Proc. Natl. Acad. Sci. USA
Vol. 94, pp. 8284–8291, August 1997
Colloquium Paper
This paper was presented at a colloquium entitled “Carbon Dioxide and Climate Change,” organized by Charles D. Keeling, held November 13–15, 1995, at the National Academy of Sciences, Irvine, CA.
Potential responses of soil organic carbon to global environmental change
S USAN E. T RUMBORE
Department of Earth System Science, University of California, Irvine, CA 92697–3100
ABSTRACT Recent improvements in our understanding of the dynamics of soil carbon have shown that 20–40% of the approximately 1,500 Pg of C stored as organic matter in the upper meter of soils has turnover times of centuries or less. This fast-cycling organic matter is largely comprised of undecomposed plant material and hydrolyzable components associated with mineral surfaces. Turnover times of fast-cycling carbon vary with climate and vegetation, and range from <20 years at low latitudes to >60 years at high latitudes. The amount and turnover time of C in passive soil carbon pools (organic matter strongly stabilized on mineral surfaces with turnover times of millennia and longer) depend on factors like soil maturity and mineralogy, which, in turn, reflect long-term climate conditions. Transient sources or sinks in terrestrial carbon pools result from the time lag between photosynthetic uptake of CO2 by plants and the subsequent return of C to the atmosphere through plant, heterotrophic, and microbial respiration. Differential responses of primary production and respiration to climate change or ecosystem fertilization have the potential to cause significant interrannual to decadal imbalances in terrestrial C storage and release. Rates of carbon storage and release in recently disturbed ecosystems can be much larger than rates in more mature ecosystems. Changes in disturbance frequency and regime resulting from future climate change may be more important than equilibrium responses in determining the carbon balance of terrestrial ecosystems.
Soil carbon inventories and turnover rates are influenced by climate, vegetation, parent material, topography, and time, the fundamental state factors outlined by Jenny (1 , 2). Studies attempting to understand the influence of a specific factor (e.g., temperature or moisture) on soil properties have found it useful to identify a suite of soils for which the factor in question varies whereas the others are held constant (1 , 2 , 3 and 4). This approach has been used successfully to look at the role of temperature (3 , 5) and time (6 , 7 , 8 , 9 and 10) on the turnover of soil C. Ecosystem models such as CENTURY (11 , 12), CASA (13), or the Rothamsted model (14 , 15) predict the sensitivity of soil C inventory and turnover to climate, vegetation, and parent material, but as yet few data exist to test these predictions. Parameterizations of decomposition used in these models are based on empirical fits to specific calibration sites and may not include enough basic understanding of the interaction between plant substrates and the soil environment to make successful predictions in different environments (16).
The reservoir of soil carbon has been proposed as both a significant source and sink of atmospheric CO2. A soil source results when net decomposition exceeds C inputs to the soil, either as a result of human activities such as clearing forests for agriculture (17 , 18) or because of increased decomposition rates due to global warming (12 , 14 , 19 , 20). Net sinks of C in soils are postulated from the difference between net ecosystem C uptake and tree growth rates (21) or from presumed increases in net C inputs from CO2 or N-fertilization of plants (19, 20, 22, 23, and 24). In both cases, the magnitude and timing of the response depends on the amount of carbon in pools that respond quickly to changes in climate and vegetation, and to the time lag between fixation of C by plants and its subsequent release to the atmosphere during decomposition.
This paper will describe recent approaches used to study soil C dynamics, and preliminary applications of these tools to the problems of soil C response to global environmental changes. The results indicate the importance of the global soil C pool to the global C cycle on interrannual to century time scales and suggest profitable areas for future research.
The Nature of Soil Organic Matter (SOM)
SOM is defined here as the nonliving component of organic matter in soil. The ultimate source of organic matter in soils is CO2 fixed by plants, including leaf litter, roots, and root exudates. The activity of soil fauna (especially fungi and microbial communities) metabolizes some of these substrates and transforms others into more resistant organic compounds (collectively referred to as humus). The stabilization and fate of organic matter residues is affected by the quality of the original plant substrate (25), and the physical environment in the soil [clay content and mineralogy (26), pH, O2 availability (27), formation and disruption of soil aggregates (28, 29 and 30)]. Carbon is lost from soil mostly as CO2 produced during decomposition of organic matter, though losses of carbon through leaching or erosion may be important when considering C balance in soils on long time scales (29, 31, 32). Organic matter in soils plays important roles in determining soil water-holding capacity and soil structure, and provides a long-term store of nutrients needed by plants. Thus changes in SOM will have important feedbacks on hydrology and plant productivity (33).
SOM is difficult to study because it is a complex mixture of substances having turnover rates that range from days to millennia. The average global turnover time for soil organic carbon (to 1-m depth) was estimated as 32 years by Raich and Schlesinger (34), who divided the total C stock in soils by the average CO2 flux from soil (corrected for root respiration contribution). Turnover times varied from 14 years to 400 years for different ecosystems in their study. Radiocarbon measurements of bulk soil C, however, often show that the average age of C in soils is several hundred to several thousand years (35, 36, 37 and 38). Both results are explained if SOM contains components that turn over slower and faster than the several-decade average.
|
© 1997 by The National Academy of Sciences 0027-8424/97/948284-8$2.00/0 PNAS is available online at http://www.pnas.org. |
Much of the work in understanding and modeling soil C is the study of relative decomposition rates for various organic substrates and organic matter byproducts. While organic matter may actually be a continuum, best represented by a distribution of turnover times (39), continuous distributions are difficult to constrain with field measurements. There is general agreement that SOM contains at least three identifiable C pools: root exudates and rapidly decomposed components of fresh plant litter (“active” pool); stabilized organic matter that persists in soils over several thousands of years (“passive” pool); and a poorly defined “intermediate” or “slow” C pool that has turnover times in the range of years to centuries (Fig. 1). I will use these terms in this paper, and in addition will refer to fast-cycling C as the combined active and intermediate pools (all nonpassive C).
