between the time that Darwin conceived of descent with modification and 1859, the year that he revealed his ideas to the world in On the Origin of Species. Throughout these 20 years, Darwin did what scientists today do: he tested his ideas of how things work with new observations and experiments. In part, he did this by thinking up every possible objection he could to his own hypothesis.
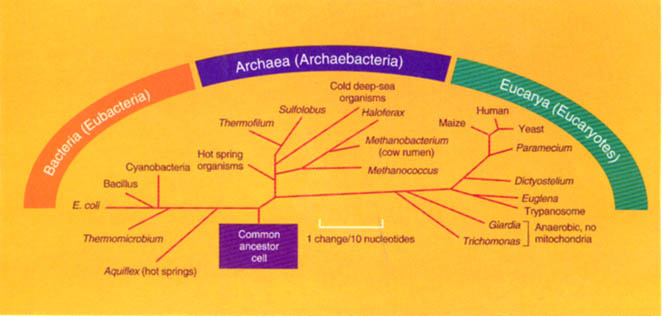
The ability to analyze individual biological molecules has added great detail to biologists' understanding of the tree of life. For example, molecular analyses indicate that all living things fall into three domains—the Bacteria, Archaea, and Eucarya—related by descent from a common ancestor.
For each such argument, Darwin tried to find an observation made by others, make an observation, or do an experiment of his own that might imply that his ideas were in fact not valid. When he could successfully counter such objections, he strengthened his theory. For example, Darwin's ideas readily explained why distant oceanic islands were generally devoid of terrestrial mammals, except for flying bats. But how could the land snails, so common on such islands, have traversed the hundreds of miles of open ocean that separate the islands from the mainland where the snails first evolved? By floating snails on salt-water for prolonged periods, Darwin convinced himself that, on rare occasions in the past, snails might in fact have "floated in chunks of drifted timber across moderately wide arms of the sea."
This example shows how a hypothesis can drive a scientist to do experiments that would otherwise not be done. Prior to Darwin, the existence of land snails and bats, but not typical terrestrial mammals, on the oceanic islands was simply noted and catalogued as a fact. It is unlikely that anyone would have thought to test the snails for their ability to survive for prolonged periods in salt water. Even if they had, such an experiment would have had little meaning or impact.
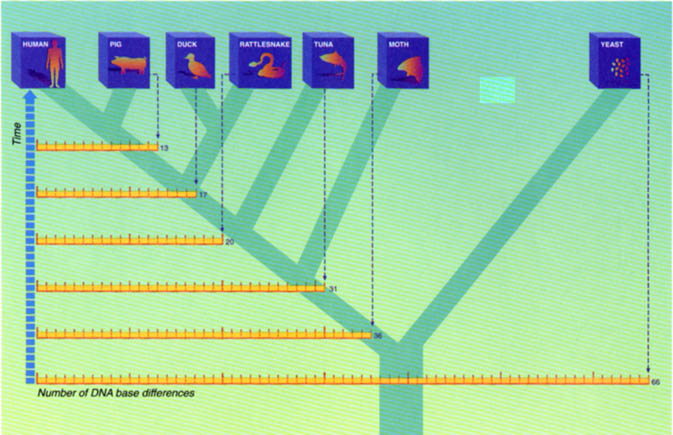
Organisms ranging from yeast to humans use an enzyme known as cytochrome C to produce high-energy molecules as part of their metabolism. The gene that codes for cytochrome C gradually has changed over the course of evolution. The greater the differences in the DNA bases that code for the enzyme, the longer the time since two organisms shared a common ancestor. This DNA evidence for evolution has confirmed evolutionary relationships derived from other observations.
By publishing his ideas, Darwin subjected his hypothesis to the tests of others. This process of public scrutiny is an essential part of science. It works to eliminate individual bias and subjectivity, because others must also be able to determine whether a proposed explanation is consistent with the available evidence. It also leads to further observations or to experiments designed to test hypotheses, which has the effect of advancing science.
Many of the hypotheses advanced by scientists turn out to be incorrect when tested by further observations or experiments. But skillful scientists like Darwin tend to have good ideas that end up increasing the amount of knowledge in the world. For this reason, the ideas of scientists have been—over the long run—central to much of human progress.
Science as Cumulative Knowledge
At the time of Darwin, there were many unsolved puzzles, including missing links in the fossil record between major groups of animals. Guided by the central idea of evolution, thousands of scientists have spent their lives searching for evidence that either supports or conflicts with the idea. For example, since Darwin's time, paleontologists have discovered many ancient organisms that connect major groups—such as Archaeopteryx between ancient reptiles and birds, and Ichthyostega between ancient fish and amphibians. By now, so much evidence has been found that supports the fundamental idea of biological evolution that its occurrence is no longer questioned in science.
