8
Electric Power and Propulsion
Introduction
The panel focused on those electric power generation, storage, and propulsion technologies that, when applied as a system, will support the electrification of ships, submarines, and land-based vehicles. It surveyed the status of key elements of power systems such as energy storage devices, electric power recovery subsystems, and battery technologies. Industrial development is ongoing in most of these areas, and current trends suggest steady improvement in capacity, density, modularity, and reliability. The naval forces can take advantage of commercial developments by actively monitoring the commercial marine power and propulsion sector and by applying a top-down systems engineering process to fleet capability upgrades.
A recent naval planning document1 outlined a development path for modernizing the power and propulsion capabilities of future surface ships and submarines. The plan does the following:
- Takes a systems approach, allowing appropriate trades for optimization of performance within the constraint of affordability;
- Takes a design approach in requiring modularity, commonality, and use of commercial technology for economy of scale wherever appropriate;
- Has initiated, and is following, a development path for those basic power generation, distribution and control, and propulsion technologies that are critical for shipboard implementation;
- Has initiated a path whereby this capability can be used to backfit existing hulls in a hybrid configuration and be fully electric in new construction platforms; and
- Provides a path for developing those critical technologies and beginning the integration of both hybrid and fully electric systems into the fleet during the next decade.
The concept appears to be sound, the enabling technologies are achievable, and the opportunity is now available to initiate a major change in the generation and use of electric power aboard ship. The benefits to the naval forces could be significant and far reaching. Advances in materials used in electromagnetic machinery, such as high-field permanent magnets and high-temperature superconductors, present opportunities for substantial increases in power density and efficiency. The electric ship approach is enabled by the ongoing revolution in electronics where advancements in solid-state semiconductor technology are being applied to power semiconductor devices. The emergence of direct electric conversion technologies, such as fuel cells, offers potential for fuel-efficient, low-emission, and low-noise sources of electrical power.
Within the plan, the Department of the Navy's integrated propulsion system (IPS) architecture will allow incorporation of developing technologies such as permanent magnet (PM) electric machines, fuel cells, and the power electronic building block (PEBB) architecture into future ship designs as programmed, preplanned replacements for the core technology in the first-generation IPS modules. Use of technology-independent module interface standards will facilitate technology insertion with minimum impact on the ship design and construction process.
IPS with electric propulsion motors allows for consideration of integrated motor and propulsor concepts. The steerable podded propulsor provides the greatest potential for meeting naval hydrodynamic and hydroacoustic requirements while also being competitive with conventional propulsors on commercial ships.
The benefits of ship electrification are as follows:
- Combat systems effectiveness. Integrated electric power and propulsion systems enable design flexibility that, in turn, will facilitate the optimization of topside arrangements for maximum combat system effectiveness.
- Survivability. The elimination of gear trains and propeller shafts, together with the flexibility and modularity of electric systems, will enable graceful degradation and rapid reconfiguration of vital systems, thus enhancing survivability.
- Signature reduction and quieting. By eliminating the mechanical link between the power plant and the propulsor (i.e., propeller), electric drive will
- enable reduction of noise and vibration by allowing acoustic isolation of the engine generators.
- Improved operational flexibility and reliability. With the power station concept, all power will be supplied by a set of prime movers that provide power to propulsion, ship service, and other designated loads. This approach will provide the flexibility to shift power between propulsion, ship service, and other electrical loads, which cannot be done with a mechanical drive ship, and can enable improved speed control and steaming efficiency and the elimination of less efficient controllable pitch propellers in favor of fixed pitch types.
- Increased flexibility and adaptability. Integrating electric power and propulsion systems will provide flexibility in servicing other loads such as environmental controls (air conditioning) and launch and recovery systems, electric armor, high-power advanced electronics, and electric weapons.
- More space available. Eliminating gears and shafts from the propulsion system will make more space available for other uses. Also, the prime mover will no longer be tied to the propeller shaft line, and the power sources can be distributed throughout the ship as necessary.
- Reduced manning. Digital control and automation, which will be an integral part of ship electrification, will reduce the requirements for human operators (in machinery spaces, for example).
- Reduced logistics. Common power and propulsion modules can be used across the fleet.
- Reduced costs. Commercial technology appears likely to be available for many of the system elements.
- Life-cycle cost and fuel-consumption savings. Overall fuel efficiency can be improved if a ship is able to operate at varying speeds over a substantial part of its operational profile, and variable speed propulsion will be enabled by electric drive.
In general, the same line of reasoning that applies to surface ship power and propulsion systems also applies to submarines. Optimization of submarine systems will be driven by stealth, safety, power density, and other requirements that differentiate surface and undersea vehicles. Submarine power and propulsion system technologies of the future will include the following:
- Very low harmonic motor controllers;
- High-power-density and high-performance solid-state inverters and converters and the components thereof;
- Electrical substitutes for systems and components that now rely on fluid transport for energy and actuation, e.g., electric actuators, electromagnetic launchers, and thermoelectric coolers;
- Motors and generators with very low acoustic and magnetic signatures, including versions that can operate submerged in seawater at high pressure; and
- New technologies for motors and generators such as superconducting magnets, cryogenic coolers, current collectors, high-field permanent magnets, liquid cooling, and active noise control.
A HTS high-torque drive motor can be one-fourth the size of a permanent magnet motor, and its efficiency can be high enough to compete with existing reduction gear/steam turbine systems. HTS motors and generators can also be quieter because the high-density magnetic fields produced allow elimination of iron cores, simplification of armature designs, and elimination of other components that create noise.
The Marine Corps relies heavily on power and propulsion technology for its vehicle power systems. The requirements for higher water speed amphibious vehicles, signature reduction, adaptability to electric power, future electric weapons, and reduction of vehicle weight all lead to the need for an integrated electric power system for future land and amphibious vehicles.
Key technologies for land and amphibious vehicles mirror those described for surface ship systems but are driven by a different set of requirements. These include the following:
- Pulsed-power systems including energy storage pulse-forming networks and very high power solid-state switching;
- Flywheel energy storage;
- High-power-density engines, including electric drive systems with a unit size of several hundred horsepower; and
- Electrical steering, suspension, and actuators.
In conclusion, the panel believes that electric power science and technology holds great promise for major technical progress and payoff in the future. The remainder of this chapter is organized to first discuss electric power generation and storage technologies and then focus on the electric ship concept and associated technologies.
Electric Power Generation and Storage Technology
Introduction
Warfare in the early 21st century will involve the use of advanced technologies and combat systems with ranges, lethality, and detection capabilities surpassing anything known in contemporary warfare. In the battle zone surrounding and including the battle group and expeditionary forces, there will be requirements for electrical power at levels from tens of watts for surveillance and communication, to kilowatts for radar and for a variety of electric motors, to hundreds of kilowatts for field-base power demand and multi-megawatts for advanced
weaponry, countermeasures, and propulsion systems. Mobility of all system elements will be essential for survival and success. Therefore, although fuel supply may dominate the total system operational weight for nonnuclear power, mobile weapons and sensors should be powered by supplies that have high specific power, are compact and quiet, and have minimal signatures in the full electromagnetic and acoustic spectra.
Nonpropulsive power generation requirements and implementations will be integrated into a single system with propulsive power generation. This, and the continued deployment of special electric loads, will determine the requirements for power distribution, storage, and conditioning.
The concept of an all-electric, or more electric, ship is gaining momentum in both U.S. and foreign navies. Simply stated, this concept embodies the use of electrical means for all power needs in lieu of other means such as mechanical, pneumatic, and hydraulic. This then provides an opportunity to standardize components and systems, reduce signatures, and better integrate the power and propulsion systems for all vehicles. The move toward increased reliance on electricity is also being applied to air vehicles and to Marine Corps amphibious and land vehicles.
Versatility in electric power availability will enable new varieties of weapons and ship systems. Some proposed new weapons present especially rigorous power supply requirements. Electrothermal, chemical, and electromagnetic guns and high-powered laser or microwave directed-energy weapons, for example, require large amounts of power over very short time periods, as do electric rail aircraft launchers (electric catapults), which are technically similar to electromagnetic guns. Sophisticated power generation, distribution, and control systems will enable local-zone power distribution and conditioning, which will enable lower platform signatures and localized platform damage control. An order-of-magnitude evolution in size and power requirements for specialized sensors and unmanned systems presents new application requirements for power storage systems.
A general schematic for the type of power system addressed by the panel is presented in Figure 8.1. A prime electric power source feeds directly, or via a load-leveling or storage stage, into a conditioning stage to alter the electrical parameters for optimum reliable power distribution to a load.
The panel believes that the following three technologies will require special attention and support if the full potential of integrated electric power and propulsion systems is to be realized:
- Power generation, including advanced fuel-efficient turbogenerator power units for continuous power and for pulsed or short-duration power generation applications and direct electrochemical and electrothermal generators such as fuel cells;
- Power conditioning and distribution, including passive components, such as capacitors, inductors, and transformers, and active devices, such as power
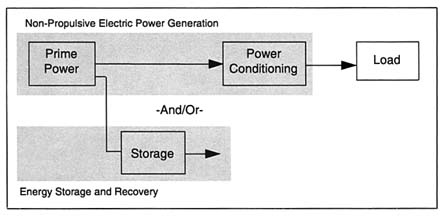
FIGURE 8.1 Schematic of a generic power and propulsion system.
- electronic switches and inverters for continuous as well as pulsed or short-duration, high-power conditioning and distribution; and
- Energy storage, including primary and secondary electrical energy storage such as batteries, flywheels, and generator combinations, and magnetic energy storage devices.
Table 8.1 lists the technology areas covered by the panel. Technology forecasts are provided in the sections that follow.
TABLE 8.1 Electric Power Technology Areas
Electric Power Generation |
Energy Storage and Recovery as Electric Power |
Nuclear-electric generators |
Primary batteries |
Turboshaft engine generators |
Rechargeable batteries |
Piston-engine generators |
Superconducting magnets |
Fuel cells |
Flywheels |
H2 |
Pumped liquids |
Other |
Compressed gases |
Explosive/magneto-hydrodynamic |
Thermal storage |
Power conditioning |
|
Bus power |
|
Slow power |
|
Fast power |
|
SOURCE: Adapted from the Board on Army Science and Technology, 1993, "Electric Power Technology for Battle Zones," STAR 21: Strategic Technologies for the Army of the Twenty-First Century, National Academy Press, Washington, D.C., Table 43-1, pp. 539–540. |
Power Generation
The concept of the electric ship is a powerful enabler for new concepts in ship design and operation. Independent placement of propulsion and power generation elements and the integrated approach to electric power distribution will rely for their efficiency on new developments in prime power generation, conditioning, and distribution.
Electric propulsion assumes continuous generation capacity that will also provide electric power for ship auxiliary functions from the most mundane application to the more exotic ones, such as catapults and electric weapons. It will often be necessary to provide auxiliary power generation for backup, or for specific subsystems, or for zonal requirements. Aircraft and launch and recovery systems, electric armor, high-power advanced electronics, and electric weapons all require pulsed-power systems whose average power for the duration of output ranges to hundreds of megawatts. The mass and volume of generators in this class are half power unit and half power conditioning. The physical decoupling of power and propulsion subsystems afforded by electric drive will be a powerful enabler for compact, versatile, and powerful future ships.
Continuous Power Generators
For nonnuclear operation even the most efficient internal combustion engines consume their own weight in fuel in about 10 hours. Therefore, fuel storage determines overall operational system weight, and fuel supply will dominate logistics considerations. For mobile electrical generators and power conditioners, substantial weight reduction can be achieved by increasing the generating and distribution frequency from the present 60-Hz standard up to the 400-Hz range.2
Of the continuous power generation systems listed in Table 8.1, some are already in use by the naval forces. Nuclear-electric generators have potential for significant advances in specific power output. Although the pressurized water nuclear power plants currently in use do not hold much promise for greatly improved power density, a new generation of high-power-density nuclear power plants could be developed but would require substantial R&D investment. A development program could build on past R&D programs that have focused on compact, high-output reactor designs.
Turboshaft engine-generators, which represent another important class of continuous power generation systems, are currently used in military helicopters and some specialized boats. They are also being incorporated into mobile electric
power units, primarily by the Army. They burn jet fuel and have rotational speeds in excess of 20,000 rpm. The high rotational speed could be used to advantage in a high-frequency, lightweight, direct-coupled electric generator operating at 24,000 rpm (400 Hz) or higher. Typically, however, they are geared down to 60-Hz alternators, and these gears increase weight and noise.