The mechanisms through which climate or land-use change may influence soil carbon cycling include a combination of biotic controls that affect the amount and resistance to decay of C added to soils by plants, and physical controls, including the area and chemistry of surfaces for stabilization of organic matter, and the availability of oxidants to decomposers. These mechanisms operate on vastly different time scales. Plant productivity and soil microbial communities respond to shifting climate conditions within hours (41), whereas changes in production and respiration on an annual basis are linked to interrannual variability in climate (42, 43). Persistent changes in climate can lead to species changes over time scales of decades to centuries (44), with concomitant shifts in plant production and litter quality (45). Soil minerals, forming over millennial time scales, control how much of the soil carbon is chemically/physically protected from decomposition (46, 47, 48 and 49). Finally, ecosystem disturbance caused by fire, flooding, tree blow-down, etc. will cause drastic changes in plant production and soil conditions for decomposition that far exceed those associated with interrannual variability. Thus the general design of experiments to understand soil C dynamics should depend on sampling existing environmental and disturbance gradients as well as manipulations to test soil forming factor effects on the amount and turnover times of C in active, intermediate, and passive C pools.
Tools to Study SOM
No single, satisfactory method yet exists to separate soil C into components with different turnover times. Instead, the dynamic makeup of soil C is deduced using many constraints, including: physical and chemical fractionation of organic matter, field and laboratory decomposition studies, measures of C fluxes into and out of the soil, measurements of 14C in soils sampled at various times before and after the peak of atmospheric nuclear weapons testing, changes in the 13C content of SOM after a vegetation change from plants with C3 to C4 photosynthetic pathway, and measurements of changes in the total amount of C in soils of different age or after disturbance. Each of these tools is suitable for determining different time scales of soil C dynamics, and much confusion may result from mixing of terms related to turnover times (active, intermediate, or passive) with those derived from measured soil parameters. For example, the increase in bomb 14C, or changes in 13C in SOM during the first several decades after conversion of a C3-dominated forest vegetation to C4-dominated pasture vegetation may be used to determine the amount and average turnover time of C that is fast-cycling (nonpassive). Fast-cycling C may be further comprised of active and intermediate pools with different turnover times, but isotopic techniques are not suitable for distinguishing this split.

FIG. 1. Conceptual model of SOM dynamics used in this paper (after ref. 40).
Active Carbon Pools. Field decomposition studies involve both observation of loss of native plant litter and differences in decomposition of a common litter substrate at different sites. Isotope labeling studies usually follow specific compounds or compound classes (such as amino acids or carbohydrates), but also may follow the fate of below-ground C allocation, as in pulse-labeling studies. Although some incubations have been followed over periods of more than 10 years (26, 50), most are designed for shorter time periods. These studies are used to provide multiple rate constants for decomposition models like those of Jenkinson and Raynor (15).
Soil-respired CO2 is produced either by metabolic root respiration or by decomposition of fast-cycling SOM pools (Fig. 1). In addition, CO2 fluxes measured at the soil surface are most likely dominated by decomposition in near-surface layers (including surface detritus). With these caveats, CO2 flux measurements provide an estimate of total decomposition C flux from the soil, if correction for root-respiration can be made (34). Radiocarbon measurements of respired CO2 may help distinguish sources of respiration experimentally. Root respiration and CO2 derived from decomposition of C with very rapid turnover should have ∆14C close to atmospheric values for the year of sampling, whereas CO2 derived from slow C pools will have higher or lower ∆14C values, depending on the average turnover time.
Field measurements of decomposition and CO2 flux often are complicated by seasonal differences in the quality and quantity of detrital material (for example, in temperate zones, deciduous leaves are shed in autumn, but may be responsible for a pulse of decomposition the next spring). Laboratory incubations, where soil is separated from roots, allow observation of the CO2 evolved from decomposition alone (51). Long-term incubations (>1 year) allow estimation of the relative contributions of active and slow pools to soil CO2 flux (5). However, the magnitude of laboratory fluxes of C may not be easily mapped to field conditions.
Experiments manipulating soil temperature to determine the net response of soils (19) or soils plus vegetation (52) to increased temperature are now in progress in several places. These treatments have been in place in most cases for <10 years, and preliminary results are reflective of adjustments in the fast-cycling pools.
Passive Soil Carbon and the Depth Distribution of 14C. The existence of passive SOM is deduced from radiocarbon measurements and the fact that some C3-plant-derived organic matter persists in disturbed soils even after a century or more of cultivation with C4 plants. In general, the amount and 14C content of SOM decrease with depth in soil profiles, indicating the increasing importance of passive organic matter with depth in the soil (37, 53).