Even more striking has been the information obtained during the 20th century from studies on the molecular basis of life. The
theory of evolution implies that each organism should contain detailed molecular evidence of its relative place in the hierarchy of living things. This evidence can be found in the DNA sequences of living organisms. Before a cell can divide to produce two daughter cells, it must make a new copy of its DNA. In copying its DNA nucleotides, however, cells inevitably make a small number of mistakes. For this reason, a few nucleotides are changed through random error each time that a cell divides. (For example, an A in the DNA sequence of a gene in a chromosome may be replaced with a G in the new copy made as the cell divides.) Therefore, the larger number of cell divisions that have elapsed between the time that two organisms diverged from their common ancestor, the more differences there will be in their DNA sequences due to chance errors.
This molecular divergence allows researchers to track evolutionary events by sequencing the DNA of different organisms. For example, the lineage that led to humans and to chimpanzees diverged about 5 million years ago—whereas one needs to look back in time about 80 million years to find the last common ancestor shared by mice and
Continental Drift and Plate Tectonics: A Scientific Revolution of the Past 50 Years The theory of plate tectonics demonstrates that revolutions in science are not just a thing of the past, thus suggesting that more revolutions can be expected in the future. World maps have long indicated a curious "jigsaw puzzle fit" of the continents. This is especially apparent between the facing coastlines of South America and Africa. Alfred Wegener (1880 to 1930), a German meteorologist who was dissatisfied with explanations that relied on expanding and contracting crust to account for mountain building and the formation of the ocean floor, pursued other lines of reasoning. Wegener suggested that all of earth's continents used to be assembled in a single ancient super-continent he called Pangea. He hypothesized that Pangea began to break up approximately 200 million years ago, with South America and Africa slowly drifting apart to their present positions, leaving the southern Atlantic Ocean between them. This was an astonishing hypothesis: could huge continents really move? Wegener cited both geological and biological evidence in support of his explanation. Similar plant and animal fossils are found in rock layers more than 200 million years old in those regions where he claimed that different continents were once aligned. Wegener attributed this to the migration of plants and animals freely throughout these broad regions. If 200 million years ago Africa and South America had been separated by the Atlantic Ocean as they are today, their climates, environments, and life forms should have been very different from each other—but they were not. Despite Wegener's use of evidence and logic to develop his explanations, other scientists found it difficult to imagine how solid, brittle continents could plow through the equally solid and brittle rock material of the ocean floor. Wegener did not have an explanation for how the continents moved. Since there was no plausible mechanism for continental drift, the idea did not take hold. The hypothesis of continental drift was equivalent to the hypothesis of evolution in the decades before Darwin, when evolution lacked the idea of variation followed by natural selection as an explanatory mechanism. The argument essentially lay dormant until improved technologies allowed scientists to gather previously unobtainable data. From the mid 1950s through the early 1970s, new evidence for a mechanism to explain continental drift became available that the scientific community could accept. Sonar mapping of the ocean floor revealed the presence of a winding, continuous ridge system around the globe. These ridges were places where molten material was welling up from the earth's interior and pushing apart the plates that form the earth's surface. |
humans. As a result, there is a much smaller difference between human and chimpanzee DNA than between human (or chimpanzee) and mouse DNA. In fact, scientists today routinely use the differences they can measure between the DNA sequences of organisms as "molecular clocks" to decipher the relationships between living things.
The same comparisons among organisms can be made using the proteins encoded by DNA. For example, every living cell uses a protein called cytochrome c in its energy metabolism. The cytochrome c proteins from humans and chimpanzees are identical. But there is only an 86 percent overlap in the molecules between humans and rattlesnakes, and only a 58 percent overlap between us and brewer's years. This is explained by the evolutionary proposition that we shared a common ancestor with chimps relatively recently, whereas the common ancestor that we, as vertebrates, shared with rattlesnakes is much more ancient. Still farther in the past, we and yeast shared a common ancestor—and the molecular data reflect this pattern.
In the past few decades, new methods have been developed that are allowing us to
obtain the exact sequence of all of the DNA nucleotides in chromosomes. The Human Genome Project, for example, will produce when completed the entire sequence of the 3 billion nucleotides that make up our genetic inheritance. The complete sequence of the yeast genome (12 million nucleotides) is already known, as are the genomes for numerous species of bacteria (from 0.5 to 5 million nucleotides each, depending on the species). Similar sequencing efforts will soon yield the complete sequences for hundreds of bacteria and other organisms with small genomes.