The panel evaluated the various continuous and pulsed/short-duration power generation, power-conditioning, and energy-storage technologies by creating figures of merit in the near term (year 2000) and far term (year 2020). The selection of certain technology areas for inclusion in this report was based in part on the potential to achieve high values for figures of merit in the future. The figures of merit include functionality, mobility, supportability, manpower requirements, and cost. A significant improvement in any of these figures of merit could justify adoption of the technology by the Department of the Navy.
The panel believes that, of the various technologies evaluated, turboshaft-engine-driven alternator systems have the best potential for practical, continuous improvement in all figure of merit areas. To realize this potential, the Department of the Navy should support the development of advanced turboshaft engines such as in the DOD/NASA Integrated High-Performance Turbine Engine Technology (IHPTET) program and carry out an aggressive development effort in advanced lightweight alternators, power-conditioning control systems, and unit integration and modularization.
The electric power systems driven by turboshaft engines have the best potential for evolving into compact, lightweight units with reasonable noise control. Systems with these characteristics will be required in the future, particularly for the intermediate power ranges from 50 kW up to the megawatt level. The most significant factors controlling the specific power of an integrated unit are engine rotating speeds, generator frequency, and generated voltages. To a first approximation, the physical size of an engine is proportional to torque, and increasing the speed increases the shaft power proportionately with only a modest change in weight. The weight advantage gained by high-speed operation can be lost, however, by coupling through a gear box to heavy low-frequency generators and power conditioners. The present standard of 60-Hz generators requires that either engines with speeds greater than 3,600 rpm must be geared down to 3,600 rpm or the high-frequency alternating current (ac) that is directly generated must be rectified to direct current (dc) and then converted back to 60-Hz ac with an inverter.3 Both approaches introduce weight and cost into the system.
Design optimization studies performed for the international space station suggest a different overall approach: Let the alternator rotate at the turbine speed and produce power at that frequency. Turbine speeds of 24,000 rpm or higher are
achievable and efficient. When directly coupled, 24,000 rpm yields ac power at 400 Hz, which is the current aviation standard. Using these higher frequencies for power generation can reduce the weight of the active components in the system by a factor of about six, compared with a 60-Hz system. Solid-state converters could then be used to shift the frequency to 60 Hz for distribution. Because of the ease of converting dc to any desired frequency, it is feasible to consider dc distribution in many cases.
The voltages used for power generation and distribution also have leverage with respect to high specific power. The required conductor cross section, and hence the weight, decreases as the square of the voltage, so operating voltage is an important parameter.4 The practical voltage limit (currently about 1 kV) for mobile systems is set primarily by the reliability limits of semiconductor devices. Improvements in high-voltage semiconductor devices could permit more system-optimized voltages to be used up to the Navy standard bus transmission level of 5 kV.
As the technologies evolve over the next 20 years, the specific power of high-rpm engines should approach 10 kW/kg. Similar progress is anticipated for high-rpm alternators with stationary armatures and separately excited rotating-magnetic-flux generating units. The weights of these generators are almost inversely proportional to their rotating speed (rpm). Thus, they could be designed for 400-Hz operation and directly coupled to the gearbox output shaft at 24,000 rpm. Although some special generator units have been operated at specific powers as high as 20 kilowatts of electric power output per kilogram (kWe/kg), generators built to military standards will more generally achieve 10 kWe/kg.
At high-frequency operation, power-conditioning components are small, lightweight, and highly efficient, particularly when series resonant conversion is used in converters. Advanced technology should yield systems with power densities in the range of 15 to 30 kW/kg. Power densities as high as 100 kW/kg are conceivable for power conditioners when integrated-circuit fabrication techniques are used to manufacture integrated converters.
Figure 8.2 shows how the operating time affects the total specific power (power/weight ratio) for existing and estimated future mobile electric power units. The diagonal limiting line in the figure represents the maximum specific energy available from combustion of the JP-8 fuel that will be used in the Navy's diesel engines or turbine engines. An overall efficiency of 39 percent is assumed from maximum fuel heating value to conditioned electric power. The ordinate gives the specific power based on the total system weight: kilowatt-hours divided by the weight of the total integrated system, including engine, generator, power conditioner, integration factor, and fuel consumed. For many engagements
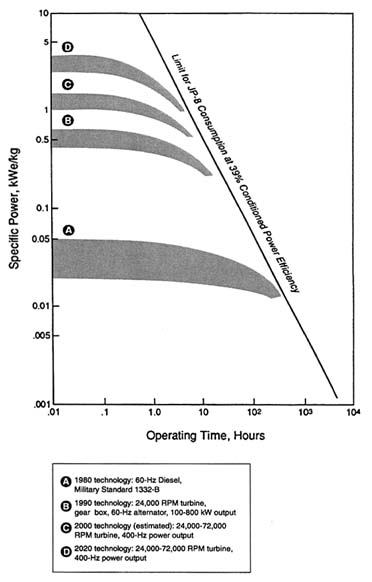
FIGURE 8.2 Specific power versus operating time for advanced turbogenerators. SOURCE: Adapted from the Board on Army Science and Technology, 1993, "Electric Power Technology for Battle Zones," STAR 21: Strategic Technologies for the Army of the Twenty-First Century, National Academy Press, Washington, D.C., Figure 43-1 p. 547.
in the future, actual operating times may be relatively short (hours to hundreds of hours). Therefore the specific power of the hardware units will be critical to mobility and initial operational capability.
Fuel cells hold promise for zonal power generation. They are a particularly efficient and clean method of utilizing hydrocarbon fuels and are appropriate for medium-sized power generation units (1 to 4 kW). Hydrogen-based fuel cells electrochemically oxidize hydrogen with oxygen (or air) and directly convert chemical energy to electric energy. The product of this combustion is water, and this combined with the extremely low signature of the cell make it a very attractive power alternative for Navy and Marine Corps operations. Because they are essentially isothermal devices and therefore not limited by heat engine thermodynamics, they can in principle have very high energy densities (watt-hours per kilogram), limited only by the specific free energy change in converting hydrogen and oxygen to water. The great obstacle to hydrogen-based fuel cells for mobile, battle-zone applications is the volumetric problem of storing hydrogen. Although hydrogen produces a great deal of energy on a weight basis, it produces very little energy on a volume basis, even when stored at high pressure.
Today hydrogen is the only fuel that can be electrochemically oxidized in a fuel-cell system at practical rates. The ideal system would directly convert the free energy of combustion of other fuels, such as hydrocarbons, into electric energy. Other fuels are used in prototype fuel-cell systems by either externally or internally reforming them to a mixture of hydrogen and carbon dioxide, then using the hydrogen electrochemically in the fuel cell. A major breakthrough in electrocatalysis would be required before other fuels could be electrochemically oxidized at useful rates.
Hydrogen fuel cells are impractical for at-sea applications because of the space required to store hydrogen, but hydrocarbon fuel conversion designs would be directly applicable to Navy use. Most units under development (primarily in the automotive industry) require higher-quality fuels than standard Navy distillates. Although there is no current evidence of an impending technical breakthrough in this area, the promise is such that near-term programs to develop converters for standard Navy fuels should be encouraged.
Pulsed-power Generators
Pulsed-power generation systems can be divided into two major subsystems as illustrated in Figure 8.3: (1) energy storage or prime-power generation, followed by (2) power conditioning. The panel considered five classes of pulsed-power generation as listed below:
- Open-cycle, combustion-driven gas turbine generators. These systems burn fuel in excess air to produce hot combustion gases, which are then expanded through a turbine to drive a generator, producing the prime power. They have
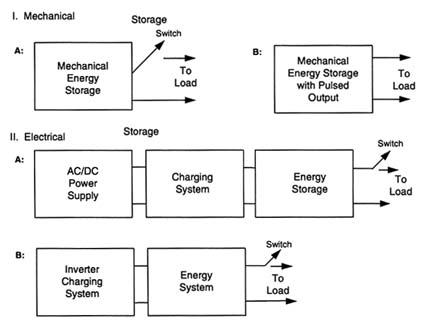
FIGURE 8.3 Alternative techniques to provide multi-megawatt prime pulse power and power conditioning. Which is chosen depends on the load to be served. SOURCE: Adapted from the Board on Army Science and Technology, 1993, ''Electric Power Technology for Battle Zones," STAR 21: Strategic Technologies for the Army of the Twenty-First Century, National Academy Press, Washington, D.C., Figure 43-3, p. 552.
- very attractive power densities, particularly for high power levels, and they are good candidates for future pulsed-power generation sources.
- Magnetohydrodynamic generator driven by solid or liquid propellants . A magnetohydrodynamic channel consists of a linear array of electrodes on either side of the inside of a rectangular duct. A magnetic field is created by permanent or electromagnets oriented parallel to the electrode faces. Ionized gas is forced through this channel, and the velocity of the gas interacting with the magnetic field creates a voltage differential at the electrodes. To produce the ionized gas in these systems, solid-propellant grains or a bipropellant liquid combination burns at elevated pressure and extremely high temperature, and the propellant is seeded with an ionizable material such as potassium and then passed through a magnetohydrodynamic channel where part of the energy in the ionized gas is directly converted to electric power.
- Explosive-driven magnetohydrodynamic generator. In this system, an explosive charge is used to drive a single-shot magnetohydrodynamic power generator. The advantage of this system is that a pulse with a very high power
- level is produced, thus avoiding much of the power-conditioning requirements of other systems.
- Flywheel energy storage and power generation. Large amounts of power can be stored in a flywheel as it is spun up. By coupling the flywheel to a generator that power can be tapped rapidly. New composite materials have greatly increased the efficiency (inertia) of flywheel systems.
- Superconducting magnet energy storage and power generation. Superconducting magnet energy storage (SMES) systems make it possible to store electrical energy directly with little loss other than the power required to cool the superconductor. Direct current can be fed into a superconducting coil to create a powerful magnetic field that stores the electrical energy. The field can then be discharged through a solid-state inverter to produce pulsed power as needed. The switching times between discharge cycles can be milliseconds, and charge and discharge cycles can differ by orders of magnitude. New superconducting materials and manufacturing technologies will provide rapid development for these devices in the future.
The panel believes that three of the five categories of energy storage or prime-power generation discussed above have broad-based applicability to naval force needs for high-energy pulsed power by the year 2020. These are (1) open-cycle, combustion-driven gas turbine systems, (2) flywheel energy storage and power generation, (3) fuel cells, (4) other direct conversion technologies, and (5) superconducting magnet storage and power generation. The panel anticipates major improvements in these systems over the next 30 years.
Open-cycle Combustion
Continuous development and improvement of open-cycle, combustion-driven gas turbine systems is expected, including systems that can use a broader range of fuel types, thus easing the logistics problem. These new systems will be much more rugged than today's turbogenerators because of the introduction of advanced materials and processing methods. Also anticipated are approaches to reducing signature of the effluent stream, thus enhancing survivability.
Flywheels
Flywheels have potential for improvement in the areas of functionality, mobility, supportability, manpower, and cost. Most of the anticipated improvements in flywheels will come from major advances in composite materials with ultra-high strength-to-weight ratios. These materials will enable stored energy density to increase by an order of magnitude over high-strength steels because they can
sustain higher angular velocities. The cost of fabricating composite flywheels with optimally tailored structural properties will decline dramatically over the next 30 years, as more military and industrial applications are found.
Fuel Cells
Unlike turbogenerator technology, practical fuel cells that run on liquid hydrocarbons do not exist today. They are certainly a possibility, however, particularly in light of the advances made in the previous decade in understanding the catalytic reforming of hydrocarbons. Research in this area with the specific goal of developing a fuel cell that would run on liquid hydrocarbons (Navy distillate) and air would be worth supporting. If a breakthrough were to occur, the implications for the Navy of the future could be enormous.
Other Direct Conversion Technologies
There are other direct electrical-conversion technologies besides fuel cells, including thermionics and thermophotovoltaics, that may become viable in the future, but probably beyond the 2010 time frame. In thermionic conversion, heat is used to create electrical flow between electrodes. In the thermophotovoltaic converter, a two-step conversion process is used with heat causing an emitter to create photons that are captured by a photovoltaic cell to produce electrical energy. These technologies will likely be introduced in cogeneration schemes such as topping cycles for gas turbines or direct heat sources such as nuclear reactors or thermoelectric nuclear devices.
