14
Advances in Ambulatory Monitoring: Using Foot Contact Time to Estimate the Metabolic Cost of Locomotion
Reed W. Hoyt1and Peter G. Weyand
INTRODUCTION
Physical activity plays a pivotal role in maintaining the physical fitness, trim appearance, and health of members of both the public and the U.S. military (AR 600-9, 1986; Byers, 1995; U.S. Department of the Army, 1992). However, a shortage of valid and reliable instrumentation to assess the physical activity and physical fitness of free-living humans has hampered understanding of this complex subject (Montoye and Taylor, 1984; Powell and Paffenbarger, 1985; Taube, 1995). The ambulatory foot contact monitor (FCM), which accurately estimates the metabolic cost of locomotion, is a new addition to the existing array of methods used to assess physical activity (Hoyt et al., 1994; LaPorte et al., 1985; Saris, 1986). This technology offers new opportunities to study the
dose-response relationships of walking and running to macronutrient requirements, weight control, physical fitness, and the incidence of disease and injury.
The first of the following sections describes FCM technology and some potential applications. Next, the fundamental research linking the energetics and mechanics of human locomotion is reviewed. Finally, advantages and disadvantages of the FCM technology and suggestions for future research are discussed.
DESCRIPTION OF FOOT CONTACT MONITOR TECHNOLOGY
The ambulatory FCM accurately estimates the metabolic cost of locomotion (Mloco = total rate of energy expenditure — estimated rate of resting energy expenditure) from the ratio of total body weight to the time during each stride that a single foot is in contact with the ground (Hoyt et al., 1994). As discussed below, laboratory research has shown that the ˙Mloco is determined primarily by the rate of force generation needed to support total body weight (Kram and Taylor, 1990). The rate of force generation can be estimated from the ratio of total body weight to foot contact time. At a given total body weight, increases in the speed of locomotion are associated with decreases in foot contact time, increases in the rate of force generation, and increases in the ˙Mloco.
The FCM is a relatively simple electronic device that measures the time the foot is in contact with the ground, and the time the foot is in the air, during each stride (see Figure 14-1). This is accomplished by a sensor circuit, an analog-to
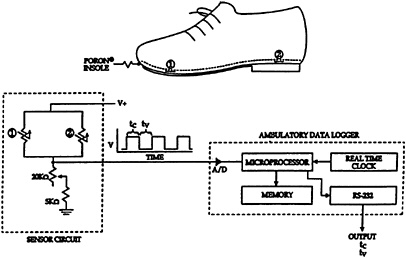
FIGURE 14-1 Force-sensing resistors Schematic diagram of ambulatory electronic foot contact monitor. (1,2) were placed under the ball and heel of one foot. A/D, analog to digital converter; tc, time of foot contact for each stride; tv, time foot is in the air for each stride; V, voltage; O, ohm (the unit of electric resistance equal to the resistance of a circuit in which a potential difference of 1 volt produces a current of 1 ampere). SOURCE: Journal of Applied Physiology (Hoyt et al., 1994), used with permission.
digital converter, and a microcontroller (microprocessor, memory, real time clock, and interface). The sensor circuit consists of two force sensors (consisting of either switches or force-sensitive resistors) located in the insole of a shoe or boot, one sensor under the ball and the other under the heel of the foot. These force sensors, which form part of a voltage divider circuit,2 simply indicate whether or not the foot is in contact with the ground. During foot contact, the sensor circuit resistance decreases dramatically, and the voltage output becomes more positive. When the foot is elevated, sensor circuit resistance increases, and the voltage output becomes less positive. An analog-to-digital converter converts the output of the voltage divider sensor circuit into a digital signal that is processed and stored by a low cost microcontroller. The duration of each foot contact and noncontact period is recorded, and minute-to-minute averages of the ˙Mloco are calculated and displayed and/or stored.
The FCM was used in a laboratory study to derive and cross-validate an equation for estimating ˙Mloco from body weight (Wb) and foot contact time (tc) (Hoyt et al., 1994). Indirect calorimetry was the criterion method used to measure the total rate of energy expenditure. Specifically, the rate of energy expenditure at each speed during treadmill exercise was calculated from oxygen consumption (˙VO2) and carbon dioxide production (˙VCO2) using conventional indirect calorimetric relationships (Lusk, 1928, 61–74). Each subject was tested at three walking speeds and three running speeds. Resting energy expenditure was estimated from height, weight, and age by the Harris-Benedict equation (Harris and Benedict, 1919). ˙Mloco was calculated by subtracting resting energy expenditure from total energy expenditure. Twelve young males were tested during horizontal treadmill walking and running. The equation to estimate ˙Mloco was derived in six randomly selected subjects:

Cross-validation in the remaining six subjects showed that estimated and measured ˙Mloco were highly correlated (r2 = 0.97) (Figure 14-2). The average individual error between estimated and measured ˙Mloco was 0 percent (range = –22 to 29%). A simple ambulatory FCM, calibrated only for the individual's body weight, apparently can be used to estimate the ˙Mloco (kcal/min, watts) accurately over a full range of walking and running intensities.
APPLICATIONS OF FCM TECHNOLOGY
The FCM can be used to estimate the ˙Mloco of individuals in their natural environment without complex equipment. Minute-to-minute or day-to-day pat-
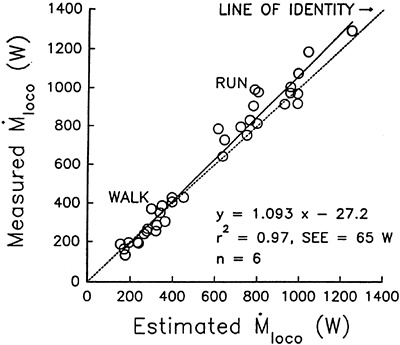
FIGURE 14-2 Relationship of measured metabolic cost of locomotion and the estimated metabolic cost of locomotion. Estimated ˙Mloco was calculated using the equation ˙Mloco = 3.702(Wb/tc) – 149.6 derived in a separate group of six young men. The 36 data points represent 6 subjects tested at 6 speeds (3 walking speeds and 3 running speeds). W, watts. SOURCE: Journal of Applied Physiology (Hoyt et al., 1994), used with permission.
terns of energy expended in walking and/or running can be determined. In addition, FCMs can be used to monitor changes in absolute or relative aerobic fitness.
The FCM could be used to address a variety of basic questions regarding physical activity patterns and physical fitness in free-living humans, particularly in relationship to the incidence of obesity, disease, and injury (Blair, 1993; Blair et al., 1992). Assessing low-level activity such as walking is of particular interest, given its apparent importance in maintaining health and energy balance and avoiding obesity (Hovell et al., 1992). Furthermore, FCMs with alphanumeric displays could provide the user with an immediate source of information about energy expenditure and physical fitness. This type of feedback could be beneficial in weight management, aerobic conditioning, and rehabilitation programs (Dausch, 1992).
Detailed information on the energetic costs of locomotion could also be useful in (1) determining the macronutrient and water requirements of soldiers in the field, (2) defining the metabolic cost of the numerous military tasks that involve movement by foot and load bearing (Patton et al., 1991), (3) improving
heat strain prediction models (Kraning, 1991), and (4) clarifying the relationship of walking and running with the incidence of training injuries.
Macronutrient Requirements of Soldiers in the Field
A recurrent theme of meetings of the Committee on Military Nutrition Research is the need for more accurate and detailed estimates of the intensity, duration, and frequency of exercise performed by soldiers. This type of information, which FCM technology could help obtain, is needed to calculate actual macronutrient requirements and design better operational rations. In addition, quantitative data on the energy expenditure of soldiers are necessary in calculating water requirements (Hoyt and Honig, 1996; Kraning, 1991).