The 14C age of passive organic matter may give clues to the mechanisms that cause C to persist in soil over millennial time scales. Three approaches to isolating the properties of passive C from the bulk soil have been used. The first, proposed by O’Brien and Stout (37, 54) and used by Harrison et al. (22) uses a 14C measurement from deep in the soil as an estimate of the passive pool 14C content. This approach attributes decreases in C and 14C with increasing soil depth to a decrease in the abundance of fast-cycling C pools to near-zero at depth, while assuming that the abundance and properties of passive C remain constant over the same depth interval.
A second approach toward determining the 14C content of passive SOM is based on operationally defined physical and chemical fractionation of the organic matter. Several investigators (36, 38, 53, 55, 56, 57 and 58) have shown that repeated extractions of SOM with acids and bases of increasing strength will leave a residue with a lower 14C/12C ratio than the starting material (a density separation is performed first to remove relatively intact vascular plant material, which can represent up to 80% of the C in soil A horizons).
A third way to estimate the turnover rates in the passive fraction is based on a chronosequence approach (9, 59, 60). A chronosequence is a series of soils of different age, but developed on the same parent material with the same vegetation and climate (for example, soils formed on a series of lava flows or river terraces of different age). During the first several hundred years of soil formation, C accumulation in chronosequences is rapid as vegetation is assumed to establish steady state with fast-cycling soil C components. Accumulation of passive C over millennial time scales in soils (perhaps as authigenic soil minerals are formed and provide new surfaces for organic matter stabilization) is presumably responsible for long-term C increases. Increases in the amount of passive C over time should cause a steepening of the 14C-depth curve (6, 61). Goh et al. (8) reported decreasing 14C contents in deeper soil horizons with increasing soil age in a chronosequence developed on aeolean sand.
Tools to Study the Intermediate Pool. The intermediate pool of soil C, with turnover times that vary from years to decades, is the largest fraction of organic matter in most soils. It is also the most difficult to study, as it is itself almost certainly made up of fractions with different turnover times. Ecosystem models such as CENTURY and the Rothamsted model subdivide fast-cycling soil C into readily decomposable and resistant plant debris, as well as an intermediate C pool. In temperate forests soils with low clay content, relatively undecomposed plant debris (the low-density fraction of SOM) can represent up to 80% of the C in A horizon SOM (3) and have turnover times of years to several decades. In tropical soils, low-density organic matter has turnover times of < 5 years and makes up roughly 10–30% of the total SOM in the upper 10 cm of soil. Mineral-associated (high-density) C that is hydrolyzable in acids and bases, makes up about 50% of the A horizon C, and has turnover times of 20–30 years (62). While it is tempting to map the hydrolyzable C onto the models’ intermediate C pool, operationally defined extraction methods give little practical insight into the mechanisms that are stabilizing this organic matter fraction.
The Bomb 14C Tracer. The incorporation of 14C produced in the early 1960s by atmospheric thermonuclear weapons testing (bomb 14C) into SOM during the past 30 years provides a direct measure of the amount of fast-cycling (active + slow) SOM (5, 22, 35, 37, 63, 64, 65, 66 and 67). The most straightforward approach, which compares 14C measurements of SOM sampled before 1960 with contemporary samples from the same location, is summarized in Fig. 2 (62, 64, 68). A large increase in 14C content over the past 30 years indicates that significant portions of the SOM are exchanging carbon with atmospheric CO2 on decadal and shorter time scales. Harrison et al. (22) modeled the 14C increase with time for SOM measured over the past 30 years and reported in the literature (for mostly temperate soils) and obtained an average turnover time of about 25 years for fast-cycling C. Work in other locations has shown that turnover times may be either faster or slower than this average value.
Trumbore et al. (3) used the bomb 14C technique to determine how the turnover time of fast-cycling organic matter varied along an elevation transect in the Sierra Nevada mountains of central California. Temperature decreases with elevation along the transect, though vegetation and annual precipitation also vary. C inventory in soil A horizons is predominantly in low-density fractions (<2.0 g/cm3; 50–80% of the total SOM), with another 10–30% in the hydrolyzable fraction of organic matter in the >2.0 g/cm3 fraction. Comparison of the 14C content of these fractions isolated from 0–20 cm soil
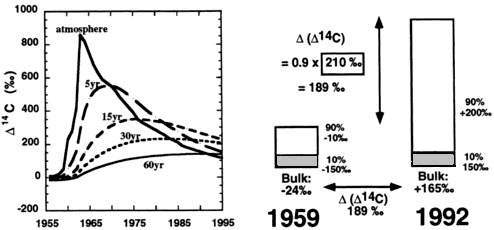
FIG. 2. (Left) Change in ∆14C in atmospheric CO2 and in two homogeneous C pools with turnover times of 5, 10, 50, and 70 years. (Right) Effect of passive organic matter on ∆(∆14C) values in bulk SOM. Failure to account for 10% of the carbon in a passive fraction that does not change in 14C content between 1959 and 1992 will result in an underestimate of the increase in fast-cycling ∆14C and therefore of turnover time in that fraction. Thus it is important to assess whether passive components such as charcoal are present in presumed fast-cycling pools (such as low-density carbon).
collected in 1959 and 1992 (Fig. 3) shows turnover is fastest in low-density material and slowest in nonhydrolyzable organic matter. Translating the 14C increases into turnover times according to procedures in Fig. 2 for the low-density + hydrolyzable organic matter gives turnover times of 8–12 years at low elevation (highest temperature) and 60 years at high elevation (low temperature). Fig. 3 also shows that the residue after repeated hydrolysis of mineral-associated organic matter in acids and bases still may contain some fast-cycling carbon (as evidenced by an increase in 14C in the past 40 years).