Superconducting Magnet Energy Storage and Generation
Superconducting magnet energy storage (SMES) is a scalable technology, and it is reasonable to consider storage capacities ranging from less than 0.001 MW/h to more than 10,000 MW/h. The technology has received government support since the mid-1980s—for example, Air force has conducted a program that demonstrates small, economically viable SMES systems for uninterruptible power and power-quality management, and the Los Alamos National Laboratory has run a program for high-discharge-capacity storage—commercial applications have focused on the high end of the scale (such as an operating load-leveling unit at the Bonneville Power Administration in Tacoma, Washington).
To achieve naval application, continued development of materials and manufacturing methods for SMES systems is required.
Power Conditioning and Distribution
Power conditioning currently represents between 50 and 80 percent of the total mass of high-power pulsed generator systems. For future Navy and Marine Corps applications, it will be essential to greatly increase the specific power density of these power conditioners. A reduction of at least an order of magnitude in specific weight is possible before intrinsic physical, chemical, or mechanical limits are reached.5 The advances will come about primarily through new, molecularly tailored materials for passive components, advances in solid-state power electronic devices, and improved techniques for heat rejection and thermal management, such as heat pipes. High-temperature superconducting cables could increase current carrying capacity by factors of three to five times that of conventional copper cables. This factor includes parasitic cooling loads. The liquid nitrogen coolant required for HTS systems is nonflammable and would tend to extinguish any electrical fire that might arise from battle damage to the cable.
Power-conditioning systems that evolve over the next 30 years will be designed to degrade gracefully. In other words, through zonal power generation and distribution and intelligent management, single-point failures will result in only a small decrement to platform performance because the systems will be designed in a way that enables the automatic bypass of the degraded element.
Current and Near-term (Out to 10 Years) Characteristics
The three main elements of all power-conditioning systems are bus power conditioning, slow power conditioning, and fast power conditioning, as described below:
- Bus power conditioning. This technology takes the electrical feed from one of the prime power sources listed above into a conditioning stage, where the voltage level is altered for optimum, reliable power distribution to a slow power conditioning stage. The major components of this first stage of power conditioning are inverters, transformers, and rectifiers. The application of HTS technology could improve transformer efficiency and yield safer equipment by eliminating the dielectric oil that sometimes poses fire and toxicity hazards.
- Slow power conditioning. The slow power-conditioning stage produces repetitive electrical pulses in the millisecond time frame. Switches, capacitors, and transformers are the major components used in this stage of power conditioning.
- Fast power conditioning. If required for the particular application, a fast
5 |
Board on Army Science and Science and Technology. 1993. STAR 21: Strategic Technologies for the Army of the Twenty-First Century, National Academy Press, Washington, D.C. |
TABLE 8.2 Forecast of Power Conditioning Module Power and Power Density
Parameter |
Today (per system) |
Tommorrow (10 years) (per module) |
Future (30 years) (per module) |
Average power |
|
|
|
kWe/kg |
0.05 |
10 |
>100 |
kWe |
100 |
100 |
1,000 |
Pulsed power (as pulsed energy) |
|
|
|
kJ/kg |
0.3 |
1 |
>10 |
kJ |
50 |
500 |
5,000 |
SOURCE: Adapted from the Board on Army Science and Technology, 1993, "Electric Power Technology for Battle Zones," STAR 21: Strategic Technologies for the Army of the Twenty-First Century, National Academy Press, Washington, D.C., Table 43-3, p. 543. |
-
power-conditioning stage can be used to convert the pulses with a millisecond duration from the slow power-conditioning stage to pulses of a microsecond or less. This stage uses transmission lines, capacitors, switches, and magnetic devices as the primary components.
The anticipated progress in key parameters for power conditioning subsystems is indicated in Table 8.2.
Technology Forecasts
Capacitors
As naval force requirements for compact electrical power systems grow substantially over the next 30 years, the development of capacitor technology will be a major enabling technology element. For microsecond to fractional-second energy storage and discharge, capacitor technology is unequaled in the flexibility and adaptability needed to meet a broad range of future requirements.6
At present, energy conditioning at pulse repetition rates of less than 1 Hz and 10 to 30 MJ per pulse has been achieved for pulse durations from 0.05 to more than 1,000 µs. The maximum voltages decrease from megavolt levels at shorter pulse durations to tens of kilovolts at the longer pulse durations. Voltage levels are determined by the nature of the load. Innovative capacitor systems, operating at near their ultimate voltage breakdown limits, which are 10 to 15 times today's operational levels, would enable the development of lightweight systems. The
6 |
Board on Army Science and Technology. 1993. STAR 21: Strategic Technologies for the Army of the Twenty-First Century, National Academy Press, Washington, D.C. |
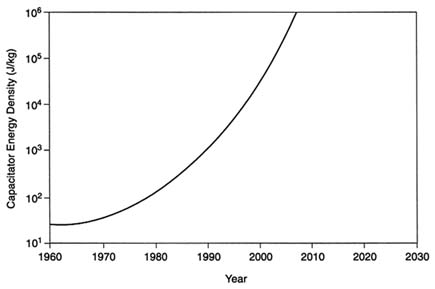
FIGURE 8.4 Projection of specific energy density for new capacitor technology.
SOURCE: Adapted from the Board on Army Science and Technology, 1993, "Electric Power Technology for Battle Zones," STAR 21: Strategic Technologies for the Army of the Twenty-First Century, National Academy Press, Washington, D.C., Figure 43-4, p. 557.
best commercial capacitors today achieve specific power densities of 1 to 10 kWe/kg and specific energy densities of 1 to 300 J/kg. Repetition rates can be as high as several kilohertz, necessitating the development of capacitor technology integrated with that of negligible-loss switching topologies and voltage-multiplication pulse transformers. Most systems can be classified by voltage according to their average power and run-time characteristics. For future naval needs (30 years out), compact systems would be enabled by capacitor energy and power densities 10 to 100 times those available today. Development of new solid and liquid materials, in conjunction with advancing methods of manufacturing technology, will likely be feasible with the use of tools emerging from current technology programs. What will be required is a tightly integrated development program for materials and components, tailored to areas of need for the three main classes of capacitor technology: polymer films, ceramics, and electrolytic capacitors.
Figure 8.4 shows recent and projected progress in capacitor energy density. Industrial capacitor developers have taken a preeminent role in the integration of materials development with the practical realization of advanced capacitors. The Department of the Navy will need to support a system-responsive, technology-based development program in each of these capacitor areas. The program goals should be demonstration hardware that will operate at the required power and energy densities.
Capacitors with specific power densities of 200 to 1,000 kWe/kg and specific energy densities of up to 20 kJ/kg may be feasible in the future. Recent discoveries have resulted in capacitors whose performance degrades gracefully and does not result in single-point failure for the system. Now the potential exists for novel capacitor technologies that offer radically different availability over the system life. Indeed, throughout the system's normal life, even in adverse environments, the failure modes of new systems will provide graceful and predictable reduction in performance, so that total system operation can be retained, although at a reduced performance level.
Table 8.3 projects the performance trends for state-of-the-art capacitor technology. It lists selected examples of several classes of advanced capacitors that further research could develop as practical, highly compact units. For large production volumes, the unit cost of these advanced units is projected to be comparable to the cost of current technology.
Inverters
The naval forces' requirement for efficiently conditioned electrical power will continue to grow dramatically over the next 30 years. Part of this requirement will come from the conditioning needed to provide a low noise—hence dc—electrical bus with the power quality, reliability, and maintainability that will keep electric-powered systems operational during warfare. Eliminating the
TABLE 8.3 Performance of State-of-the-Art and Projected Advanced Capacitor Systems
|
Energy Density (kJ/kg) |
|
Power Density (kW/kg) |
|
||
Capacitor System |
Now |
Future (2020) |
Now |
Future (2020) |
Repetition Rate (Hz) |
Critical Research Areasa |
Polymer film |
1.5 |
10 to 20 |
60 |
2,000 |
8100 |
Film impregnants, foils, &277b;150 °C, 1 MJ/unit |
Ceramic |
0.01 |
5 |
100 |
50,000 |
10,000 |
Ceramics, electrodes, >500 °C, 1 kJ/unit |
Electrolytic |
0.2 |
2 |
20 |
200 |
100 |
Electrolytes, separators, >200 °C, 10kJ/unit |
|
present single-point failure topologies that result in catastrophic failure of electrically powered assets can be accomplished by developing zonal control architectures that will be enabled by advanced inverters.7
The objective is to provide the platform with a power source that degrades gracefully when damaged during war, continuing to operate, albeit at reduced performance, so that electric-powered assets that are not directly damaged remain available for their missions. In point-failure situations, bus conditioning via bidirectional inverters would continue to supply power from generator-to-generator feed lines redundantly, isolating damaged generator members of the system and permitting load sharing under inverter control. Because no other power conditioning technology can provide this control, inverter dc bus power-conditioning is vital to the electrically powered naval forces of the future, and the Department of the Navy must ensure the development of this technology. As illustrated in Figure 8.5, up and into the early 1990s little substantive progress in cost-performance ratio was made in the power-density scaling of bus power elements for main computer systems.
Integrated, Modular Power Electronics
The Department of the Navy in partnership with industry has pursued the development of standardized, solid-state, integrated power-conditioning modules and has succeeded in achieving reductions in size and increases in efficiency, if not reduction of cost. The requirements of future weapon systems could be met through a focused development program that increases the inverter subsystem power densities by 10 to 100 times over the 0.01 to 0.2 kWe/kg of current military systems. There is a need, for example, for modular, molecularly grown, block power conditioners that are fault tolerant and whose performance degrades gracefully and for voltage management modules that are interchangeable and stackable and can be connected in parallel to create the quality of ac/dc voltages needed in the field.
Interchangeable voltage management modules would provide universal, intelligent, dc bus power conditioning, with per-module, power-break sizes that are cost effective for the Navy. Modules would probably come in sizes of 1, 10, 100, 1,000 and 10,000 kW. Power quality and electromagnetic interference management functioning would be integrated into each module. Such modules will need to integrate the passive component technologies for capacitors, inductors, and the like, with advanced power switches and intelligent control capability. A program now under way within the Department of the Navy to develop a PEBB is a step in the right direction. This effort must incorporate the best available technology into successive generations of power-conditioning systems for naval applications.
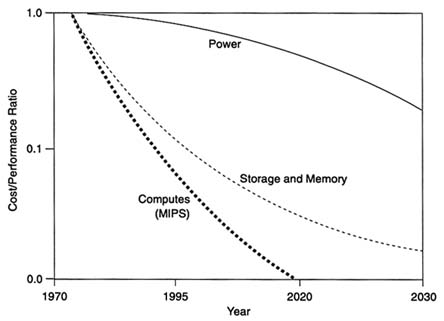
FIGURE 8.5 Technology advancement with time of major computer systems elements as a function of cost versus performance ratio. SOURCE: Adapted from the Board on Army Science and Technology, 1993, "Electric Power Technology for Battle Zones," STAR 21: Strategic Technologies for the Army of the Twenty-First Century, National Academy Press, Washington, D.C., Figure 43-5, p. 560.
The modular approach to improved power electronics will leverage commercial products for military use while reducing the effort required to develop new applications. Detailed circuit design will be unnecessary, allowing designers to concentrate on optimizing system performance.
Energy Storage and Recovery
By the year 2035, there will be a greater dependence on electric power for vehicle drives as well as for a wide array of battlefield electronics and for new electrically driven weapon systems. The reduction of the signature of power generation units will become increasingly important in the battle zone of the future, and this will likely increase the dependence of the naval forces on compact energy storage systems, either chemical or mechanical, in which the stored energy can be rapidly recovered as electric output. Electric vehicle research in the automotive industry is an important contributor to developments in long-life, high-capacity, quick-response electric energy systems and currently sustains work
in the development of reliable and highly efficient chemical and mechanical energy storage systems.
Five classes of energy storage and electric-power recovery technologies that could apply to the forces' future needs were considered by the panel, as follows:
- Batteries,
- Flywheels,
- Inductive energy storage,
- Capacitive energy storage, and
- Compressed gas or steam.
The panel concluded that of these five storage technology areas, batteries show the greatest promise for meeting near-term needs, although flywheels may provide some capability in hybrid vehicle propulsion systems. Inductive and capacitive energy storage media will require continued development to reach a level of performance to be useful in fielded systems. Compressed gas is currently used to start engines on surface ships and for ballast blow and weapons launch in submarines. Steam is used for aircraft launch on carriers. The compressed gas or steam approach is fundamentally limited and ripe for replacement by the other candidates listed above.
Batteries
The science of electrochemical energy storage is fundamentally mature. The pace of development is relatively slow, but the need for safe and reliable electric power is so pervasive that there is a constant high level of research and development. New commercial battery products find their way onto the market, as historical shortcomings in the safety and stability of materials and chemical reactions are resolved. This was the case, for instance, in the recent development of the nickel metal hydride (NiMH) battery as a replacement for the environmentally unsound nickel cadmium (NiCd) battery.