Weight Management
Obesity and lack of physical fitness are common in the United States (Russell et al., 1995) and often go hand in hand. This is reflected in the primary goal of Army Regulation 600-9 (1986), ''The Army Weight Control Program," which "is to insure that all personnel—(1) Are able to meet the physical demands of their duties under combat conditions. (2) Present a trim military appearance at all times."
In a behavioral treatment program for obesity, an experimental group that used an interactive computer program to monitor energy balance was more successful at losing weight and maintaining a lower body weight than was a control group (Burnett et al., 1985). Analogously, an FCM displaying cumulative and real time estimates of the energy cost of locomotion, and even estimated speed and distance traveled, may encourage walking and running. More effective monitoring of personal physical activity patterns also may improve the typically poor adherence to exercise routines (Hakala, 1994; King et al., 1992).
Another way to promote regular physical activity may be to encourage an increase in the level of routine activities (Brownell et al., 1980). Walking and running, two major components of normal physical activity (Perrier Study of Fitness in America, 1979), could be fostered through the use of FCM technology. The FCM method is unique among ambulatory monitoring technologies in its ability to estimate the energy cost of walking. While walking is often very low in intensity, it is more effective than higher intensity exercise in promoting body fat combustion (Zierath and Wallberg-Henriksson, 1992).
Physical Fitness
The FCM technology could be used to help athletes and soldiers meet physical fitness goals (U.S. Department of the Army, 1992) in two ways. First, the FCM method can be used to monitor the frequency, duration, and absolute
intensity of walking or running exercise. Second, the relationship of heart rate to the ˙Mloco estimated by the FCM method can be used to improve the effectiveness of aerobic training programs. Total energy expenditure can be estimated from heart rate if the relationship of heart rate to oxygen consumption and energy expenditure is established for each individual. The ˙Mloco also can be calculated as the difference between total energy expenditure and resting energy expenditure. The heart rate method provides useful estimates of energy expenditure at moderate-to-high work rates but is inaccurate at low activity levels (Ceesay et al., 1989; Christensen et al., 1983; Meijer et al., 1989; Spurr et al., 1988).
Although it is easy to set guidelines for exercise duration and frequency, it is much more difficult to specify the exercise intensity needed for an aerobic training effect since it varies widely both among and within individuals as a function of fitness (Ästrand and Rodahl, 1986; Pollock, 1973; Shephard, 1968). Classically, the effects of aerobic training on cardiorespiratory fitness are assessed by measuring maximal oxygen uptake during exercise to exhaustion (Clausen, 1977).
A simpler approach is to use FCM estimates of ˙Mloco to monitor aerobic fitness. When an accurate comparison of different individuals is needed, absolute aerobic fitness can be estimated from measurements of the maximal ˙Mloco. However, a submaximal test can be used to assess relative changes in individual aerobic conditioning reliably during the course of an exercise program.
Specifically, the efficacy of aerobic fitness programs can be assessed by following changes in the linear relationship of heart rate to ˙Mloco in response to training. A relative increase in an individual's aerobic fitness will be reflected in decreases in heart rate at specific work loads, and vice versa (Kappagoda et al., 1979). The feedback provided by periodic determination of heart rate at various standardized submaximal ˙Mloco solves the central problem of defining the intensity required for an individual to increase aerobic fitness.
Unfit or obese individuals, or those with metabolic disorders, may benefit from a progressive approach to exercise training (Young, 1995). Additionally, the individually tailored aerobic training programs made possible by FCM technology should help reduce high attrition rates often associated with training programs involving high-intensity physical activity (Westerterp et al., 1992).
Epidemiology and Patient Rehabilitation
Although the impact of physical activity on long-term and short-term health is significant (Blair et al., 1992; Byers, 1995; Paffenbarger et al., 1986; Siscovick et al., 1985), the dose-response relationships have been difficult to quantify in free-living humans (LaPorte et al., 1985). The FCM could help solve this problem by facilitating the collection of detailed information on the intensity, frequency, and duration of walking and running. This type of information
also is needed in studies of the etiology of running injuries (van Mechelen, 1992). The FCM technology could also facilitate the monitoring and management of patients involved in orthopedic and cardiorespiratory rehabilitation (Ettinger and Afable, 1994; Todd et al., 1992).
FOOT CONTACT TIMES AND METABOLIC RATES DURING LOCOMOTION: A CENTURY OF EXPERIMENTATION LINKING ENERGETICS AND MECHANICS
The relationship between energetics and mechanics during exercise was considered by Nobel Laureate A. V. Hill and his coworkers early in this century. While performing isolated muscle experiments that established the fundamental mechanical properties of skeletal muscle, Hill's group also was interested in understanding how muscles are used during exercise. Hill's original ideas were both intuitive and reasonable, but they proved to be incorrect. Today's answers, based in part on his isolated muscle experiments, are less intuitive, but nonetheless simple. A century of experimentation has led to a general theory of how muscles are used during locomotion and how their use is related to the metabolic costs that are incurred. The theory is based on the invariant properties of the muscles, bones, and tendons used for support and movement. It incorporates functional principles from molecular, cellular, and tissue levels to explain how muscles function in the multijointed lever systems of whole animals. It provides simple quantitative relationships that account for the energy cost of running, virtually over the entire continuum of animal sizes and running speeds. Although it was developed to explain the metabolic cost of locomotion, the general nature of the theory suggests that it also will apply to other forms of exercise.
One practical outcome of the progress in scientific understanding of the energetics and mechanics of locomotion is the potential for accurate determination of energy expenditure without the collection of expired gases. The development of the less cumbersome technology will allow metabolic rate to be quantified more accurately in many settings. What follows is the experimental evolution of our understanding of the energetics and mechanics of terrestrial locomotion that provided the background for the development of this technology.
Fundamentals of Terrestrial Locomotion
The first measurements of the energetic requirements of locomotion were made by the German scientist Zuntz (1897) at the end of the nineteenth century. He measured the rates of oxygen consumption to determine the metabolic energy expended by humans and dogs walking and running on a treadmill at different speeds over various inclines. He found that metabolic rate increased linearly with speed for both humans and dogs during both level and inclined running. Subsequent measures showed that this relationship is linear for nearly all
terrestrial animals that run (Figure 14-3) and established the linear increase in metabolic rate with running speed as the first fundamental principle of the energetics of terrestrial locomotion (Taylor et al., 1970, 1982).
One consequence of the linear increase of oxygen consumption with speed is that the same amount of energy is expended to run a mile (calculated as the slope of the oxygen consumption vs. running speed) regardless of running velocity. This cost is generally expressed in body mass-specific terms (J/nm) and is referred to as the cost of transport. The measurements taken on animals of different sizes since Zuntz's initial experiments have shown that oxygen consumption increases more rapidly with speed in smaller animals. The systematic nature of these differences established the second fundamental principle of the energetics of terrestrial locomotion: the cost of transport decreases in a regular manner with increases in body size, scaling with body mass to the -0.30 power (Taylor et al., 1980) (Figure 14-4). Thus, if size extremes in nature are considered, the 10-g shrew expends 100 times more energy per unit body mass than the 2,000-kg elephant to run a given distance.