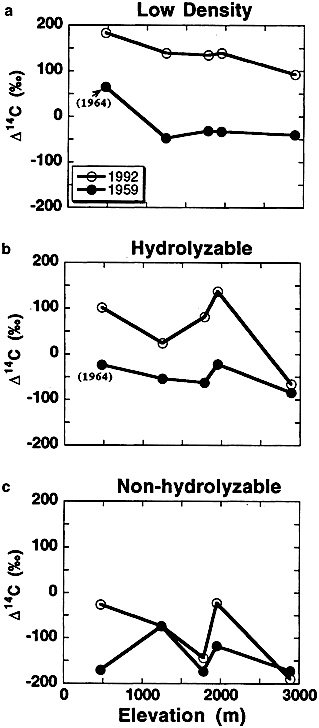
FIG. 3. Increases in ∆14C between 1959 (1964 for lowest elevation site) and 1992 for (a) low density (<2.0 g/cm3), (b) hydrolyzable (>2.0 g/cm3), and (c) nonhydrolyzable (>2.0 g/cm3) portions of SOM from an elevation transect in the Sierra Nevada (3). The fraction of organic matter with the fastest turnover time will have the highest pre-1963 14C values and show the largest increase in 14C between 1959 and 1992 (a) while the fraction with slowest turnover has lowest initial 14C values and shows little increase in 14C over the past 30 years (c).
Without prebomb samples for comparison, 14C measurements of contemporary soils may yield a very misleading picture of soil C dynamics. For example, the bulk 14C contents of the 0–20 cm layer of tropical soils sampled in the early 1990s range from −70% (Hawaii; ref. 5) to +210% (Manaus; ref. 67). The average turnover times of fast-cycling components of these soils, derived from 14C and other measures (see below), is similar (10–20 years). The amounts and 14C contents of carbon in the passive carbon pool are responsible for bulk 14C differences between these sites. The Hawaiian soils are developed on basaltic lava (Andisols) and stabilize large amounts of old carbon in noncrystalline minerals (6), whereas the soils in Manaus have very high percentages of sesquioxides that do not stabilize organic matter as well. Based on 14C measured on bulk SOM alone, one could mistakenly assume very slow turnover rates for the Hawaiian soils. While this is true for average soil C, the average is not a useful number for predicting the response of Hawaiian soils to climate or land-use change.
Townsend et al. (5) report soil C turnover times for fast-cycling C along an elevation-based temperature transect of Andisols on the slope of Mauna Kea, near Lapahoehoe, Hawaii. No prebomb archived soil samples were available from these sites. Therefore, a suite of constraints, including 14C measurements, were used to determine turnover times of active, slow, and passive organic matter. The 13C changes in SOM accompanying C3 forest to C4 pasture conversion from three sites reflect the partitioning of fast-cycling (≈75% of the total SOM) vs. passive (≈25%) carbon pools in pastures that were roughly a century old. The application of this method is much the same as looking for the increase in bomb 14C in soils; carbon turning over on decadal and shorter time scales will reflect recent isotopic changes in C sources, whereas passive carbon pools will not (26, 69, 70, 71 and 72). Townsend et al. (5) estimated the breakdown of fast-cycling C into active and intermediate C pools using long-term (1 year) incubations—the ratio of CO2 evolution after 1 year to the initially observed CO2 evolution was assumed to equal the ratio of CO2 contributed from decomposition of intermediate C pools to that from active C pools. Estimates of passive pool 14C content were made in two ways: using the method of O’Brien and Stout (37) or calculating from a knowledge of the soil age (15,000 years) and assuming constant accumulation of passive C since the time of soil formation (73). Intermediate pool turnover times then were constrained to reproduce 1990 14C values and CO2 fluxes.
Trumbore et al. (62) use a different set of constraints with 14C measurements to deduce the amount and turnover time of fast-cycling C in tropical forest soils in eastern Amazonia. Estimates of annual carbon input to the soil were derived from measurements of litterfall and fine root biomass. The 14C content of CO2 measured in the soil air space was greater than that of atmospheric CO2 for the year in which it was measured (73, 74), indicating that a large portion of the carbon contributing to soil respiration was fixed from the atmosphere by plants over the past several years to decades. The 14C content of CO2 evolved from SOM decomposition can be used as an additional constraint to break fast-cycling organic matter into active and intermediate pools.
C Inventory Changes with Land Use. Changes in soil carbon accompanying human or natural disturbance also provide clues about C dynamics in soils. Decreases in soil C inventory associated with cultivation on average may be of the order of 10–40% of initial C inventories (18), but may be smaller and difficult to measure in some areas (62). Changes in 13C abundance in SOM after a shift in vegetation with C3 to C4 photosynthetic pathway (or vice versa) can be used to identify fast-cycling organic matter. These methods rely on accurate
measures of C inventory change over time (26, 69, 70, 71 and 72), and a knowledge of the 13C content of new and old vegetation C inputs to soil. Comparison of 14C in paired undisturbed and disturbed ecosystems can be a sensitive measure of net C storage or loss over the past 30 years (62, 75). Soils that are accumulating C will have higher ∆14C values compared with an undisturbed ecosystem (presumed at steady state), whereas soils that are losing C will have lower 14C than the undisturbed site (73).