Technology development promises evolutionary progress in the realization and utility of the physical potential within the basic chemistries. Several long-term efforts in commercial and government establishments focus on improvement in shelf life, safety, volumetric and gravimetric energy values, and, in the case of secondary batteries, smart controls for charging efficiency and cycle lifetimes.
Nonrechargeable, or primary, batteries are currently used in a wide range of applications in the Navy where very low levels of electric energy (i.e., watt-hours) are required. Lithium cells with lives of 5 to 10 years are available and will undoubtedly be improved over the next 30 years. Tables 8.4, 8.5, and 8.6 summarize primary battery technology.
TABLE 8.4 Primary Cell Types With Little or No Chance of Further Development
Chemistry |
Cell Volt |
W-h/kg |
Notes |
Aluminum-Manganese Dioxide Al-MnO2 |
1.6 |
|
Unpredictable storage life; anode corrosion problems; voltage delay |
Magnesium-Air Mg-O2 |
3.0 |
|
Anode corrosion problems |
Magnesium-Bismuth OxideMg-Bi2O3 |
1.6 |
|
Voltage delay; poor, intermittent performance |
Magnesium-Sulfur Mg-S |
1.6 |
|
Voltage delay; high impedence; poor, intermittent performance; H2 S byproduct |
Zinc-Potassium Iodate Zn-KIO3 |
1.9 |
31 |
High rate; low shelf life; high reaction temperature; poor low-temperature performance |
Zinc-sodium Dichromate Zn-Na2Cr2O7 |
2.1 |
0.25 |
Anode corrosion problems; H2 products |
SOURCE: Compilation of data from Bis, R.F., J.A. Barnes, W.V. Zajac, P.B. Davis, and R.M. Murphy, 1986, Safety Characteristics of Lithium Primary and Secondary Battery Systems, NSWC TR 86-296, Naval Surface Weapons Center, Silver Spring, Md., July; and Bis, R.F., and R.M. Murphy, 1986, Safety Characteristics of Non-lithium Battery Systems, NSWC TR 86-302 Rev. 1, Naval Surface Weapons Center, Silver Spring, Md., July. |
TABLE 8.5 Primary Cell Types with Promise as Prospective Power Sources
Chemistry |
Cell Volt |
W-h/kg |
Notes |
Aluminum-Silver Dioxide Al-Ag2O2 |
1.57 |
|
Pumped electrolyte; heat exchanger required |
Calcium-Thionyl Chloride Ca-SOCl2 |
3.0 |
|
Increased tolerance to abuse over Li |
Calcium-Sulfuryl Chloride Ca-SO2Cl2 |
3.2 |
|
Increased tolerance to abuse over Li |
SOURCE: Compilation of data from Bis, R.F., J.A. Barnes, W.V. Zajac, P.B. Davis, and R.M. Murphy, 1986, Safety Characteristics of Lithium Primary and Secondary Battery Systems, NSWC TR 86-296, Naval Surface Weapons Center, Silver Spring, Md., July; and Bis, R.F., and R.M. Murphy, 1986, Safety Characteristics of Non-lithium Battery Systems, NSWC TR 86-302 Rev. 1, Naval Surface Weapons Center, Silver Spring, Md., July. |
TABLE 8.6 Primary Cell Types Currently Available
Chemistry |
Cell Volt |
W-h/kg |
Notes |
Cadmium-Mercuric Oxide Cd-HgO |
0.9 |
|
Long shelf life; sealable |
Calcium-Calcium Chromate (thermal) Ca-CaCrO4 |
3 |
|
Long shelf life; high voltage; 1 s to 1 h operating life; tolerates extreme environments |
Magnesium-Lead Chloride Mg-PbCl2 |
1.1 |
|
Seawater activited: torpedoes; emergency signaling; sonobuoys |
Magnesium-Manganese Dioxide Mg-MnO2 |
1.8 |
|
Transceivers; sonobuoys; beacons; good temperature performance |
Magnesium-Silver Chloride Mg-AgCl |
1.7 |
|
Seawater activated |
Zinc-Air Zn-O2 |
1.4 |
440 |
High capacity |
Zinc Manganese Dioxide (Leclanché) Zn-MnO2 |
1.5 |
|
|
Zinc-Carbon Zn-C |
3 |
105 |
Everyday flashlight cell |
Alkaline Zn-MnO2 |
1.25 |
100 |
|
Mercury Zn-HgO |
1.3 |
105 |
|
Zinc-Silver Chloride Zn-AgCl |
1.0 |
2.1 |
Extremely long shelf storage: low rate supply |
Zinc-Silver Oxide (Silver Zinc) Zn-Ag2O(2) |
1.6/1.8 |
50 to 200 |
High energy/high rate: torpedo; space; submarine; ignition; rocket |
SOURCE: Compilation of data from Bis, R.F., J.A. Barnes, W.V. Zajac, P.B. Davis, and R.M. Murphy, 1986, Safety Characteristics of Lithium Primary and Secondary Battery Systems, NSWC TR 86-296, Naval Surface Weapons Center, Silver Spring, Md., July; and Bis, R.F., and R.M. Murphy, 1986, Safety Characteristics of Non-lithium Battery Systems, NSWC TR 86-302 Rev. 1, Naval Surface Weapons Center, Silver Spring, Md., July. |
Rechargeable battery systems capable of a large number of charging and discharging cycles will play a much greater role in the Navy and Marine Corps of the future. A significant part of future electric power requirements during combat engagements can come from advanced battery systems. Tables 8.7, 8.8, and 8.9 summarize secondary battery technology.
TABLE 8.7 Secondary Cell Types with Little or No Chance of Further Development
Chemistry |
Cell Volt |
W-h/kg |
Notes |
Cadmium-Silver ChlorideCd-AgCl |
1.0 |
|
Very fast charging; fast storage loss |
Zinc-Lead DioxideZn-PbO2 |
2.5 |
108 |
Anode corrosion problems; poor charging performance |
SOURCE: Compilation of data from Bis, R.F., J.A. Barnes, W.V. Zajac, P.B. Davis, and R.M. Murphy, 1986, Safety Characteristics of Lithium Primary and Secondary Battery Systems, NSWC TR 86-296, Naval Surface Weapons Center, Silver Spring, Md., July; and Bis, R.F., and R.M. Murphy, 1986, Safety Characteristics of Non-lithium Battery Systems, NSWC TR 86-302 Rev. 1, Naval Surface Weapons Center, Silver Spring, Md., July. |
As the ships and aircraft of the future incorporate more electronics, they will require lightweight, reliable primary power sources and backup systems. This need has spurred interest in further development of thermal batteries that are designed to use the high thermal energy of combustion or an explosion to generate electricity for a very short period of time. Current thermal systems are characterized by nearly unlimited shelf life because of their nonvolatile chemistries and short-term, high-energy power output. They are currently in use in tactical missiles and short-term power backup systems. Longer operating life will enable their use in cruise missiles and sonobuoys.
Where weight is a major concern or the application calls for both long shelf and operating lives, lithium-based chemistries are a clear leader.8 Primary lithium batteries with 20-year shelf lives are in current use in smart mines and kinetic kill weapons. The U.S. Army has been a leader in the development of high-density, high-power, 6- to 12-V battery power systems for vehicles, pulsed-power weapons, remote power backup, and missile systems.9 The utility of high-power systems that are capable of operating in severe environments is driving further research on lithium battery safety issues such as handling and mitigation of volatile chemistry systems.
Nickel-hydrogen has long been the preferred spaceborne secondary battery technology. Capable of high discharge rates and nearly infinite charge-discharge cycling, nothing currently matches its reliability. Underwater applications have been dominated by silver zinc (AgZn) systems. Some silver aluminum units are in use for lower power applications such as small torpedoes and the smaller
TABLE 8.8 Secondary Cell Types with Promise as Prospective Power Sources
Chemistry |
Cell Volt |
W-h/Kg |
Safety |
Aluminum-Air Al-O2 |
1.5 to 2.1 |
80 |
Seawater activated |
Cadmium-Air Cd-O2 |
1.9 |
<440 |
High cost |
Hydrogen-Air LaNi5H6-O2 |
1.2 |
3,650 theoretical; 380 actual |
Gas cross-leakage; short cycling life; low rate |
Iron-Air Fe-O2 |
1.2 |
715 theoretical; 90 actual |
Electric vehicle (EV); H2, O2 production, poor thermal operation |
Iron-Chromium (redox) Fe-Cr |
1.2 |
120 theoretical; 30 actual |
Reactant cross-diffusion problems |
Nickel-Iron Fe-NiOOH |
1.3 |
263 theoretical |
EV; long life; high H2 |
Iron-Silver Oxide Fe-Ag2O(2) |
1.2/1.5 |
106 |
To improve Zn-Ag2O(2) life |
Sodium-Sulfur (glass) Na-S |
1.8 |
760 theoretical |
High temperature (350 °C) |
Zinc-Bromine Zn-Br2 |
1.8 |
80 |
EV; electric load leveling; Br release danger |
Zinc-Chlorine Zn-Cl2 |
2.1 |
826 theoretical; 200+ actual |
EV; electric load leveling; chilled recharge required |
Zinc-Nickel Zn-NiOOH |
1.5 |
345 theoretical; 81 actual |
EV; H2, O2 production |
Zinc-Silver Oxide Zn-Ag2O(2) |
1.8/1.6 |
130 |
Torpedoes, submarines, and so on |
Zinc-Air Zn-O2 |
1.2 |
185 |
High-capacity replacement for Ni-MH |
SOURCE: Compilation of data from Bis, R.F., J.A. Barnes, W.V. Zajac, P.B. Davis, and R.M. Murphy, 1986, Safety Characteristics of Lithium Primary and Secondary Battery Systems, NSWC TR 86-296, Naval Surface Weapons Center, Silver Spring, Md., July; and Bis, R.F., and R.M, Murphy, 1986, Safety Characteristics of Non-lithium Battery Systems , NSWC TR 86-302 Rev. 1, Naval Surface Weapons Center, Silver Spring, Md., July. |
TABLE 8.9 Secondary Cell Types Currently Available
Chemistry |
Cell Volt |
W-hr/kg |
Notes |
Nickel-Cadmium (NiCd) Cd-NiOOH |
1.2 |
25/35/210 |
Cyl/button/vented; long life; memory problems |
Nickel-Metal Hydride Ni-MH |
1.2 |
65 |
Replaces Cd in NiCd |
Cadmium-Silver Oxide Cd-Ag2O(2) |
1.4 |
75 |
Like NiCd but lower rate |
Hydrogen-Nickel H2-NiOOH |
2.5 |
50 |
Pressure vessel; extremely long life; satellites |
Hydrogen-Silver H2-Ag2O(2) |
1.4/1.1 |
80 |
Pressure vessel; extremely long life; deep discharge |
Lithium-Thionyl Chloride Li-SOCl2 |
3.4 |
700 |
Primaries available as buttons; very wide temperature range; 20-year life |
Lithium Ion Family |
|
|
|
Lithium-Sulfur Dioxide Li-SO2 |
2.8 |
275 |
Wide temperature range; 20-year life |
Lithium-Manganese Dioxide Li-MnO2 |
3.1 |
300 |
|
Lithium-Titanium Sulfide Li-TiS2 |
3.6 |
|
|
Lithium-Manganese Disulfide Li-MnS2 |
3.6 |
|
|
Lithium-Sulfur Dioxide Li-SO2 |
2.8 |
275 |
Wide temperature range; 20-year life |
Lithium Carbon Monofluoride Li-(CF)n |
2.8 |
310 |
Long shelf life |
Lithium-Iodine Li-I2 |
2.7 |
230 |
Long service life (>10 years) |
Lithium-Copper Sufide Li-CuS |
|
|
|
Lead-Acid Pb-PbO2 |
|
100 |
Fuses; torpedoes; radio sonobuoys |
(sulfuric acid) |
2.0 |
|
|
(fluoroboric) |
1.8 |
|
|
(perchloric) |
1.9 |
|
|
Zinc-Silver Oxide Zn-Ag2O(2) |
|
|
|
Zinc-Manganese Dioxide Zn-MnO2 |
1.2 |
|
"Renewal" brand; needs smart charger |
SOURCE: Compilation of data from Bis, R.F., J.A. Barnes. W.V. Zajac, P.B. Davis, and R.M. Murphy, 1986, Safety Characteristics of Lithium Primary and Secondary Battery Systems, NSWC TR 86-296, Naval Surface Weapons Center, Silver Spring, Md., July; and Bis, R.F., and R.M. Murphy, 1986, Safety Characteristics of Non-lithium Battery Systems, NSWC TR 86-302 Rev. 1, Naval Surface Weapons Center, Silver Spring, Md., July. |
off board vehicles, but AgZn batteries are widely used for high-power applications. These can provide up to 100 kW over 15 minutes and, together with advanced electric motors, can propel a torpedo at high speed.