These molecular studies are powerful evidence for evolution. The exact order of the genes on our chromosomes can be used to predict the order on monkey or even mouse chromosomes, since long stretches of the chromosomes of mammalian species are so similar. Even the parts of our DNA that do not code for proteins and at this point have no known function are similar to the comparable parts of DNA in related organisms.
The confirmation of Darwin's ideas about "descent with modification" by this recent molecular evidence has been one of the most exciting developments in biology in this century. In fact, as the chromosomes of more and more organisms are sequenced over the next few decades, these data will be used to reconstruct much of the missing history of life on earth—thereby compensating for many of the gaps that still remain in the fossil record.
Conclusion
One goal of science is to understand nature. "Understanding" in science means relating one natural phenomenon to another and recognizing the causes and effects of phenomena. Thus, scientists develop explanations for the changing of the seasons, the movements of heavenly bodies, the structure of matter, the shaping of mountains and valleys, the changes in the positions of continents over time, and the diversity of living things.
The statements of science must invoke only natural things and processes. The statements of science are those that emerge from the application of human intelligence to data obtained from observation and experiment. These fundamental characteristics of science have demonstrated remarkable power in allowing us to describe the natural world accurately and to identify the underlying causes of natural phenomena. This understanding has great practical value, in part because it allows us to better predict future events that rely on natural processes.
Progress in science consists of the development of better explanations for the causes of natural phenomena. Scientists can never be sure that a given explanation is complete and final. Yet many scientific explanations have been so thoroughly tested and confirmed that they are held with great confidence.
The theory of evolution is one of these explanations. An enormous amount of scientific investigation has converted what was initially a hypothesis into a theory that is no longer questioned in science. At the same time, evolution remains an extremely active field of research, with an abundance of new discoveries that are continually increasing our understanding of exactly how the evolution of living organisms actually occurred.
THE CONCERNS OF SCIENCE An Excerpt from the Book This Is Biology: The Science of the Living World (1997) By Ernst Mayr It has been said that the scientist searches for truth, but many people who are not scientists claim the same. The world and all that is in it are the sphere of interest not only of scientists but also of theologians, philosophers, poets, and politicians. How can one make a demarcation between their concerns and those of the scientist? How Science Differs from Theology The demarcation between science and theology is perhaps easiest, because scientists do not invoke the supernatural to explain how the natural world works, and they do not rely on divine revelation to understand it. When early humans tried to give explanations for natural phenomena, particularly for disasters, invariably they invoked supernatural beings and forces, and even today divine revelation is as legitimate a source of truth for many pious Christians as is science. Virtually all scientists known to me personally have religion in the best sense of this word, but scientists do not invoke supernatural causation or divine revelation. Another feature of science that distinguishes it from theology is its openness. Religions are characterized by their relative inviolability; in revealed religions, a difference in the interpretation of even a single word in the revealed founding document may lead to the origin of a new religion. This contrasts dramatically with the situation in any active field of science, where one finds different versions of almost any theory. New conjectures are made continuously, earlier ones are refuted, and at all times considerable intellectual diversity exists. Indeed, it is by a Darwinian process of variation and selection in the formation and testing of hypotheses that science advances. Despite the openness of science to new facts and hypotheses, it must be said that virtually all scientists—somewhat like theologians—bring a set of what we might call "first principles" with them to the study of the natural world. One of these axiomatic assumptions is that there is a real world independent of human perceptions. This might be called the principle of objectivity (as opposed to subjectivity) or common-sense realism. This principle does not mean that individual scientists are always "objective" or even that objectivity among human beings is possible in any absolute sense. What it does mean is that an objective world exists outside of the influence of subjective human perception. Most scientists—though not all—believe in this axiom. Second, scientists assume that this world is not chaotic but is structured in some way, and that most, if not all, aspects of this structure will yield to the tools of scientific investigation. A primary tool used in all scientific activity is testing. Every new fact and every new explanation must be tested again and again, preferably by different investigators using different methods. Every confirmation strengthens the probability of the "truth" of a fact or explanation, and every falsification or refutation strengthens the probability that an opposing theory is correct. One of the most characteristic features of science is this openness to challenge. The willingness to abandon a currently accepted belief when a new, better one is proposed is an important demarcation between science and religious dogma. The method used to test for "truth" in science will vary depending on whether one is testing a fact or an explanation. The existence of a continent of Atlantis between Europe and America became doubtful when no such continent was discovered during the first few Atlantic crossings in the period of discoveries during the late fifteenth and early sixteenth centuries. After complete oceanographic surveys of the Atlantic Ocean were made and, even more convincingly, after photographs from satellites were taken in this century, the new evidence conclusively proved that no such continent exists. Often, in science, the absolute truth of a fact can be established. The absolute truth of an explanation or theory is much harder, and usually takes much longer, to gain acceptance. The "theory" of evolution through natural selection was not fully accepted as valid by scientists for over 100 years; and even today, in some religious sects, there are people who do not believe it. Third, most scientists assume that there is historical and causal continuity among all phenomena in the material universe, and they include within the domain of legitimate scientific study everything known to exist or to happen in this universe. But they do not go beyond the material world. Theologians may also be interested in the physical world, but in addition they usually believe in a metaphysical or supernatural realm inhabited by souls, spirits, angels, or gods, and this heaven or nirvana is often believed to be the future resting place of all believers after death. Such supernatural constructions are beyond the scope of science. |
Dialogue
TEACHING EVOLUTION THROUGH INQUIRY
The following dialogue demonstrates a way of teaching about evolution using inquiry-based learning. High school students are often interested in fossils and in what fossils indicate about organisms and their habitats. In the investigation described here, the students conduct an inquiry to answer an apparently simple question: What influence has evolution had on two slightly different species of fossils? The investigation begins with a straightforward task—describing the characteristics of two species of brachiopods.