Prediction of the Response of Soil C to Global Change
Total C Inventory in Soils. Estimates of the amount of C stored in soils fall in the range of 1,200 to 1,500 Pg C (46, 76, 77). These estimates are based on relatively few soil C inventory data from many important regions, especially the tropics and northern wetlands. Global estimates are extrapolated using correlations of SOM content with vegetation/climate regime (46, 76) or by soil mapping unit (77). The apparent overall agreement between these estimates masks large uncertainties at the regional scale. For example, moist and wet tropical forest profiles reported in Schlesinger (76) have C inventories ranging from 6.5 to 20.5 kg C m−2 (average of 10.4 kg C m−2). Post et al. (46) use values of 11.5 and 21 kg C m−2 as averages for tropical moist and wet forests, respectively. Davidson and Lefebvre (78) discuss potential uncertainties involved in estimating C stores from large-scale soil map units.
Present estimates of global soil C stocks are based on the upper meter of soils, without reference to what fraction of that organic matter is in active, intermediate, or passive pools. Sombroek (79) points out that many tropical soils extend deeper than 1 m, and adding deep C inventories can increase soil C stocks in the tropics markedly. The question of what depth should be the cutoff for counting soil C stores really depends on where and how much of the C is in fast-cycling pools, and how much of the passive soil C pool may be inherited in the case of sedimentary parent material. Ecological models that include cycling of SOM generally assume that only the 0- to 20- or 0- to 30-cm layer of soils contains significant amounts of fast-cycling organic matter. Estimates of fast-cycling SOM pools derived from these models (12, 13) range from about 250 to 530 Pg C globally.
The assumption that organic matter deep in soils is not important in C cycling is not always correct. The presence of live roots to depths greater than 15 m in 40-m deep soils of seasonal tropical forests in eastern Amazonia (74) drives a fast carbon cycle to depths of at least 8 m (62, 74). Although bulk C concentrations and 14C contents of SOM at >1-m depths are low, estimates of turnover from fine-root inputs, CO2 production, and the 14C content of CO2 produced at depth show that up to 15% of the carbon inventory in the deep soil has turnover times of decades or less. In these soils, the amount of fast-cycling soil carbon between 1- and 8-m depths (2–3 kg C m−2, out of 17–18 kg C m−2) is significant compared with the amount present in the upper meter of soil (3–4 kg C m−2 out of 10–11 kg C m−2) (62).
Predicted Changes in Soil C Due to Increased Plant Production. The simplest approach to predicting the response of soil C changes in plant productivity is to assume that the size of C pools will adjust to a new steady state with increased in C inputs. Fast-cycling pools will adjust most rapidly to new steady state conditions. Harrison et al. (22), assuming a β (growth-enhancement) factor of 0.35 and turnover time of fast-cycling C of 25 years, calculated that increased C inputs to soils should have resulted in net storage of 0.5 Gt C/yr in fast-cycling C in the 1980s (with an additional 0.1 Gt C/yr added to surface litter). This approach assumes no change in decomposition rates due to changes in the quality of those plant substrates, an assumption that has yet to be tested.
The changes in C inventory predicted by Harrison et al. ( 22) are almost impossible to measure directly (62, 80). For example, even a predicted 0.5 Gt C/yr sink integrated over 30 years increases the total soil C pool only 1% (the fast-cycling pool by 3%). C inventory measurements, plagued by small-scale heterogeneity and comparatively large analytical uncertainties (for example, in bulk density measurements), cannot distinguish such small increases on local to regional scales. Thus, other methods must be discovered to determine whether C accumulation of this magnitude is actually occurring in soils.
After CO2 fertilization, the most often-cited mechanism leading to a terrestrial carbon sink is enhanced nitrogen availability to plants due to enhanced nitrate deposition (19, 20, 22, 23, 81). Nitrogen availability also will be increased if there is net decomposition of SOM, because SOM is a major source of nitrogen for plants. Although nitrogen mineralization is accompanied by CO2 efflux, the transfer of nitrogen from soil to plants would result in net carbon sequestration because the C:N ratio of plants is roughly 10 times the ratio in SOM. Decomposition rates are predicted to increase as a result of warmer temperatures (see below).
Response of Fast-Cycling Soil C to Climate Change. The amount of organic matter in soils is positively correlated with moisture and negatively correlated with precipitation (2, 46). Carbon turnover rates for combined fast-cycling C pools discussed above from the Sierra Nevada, Hawaii, and Paragominas, Brazil are plotted against mean annual temperature in Fig. 4. Although these soils differ in vegetation cover, precipitation (greater than 90 cm/yr with one exception), and soil parent material, a strong relation between turnover time of the fast-cycling C pools and mean annual (air) temperature is observed. This suggests that temperature is a major control of the turnover rate of fast-cycling soil C, in accord with recent modeling and data-based studies (5, 12, 20, 34, 50, 82, 83 and 84). The overall gradient with latitude from the tropics to boreal forests is in accord with trend derived from local elevation gradients.