Anticipated Technology Advances
The maturity of research in electrochemical power systems provides the benefit of some measure of predictability of future developments. There is nothing that currently matches the high power densities of vented nickel-cadmium batteries to provide the starting power for an aircraft engine in -40 °C weather, and there will not likely be a shift from conventional lead acid and NiCd batteries in this application for the next 10 or 15 years. New aircraft designs, including the F-22, are incorporating existing battery systems. Sealed NiCds are slowly being developed for these applications and are in limited flight use in the commercial sector, but they have yet to demonstrate the high power densities and low maintenance levels of the mainline systems. And, only slightly further in the future, NiMH developments show great promise for increased power densities and lower cost.
Most battery chemical reactions are bidirectional and can be implemented in either primary or secondary cell designs. Those chosen for today's array of secondary battery types are those for which the characteristics of the charge/discharge cycle are the most benign. Whether for these more natural secondary batteries or for tomorrow's more critical designs, positive control over the battery's operational status and charging parameters is becoming a critical enabling technology. The recent commercial availability of rechargeable alkaline batteries is a case in point.
Battery charger technology has advanced markedly in recent years.10 Propelled by the adoption of the more exotic commercial batteries such as lithium cells, the smart charger is becoming ubiquitous in laptop computers, for instance. Most of the problems associated with electrochemical cell development have to do with their susceptibility to anode corrosion, the production of explosive or noxious gases during charging, and the many deleterious effects of overcharging. Built-in sensors for determining temperature, pressure, along with state of charge, and microcontrollers built into the battery effectively control most of these effects and consequently improve battery life, performance, reliability, and safety. Cooperative strategies between battery controllers and the host system controller can optimize selected system/battery performance parameters such as individual cell control, load shedding, or temporary capacity reduction in all or part of the host.
For advanced rechargeable battery systems, significant improvements in mobility and supportability will derive from improvements in the energy density of new batteries by as much as fivefold over current state-of-the-art lead-acid
systems. Most batteries today operate at specific gravimetric capacities well below their theoretical limits. Significant improvements in battery operation can be afforded by active management techniques such as charge and discharge rate management within instantaneous chemistry, temperature, and load conditions. In addition to these operational improvements, fundamental research continues in the materials and structure of alloys, separator materials, and seals that will inevitably produce tomorrow's breakthroughs in battery size, output, and efficiency. A factor of two to three improvement over lead-acid batteries is predicted for power density. To be applicable to the needs of the naval forces, devices in research today must evolve into rugged, fully reliable batteries. Table 8.10 presents some parameters of interest for several rechargeable battery concepts.
It will be important that the Department of the Navy focus its development efforts with the objective of developing, at most, a few battery systems that will cover the broad range of future Navy and Marine Corps needs. Attention to the standardization of form factors, interfaces, and charging and handling systems will be important for reducing the logistics burden associated with widespread battery usage. For example, of the 500 battery types available to U.S. Army quartermasters, probably the world's largest battery user, 10 types represent about 90 percent of usage. Consolidation and rationalization of the mix of battery types and applications are ongoing. Military expenditures on batteries have grown from approximately $50 million is 1981 to over $190 million today.
Special Application of Batteries: Power for Remote Sensors
Future naval forces will depend on remote sensors in a variety of situations: Unmanned vehicles will patrol the skies; microbuoys will monitor the seas; target-sensitive triggers will proliferate throughout the arsenal; randomly deployable sensors will locate and monitor enemy command and control; and the marines will cast off miniature, self-forming communications networks as they advance through the littoral. These capabilities will result from the size reduction and increased functionality of microelectromechanical systems and small, long-lived, power sources. The implications for the direction of battery power evolution are clear: higher density; higher efficiency, and intelligent power management.11
Sensor Types
Airborne Sensors
Much battle space awareness information will be derived from sensors mounted on unmanned aerial vehicles (UAVs). Increased capability aboard these
TABLE 8.10 Performance of State-of-the-Art and Advanced Battery Systems
|
W-h/kg |
|
W/kg |
|
W-h/liter |
|
$/kW-h |
|
|
Battery System |
Now (to 2000) |
Future (2020) |
Now (to 2000) |
Future (2020) |
Now (to 2000) |
Future (2020) |
Now (to 2000) |
Future (2020) |
Problems |
State-of-art lead-acid |
35 |
40 |
110 |
150 |
80 |
100 |
150 |
100 |
Low energy density, fairly high self-discharge |
Bipolar lead acid |
38 |
50 |
— |
79 |
— |
85 |
— |
100 |
Costs more than conventional lead-acid, low specific power |
Na/S |
90 |
120 |
110 |
140 |
140 |
180 |
1,000 |
100 |
Operates at 350 °C |
Ni/Cd |
35 |
40 to 50 |
50 to 60 |
80 to 90 |
80 |
90 to 100 |
250 to 300 |
200 |
Sensitivity to overcharge |
Zn/Air |
90 |
140 |
100 |
150 |
— |
— |
— |
35 to 45 |
System weight dominated by elctrolyte weight |
|
Low energy efficiency |
||||||||
AL-Li/FeS |
80 |
150 to 200 |
95 |
180 to 220 |
— |
— |
High |
100 |
Operates at 400 °C |
SOURCE: Board on Army Science and Technology. 1993. "Electric Power Technology for Battle Zones," STAR 21: Strategic Technologies for the Army of the Twenty-First Century, National Academy Press, Washington, D.C., Table 43-5, p. 571. |
space-limited vehicles will pay dividends in the reach and effeciency of the fleet sensor suite. Miniaturization and modularity will allow rapid tailoring of these vehicles for a variety of jobs at hand, from microclimatology to target detection and location, to battle damage assessment.
Microbuoys and Unmanned Undersea Vehicles
The same technology that will empower the UAV as an inexpensive and versatile sensor platform will also enable the creation of seaborne platforms with specialized capabilities for use in the future. Deployed fleets of these robots will be capable of underwater communications, underwater intelligence actions, anti-submarine warfare, mine detection and destruction, oceanography, and seabed mapping, particularly in the littoral waters where operation with conventional submarines is problematic.
Smart Triggers
As smart weapons gain autonomy the on-board sensor and control electronics requirements will grow. As autonomous capability becomes more robust and reliable, smart triggers will be deployed on a wider variety of weapons including mines and antimine devices, long-dwell ASW robots, and long-range bombardment projectiles.
Sensor Deployments
Autonomous Sensors
High-energy, low discharge rate batteries extend available operating times, thus enabling more autonomous operation of sensor systems. Current systems are occasionally fielded for special-purpose remote sensing with electronics packages that are as large as a suitcase and with a battery suite that may be two or three times larger than that. Although the number, capability, and support requirements for smaller, more portable designs will enable a new range of remote sensor possibilities, these new sensors will also require the enhanced computational capabilities of microelectronic processors and validated, robust control programs.
Networked Sensors
A clear enabler of networked cooperative sensors is the development of reliable, very low power command and control and data exfiltration mechanisms. Microelectronic transceivers will be the key to the development of self-forming communications networks. Current commercial technology has deployed medium-bandwidth,
shoebox-sized, self-forming, and self-managing RF communications networks. These digital networks provide convenient access to mobile users. Each network component knows or can calculate its own location and locate its nearest neighbors dynamically. It is an easy extrapolation of this concept to the military battle space in the form of randomly deployable sensors or network nodes. Microelectronics and efficient power will enable miniaturization and disposability.
Stationary and Vehicle Flywheels
Flywheels are covered briefly in a previous section as energy-generation devices. Flywheels coupled directly to a variable-speed motor-generator have the potential of compactly storing large amounts of energy and rapidly delivering electric power. The motor-generator would function as a generator when the system was drawing on the energy stored in the flywheel and as a motor when energy was being stored in a flywheel by spinning it up. For many configurations, flywheels could also be spun up mechanically through the use of reciprocating or turboshaft engines. Through the use of advanced composite materials, flywheels could store as much as an order of magnitude more energy per unit of flywheel mass than can be obtained with flywheels of the best alloy steel.
The government-industry partnerships addressing hybrid electric drive for wheeled or tracked vehicles have produced innovative flywheel and motor-generator technology at the few tens of kilowatt level. U.S. Army-supported programs have developed technology for rotating inertial energy for tracked-vehicle, pulsed-power weapons applications that, although not successful to date, may have future applications to both ships and amphibious or land vehicles.
Inductive Energy Storage
Inductive energy storage, generally for use with pulsed-duty applications, has been evolving over the last decade. Cryogenically cooled aluminum inductors have been developed for low-loss, very-short-term energy storage in pulse-forming networks. SMES as described above was developed for use in high-power, directed-energy weapons applications but has evolved into applications for long-term energy storage for uninterruptible power sources. The key technologies for SMES development in the future are superconducting materials and high-strength composite materials for containment of the large forces associated with magnetic energy storage. Trends in these areas would indicate that practical superconductors operating above 100 K and composite materials with yield strengths approaching 106 psi should be available 20 to 30 years hence.
The Electric Ship
The Navy is engaged in an ongoing effort to develop an electric ship. When all the key technologies are sufficiently developed to allow the electric power generation and distribution system to be integrated with the electric propulsor, the resulting capability will represent a major change in the performance features and operating characteristics of shipboard power and propulsion systems.
The Electric Ship Concept
The electric ship approach is enabled by the so-called second electronic revolution where the rapid advancements in solid-state semiconductor technology that led to integrated circuits, microprocessors, and computers are now being applied to power semiconductor devices. Advances in the materials used in electromagnetic machinery such as permanent magnets and high-temperature superconductors also present opportunities for substantial increases in power density and efficiency. And the emergence of direct electric conversion technologies such as fuel cells provides the potential for fuel-efficient, low-emission, and low-noise sources of electrical power.
The Navy's IPS program utilizes an open-architecture, common-module approach that builds on existing commercial efforts. Within the IPS program, a family of modules is being defined that will serve as the building blocks for designing, procuring, and supporting marine power systems applicable across a broad range of ship types. To this end, shipboard power systems are divided into the following seven elements or module types:
- Power generation,
- Energy storage,
- Power conversion,
- Power distribution,
- Propulsion motors,
- Platform loads, and
- Power control.
IPS is a flexible and scalable power system by virtue of its open architecture and use of common machinery modules. It has the potential to revolutionize the design, construction, and operation of U.S. naval ships by using electricity as the primary shipboard energy medium. IPS minimizes the number of prime movers by permitting any generating unit to supply either propulsion or ship service power to support ship operational priorities at any given time. The flexibility of electric power transmission allows power generation modules of up to 20 MW to be located independently from the propulsion motors and ship service power converters in whatever arrangement supports the ship's mission at the lowest
overall cost. The ability to independently position the minimum amount of machinery components in small, unmanned modules avoids the need for large engine rooms, which in turn permits greater separation and compartmentation in the ship, accruing significant benefits in manning, safety, and ship survivability. The use of small, unmanned machinery modules also permits the use of non-chlorofluorocarbon (CFC)-based fire suppression systems similar to those currently used in fleet propulsion gas turbine enclosures.
The Department of the Navy's full-scale advanced development (FSAD) system prototype incorporates a commercial marine approach to electric power generation and propulsion distribution in combination with a zonal dc electrical distribution system for ship service power. The generator is a conventional two-pole 60-Hz machine that produces the required 22-MW output power at 4,160 volts alternating current (Vac). The power is distributed in this form via conventional medium-voltage cable and switchgear to the propulsion converter that produces the voltage and frequency required by the propulsion motor. The converter consists of a diode-rectifier front end and multiple pulse-width-modulated (PWM) inverter sections connected in parallel to achieve the desired power levels. The inverter section development extends the state of the art in insulated gate bipolar transistor (IGBT)-based PWM technology to the medium-voltage range. The motor is a squirrel cage induction motor based on severe-duty commercial marine and industrial designs. The ship service dc power is produced by a conventional transformer and rectifier and distributed via dc cable and converted by ship service inverter modules (SSIMs) to 60 or 400 Hz, or variable frequency and voltage as required.