Hill (1950) attributed both the linear increase in metabolic cost with running speed and the lower transport costs of larger animals to differences in the rate at which muscles performed the mechanical work of running. This explanation later proved to be incorrect due to Hill's assumptions about the relationship
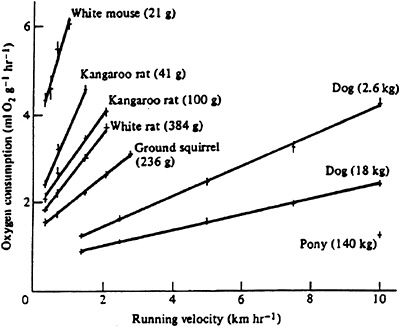
FIGURE 14-3 Oxygen consumption increases linearly with running speed in terrestrial animals. SOURCE: American Journal of Physiology (Taylor et al., 1970), used with permission.
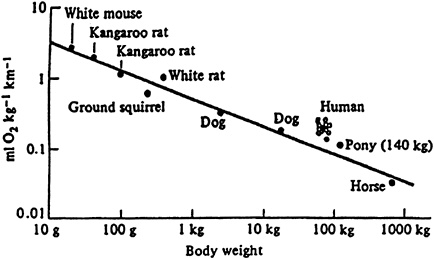
FIGURE 14-4 The cost of transport (the slope of the relationship between oxygen consumption and running speed) is higher in small animals than in large, scaling with body mass to the -0.30 power. SOURCE: American Journal of Physiology (Taylor et al., 1970), used with permission.
between metabolic cost and the work of running. It would require decades of experimentation before it would become clear which of the mechanical tasks performed during running required metabolic energy.
What Sets Cost?
The Mechanical Work of Running and Metabolic Cost
The movements of an animal or a human running steadily at a brisk pace must be finely coordinated in time and space. Airborne and grounded periods alternate regularly. Limbs oscillate in relation to one another and the torso. The center of mass rises and falls, and it accelerates and decelerates during the period of support. These movements involve fluctuations in both the kinetic and gravitational potential energy of the animal's center of mass and limbs, and it is logical to hypothesize that muscles perform the work necessary to effect these energy changes as efficiently as possible. Indeed, the scientists who first considered the question started with this hypothesis.
Cavagna and Margaria were among the first to attempt to determine muscular efficiency (mechanical work of running/metabolic energy expended) during locomotion (Cavagna et al., 1964). They expected to find that the mechanical work of running would be performed at the maximal efficiency (20–25%) that Hill had measured for isolated muscle. Using a force platform and video analysis to measure the work of running, their measurements produced a surprising result. The efficiency of running exceeded the maximal efficiency of isolated
muscle. In fact, at high speeds, the efficiency reached 70 percent, roughly three times the maximal value for isolated muscle. How could such a result be explained? They concluded that muscles did not perform all of the positive work done during each running stride. The cyclical positive and negative oscillations of the kinetic and gravitational potential energy that corresponded to the changes in the speed and height of the body apparently allowed much of the work to be performed conservatively by springs. Because the fluctuations that they measured in the kinetic and gravitational potential energy of the body's center of mass were in phase, they concluded that muscles and tendons stored potential energy elastically during one portion of the stride and released it during another, in a manner analogous to a spring or bouncing ball. Clearly, not all of the work of running required metabolic energy.
Perhaps the energy saved by "running springs" was related to the linear increase in oxygen consumption with running speed and to the higher transport costs of smaller animals. Subsequent investigations upheld this possibility. Models of the mechanics of running, which treated humans and animals as "linear springs" with masses concentrated above their support limbs, predicted almost perfectly the changes in stride lengths, frequencies, landing, and takeoff angles that occurred during actual running (Blickhan, 1989; McMahon and Cheng, 1990). Mechanical tests on tendons, conducted by Alexander and colleagues (1981), indicated that in running humans, 50 percent of the mechanical work required could be stored and released by the springs in the Achilles tendon and the arch of the foot alone (Ker et al., 1987). Furthermore, Cavagna's force platform and video technique indicated that there might be important differences in how well springs worked in different-sized animals (Cavagna et al., 1964). The efficiencies of large, fast animals, such as ponies, reached values as high as 150 percent, while those of small animals, such as rats and mice, reached only 6 percent at their top aerobic speeds (Heglund et al., 1982). Did the better springs of larger animals explain their lower transport costs?
This question was difficult to resolve because the relationship of the efficiencies of whole animals indicated little about the efficiencies of their running muscles. This was true for several reasons. It was not possible to partition the total mechanical work into that performed by the muscles and that conserved by springs and pendulums. Neither the energy stored elastically in springs nor the energy transferred among body segments and the torso could be quantified directly. This made it impossible to know how much of the total work the muscles actually were doing. Also, these work measurements included only the positive work done during each stride. The running muscles were certainly active while they were being lengthened by gravity. The forces they generated during lengthening incurred a metabolic cost, but this was not included in this analysis. Therefore, determining how much of the chemical energy released by the running muscles was converted into mechanical work (as in Hill's isolated muscle experiments) was simply not possible with this technique. More evidence was
needed before the mechanical tasks performed by the running muscles that required metabolic energy could be identified.
Generating Force to Support the Weight of the Body
The lack of definitive answers from measurements of the mechanical work of running and further consideration of running mechanics prompted C. R. Taylor and colleagues to adopt a different approach. These scientists recognized that doing work is not the only requirement of an animal running on level ground at a constant speed. Because the height of the body's center of mass does not change over time and the amount of work required to overcome wind resistance is generally small, the net work done by the body on the environment is very close to zero. However, even if the conservation of the mechanical energy required for running at a constant speed were perfect (i.e., 100%, so that the muscles need not perform any work), the active muscles would still be required to generate a time-averaged ground force equal to the weight of the body. With these thoughts in mind, Taylor and colleagues undertook a number of experiments to test the relationship between supporting body weight and metabolic cost.
One of their first experiments involved having animals ranging in size from mice to horses run on treadmills while carrying loads weighing between 5 and 30 percent of their body masses (Taylor et al., 1980). They found that at the same speeds, animals ran with the same stride lengths and stride frequencies in the weighted and unweighted conditions. This finding was fortuitous because it meant that the forces generated by the running muscles to support the weight of the body increased in direct proportion to the load on the animal. The results showed that metabolic cost increased in direct proportion to the weight carried by the animal; that is, a 10 percent increase with a load equal to 10 percent of body weight, a 20 percent increase with a load equal to 20 percent of body weight, and so on. This was an important result. It suggested that generating force to support the weight of the body during running was directly related to metabolic cost.
Equivalent experiments were conducted in simulated reduced gravity, which allowed the vertical force that was generated to be reduced by different amounts (Farley and McMahon, 1992). In these experiments, the metabolic cost of running decreased in direct proportion to the amount of force required to support the weight of the body under the different conditions. In both the weighting and the reduced gravity experiments, there was a 1:1 relationship between the force generated to support the weight of the body and the metabolic cost of running.
The weighting and reduced gravity experiments indirectly supported the related conclusion that the metabolic importance of swinging limbs was small. If the kinetic energy required to swing limbs was responsible for a significant portion of the metabolic energy expended by the animal, loading or unloading a
person or animal by a percentage of its body weight ought to alter the metabolic cost by some lesser factor. However, this was not the case under any of the conditions. This conclusion reinforced one reached previously through a different experimental approach (Taylor et al., 1974). These researchers selected animals of the same weights (goats, cheetahs, and gazelles) but different distributions of that weight between the limbs and torso. They found that the energetic cost of treadmill running in the three species was identical. This was true despite vast differences in the amount of work necessary to swing the slight limbs of the gazelle in comparison to the sturdy limbs of the cheetah. If the work of swinging limbs demanded metabolic energy, the cheetah should have higher metabolic costs, but this was not the case.