Fig. 4 also plots temperature-turnover time relations predicted by the CENTURY model (12) and a literature review of surface litter decomposition rates (51). Turnover times derived from CENTURY are longer than values derived from field measurements, and show a less steep relation to temperature. The relation derived by Kirschbaum (51) plotted on Fig. 4 is a literature summary of the rates of decomposition derived from laboratory incubations of surface litter. These data suggest turnover of surface detrital pools is faster and has greater temperature dependence than fast-cycling organic matter in soils. Mean annual air temperature is plotted in Fig. 4, but obviously soils (especially surface detritus) experience seasonal and diurnal temperature extremes that may exceed those of the air above.
Both vegetation inputs and decomposition rates are expected to increase with increases in temperature (all other factors remaining equal). Soils become an amplifying feedback if the temperature dependence of decomposition is steeper than that of plant productivity (e.g. ref. 5). At present, this is still an open question. Kirschbaum (51) summarized data from incubation experiments for various types of plant litter and found that Q10 values for decomposition ranged from about 2.5 at 20°C to about 4.5 at 10°C. Data from field-based studies using climosequences (summarized in Fig. 4) suggest a Q10 for fast-cycling SOM of around 3, but are too few (particularly in tropical regions) to distinguish between cases with constant and changing Q10 values over a wide range of temperatures.
Schimel et al. (12) summarize modeling and data-based predictions of C release from SOM for a 1°C increase in global mean annual temperature. The calculated net release ranges from 11.1 to 33.8 Pg C once a new steady state is reached. The lowest value is predicted by CENTURY, which accounts for feedbacks between plant productivity and release of nutrients that accompanies net SOM decomposition. In N-limited plant
communities, the release of N through increased decomposition in a warmer world could further stimulate plant growth, and even cause net sequestering of C as nutrients are transferred from soils (low C:N ratios) to plant reservoirs with higher C:N ratios (19).
One consequence of all studies of C turnover as function of temperature is that soils already should be a net source of C to the atmosphere due to increased decomposition rates because of documented temperature increases over the past century. This present net C loss presumably would offset hypothetical increases in soil C stores from increased plant production due to CO2 fertilization.
The rate at which steady state is approached when fast-cycling C pools respond to a temperature change demonstrates the importance of recognizing differences in turnover rates of soil C between ecosystems. Trumbore et al. (3) estimate the response after changes in net primary production in tropical, temperate, and boreal forest soils. According to these calculations, interrannual variations in net primary production may cause significant interrannual variability in soil-atmosphere CO2 fluxes, with largest and fastest response in tropical regions.
Moisture changes. Soil organic carbon inventories increase regionally from arid to wet environments (2 , 46), whereas incubation and field decomposition studies show increases in decomposition rates with added moisture in aerobic soils. Decomposition rates for organic matter decrease dramatically under waterlogged conditions. Low C inventories in arid soils may be due to decreased C inputs, faster C turnover, or differences in the quality of plant material. As yet, the relative contributions of these factors are unknown.
Disturbance. Soils accumulate organic carbon over at least the first 10,000 years of development (1 , 9). Schlesinger (7) showed that the rates of C accumulation in young (thousands of years old) soils are an order of magnitude lower those needed for soils to be a significant C sink for anthropogenic CO2. The changes in C inventory over millennial time scales in soils most likely are caused by the development of secondary soils minerals with stabilization on new mineral surfaces (6).
More rapid rates of C accumulation and loss may occur over shorter time scales as the large component of fast-cycling soil C responds to disturbance such as a change in vegetation. As discussed above, soils may lose a significant portion of their carbon after cultivation; these changes represent a loss of fast-cycling C rather than passive C pools (30, 33, 62). Work in boreal forest ecosystems (85) shows high rates of net C sequestration in regrowing surface moss layers in the decades after stand-killing fire events. In these systems, decomposition in mosses is so slow as to be less important than periodic fire at controlling the status of these soils as net C sinks. Thus, particularly in regions where decomposition rates are slow, changes in fire frequency linked to climate or to land use (fire suppression) may be the ultimate control of regional status as a C source or sink. The same may be true in other fire-dominated ecosystems, such as chaparral (68), where organic matter accumulates rapidly between fire events.
Wetland areas store organic matter that is climatically stabilized; it is decomposing slowly only because of a lack of oxidants. Warming and draining of wetlands results in a loss of this stored organic matter (86, 87). In northern wetland soils (which contain an estimated one-fifth of total global soil C reservoir), this could lead to large positive feedbacks in warming.
Conclusions
Climate, vegetation, parent material, and time all affect the processes controlling accumulation and decomposition of organic matter in soils as has been known for decades. The quantification of these insights, however, is still in the beginning stages. Of the roughly 1,500 Gt of C stored in organic matter in soils, an estimated 250–530 Gt resides in C pools with turnover times of decades or less. Models extrapolating the response of the fast-cycling soil C pool on the global scale show its importance in affecting atmospheric CO2. A great deal of work remains to be done to improve our assessments of the role of soils in the global C cycle. Some areas of particular importance are:
-
Tying soil C pools with different turnover times to specific organic compound classes or structures found in soils, to understand how soil environment and plant litter quality interact to stabilize organic matter.
-
Better understanding of C accumulation and turnover in wetlands and how these will be affected by climatic and land use change. Present ecosystems models usually exclude wetland C pools from global studies.
-
Investigation of the role of landscape-scale disturbances, such as fire, in switching soils from net sinks to net sources of C.