The IPS architecture will allow incorporation of developing technologies such as PM electric machines, fuel cells, and PEBB into future ship designs as programmed, preplanned replacements for the core technology in the first-generation IPS modules. Use of technology-independent module interface standards will facilitate technology insertion with minimum impact on the ship design and construction process. Figure 8.6 is a diagram of the basic IPS architecture.
Power Generation for Surface Ships
Intercooled Recuperated Gas Turbine
The intercooled recuperated (ICR) gas turbine currently under development is a derivative of the Rolls-Royce RB211 aeroengine used in air fleets around the world. Three elements have been added to the core engine to produce its 29,000 brake-horsepower (bhp) rating and 30 percent total mission fuel savings (relative to comparable existing power plants). First, an intercooler cools the gas after low-pressure compression and before second-stage compression. This reduces compressor work, allowing more power from a given engine core. This is the key to attaining the 29,000 bhp from the RB211, and it also adds to overall engine
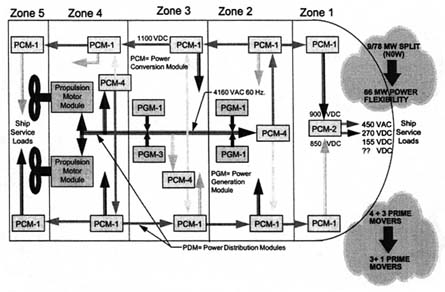
FIGURE 8.6 Integrated power system basic architecture. SOURCE: Adapted from (1) Advanced Surface Machinery Programs Office, 1996, ''A Strategy Paper on Power for U.S. Navy Surface Ships," draft, Naval Sea Systems Command, Arlington, Va.,Figure A-1, April 8, and (2) Krolick, C.F., 1996, "Technology Insertion," in the briefing"Advanced Surface Machinery Programs (ASMP)," presented to the Panel on Technology by the Naval Sea Systems Command, Arlington, Va., May 2.
efficiency. Next, a recuperator recovers exhaust heat wasted by simple-cycle engines to preheat combustion air before ignition. Finally, variable area nozzles on the first stage of the power turbine restrict flow when operated at less than full power, raising exhaust temperature and thereby increasing recuperator effectiveness at low power. The ICR has a lower exhaust temperature than comparable engines, resulting in reduced IR signature.
Although the ICR maintains the footprint of the engines it is designed to replace, it weighs roughly twice as much and costs about $1.5 million more per engine. Recent studies on fleet introduction in the DDG-51 (forward-fit) indicate an average follow-on ship acquisition increase of $10 million per ship (including engine costs) while saving $1.5 million per ship per year in fuel costs. Studies for a two-engine surface combatant indicate that, in a new design, the fuel load and intake/exhaust reductions compared with those in simple-cycle engines more than offset the increased engine weight. The net result is that even with an increase in engine cost the overall ship acquisition cost is the same, with fuel cost savings comparable to those for the DDG-51.
The current development schedule (incorporating the recuperator recovery plan) supports introduction on the DDG-51 during FY 2001, with 3,000-hour
qualification and shock testing in FY 1998–1999. The ICR is the engine incorporated into the first IPS power generation module (PGM-1), which is envisioned to be the main power unit of the future fleet.
Advanced-cycle Gas Turbines
In addition to the 29,000 bhp ICR, advanced-cycle gas turbines that are derivatives of existing commercial engines can be incorporated into turbine-generator sets in the 1- to 4-MW power range. Examples of these are the Solar Taurus-R and Allison 501 K34 ICR. The alternatives employ various combinations of recuperation, intercooling, and variable geometry to achieve substantial improvements in fuel efficiency over currently available engines—which includes the Navy's Allison 501 K34. It is anticipated that these developmental engines would cost more than their simple-cycle counterparts, and feasibility would have to be based on comparison of fuel savings versus development, fleet-introduction, and acquisition costs. For some of these engines, the improved fuel efficiency comes at the expense of response time. Current ship service generator set voltage and frequency specifications require transient-response characteristics that these advanced turbine generator sets may not be able to meet without the addition of solid-state power conversion equipment on the output side. The IPS electrical distribution interface specification has the potential for relaxing engine transient response requirements, allowing realization of fuel savings without additional equipment size or cost. As with the large ICR, recuperation will reduce IR signature, and the higher efficiency should lead to emissions reductions.
Since each core engine is unique, these engines would not constitute a modular family. Development and qualification costs on the order of $100 million dictate selection of the single rating best matched to the range of fleet requirements. Typical development times for these engines is about 5 years from contract award to delivery of production engines.
Medium-/High-speed Diesels
There are a number of existing families of medium- to high-speed diesel generator sets that cover the 1- to 4-MW power range. Typically, these generator sets are substantially more efficient than simple-cycle gas turbines and often less expensive. Although they take up comparable space as gas turbines and have much lower intake/exhaust air requirements, they are much heavier and require more acoustic treatment (mufflers, isolation mounts), which adds to ship size and cost. Although poor emissions have been attributed to the diesel in the past, microprocessor-based control and monitoring systems are being employed in modern diesel engines to improve performance and reduce emissions.
Within a given family, diesels are somewhat modular in that they are based
on multiples of a particular cylinder size. Since these are typically existing commercial engines, members of a family could be qualified as needed to support the range of fleet requirements. For example, the CAT 3512 diesel generator set is being considered for qualification for submarine emergency generator applications. The qualification effort is estimated to cost approximately $5 million and should take about 2 1/2 years.
Fuel Cells
Fuel cells convert chemical energy directly into electrical energy and as a power generation module can be viewed as a continuously fueled battery. They take in fuel and oxidant and produce electricity, water, and heat. In addition to the fuel cell stacks, the power generation module includes heat exchangers, regulators, controls, and fuel-processing and other auxiliary equipment. Besides enhanced energy density and efficiency, fuel cells have the potential for providing power with little or no emission of regulated pollutants. Other attributes include low-noise, reduced-exhaust IR signature, improved reliability and maintainability, and a high degree of modularity. Table 8.11 provides a summary of characteristics for the alternative fuel cell technologies being considered. It is anticipated that when fully matured, fuel cell costs could be as low as $500/kW.
Most of the fuel cell development to date has been focused on electric utility applications that use natural gas for fuel and for which compactness is not required. Recent developments include transportation applications using methanol as fuel and where power density is an issue. For shipboard use, a diesel fuel reformer and desulfurizer would be required, and, although power density is an issue, it must be weighed against impact on acquisition cost—the further removed from commercial practice, the more expensive it will become. Existing Navy exploratory development efforts are focused on the fuel-processing issues of reforming and desulfurization with a 10-kW fuel cell demonstration planned. DARPA is supporting development of fuel cells that operate on logistic fuels (JP8 or DF2).
Although the fuel cell stacks are very modular, the fuel processor and auxiliary equipment will benefit from economics of scale. Therefore, a family of ratings is likely (e.g., 500 kW, 1 MW, 2 MW, 4 MW) that uses multiples of a common stack element with appropriately rated fuel processor and auxiliaries. Commercial stacks and auxiliaries might be used with a fuel processor designed to meet ship requirements. This might take about 3 1/2 years and cost roughly $11 million to develop.
Integrated Power System Family of Modules
At present the IPS notional family of modules includes the following power generation modules (PGMs):
TABLE 8.11 Available Fuel Cell Systems
Type |
Electrolyte |
Operating Temperature (°F) |
Efficiency |
lb/kW |
ft3/kW |
Phosphoric acid |
Phosphoric acid (liquid) |
350 to 450 |
38 to 40 |
30 to 46 |
0.93 to 1.5 |
Molten carbonate |
Carbonate salts (liquid) |
1,200 to 1,400 |
40 to 55 |
40 to 60 |
0.98 to 2.1 |
Solid oxide (tubular) |
Zirconium oxide (solid) |
1,700 to 1,900 |
45 to 60 |
20 to 30 |
0.6 to 1.2 |
Solid oxide (planar) |
Zirconium oxide (solid) |
1,700 to 1,900 |
45 to 60 |
8 to 13.5 |
0.3 to 0.81 |
Proton exchange membrane |
Sulfonated polymer (solid) |
180 to 250 |
39 to 42 |
6 to 11.9 |
0.19 to 0.3 |
SOURCE: Adapted from the Advanced Surface Machinery Programs Office, 1996, "A Strategy Paper on Power for U.S. Navy Surface Ships," draft, Naval Sea Systems Command, Arlington, Va., Table A-1, p. A-7, April 8. |
- PGM-1—21 MW (29 KHP) 4,160 Vac, three-phase, 60-Hz ICR gas turbine-driven generator;
- PGM-2—3.75 MW 4,160 Vac, three-phase, 60-Hz diesel-driven generator;.
- PGM-3—3 MW 4,160 Vac, three-phase, 60-Hz DDA501-K34 gas turbine-driven generator;
- PGM-4—TBD MW efficient auxiliary gas turbine-driven generator;
- PGM-5—TBD MW fuel cell;
- PGM-6—8 MW 4,160 Vac, three-phase, 60-Hz diesel-driven generator; and
- PGM-7—12 MW 4,160 Vac, three-phase, 60-Hz diesel-driven generator.
The IPS architecture does not preclude configurations that use combinations of these PGMs for providing electrical power, but not propulsion, e.g., gas-turbine or steam-turbine (fossil or nuclear fueled) driving propellers via reduction gears. Much of the benefit of IPS is in power integration and optimization of installed and operating power sources. Determination of the set of future PGMs must take into account all of the attributes of the alternatives and how those attributes match up with the overall fleet requirements, as well as the power system architecture. In an ICR/IPS-based fleet, fuel efficiency of the lower rated PGMs may not have as much leverage as in today's ships. Acquisition cost and the maintenance and manning aspects of operations and support costs may be the big drivers. Emissions quality will become increasingly important, as will arrangement flexibility to facilitate integration into low-observable platforms. Also, logistics support considerations may dictate selection of the module(s) that covers the widest range of requirements versus those that optimize for each requirement.
Power Distribution and Conversion
Distribution
As indicated in the IPS discussion, a dc zonal electrical distribution system (dc ZEDS) is envisioned as the ship service power architecture for future surface combatants. It employs the dual port/starboard bus configuration used in the ac ZEDS introduced on the DDG-51 in FY 1994, but with dc buses replacing the ac buses, and integrated power conversion centers (IPCCs) replacing the load centers in each zone. The IPCCs contain power conversion modules that convert dc power to the type required by the loads in the respective zones. On existing ships this is 450 or 120 Vac, at 60 or 400 Hz, but in the future there is opportunity to provide dc directly to the end users. For many users, particularly the combat system loads, the various forms of ac power are immediately rectified to dc for internal distribution within the cabinets, and the availability of a dc feed would eliminate the internal conversion equipment and also simplify the power interface.
Many of the auxiliary systems are connected to the electrical distribution system via electric motors and their associated controllers. As the size and cost of solid-state motor controllers continue to fall, they will begin to replace the existing electromechanical devices in ship applications. It will be possible to provide a dc feed to these solid-state controllers and to any power supplies on the auxiliary skid for monitoring and control systems or sensors.
A study on dc power for combat systems on the DDG-51 indicated potential for reductions of internal power supplies totaling 17 ton and 400 ft3. A comparison of dc zonal systems with partial load integration (dc feeds to some auxiliary equipment only) to the ac zonal systems on the DDG-51 showed a potential savings of 35 tons and $1.1 million. In addition to these cost and weight savings, dc zonal systems provide enhanced survivability in that the IPCCs prevent damage or faults occurring in one zone from propagating electrically to other zones.
Power Electronics
A major factor in pursuing the more electric architecture for Navy ships is the anticipation of major advances in the state of the art of power electronics much as has occurred in microelectronics. Power electronic devices, like microelectronic devices, are semiconductor devices that switch electrical currents on and off. Microelectronic devices switch very small currents at low power levels to control the flow of information. Power electronic devices switch large currents at high power levels to control, distribute, and process electrical power. Compared with traditional electromechanical switches, they are faster, smaller, more precise, easier to control, less expensive, and more efficient. Improved performance will be spurred by development of the n-type metal oxide semiconductor (MOS)-controlled thyristor (n-MCT), an advanced power semiconductor device,and the PEBB, a multichip microsystem enabled by the n-MCT.