The view that emerged from the weighting, reduced gravity, and limb inertia experiments differed but did not contradict that provided by the mechanical work experiments. Measurements of the mechanical work of running and its relationship to metabolic cost (i.e., efficiency) were not meaningful if much or all of the mechanical work being performed was conserved by springs and pendulums. The direct relationship between metabolic cost and the forces generated by the active muscles to support the weight of the body raised what seemed to be an unlikely possibility: springs and pendulums performed all of the work of running regardless of animal size and running speed.
Linking Force Generation to Energetic Fundamentals
Even though the results of the mechanical work, reduced gravity, and weighting experiments were consistent and suggested a direct relationship between vertical force and metabolic cost, these experiments were indirect and not completely conclusive. The results did not provide an explanation for the linear increase in metabolic rate with running speed, nor for the lower transport costs of larger animals. Explaining the basic energetic principles of locomotion required linking the mechanical and metabolic properties of muscles to force generation during locomotion in whole animals.
The mechanics of running, like the energetics of running, appeared to conform to general patterns. For example, the mechanics of running at different speeds and in different-sized animals appeared to be achieved through equivalent movements that took place at different rates. In both humans and animals, the mechanics of what the muscles and tendons did during the support phase, and when the critical vertical forces were generated, were virtually the same across the range of running speeds. This was true of the angles of individual joints during support (Biewener et al., 1981; McMahon and Cheng, 1990), the stiffness of leg springs (force/leg displacement) (Farley et al., 1993; McMahon and Cheng, 1990), and step lengths (defined as the horizontal distance moved by the center of mass while the foot is in contact with the ground) (Taylor et al., 1980) (see Figure 14-5). However, at faster running speeds, and in smaller animals, these movements took place more rapidly.
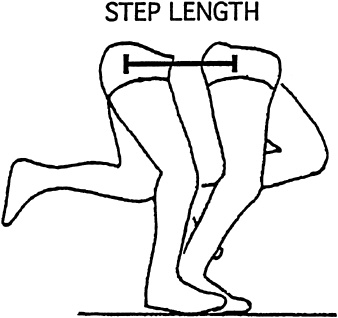
FIGURE 14-5 Step length is the horizontal distance moved by the center of mass while a single foot is in contact with the ground. SOURCE: Wright (1995), used with permission.
There also was evidence from different-sized animals that the differences in mechanical activity were directly related to the differences in metabolic cost (Heglund and Taylor, 1988). Smaller animals had higher metabolic costs and ran with higher stride frequencies. With increases in running speed, the metabolic cost per stride increased in all animals. However, when metabolic cost was expressed on a per stride basis at equivalent speeds, such as the trot-gallop transition, it was the same for all animals. The fact that at equivalent speeds, different-sized animals had the same ratio of support time/total time indicated that the size differences in metabolic cost were proportional to the periods of ground contact (tc) in large and small animals and suggested a link to the time available for force development (Rome, 1992).
If the time course of foot contact was a determinant of metabolic cost, as previous results suggested it might be, changing this variable ought to change cost. This easily could be achieved by having humans change their natural stride frequencies and support periods by running in time to a metronome. When humans either increased or decreased their freely chosen step lengths, the metabolic cost of running was greater than that at their naturally chosen frequency (Cavanaugh and Williams, 1982; Farley and Gonzalez, 1996). Clearly, the freely chosen step lengths and corresponding contact times minimized metabolic cost.
Insights from Changing Contact Times
What then dictated the lengths of the steps and contact times that were naturally selected at any running speed? Again, the answer was provided by human experiments in which the naturally chosen frequencies during running and hopping were altered (Farley and Gonzalez, 1996; Farley et al., 1991). During both running and hopping, the body behaved as a spring; that is, force had the same relationship to displacement during both the yield and rebound stages of the support phase. When stride or hopping frequency was decreased and the ground contact period increased, the body no longer behaved this way. The higher forces during the rebound phase and the increased metabolic rate suggested that additional mechanical work from the muscles had to be supplied to replace the work not being performed by the body's springs (Figure 14-6). Slowing the rate of force generation disrupted the body's springs and made the muscles do more work.
Metabolic rate also increased when humans ran at higher-than-preferred stride frequencies, which decreased the period of ground contact at any running or hopping speed. Force displacement curves indicated that the running springs worked nearly perfectly under this condition, as they did at the preferred frequency, which suggested that muscular work did not change, but that the mus-
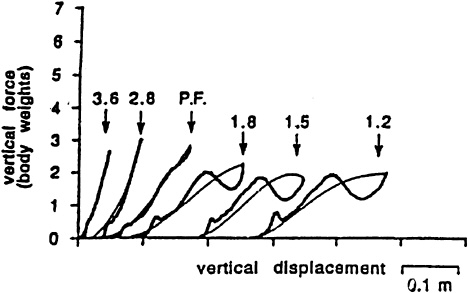
FIGURE 14-6 During human hopping (and also running) at natural frequencies (PF) and above, forces when the body moves toward (thick lines) and away (thin lines) from the earth during the support phase are the same at any displacement of the center of mass; that is, the body behaves as a spring. At lower frequencies, the higher forces during the rebound phase indicate the body no longer behaves this way. SOURCE: Journal of Applied Physiology (Farley et al., 1991), used with permission.
cles had to support the weight of the body in a shorter period of time. The increase in metabolic cost when humans ran at higher-than-preferred frequencies was directly related to the decreased period of contact during which the muscles developed vertical force (Farley and Gonzalez, 1996). The stride length experiments added further evidence that the rate of vertical force development was a fundamental determinant of the metabolic cost of running. Specifically, they indicated that chosen step lengths and periods of force generation minimized metabolic cost by allowing force to be developed at the slowest rates that still allowed the body to behave as a natural spring.
Equally interesting was the finding that the step lengths that dictated ground contact times were nearly unchanged across running speeds. This suggested an optimal mechanical solution for minimizing the muscular forces and the cost of generating vertical force against the ground. A consequence of the near constancy of step lengths was a regular decrease in the period of time the foot was in contact with the ground, with increases in running speed. This was true regardless of whether the increases in running speed were brought about by increases in stride frequency or stride length. Thus, the generation of a time-averaged ground force equal to body weight takes place in progressively shorter periods of time as running speed is increased (Figure 14-7). Taylor and colleagues recognized that the period of ground contact must set the rate at which force is developed to support the weight of the body. This prompted them to examine the relationship between the metabolic cost of running and the period of ground contact in different-sized animals across their range of aerobic running speeds where cost could be measured (Kram and Taylor, 1990). Using the inverse of ground contact times to estimate the rates of muscular force generation needed to support the weight of the body, they found a constant relationship between metabolic rate and the inverse of the ground contact (1/tc) period across a 10-fold range of running speeds and a 4,500-fold range of body masses in both quadrupeds and bipedal hoppers.
Why Do Contact Times Set Metabolic Rates?