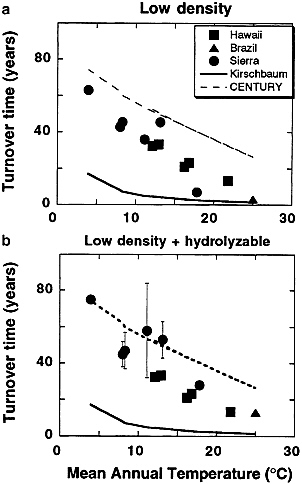
FIG. 4. Turnover times for fast-cycling fractions for a number of sites plotted against mean annual temperature: (a) low-density C in the Sierra Nevada transect (3); combined active plus slow C from the Hawaii temperature transect (5); low-density C from a mature seasonal tropical forest in eastern Amazonia (60); (b) using low-density plus hydrolyzable carbon for Sierra and Brazil sites. All data represent approximately the upper 20 cm of soil. Also shown are predicted turnover time-temperature relationships estimated from the CENTURY model and from the literature review of Kirschbaum (51). The CENTURY curve is based on model results reported in Schimel et al. (12) assuming the temperature dependence reported in that paper, and combining pools assuming 5% of the C to 0–20 cm is contained microbial biomass pool, 20% in the detrital pool, and 50% in the slow pool.
-
Manipulations (field incubations) to look at specific responses of coupled soil and vegetation pools to changes in CO2, N deposition, or climate change. Most manipulations presently are taking place in temperate ecosystems; short-term manipulations may yield larger signals in the tropics, where turnover times for fast-cycling soil C are shorter.
-
For larger scale responses over longer timescales, more work needs to be done along naturally occurring environmental gradients, especially those that may have been previously studied and may have archived soils for isotopic analysis.
Data presented here are the result of collaborative work with Eric Davidson, Dan Nepstad, Oliver Chadwick, Ron Amundson, Jennifer Harden, Alan Townsend, Margaret Torn, and Peter Vitousek. John Southon, Michaele Kashgarian, Jay Davis, and Ivan Proctor at the Center for Accelerator Mass Spectrometry at Lawrence Livermore Laboratory continue to provide valuable collaboration by measuring 14C. I thank the A. W. Mellon Foundation, the National Science Foundation, and the National Aeronautics and Space Administration’s Mission to Planet Earth Terrestrial Ecology Program for support.
1. Jenny, H. ( 1941 ) Factors of Soil Formation ( McGraw–Hill , NewYork ).
2. Jenny, H. ( 1980 ) The Soil Resource: Origin and Behavior ( Springer , New York ).
3. Trumbore, S. E. , Chadwick, O. A. & Amundson, R. ( 1996 ) Science 272 , 393–396 .
4. Vitousek, P. M. , Turner, D. R. & Kitayama, K. ( 1995 ) Ecology 11 , 189–203 .
5. Townsend, A. R. , Vitousek, P. M. & Trumbore, S. E. ( 1995 ) Ecology 11 , 721–733 .
6. Torn, M. S. , Trumbore, S. E. , Chandwick, O. A. , Vitousek, P. M. & Hendricks, D. M. ( 1995 ) EOS 76 , 73 .
7. Schlesinger, W. H. ( 1990 ) Nature (London) 348 , 232–234 .
8. Goh, K. M. , Rafter, T. A. , Stout, J. D. & Walker, T. W. ( 1976 ) N. Z. J. Soil Sci. 27 , 89–100 .
9. Harden, J. W. , Sundquist, E. T. , Stallard, R. F. & Mark, R. K. ( 1992 ) Science 258 , 1921–1924 .
10. Chadwick, O. A. , Kelly, E. F. , Merritts, D. M. & Amundson, R. G. ( 1994 ) Biogeochemistry 24 , 115–127 .
11. Parton, W. J. , Schimel, D. S. , Cole, C. V. & Ojima, D. S. ( 1987 ) Soil Sci. Soc. Am. J. 51 , 1173–1179 .
12. Schimel, D. S. , Braswell, B. H. , Holland, E. , McKeown, R. , Ojima, D. S. , Painter, T. H. , Parton, W. J. & Townsend, A. R. ( 1994 ) Global Biogeochem. Cycles 8 , 279–294 .
13. Potter, C. S. , Randerson, J. T. , Field, C. B. , Matson, P. A. , Vitousek, P. M. , Mooney, H. A. & Klooster, S. ( 1993 ) Global Biogeochem. Cycles 7 , 811–842 .
14. Jenkinson, D. S. ( 1990 ) Philos. Trans. R. Soc. London B 329 , 361–368 .
15. Jenkinson, D. S. & Raynor, J. H. ( 1977 ) Soil Sci. 123 , 298–305 .
16. Davidson, E. A. , Agren, G. , Daniel, O. , Emeis, K.-C. , Largeau, C. , Lee, C. , Mopper, K. , Oades, J. M. , Reeburgh, W. S. , Schimel, D. S. & Zepp, R. G. ( 1995 ) in What Are the Physical, Chemical, and Biological Processes That Control the Formation and Degradation of Nonliving Organic Matter? , ed. Sonntag, R. G. Z. A. C. ( Wiley , New York ), pp. 305–324 .