The PEBB will integrate within a single unit all the elements required for generalized power processing and will replace many single-application, multi-component power control circuits with a single device that delivers digitally synthesized power. This advanced power transformer will be a three-port device—two ports for power and one port for control—with bidirectional power flow converting power from one type to another based on the control algorithm being executed within the PEBB and the information available via the control port. The PEBB family will constitute a standard set of snap-together parts that start at the semiconductor-chip level and build up to the system level while integrating intelligence at various levels for custom performance—a power electronic analog of a microprocessor.
A PEBB consists of an array of power electronics switches, gate drivers, sensors, a microcontroller, filters, a fiber-optic control interface, and advanced packaging. Although PEBBs could be constructed using virtually any semiconductor device, the n-MCT meets the broadest range of requirements for military
and commercial users. Its principal advantage is its capability for simultaneous high-speed switching (&227b; 70 kHz), high withstand voltage (&227b; 1,400 V), low on-state voltage (< 2 V), and high currents (up to 1,000 amps). High-speed switching reduces the size of the input and output filters by greatly increasing the ripple frequency. For many applications, the size of the filters can be reduced to such an extent that they can be integrated directly into the PEBB package. Thus, by reducing the size of the components with high-speed switching, reducing conduction losses with low on-state voltage n-MCTs, and reducing switching losses with soft-switching topologies, it is possible to design compact and highly efficient power conditioning equipment. For an IPS ship, which employs a significant amount of power electronics, the PEBB can reduce ship acquisition cost by $4.4 million, not taking into account any effect on the ship resulting from reduced equipment size and weight.
The ONR-sponsored PEBB program uses hybrid, integrated circuit, power-semiconductor, and power-electronic technologies to design, construct, and demonstrate modular power electronics architectures for intelligent, distributed power processing. The PEBBs will be manufactured on high-volume production lines to demonstrate reliability and techniques for low-cost production. Affordability and commercial viability will be critical to the success of the PEBB. Therefore, concurrent engineering that takes manufacturing issues and customer feedback into account early in the design process and fast introduction of commercial products are integral parts of the development program. ONR's long-range plans, supported by a science program, include the insertion of advanced controls such as neural networks and artificial intelligence and the replacement of silicon with wide-bandgap semiconductor materials such as silicon carbide to increase the operating temperature and voltage of the PEBB.
Several generations of PEBBs are envisioned with each proceeding through the definition, development, and demonstration cycle. Three iterations, or generations, are planned in the 5-year program, with each generation building upon lessons learned in the previous cycles. First-and second-generation PEBBs could be employed in preprototypes of the dc ZEDS power converters and, if cost effective, could be considered for forward-fit into the ac ZEDS on the DDG-51. Third-generation devices would be available in time for production power conversion equipment for the SC-21. When available, the higher-voltage devices would be considered for propulsion power converters and for medium-voltage solid-state switchgear.
Pulse-power Distribution
This distribution provides for the generation, conversion, and transmission of prime power for high-energy pulsed loads such as electrothermal chemical (ETC) guns, electromagnetic (EM) launchers, and EM armor. In general, prime power refers to the first-step energy conversion from fuel to a form of electrical
energy that is best for transmission to the point of use. At the user end, this electrical energy is converted as required to a pulse or series of pulses tailored to the application. The most likely application for surface combatants is the ETC gun that employs a pulse-forming network (PFN) at the user end, near the gun mount, to minimize impedance in the very high current pulse cable. The PFN stores electrical energy before each shot and then discharges a pulse with characteristics specific to the gun type. Assuming capacitor-based PFNs, the various combinations of muzzle energy and repetition rate used with the ETC gun alternatives of interest all translate to roughly the same prime power requirement of 2 MW. Thus a nominal 2-MW prime power module or set of modules could be developed independent of which gun alternatives are selected.
A notional system would consist of a 13.8-kVac generator, a rectifier, and a 15-kilovolts direct current (kVdc) power cable. If the main engines are available as the prime power source, the generator could be attached to the output shaft or main reduction gear via a power-takeoff (PTO) gearbox. For IPS, the PTO, generator, and rectifier would probably be submodules within a PGM. If for some reason the main engines are not available, a stand-alone pulse prime power PGM could be developed that would include the engine, gearbox, generator, and rectifier and would add a flywheel off the PTO gear for energy storage. The IPS architecture also supports the alternative of a transformer/rectifier either as a submodule within the PGM or as a separate power conversion module. Fuel cell-based PGMs could eliminate the engine, generator, gearbox and rectifier but would require a step-up converter to provide 15 kVdc.
The IPS program is addressing the pulse power load requirement under its systems engineering efforts by using computer simulations to estimate the capacity that can be derived from the 4160 Vac distribution subsystem (PDM-1).
Propulsion Motors
The squirrel cage induction motor being produced under the IPS FSAD contract represents the state of the art in commercial ac-drive motor technology for power levels associated with ship propulsion. PM motor technology is currently competing with the commercial induction machine for lower-power-level, adjustable speed-drive applications based on higher efficiency or greater power density, depending on the needs of the application. The potential for increased power density without significant increase in cost makes PM motors attractive for ship applications where there may be some size constraints, such as small waterplane area twin hull (SWATH) or trimaran hullforms or podded propulsors. Even in-hull arrangements can benefit from reduced size for smaller-displacement ships as indicated by the projected $5.6 million acquisition cost savings for substituting the PM motor for the induction motor in the Advanced Surface Machinery Program (ASMP) surface combatant studies. In parallel with the FSAD contract, the IPS programs is developing a PM motor module in the power
range suitable for ship propulsion. This propulsion motor module will conform to the IPS interface specifications and standards and will serve as a demonstration of technology insertion in an open-architecture system.
Other machinery technology under development both by the military and commercial sectors includes homopolar motors and superconducting machines. Although the two concepts are usually linked together, homopolar motors can be excited by either conventional or superconducting magnets, and superconducting magnets can be used in both homopolar and ac motors. As with the PM motors, homopolar and superconducting motors would ideally be developed to conform to the IPS interface specifications and standards. The IPS architecture allows for extension of the specifications and standards if warranted either by commercial demand or by critical Navy-unique requirements (e.g., acoustics).
IPS with electric propulsion motors allows consideration of integrated motor/propulsor concepts. The advanced modular propulsor, for example, incorporates steering into a propulsion module that replaces the existing appendage arrangement of exposed shafting, struts, and rudder. The goal of the modular propulsor program is to define a module or family of modules based on a common set of parts that can provide the propulsion requirements for Navy and commercial ships. Affordability, which is the key to commercial viability, must include equipment acquisition and operating costs, propulsor hydrodynamic efficiency, and ship effects such as producibility enhancement and revenue-earning capacity of any space freed up by moving the motor and shaftline outside the hull. The hydrodynamic efficiency issue is complicated because Navy surface combatants must be designed for 30-plus knots maximum speed, whereas they spend most of their time operating at cruise speeds of one-half to two-thirds of maximum. For Navy applications, there is the additional consideration of hydroacoustic performance—for this effort the requirement was to be at least as good as today's surface combatants (e.g., DDG-51).
The steerable podded propulsor provides the greatest potential for meeting Navy hydrodynamic and hydroacoustic requirements while also being competitive with conventional propulsors on commercial ships. Past Department of the Navy R&D efforts on podded propulsors have adopted both improved efficiency and noise reduction as design objectives. Model tests on a 1/20th-scale DD-963 resulted in 10 to 20 percent reduction in fuel consumption. Later efforts focused on significant reductions in both propulsor and propulsion machinery noise and developed full-scale pod conceptual designs based on liquid-cooled synchronous motors driving counterrotating and postswirl propellers via epicyclic gears. Scale-model tests were conducted with geometrically similar pods on a scale model of the PG-100 (an intermediate-scale demonstration using the PG-100 was planned, but not completed). Results confirmed hydrodynamic performance projections. Also, as part of the same overall program, scale counterrotating propellers were tested in open water in the Navy's large cavitation channel, with results confirming the projections for significant increase in cavitation inception speed over fleet
propellers. Most recently, ASMP has been tracking the progress of Kvaerner Masa/ABB on their Azipod joint commercial venture. The Azipod is a steerable pod housing an electric motor driving an external propeller and is being marketed on the basis of reduced ship construction costs and improved ship maneuvering and has been demonstrated up to the 15-W power level for an icebreaker application. Counterrotating versions of the Azipod design are planned. This is a prime example of an opportunity for the Navy to leverage a commercial development.
The objective of the current modular propulsor effort was to identify technology options for providing a family of cost-effective modular propulsors for military and commercial use. The approach was to conduct a survey of future commercial and military ships and then develop a strategy for producing a common family of standardized propulsor modules. Based on the performance and affordability criteria provided by the Navy, the steerable pod propulsor emerged as the preferred concept. It was noted, however, that if reduction in propulsor noise under all operating conditions were required, then ducted propulsors (both in-hull and external configurations) should be considered. It is anticipated that motor developments under the IPS program will support pod-mounted configurations; therefore, the modular propulsor effort will concentrate on the rest of the equipment needed to produce the steerable pod module. This would include a full-scale, at-sea demonstration of propulsor performance.
Power Control
Standard Monitoring Control System
The standard monitoring and control system (SMCS) will integrate the sensing, transmission, interpretation, and display of hull, mechanical, and electric (HM&E) parameters necessary for machinery control, condition monitoring and assessment, signature management, and damage control. The system design is consistent with the total-ship integrated command and control (IC2) architecture and supports and enhances the proposed integrated survivability management system (ISMS) and the integrated condition assessment system (ICAS).
SMCS offers the potential to reduce acquisition costs and to introduce a standard system for application across multiple platforms, taking advantage of open systems architectures and commercial industry standards. It will also provide the necessary architecture to support critical imperatives for embedded readiness assessment, mission planning and training, and condition-based maintenance.
The IPS system monitoring and control subsystem consists of the software necessary to implement power management, fault response, and system human-computer interface. This subsystem is composed of system-level control software (PCON-1) and of zonal-level control software (PCON-2). The system monitoring and control subsystem is assumed to reside on a computational and
networking infrastructure that is external to IPS. Currently, this external infrastructure is SMCS compliant. Designing the IPS control software to be independent of the host hardware as much as possible should enable the exploitation of future advances in computers and networks.
Intelligent Ship Control
Support of reduced manning on future ships requires safety critical automation systems that can be trusted to operate reliably under all conditions, including battle damage. They must possess sufficient system integrity and fault tolerance to enable significant reduction in the ship's crew. The SMCS provides an affordable, modular, distributed, open-architecture, machinery-monitoring and control system based on commercial and dual-use standards and technology, but requires enhanced automation, integration, system integrity, and fault tolerance to satisfy future affordability and manning goals.
ONR has an ongoing program to develop and demonstrate the required integrated ship control (ISC) automation technology base. This automation will support both low manned and advanced machinery ship concepts. ISC will fully support highly integrated ship operation and vital combat system support within emerging U.S. Navy dual-use and open-architecture standards for communications and computer resources.
Future Impact on the Navy
The Advanced Surface Machinery Program employs a systems engineering approach that maintains flexibility and minimizes investment until technologies are demonstrated, evaluated, and brought together for optimum total-ship cost-effectiveness. Affordability assessments have been made of individual program elements, but it is in the context of the full set of ASMP elements, integrated first as a total machinery system and further as part of the total-ship concept, that the most significant gains are achieved. Figure 8.7 indicates the system improvements and expected fuel savings.
These fuel savings are based on equal shaft power requirements and do not take into account any change in hull drag that may result from resizing the ship. ASMP has conducted affordability assessments that take into account ship impact costs as well as component cost and ship producibility effects as part of overall ship acquisition cost. Operating and support costs included manning, maintenance, and training in addition to fuel.
ASMP systems engineering has followed a design policy of common modules and standard components and interfaces and is developing modules to perform each of the critical functions of naval ship machinery systems. A family of modules is being defined that meets the range of projected fleet requirements at minimum fleet life-cycle cost. It employs a common module interface standard
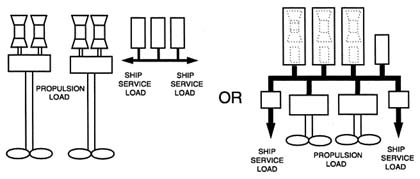
FIGURE 8.7 Integrated power system (right) advantage: Operators can determine how best to use the power, and the system saves 15 to 19 percent fuel in gas turbine surface combatants. SOURCE: Adapted from Krolick, C.F., 1996, ''Advanced Surface Machinery Programs (ASMP)," presented to the Panel on Technology by the Naval Sea Systems Command, Arlington, Va., May 2.
to facilitate rapid and affordable introduction of new technology, as driven by industrial market forces and future warfighting requirements.