The implications of this intriguing result brought Taylor and colleagues back to the experiments and questions of Hill almost 70 years earlier and pro vided an attractive link between the classic responses of muscle in in vitro and in situ preparations and their operation in living animals. The explanation for the constant relationship between the rate of force generation and metabolic rate was simple. The inverse of the time of foot-ground contact and corresponding rates of force generation dictated the speed of the muscle fibers recruited to generate the required force. As running speed increased, the time available for force generation (tc) decreased, and the rate at which force was developed (1/tc) in creased. The proposed links among running speed, rates of force generation, and fiber speed was consistent with what was known about the metabolic cost of force generation in isolated muscle (Rome et al., 1990; Woledge et al., 1985)
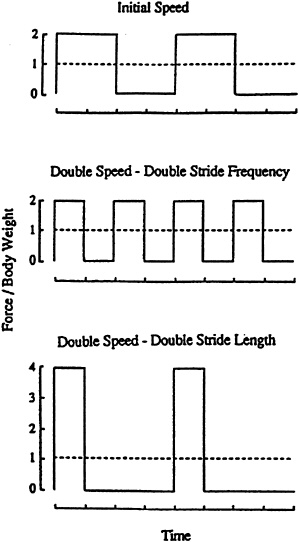
FIGURE 14-7 Whether running speed is increased by increasing stride length (period of zero force) or stride frequency (frequency of force pulses), the time of foot-ground contact (force pulse width) decreases as speed increases if step lengths are nearly constant. Shorter contact times require greater rates of force generation to support the weight of the body. The dashed line, equal to one body weight, is the time-averaged force exerted against the ground. SOURCE: Reprinted from Taylor (1992) by courtesy of Marcel Dekker, Inc.
and fiber recruitment patterns in whole animals (Armstrong and Laughlin, 1985; Henneman et al., 1965; Walmsley et al., 1978). The explanation also was supported by the close agreement between the ranges of 1/tc and those of the maximal shortening velocities of the muscles reported for both individual and different-sized animals (Rome et al., 1990). Furthermore, the constant rate-normalized cost indicated that the muscular activity at different running speeds was
equivalent. If running faster was achieved through shortening the muscles faster, as Hill had suggested, the metabolic cost of force generation also should increase as it did in isolated muscle, but this was not the case. This explanation also was consistent with the relative constancy of the mechanics of support across running speed. The simple relationship between rates of force generation and fiber speed provided a compelling explanation for how the mechanical properties of muscle on molecular, cellular, and tissue levels operate within the lever systems of animals to satisfy the mechanical requirements of running.
Why is the Metabolic Cost of Generating Force the Same in Large and Small Animals?
The truly remarkable finding was that rates of force generation could explain completely the metabolic cost of force generation in running animals, not only across running speeds but also across the entire spectrum of animal sizes. It indicated that both the activities and the volumes of muscle recruited by different-sized animals were the same. This was curious given the identical need of large and small animals to support their body weights and the more crouched posture of small animals. These postural differences required higher muscle forces in small animals to generate the same ground reaction force. Biewener (1989) defined this ratio (ground force/muscle force) as the effective mechanical advantage (EMA) and quantified it by measuring muscle moment arms (r, the distance from the muscle-tendon insertion point on the bone to the joint axis of rotation) and the perpendicular distances from the ground reaction force vectors during running to the joint axis of rotation (R) (Figure 14-8). He found that EMA scaled with body mass to the 0.80 power. How then could small animals generate higher muscle forces, which required greater muscle cross-sectional areas, with the same muscle volumes? The only possibility was for the smaller animals to generate this force with shorter muscle fibers. Indeed, nature had provided this solution. Alexander and colleagues (1981) had shown that muscle fiber length changes with body size, scaled with body mass to the 0.20 power, exactly offsetting the size differences in EMA and muscle forces to result in the same muscle volumes being recruited by large and small animals to generate force against the ground (Biewener, 1989).
Summary
In conclusion, nearly a century of experimentation produced considerable progress in the understanding of the relationship between the energetics and mechanics of exercise. The work of running was not related to metabolic cost, as Hill and others had initially hypothesized, because the muscles of animals running on level ground performed very little work. Rather, metabolic cost was set by the forces generated by the muscles to support the weight of the body.
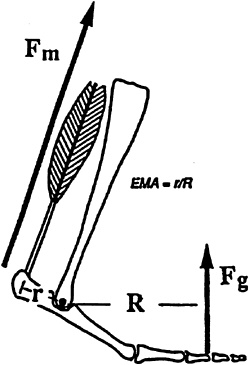
FIGURE 14-8 The effective mechanical advantage (EMA), the ratio of ground reaction force to muscle force, is determined by the ratio of the muscle moment arm (r) to the perpendicular distance at the force vector from the joint axis of rotation (R). Fm, muscle force; Fg, ground force. SOURCE: Reprinted with permission from Biewener, ''Scaling body support in mammals: Limb posture and muscle mechanics," Science 245:45–48. Copyright 1989 American Association for the Advancement of Science.
The rates at which these forces were generated resolved the mechanism underlying the fundamental energetic principles of locomotion. Both the linear increase in metabolic rate with running speed and the lower running costs of larger animals were directly accounted for by the rates at which muscular forces were generated. The establishment of this remarkable relationship accompanies the experimental evolution of a general model of how muscles are used during exercise that also may explain the metabolic costs of other activities.
ADVANTAGES AND DISADVANTAGES OF FCM TECHNOLOGY
The FCM appears to have a number of advantages compared to other techniques used to estimate ˙Mloco in the field:
-
It provides accurate estimates of the metabolic cost of both walking and running. The ability to estimate accurately the metabolic cost of both high-in-
-
tensity running and low-intensity walking is unique among ambulatory monitors. This method offers new opportunities to study activity patterns in aged, handicapped, and sedentary humans.
-
Output is in standard energy units. The linear relationship between body weight, foot contact time, and the metabolic cost of locomotion allows easy and direct calculation of the ˙Mloco in standard units (e.g., joules, kcal, watts, kcal/min).
-
FCM requires no individual calibration beyond a determination of body weight. If confirmed, this FCM characteristic should make it easier to study large groups of people.
-
FCM provides a simple means of monitoring aerobic fitness. The FCM can be used alone to estimate an individual's maximal ˙Mloco and work capacity. It also can be used in conjunction with determinations of heart rate to assess changes in relative aerobic fitness.
-
FCM is simple enough to be useful in epidemiologic studies. The FCM is simple, inexpensive, compact, lightweight, and has a low power requirement. The FCM method requires less subject cooperation than diaries and is potentially less time consuming and expensive than other techniques involving observation, diaries, activity questionnaires, and interviews (Montoye and Taylor, 1984).
Current disadvantages of the FCM are as follows:
-
The FCM does not account for energy costs of other concurrent activities such as upper body work. Humans engage in many types of physical activity. Combining the FCM with activity monitors, heart rate monitors, or questionnaires may help identify energy costs for physical activities other than walking and running (Haskell et al., 1993; Montoye and Taylor, 1984).
-
Body weight must be determined and may change over time. Total body weight is probably relatively stable over the course of a day in the general population. However, total weight can fluctuate in soldiers as equipment is donned and doffed. It is technologically possible to monitor both total weight and foot contact time.
-
Current FCM algorithms do not account for uphill or downhill movement. Research in progress suggests uphill and downhill movement can be accounted for through the changing relationship of the time the foot contacts the ground to the time the foot is in the air. As an individual moves uphill, the time of contact remains constant, but the length of time the foot is raised is reduced. The converse occurs during downhill movement.
-
Further research is needed. Studies with larger and more heterogeneous subject populations that include women, children, and the elderly are needed to establish the general validity of this method. In addition, studies are needed (1) to confirm that measurements of ˙Mloco can be made by the FCM method without complex individual calibration, (2) to quantify the effects of grade on ˙Mloco,
-
and (3) to compare ˙Mloco estimates by the FCM method with those measured by heart rate, doubly labeled water, and other methods.