********
"Students, I want you to look at some fossils," says Karen. She gives the students a set of calipers and two plastic sheets that each contain about 100 replicas of carefully selected fossil brachiopods.1 "These two sheets contain fossils from two different species of a marine animal called a brachiopod. Let's begin with some observations of what they look like."
"They look like butterflies," replies one student.
"They are kind of triangular with a big middle section and ribs," says another student.
"Can you tell if there are any differences between the fossils in the two trays?"
The students quickly conclude that the fossils have different sizes but that they cannot really tell any other difference.
"In that case, how could you tell if the fossil populations are different?" Karen asks.
"We can count the ribs."
"We can measure them."
"Those are both good answers. Here's what I want you to do. Break into groups of four and decide among yourselves which of those two characteristics of the fossils you want to measure. Then graph your measurements for each of the two different populations."
For the rest of the class period, the students investigate the fossils. They soon realize that the number of ribs is related to the size of the fossils, so the groups focus on measuring the lengths and widths of the fossils. They enter the data on the two different populations into a computer data
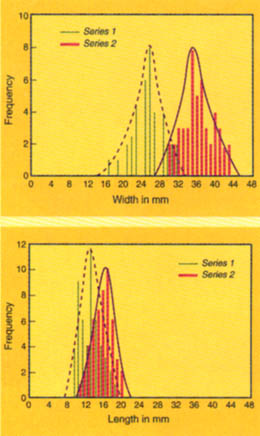
Graphs showing characteristics of brachiopod populations.
base. Two of the graphs that they generate are shown on the facing page.
"Now that we have these graphs of the fossils' lengths and widths," Karen says at the beginning of the next class period, "we can begin to talk about what these measurements mean. We see from one set of graphs that the fossils in the second group tend to be both wider and longer than those in the other group. What could that mean?"
"Maybe one group is older," volunteers one of the students.
"Maybe they're different kinds of fossils," says another.
"Let's think about that," says Karen. "How could their lengths and widths have made a difference to these organisms?"
"It could have something to do with the way they moved around."
"Or how they ate."
"That's good," says Karen. "Now, if you had dug up these fossils, you would have some additional information to work with, so let me give you some of that background. As I mentioned last week, these fossils are from marine animals known as brachiopods. When they die their shells are often buried in sediments and fossilized. What I know about the fossils you have is that they were taken from sediments that are about 400 million years old. But the two sets of fossils were separated in time by about 10 million years.
"Taking that information, I'd like you to do some research on brachiopods and develop some hypotheses about whether or not evolution has influenced their size. Here are some of the questions you can consider as you're writing up your arguments."
Karen hands out a sheet of paper containing the following questions:
-
What differences in structure and function might be represented in the length and width of the brachiopods? Could efficiency in burrowing or protection against predators have influenced their shapes?
-
Why might natural selection influence the lengths and widths of brachiopods?
-
What could account for changes in their dimensions?
The following week, Karen holds small conferences at which the students' papers are presented and discussed. She focuses students on their ability to ask skeptical questions, evaluate the use of evidence, assess the understanding of geological and biological concepts, and review aspects of scientific inquiries. During the discussions, students are directed to address the following questions: What evidence would you look for that might indicate these brachiopods were the same or different species? How could changes in their shapes have affected their ability to reproduce successfully? What would be the likely effects of other changes in the environment on the species?
NOTE
1. |
The materials needed to carry out this investigation are available from Carolina Biological Supply Company, 2700 York Rd., Burlington, NC 27215. Phone: 1-800-334-5551. www.carolina.com |