Although the current emphasis is on reducing the cost of meeting existing levels of performance, it is also necessary to assess the ability to deliver enhanced performance as required to meet new threats. For example, the fuel efficiency advantages of ICR/IPS can provide operational flexibility for a notional eastern Mediterranean transit and station-keeping mission by increasing transit speed by 7 knots, increasing days on station by 9 days, or increasing gauge by 1,100 nautical miles, while keeping fuel load constant compared with the baseline.
Developments Needed
A concurrent engineering approach is necessary that identifies modular-technology building blocks that can be used in various combinations to cover the range of possible requirement sets and then engages a development process with three overlapping phases for those modules to minimize risk and financial exposure. Development of test hardware and systems and component technologies at less than full scale would constitute the first phase. This would provide the technical basis for definition of the complete set of modules needed to support the range of projected fleet requirements, including the many alternatives being considered for the next-generation surface combatant. Application of the systems engineering process would lead to identification of a small subset that would actually be developed and demonstrated in the second phase. These would be selected on the basis of minimizing the risk of producing ship equipment that would meet some as yet unspecified requirements during the full-scale engineered development phase.
Time Scale for Development/Deployment
ASMP has recognized the need to get products into the fleet at the earliest opportunity. The goal of rapid deployment can sometimes conflict with the systems engineering approach of optimizing the total-ship design. There are two ways to address this dilemma. Designers can anticipate where technology is headed and plan for the phased introduction of advanced components as they become available. In addition, the use of open architecture and modular designs will facilitate the insertion of technology upgrades. ASMP has followed this approach with introduction of the zonal electrical distribution and SMCS architectures in DDG-51 class ships. The zonal electrical system required no technology development, and the SMCS may wind up using only the architecture, software, and local level hardware from the advanced development model (ADM) by taking advantage of its openness in choice of supervisory-level host hardware. Figure 8.8 provides a schedule of the ASMP products and their fleet transition possibilities.
IPS Architecture and Core Technology
The IPS architecture and core technology have their maximum impact on new-design ships and would be hampered in forward-fit applications by the extensive nonrecurring engineering costs and limited opportunity for taking advantage of arrangement flexibility. The first surface combatant new design opportunity is the SC-21 (FY 2003 leadership award). Completion of FSAD will enable
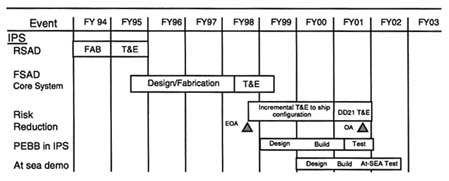
FIGURE 8.8 Integrated propulsion system. SOURCE: Adapted from (1) Advanced Surface Machinery Programs Office, 1996, "A Strategy Paper on Power for U.S. Navy Surface Ships," draft, Naval Sea Systems Command, Arlington, Va., Figure A-1,April 8, and (2) Krolick, C.F., 1996, "Advanced Surface Machinery Programs (ASMP)," presented to the Panel on Technology by the Naval Sea Systems Command, Arlington, Va., May 2.
introduction of IPS in noncombatant applications in FY 1998, which would lessen the fleet introduction cost and logistics support burden on the SC-21 class.
Introduction of IPS on aviation platforms will follow a two-step approach that straddles the SC-21 time frame. Although the ultimate objective is to provide a full-up IPS configuration for the CVX (FY 2006 award) that addresses all potential electrical loads including electric propulsion and electromagnetic catapult, the near-term CVN-77 (FY 2002 award) presents a transitional platform for introduction of a partial IPS configuration that addresses ship-service electrical loads only. The upfront systems engineering will define the CVX IPS configuration and identify those features that can be cost effectively demonstrated on CVN-77. Maximum utilization will be made of IPS SC-21 modules that will minimize development and fleet introduction and support costs and lead to identification of any additional modules needed to meet specific CVX requirements. These new modules would then be developed and qualified under the appropriate R&D program. For the large-deck amphibious ship (LHX) application, IPS offers arrangement flexibility for locating machinery relative to the well deck and engine operational flexibility, important when ship-service load is a large fraction of total installed power. Again, maximum reuse will be made of modules from previous ship applications.
Power Generation
The ICR gas turbine and its derivative, power generation module-1 (PGM-1) is envisioned as the main power unit for the IPS fleet starting with the SC-21. Selection of technology for any lower-power-rated PGMs for application to SC-21 will be driven by the requirements that emerge from the SC-21 cost and operational-effectiveness analysis (COEA) process. While these requirements are being finalized and the selection criteria developed and prioritized, it will be necessary to establish a technology base for the competitive selection process.
Commercial medium- to high-speed diesels are readily available in the power range of interest and could be deployed in the FY 2003 time frame. In any case, IPS diesel-based PGMs are likely to be identified to support the commercial end of the applications spectrum.
Advanced cycle gas turbines in the 1- to 4-MW range with fuel efficiency appreciably better than the existing DDA-501 are not commercially available at this time, although some commercial and DOE funding is going into their development. The technology exists and adequate time may be available to develop and qualify a new Navy engine from a commercial core in time for SC-21, assuming that a requirement emerges in the next year or so. The present ASMP strategy is to initiate militarization of a commercially available engine starting in FY 2000, based on the overall fleet requirements at that time.
Fuel cell developments are being supported with commercial and government funding, but largely for utility or land transportation applications that do not
use Navy distillate as fuel. The Navy should continue to ensure demonstration of the fuel-processing system at a scale adequate to level the playing field with the alternative technologies in time of the SC-21 selection process. Also, if commercial or other government-funded efforts will not provide a full-scale stack with the characteristics required by naval force applications in time for demonstration with the fuel processor, then the Department of the Navy should consider investing in accelerating development of the stack. As fuel cell technology matures, it may achieve power density and costs that make it competitive as a larger-power-rated PGM as early as the 2010 time frame.
Other direct electrical conversion technologies such as thermionics and thermophotovoltaics will begin to emerge in the 2010 time frame as part of a cogeneration scheme using conventional engines.
Power Distribution/Conversion
Direct current zonal electric distribution systems, as with IPS in general, will have the greatest impact in a new design, but the power-conversion equipment can be approached in a way that allows their introduction in the ac ZEDS on new-construction DDG-51 and/or as part of the partial IPS configuration demonstrated in CVN-77. As part of this early introduction of power conversion equipment, first- or second-generation PEBB devices may be used. This will be driven by commercial viability of these early PEBB products that will encourage investment in production capacity. The dc ZEDS for SC-21 and CVX will be fully integrated with the auxiliary and combat system equipment, and generation-three or -four PEBBs will likely be employed in the power conversion equipment. By 2010, the PEBB will be fully utilized in the implementation of an autonomic dc ZEDS. Generation-five to generation-seven PEBBs will include high-voltage applications such as solid-state switchgear for the IPS 4160V bus or for the rectifiers and inverters for a higher voltage dc bus.
Pulse-power Systems
These systems could support back-fit, forward-fit, and new design, since the ETC gun concepts conform to existing weapons modules. However, gun and associated prime power system development has been delayed such that the SC-21 now appears to be the earliest target for these systems. Pulse loads are more likely for the CVX. By 2010, high-voltage, pulse-duty PEBBs could be employed in the pulse-power rectifiers.
Propulsion Motor
Although IPS core technology can support existing ships, the PM motor and a PEBB-based propulsion power converter are candidates for the SC-21, particularly
in the lower displacement variants where power density has a greater value. Steerable pod propulsors are also viable for SC 21, most likely in conjunction with PM motor technology. Homopolar and superconducting motor technology will be available by 2010, if commercial development of high-Tc superconducting machinery is successful or if Navy-unique critical requirements (e.g., acoustics) drive their development.
Power and Propulsion
For Submarines
Future submarine power and propulsion technology will inevitably move toward increased reliance on electricity. Advanced dc power distribution using high-speed, turbine-driven rectified alternators feeding solid-state inverters through a dc distribution bus is already targeted to the new nuclear-powered submarine (SSN). Electric propulsion offers an affordable opportunity to enable new propulsor concepts that can improve signature. Studies over the past few years indicate that integration of the electric-propulsion and ship-service power-generation functions at the prime mover can result in reduced ship size and displacement because of fewer power plant components and improvements in their arrangement. Studies have also shown that eliminating centralized hydraulic systems and transporting energy electrically to the point of use will result in up to 70 tons of weight savings and potential cost reduction. In the future, this concept might be extended to other systems, such as heating, ventilation, and air conditioning.
In general, power and propulsion technologies for surface ships are also applicable to submarines. However, the optimization of submarine systems will be driven by stealth, safety, power density, and other requirements that differentiate surface and undersea vehicles.
The technologies that may be required for submarine power and propulsion systems of the future will include the following:
- Very low harmonic motor controllers;
- High-power-density, high-performance, solid-state inverters and converters and the components thereof;
- Motors and generators with very low acoustic and magnetic signature, including versions that can operate submerged in seawater at high pressure;
- New technologies for motors and generators such as superconducting magnets, cryogenic coolers, current collectors, high-field permanent magnets, liquid cooling, and active noise control; and
- Electrical substitutes for systems and components that now rely on fluid transport for energy and actuation, including electric actuators, electromagnetic launchers, and thermoelectric coolers.
For Amphibious and Land Vehicles
The Marine Corps relies heavily on power and propulsion technology for its vehicle power systems. The mandates for higher-water-speed amphibious vehicles, signature reduction, adaptability to electric power, future weapons, and reduced vehicle weight all lead to the need for an integrated electric power system for future land and amphibious vehicles.
Key drivers will be the following:
- Electric propulsion to allow the prime mover/generator to supply power to both water and land propulsion trains;
- Electric power distribution and control to direct and condition power for propulsion, weapons, and sensors as required; and
- Electric power generation and energy storage to allow operation of critical systems with reduced IR and other signatures.
Key technologies, as listed below, mirror those described above in this report for the surface-ship systems but are driven by the land and amphibious requirements:
- Pulsed-power systems including energy storage pulse-forming networks and very high power solid-state switching;
- Flywheel energy storage;
- High-power density engines;
- Electrical steering, suspension, and actuators; and
- High power density electric drives at the unit size of several hundred horsepower.
For Air Vehicles
The trend toward increased reliance on electricity is also evident in recent technical developments directed toward future aircraft.12 The drivers toward a more electric aircraft include the following:
- Reduction in aircraft weight;
- Improved survivability and reliability;
- Reduced maintenance;
- Reduced cost;
- Higher fuel efficiency; and
- Reduced need for ground-support equipment.
One solution to these drivers is to replace hydraulic, pneumatic, and mechanical aircraft systems with electrical functional equivalents and to provide the means to supply the increased electrical load (while reducing other engine loads). The key technologies that support the development of more electric aircraft mirror those that enable the electric ship. In most cases, however, the electrical power levels in aircraft systems are an order of magnitude less than those in a typical ship. Key technical developments for more electric aircraft power and propulsion include the following:
- Combined electric starter/generator for direct engine mounting;
- Smart, fault-tolerant electric power distribution and control;
- High-temperature-capable (˜500 °C), solid-state power switches for engine-mounted electronics and power distribution control elements; and
- High-temperature-capable electrically powered actuators.
Recommendations
The panel examined a wide range of technologies, trends, and the Department of the Navy drivers that will push the development of new technologies in the future. It concluded that a focus on technologies that enable higher power density and more efficient and more effective propulsion, power generation, and distribution in all naval vehicles will be necessary to ensure future performance and affordability. The general areas of electrical power science and technology, and technology that will enable the efficient conversion of fuel to electrical power, hold great promise for major technical progress and payoff in the future. The Department of the Navy should place a high priority on the development of new all-electric ships with the associated drive, power-conditioning, and distribution systems. Investment in the following areas is warranted:
- Increasing switching speed, current, voltage, and operating temperature of solid-state power switching devices;
- Solid-state power controllers that are adaptable to multiple uses, modular, and compatible with the commercial market;
- Electrically driven substitutes for mechanically driven, hydraulic, pneumatic, or steam-driven actuators;
- Means for rapidly detecting and controlling the state of complex power distribution systems;
- Electric motor and generator technologies that increase power and torque density, reduce acoustic and electromagnetic signature, operate at elevated temperatures, have higher efficiency, and/or allow operation submerged in seawater;
- High-energy-density means for both long- and short-term electrical energy storage;
- Means for high-efficiency direct conversion of fuel to electric power;
- Higher-speed, more efficient engines that increase power density while reducing fuel consumption; and
- Higher-efficiency devices at the user end of the power system where the impact of efficiency is greatest (e.g., counterrotating propulsors).