AUTHORS' CONCLUSIONS AND RECOMMENDATIONS
The ability of ambulatory monitors such as the FCM to assess physiologic function in free-living humans is rapidly improving, in parallel to the marked progress in electronic sensors, microprocessors, data storage, and telemetry. These new personal status monitors will be used to answer practical scientific questions, help meet the medical needs of soldiers injured in remote locations, and provide field commanders with the data they need to prevent environmental injuries among their troops.
The FCM technology is practical, simple to use, and represents a significant addition to existing technologies. This technology should help meet the research need for detailed information on energy expenditure patterns and physical fitness of free-living humans. The cost-benefit ratio of this technology seems favorable. Each FCM should be inexpensive enough (about $50 to $100) to be useful in large-scale epidemiologic studies. Support for continued FCM technology development is needed to expedite the production and testing of FCMs.
REFERENCES
Alexander, R. McN., A.S. Jayes, G.M.O. Maloiy, and E.M. Wathuta 1981 Allometry of the leg muscles of mammals. J. Zool. (Lond.) 194:539–552.
AR 600-9 (Army Regulation 600-9) 1986 See U.S. Department of the Army.
Armstrong, R.B., and M.H. Laughlin 1985 Metabolic indicators of fibre recruitment in mammalian muscles during locomotion. J. Exp. Biol. 115:201–213.
Ästrand, P.O., and K. Rodahl 1986 Textbook of Work Physiology, Physiological Bases of Exercise, 3d ed. New York: McGraw-Hill Book Co.
Biewener, A.A. 1989 Scaling body support in mammals: Limb posture and muscle mechanics. Science 245:45–48.
Biewener, A., R.M. Alexander, and N.C. Heglund 1981 Elastic energy storage in the hopping of kangaroo rats (Dipodomys spectabilis). J. Zool. (Lond.) 195:369–383.
Blair, S.N. 1993 Evidence for success of exercise in weight loss and control. Ann. Intern. Med. 119:702–706.
Blair, S.N., H.W. Kohl, N.F. Gordon, and R.S. Paffenbarger, Jr. 1992 How much physical activity is good for health? Annu. Rev. Public Health 13:99–126.
Blickhan, R. 1989 The spring-mass model for running and hopping. J. Biomech. 22:1217–1227.
Brownell, K.D., A.A. Stunkard, and J.M. Albaun 1980 Evaluation and modification of exercise patterns in the natural environment. Am. J. Psychiatry 137:1540–1545.
Burnett, K.R., C.B. Taylor, and W.S. Agras 1985 Ambulatory computer-assisted therapy for obesity: A new frontier for behavior therapy. J. Consult. Clin. Psychol. 53:698–703.
Byers, T. 1995 Body weight and mortality [editorial]. New Engl. J. Med. 333:723–724.
Cavagna, G.A., F.P. Sabiene, and R. Margaria 1964 Mechanical work in running. J. Appl. Physiol. 19:249–256.
Cavanaugh, P.R., and K.R. Williams 1982 The effect of stride length variation on oxygen uptake during distance running. Med. Sci. Sports Exerc. 14:30–35.
Ceesay, S.M., A.M. Prentice, K.C. Day, P.R. Murgatroyd, G.R. Goldberg, and W. Scott 1989 The use of heart rate monitoring in the estimation of energy expenditure: A validation study using indirect whole-body calorimetry. Br. J. Nutr. 61:175–186.
Christensen, C.C., H.M.M. Frey, E. Foenstelien, E. Aadland, and H.E. Refsum 1983 A critical evaluation of energy expenditure estimates based on individual O2 consumption/heart rate curves and average daily heart rate. Am. J. Clin. Nutr. 37:468–472.
Clausen, J.P 1977 Effects of physical training on cardiovascular adjustments to exercise in man. Physiol. Rev. 57:779–816.
Dausch, J.G. 1992 The problem of obesity: Fundamental concepts of energy metabolism gone awry. Crit. Rev. Food Sci. Nutr. 31:271–298.
Ettinger, W.H., Jr., and R.F. Afable 1994 Physical disability from knee osteoarthritis: The role of exercise as an intervention. Med. Sci. Sports Exerc. 26:1435–1440.
Farley, C.T., and O. Gonzalez 1996 Leg stiffness and stride frequency in human running. J. Biomech. 29(2):181–186.
Farley, C.T., and T.A. McMahon 1992 Energetics of walking and running: Insights from reduced gravity. J. Appl. Physiol. 73:2709–2712.
Farley, C.T., R. Blickhan, J. Saito, and C.R. Taylor 1991 Hopping frequency in humans: A test of how springs set stride frequency in bouncing gaits. J. Appl. Physiol. 71:2127–2132.
Farley, C.T., J. Glasheen, and T.A. McMahon 1993 Running springs: Speed and animal size. J. Exp. Biol. 185:71–87.
Hakala, P. 1994 Weight reduction programmes at a rehabilitation centre and a health centre based on group counselling and individual support: Short-and long-term follow-up study . Int. J. Obes. 18:483–489.
Harris, J.A., and F.G. Benedict 1919 A biometric study of basal metabolism in man. Publication no. 279. Washington, D.C.: Carnegie Institute of Washington.
Haskell, W.L., M.C. Yee, A. Evans, and P.J. Irby 1993 Simultaneous measurement of heart rate and body motion to quantitate physical activity. Med. Sci. Sports Exerc. 25:109–115.
Heglund, N.C., and C.R. Taylor 1988 Speed, stride frequency, and energy cost per stride: How do they change with body size and gait? J. Exp. Biol. 108:429–439.
Heglund, N.C., M.A. Fedak, C.R. Taylor, and G.A. Cavagna 1982 Energetics and mechanics of terrestrial locomotion. IV. Total mechanical energy changes as a function of speed and body size in birds and mammals. J. Exp. Biol. 97:57–66.
Henneman, E., G. Somjen, and D.C. Carpenter 1965 Functional significance of cell size in spinal motoneurons. J. Neurophysiol. 28:581–598.
Hill, A.V. 1950 The dimensions of animals and their muscular dynamics. Sci. Prog. 38:209–230.
Hovell, M.F., C.R. Hofstetter, J.F. Sallis, M.J.D. Rauh, and E. Barrington 1992 Correlates of change in walking for exercise: An exploratory analysis. Res. Q. Exerc. Sport 63:425–434.
Hoyt, R.W., and A. Honig 1996 Environmental influences on body fluid balance during exercise: Altitude. Pp. 187–200 in Body Fluid Balance in Exercise and Sport, E.R. Buskirk and S.M. Puhl, eds. Boca Raton, Fla.: CRC Press, Inc.
Hoyt, R.W., J.J. Knapik, J.F. Lanza, B.H. Jones, and J.S. Staab 1994 Ambulatory foot contact monitor to estimate the metabolic cost of human locomotion. J. Appl. Physiol. 76:1818–1822.
Kappagoda, C.T., R.J. Linden, and J.P. Newell 1979 Effect of the Canadian Air Force training programme on a submaximal exercise test. Q. J. Exp. Physiol. 64:185–204.
Ker, R.F., M.B. Bennett, S.R. Bibby, R.C. Kester, and R.M. Alexander 1987 The spring in the arch of the human foot. Nature 325:147–149.
King, A.C., S.N. Blair, D.E. Bild, R.K. Dishman, P.M. Dubbert, R.H. Marcus, N.B. Oldridge, R.S. Paffenbarger, Jr., K.E. Powell, and K.K. Yeager 1992 Determinants of physical activity and interventions in adults. Med. Sci. Sports Exerc. 24:S221–S236.
Kram, R., and C.R. Taylor 1990 Energetics of running: A new perspective. Nature 346(6281):265–267.
Kraning, K.K., II 1991 A computer simulation for predicting the time course of thermal and cardiovascular responses to various combinations of heat stress, clothing and exercise. Technical Report T13-91. Natick, Mass.: U.S. Army Research Institute of Environmental Medicine.
LaPorte, R.E., H.J. Montoye, and C.J. Casperson 1985 Assessment of physical activity in epidemiologic research: Problems and prospects. Public Health Rep. 100:131–146.
Lusk, G. 1928 The Elements of the Science of Nutrition, 4th ed. New York: Academic Press. Reprint: New York: Johnson Reprint Corp., 1976.
McMahon, T.A., and G.C. Cheng 1990 The mechanics of running: How does stiffness couple with speed? J. Biomech. 23:65–78.
Meijer, G.A., K.R. Westerterp, H. Koper, and F. Ten Hoor 1989 Assessment of energy expenditure by recording heart rate and body acceleration. Med. Sci. Sports Exerc. 21:343–347.
Montoye, H.J., and H.L. Taylor 1984 Measurement of physical activity in population studies: A review. Hum. Biol. 56:195–216.
Paffenbarger, R.S., R.T. Hyde, A.L. Wing, and C. Hsieh 1986 Physical activity, all-cause mortality, and longevity of college alumni. N. Engl. J. Med. 314:605-613.
Patton, J.F., M. Murphy, T. Bidwell, R. Mello, and M. Harp 1991 Metabolic cost of military physical tasks in MOPP 0 and MOPP 4. Technical Report T95-9. Natick, Mass.: U.S. Army Research Institute of Environmental Medicine.
Perrier Study of Fitness in America 1979 Study No. S2813. New York: Louis Harris and Associates Inc.
Pollock, M.L. 1973 The quantification of endurance training programs. Exerc. Sport Sci. Rev. 1:155-188.
Powell, K.E., and R.S. Paffenbarger 1985 Workshop on epidemiologic and public health aspects of physical activity and exercise: A summary. Public Health Rep. 100:118-126.
Rome, L.C. 1992 Scaling of muscle fibers and locomotion. J. Exp. Biol. 168:243-252.
Rome, L.C., A.A. Sosnicki, and D.O. Goble 1990 Maximum velocity of shortening of three fiber types from the horse soleus: Implications for scaling with body size. J. Physiol. (Lond.) 431:173-185.
Russell, C.M., D.F. Williamson, and T. Byers 1995 Can the Year 2000 objective for reducing overweight in the United States be reached?: A simulation study of the required changes in body weight. Int. J. Obes. 19:149-153.
Saris, W.H.M. 1986 Habitual physical activity in children: Methodology and findings in health and disease. Med. Sci. Sports Exerc. 18:253-263.
Shephard, R.J. 1968 Intensity, duration and frequency of exercise as determinants of the response to a training regimen. Int. Z. Angew Physiol. 28:272-278.
Siscovick, D.S., R.E. LaPorte, and J.M. Newman 1985 The disease-specific benefits and risks of physical activity and exercise. Public Health Rep. 100:180-188.
Spurr, G.B., A.M. Prentice, P.R. Murgatroyd, G.R. Goldberg, J.C. Reina, and N.T. Christman 1988 Energy expenditure from minute-by-minute heart-rate recording: Comparison with indirect calorimetry. Am. J. Clin. Nutr. 48:552-559.
Taube, G. 1995 Epidemiology faces its limits. Science 269:164-169.
Taylor, C.R. 1992 Cost of running springs. In Physiological Adaptations in Vertebrates: Respiration, Circulation, and Metabolism, S. Wood, R. Weber, A. Hargens, and R. Millard, eds. New York: Dekker.
Taylor, C.R., K. Schmidt-Neilsen, and J.L. Raab 1970 Scaling of the energetic cost of running to body size in mammals. Am. J. Physiol. 219:1104-1107.
Taylor, C.R., A. Shkolnik, R. Dmi'el, D. Bahrav, and A. Borut 1974 Running in cheetahs, gazelles, and goats: Energy cost and limb configuration. Am. J. Physiol. 227:848-850.
Taylor, C.R., N.C. Heglund, T.A. McMahon, and T.R. Looney 1980 Energetic cost of generating muscular force during running: A comparison of large and small animals. J. Exp. Biol. 86:9-18.
Taylor, C.R., N.C. Heglund, and G.M.O. Maloiy 1982 Energetics and mechanics of terrestrial locomotion. I. Metabolic energy consumption as a function of speed and body size in birds and mammals. J. Exp. Biol. 97:1-21.
Todd, I.C., D. Wosornu, I. Stewart, and T. Wild 1992 Cardiac rehabilitation following myocardial infarction. Sports Med. 14:243-259.
U.S. Department of the Army 1986 Army Regulation 600-9. "The Army Weight Control Program." September 15, Washington, D.C.
1992 U.S. Army field manual FM 21-20. "Physical Fitness Training." September 30. Washington, D.C.
van Mechelen, W. 1992 Running injuries. A review of the epidemiological literature. Sports Med. 14:320-335.
Walmsley, B., J.A. Hodgson, and R.E. Burke 1978 Forces produced by the medial gastrocnemius and soleus muscles during locomotion in freely moving cats. J. Neurophysiol. 41:1203-1216.
Westerterp, K.R., G.A.L. Meijer, E.M.E. Janssen, W.H.M. Saris, and F. ten Hoor 1992 Long-term effect of physical activity on energy balance and body composition. Br. J. Nutr. 68:21-30.
Woledge, R.C., N.A. Curtin, and E. Homsher 1985 Energetic Aspects of Muscle Contraction. London: Academic Press.
Wright, S. 1995 Turning the other cheeks: Insights into the cost of locomotion from running backwards. Undergraduate thesis, Harvard University, Cambridge, Mass.
Young, J.C. 1995 Exercise prescription for individuals with metabolic disorders. Practical considerations. Sports Med. 19:43-54.
Zierath, J.R., and H. Wallberg-Henriksson 1992 Exercise training in obese diabetic patients. Sports Med. 14:171-189.
Zuntz, N. 1897 Über den Stoffverbauch des Hundes bei Muskelarbeit. Arch. Gesamte Physiol Menschen Tiere 68:191-211.
DISCUSSION
BERNADETTE MARRIOTT: What about arm swing in relation to motion?
REED HOYT: It is basically unaccounted for. The calibration group swung their arms, and the experimental group swung their arms as well. If they are carrying a load in their hands, the total load would be accounted for in terms of total body weight. The extra energy needed to swing that load would not be accounted for.
JOAN CONWAY: What do these devices look like?
REED HOYT: I will pass one around. The one that is coming around is just standard type technology. Under that insole, you can see the four sensitive resistors. The surface-mount technology probably could be fitted into the heel of a shoe fairly easily and, as MIT suggests, perhaps the force of walking could be used to generate some electricity with some piezoelectric stuff to get rid of the batteries.
(Laughter)
GILBERT LEVEILLE: Have you looked at telemetry of the data so that you could be manipulating [it] during the course of the experiment?
REED HOYT: The device is compatible with telemetry. In other words, there is a data output port. The way the device is configured right now, you gather the data, plug it into your computer, and download it. It is set up electronically so that you could hook a display on to it, so there could be a display on the shoe, or you could telemeter it with the appropriate telemetry feed—you would have to feed to a telemetry unit—so it is possible.
JOHN VANDERVEEN: What information does the sensor actually give you, other than the contact?
REED HOYT: That is strictly it.
JOHN VANDERVEEN: So if they are going uphill, you cannot tell versus …
REED HOYT: The uphill-downhill work is an issue. The Concord field station is working on it. The initial suggestion is that contact time does not change; the time spent with the foot in the air does change. So clearly, as you go uphill, you have a mass gravity change and an increase in potential energy, and depending on the grade, you will be more or less efficient in that movement. I think we have a shot at sorting that out as well.
DOUGLAS WILMORE: Reed, this comes out of some discussions several of us had at lunch, and it seems to me that the future is going to be to develop a set of microelectric monitors and detectors for people to tell not only supervisors when someone is in trouble or what they need, but maybe even to sense to the individual when homeostatic mechanisms may break down, for example, hydration status, energy status, and things of that sort.
Tell us how you see this evolving. Give us your thoughts about the next 10 or 15 years of development, because clearly the electronics are available and a lot of other stuff is available, too.
REED HOYT: Things are changing very quickly in terms of the electronics. The engine, the data logger, that is on this device was $500 a year and a half ago, and it is $15 now. I think in certain instances there will be a need to monitor people.
In chemical protective suit gear, the soldiers are at risk of cooking themselves. In Ranger school, they are perhaps at risk of hypothermia or hyperthermia. I see a cascade of monitoring from a personal monitor, where it will tell you when you start to become hyperthermic and give you a yellow light or a red light to drop out of whatever activity you are engaged in. That kind of monitoring could include heat production from this device as part of the puzzle.
There is keen interest, as I mentioned, within the command and even wider, to pour some money into personal monitoring. I think that as problems arise, there may be a need to then bring that to the next stage, where the team leader might be informed or the medic might be informed. Or with somebody having a problem, they could plug in by telemedicine to Walter Reed, or wherever the experienced clinicians are, to help the medic through a problem.
I think there is a lot of interest in refining that whole process, and there will be many little pieces of the puzzle, body temperature pills, foot contact monitors, canteen drinkometers, and on and on.
ROBERT NESHEIM: One of the things that occurs to me is that this technique could be used, knowing the weight of the individual and knowing the kind of work that he is going to do, to tell that individual how many calories he had to consume at that particular time during that task in order to be able to keep himself in balance.
One issue that this committee has addressed, of course, is that soldiers tend to underconsume. Maybe this might be a way of helping to get them to think about what they are eating.
REED HOYT: That is true. You can say you have so much body fat and so much body carbohydrate stores and you are going in a hole, so you need to keep pace. It has been a dilemma for us for a while.
JOHN VANDERVEEN: I was just curious. How are these systems relative to marching in water and things of that sort? Will you get them so that you can do that?
REED HOYT: Well, I think it is easy enough to make them waterproof. There has been a variety of work done on effects of substrate on energy requirements, and postholing through the snow or walking around on loose sand or walking in water up to your knees certainly is going to have an energy cost associated with it that will not necessarily be dealt with by this device. You need to know independently what the substrate conditions were like. That would be another sensor.
DOUGLAS WILMORE: But you could code that in, too. That would be a variable that you could code in.
REED HOYT: That is right, you would have a water sensor out there.
DAVID SCHNAKENBERG: I do not know if everyone in the room is aware of it, but I think that at least a year and a half ago when I left, the Army was looking at soldier modernization, soldiers of the twenty-first century.
You now are looking at a combat soldier or infantryman carrying a sophisticated data-acquisition system and a data-processing system and necessary uplinks to a satellite computer that would involve geopositioning, where he is specifically on the battlefield, sensing something about the environment, certainly detecting an infrared target and feeding that back into microoptics that he would be wearing on his helmet, to where you could have a variety of information that could be provided either automatically or on demand.
Initially it was to provide information about the environment and perhaps about his equipment and about the enemy. We always were trying to convince those that were involved in this process that it was a great opportunity to feed the soldier some information about himself.
The question comes back to: what is the short list of biosensors that we can put noninvasively on a human, preferably, that could be used to provide information with an on board algorithm to convert it into a useful tool, the red light and the green light?
REED HOYT: From the Rangers' point of view they had two problems. One was "Where am I?" So that requires a global positioning system solution, perhaps. And the other was "How do you tell the difference between an exhausted, sleep-deprived Ranger and one who is hypothermic?" So there you need some measure of body temperature in a situation where they are at risk.
DAVID DINGES: I was going to add a comment along that line. I think you have to distinguish between the technical feasibility, which, frankly, I consider to be trivial at this point—the only reason that these miniaturized devices are not
right now in systems is because the companies who have the ability to make them do not do so until they see a market for them.
The real issues are, what do you measure physiologically that is scientifically valid, and what are the countermeasures? How do you feed that information back, or what do you tell a soldier to do when he or she is imperiled? Those are the substantive scientific issues, from my perspective.
The others are engineering issues and I think Dr. Wilmore said we have the potential right now to monitor almost anything with a miniaturized capacity with technology. The substantive issues are what, on whom, with what kind of feedback to prevent what?
KARL FRIEDL: You are exactly right. We do not want to feed individual soldiers a lot of medical or physiological information, giving them heart rate, blood pressure, and core temperature, and all that, which is really meaningless and it confuses them and it turns into something they will use for contests.
(Laughter)
We are talking about piggybacking onto a system that is going into effect with global positioning and all that. That is part of the twenty-first century land warrior system. And it finally allows the medical people to say, okay, you can put some stuff on here, now what do you want on this? And nobody really knows. We have not figured out exactly how we are going to interpret that and that is the big question. You hit it right on the head.
JAMES VOGEL: Just in follow-up, I might mention something you might be interested in, that the Ranger training regimen has agreed to be a test bed for these personal monitors, if this is interesting. Probably the very first version that will be tried this coming year will include the positioning monitoring, probably heart rate and temperature, though the other day we argued for hours about what temperature to record.
The technology is available; it is a question of where you record it from and, of course, what you do with it. Of course, these personal monitors will be monitored not by the individual, probably, to begin with, but by physician assistants or the command structure, at least in the Ranger training situation.
ARTHUR ANDERSON: Will the frequencies of these signals be in a form that cannot be monitored by the adversary? I mean, would it help them to know you are afraid?
REED HOYT: They are very sensitive about being identified by electronic emanation. Certainly the radio-temperature pill, which is one way to get a core
body temperature, has a signal that goes approximately a yard and they are concerned, so with these more powerful systems you have that as a general concern.
G. RICHARD JANSEN: I guess what I am thinking is that if something can be done, it will be done, and I am thinking about information overload. I think this is what we are seeing with computers. What we see with computers is that the more the program has to do, the slower it does it, until you need to upgrade the computer. So there are limits. I think it really comes down to what is needed, because the soldier can only process, in a minute amount of time, the most important information, and there is going to be a lot of noise transmitted as we are talking here.
ROBERT NESHEIM: As Jim Vogel was pointing out, where this information goes and who is going to have the responsibility for acting on it is one of the key issues. I do not think you can have 100 individual soldiers in a platoon reacting to their own individual data. There has to be some command structure that is going to be able to control that or we are going to have chaos out there.
REED HOYT: These systems that they are setting up now are really for command and control. The early versions of the personal status monitors are probably going to be casualty assessment tools, to know who is still out there and what level of function they are at.
DOUGLAS WILMORE: … given that the physician assistant will then have a computer that information will dump into, or he can use algorithms or something like that.