17. Houghton, R. A. , Hobbie, J. E. , Melillo, J. M. , Moore, B. , Peterson, B. J. , Shaver, G. R. & Woodwell, G. M. ( 1983 ) Ecol. Monogr. 53 , 235–262 .
18. Davidson, E. A. & Ackerman, I. L. ( 1993 ) Biogeochemistry 20 , 161–194 .
19. Melillo, J. M. , Kicklighter, D. W. , McGuire, A. D. , Peterjohn, W. T. & Newkirk, K. M. ( 1995 ) in Global Change and Its Effects on Soil Organic Carbon Stocks , ed. Sonntag, R. G. Z. A. C. ( Wiley , New York ), pp. 175–190 .
20. McGuire, A. D. , Melillo, J. M. , Joyce, L. A. , Kicklighter, D. W. , Grace, A. L., III, B.M. & Vorosmarty, C. J. ( 1992 ) Global Biogeochem. Cycles 6 , 101–124 .
21. Wofsy, S. C. , Goulden, M. L. , Munger, J. W. & Fan, S. M. ( 1993 ) Science 260 , 1314–1317 .
22. Harrison, K. , Broecker, W. & Bonani, G. ( 1993 ) Global Biogeochem. Cycles 7 , 69–80 .
23. Townsend, A. R. , Braswell, B. H. , Holland, E. A. & Penner, J. E. ( 1996 ) Ecol. Appl. 6 , 806–814 .
24. Thompson, M. V. , Randerson, J. T. , Malmström, C. M. & Field, C. B. ( 1996 ) Global Biogeochem. Cycles 10 , 711–726 .
25. Melillo, J. M. , Aber, J. D. & Muratore, J. F. ( 1982 ) Ecology 63 , 621–626 .
26. Balesdent, J. , Wagner, G. H. & Mariotti, A. ( 1988 ) Soil Sci. Soc. Am. J. 52 , 118–124 .
27. Bunnell, F. L. , Tait, D. E. N. , Flanagan, P. W. & Cleve, K. V. ( 1977 ) Soil Biol. Biochem. 9 , 33–40 .
28. Tiessen, H. & Stewart, J. W. B. ( 1983 ) Soil Sci. Soc. Am. J. 47 , 509–514 .
29. Oades, J. M. ( 1995 ) in An Overview of Processes Affecting the Cycling of Organic Carbon in Soils , ed. Sonntag, R. G. Z. A. C. ( Wiley , New York ), pp. 293–304 .
30. Cambardella, C. A. & Elliott, E. T. ( 1994 ) Soil Sci. Soc. Am. J. 58 , 123–130 .
31. Trumbore, S. E. , Schiff, S. L. , Aravena, R. & Elgood, R. ( 1992 ) Radiocarbon 34 , 626–635 .
32. Schiff, S. L. , Aravena, R. , Trumbore, S. E. & Dillon, P. J. ( 1990 ) Water Resourc. Res. 26 , 2949–2957 .
33. Tiessen, H. , Cuevas, E. & Chacon, P. ( 1994 ) Nature (London) 371 , 783–785 .
34. Raich, J. W. & Schlesinger, W. H. ( 1992 ) Tellus 44 , 81–99 .
35. Anderson, D. W. & Paul, E. A. ( 1984 ) Soil Sci. Soc. Am. J. 48 , 298–301 .
36. Campbell, C. A. , Paul, E. A. , Rennie, D. A. & McCallum, K. J. ( 1967 ) Soil Sci. 104 , 217–224 .
37. O’Brien, B. J. & Stout, J. D. ( 1978 ) Soil Biol. Biochem. 10 , 309–317 .
38. Scharpenseel, H. W. , Ronzani, C. & Pietig, F. ( 1968 ) in Comparative Age Determinations on Different Humic-Matter Fractions ( International Atomic Energy Commission , Vienna ), pp. 67–74 .
39. Bosatta, E. & Agren, G. I. ( 1991 ) Am. Nat. 138 , 227–245 .
40. Townsend, A. R. ( 1993 ) Ph.D. thesis ( Stanford University , Stanford, CA ).
41. Linn, D. M. & Doran, J. W. ( 1984 ) Soil Sci. Soc. Am. J. 48 , 1267–1272 .
42. Harte, J. , Torn, M. S. & Jensen, D. B. ( 1992 ) in The Nature and Consequences of Indirect Linkages Between Climate Change and Biological Diversity , eds. Peters, R. L. & Lovejoy, T. E. ( Yale Univ. Press , New Haven, CT ), pp. 325–343 .
43. Goulden, M. L. , Munger, J. W. , Fan, S. M. , Daube, B. C. & Wofsy, S. C. ( 1996 ) Science 271 , 1576–1578 .
44. Torn, M. S. & Fried, J. S. ( 1992 ) Clim. Change 21 , 257–274 .
45. Vitousek, P. M. , Turner, D. R. , Parton, W. J. & Sanford, R. L. ( 1994 ) Ecology 75 , 418–429 .
46. Post, W. M. , Emanuel, W. P. , Zinke, P. J. & Stangenberger, A. G. ( 1982 ) Nature (London) 298 , 156–159 .
47. Mayer, L. M. ( 1994 ) Geochim. Cosmochim. Acta 58 , 1271–1284 .
48. Mayer, L. M. ( 1994 ) Chem. Geol. 114 , 347–363 .
49. Chadwick, O. A. , Kelly, E. F. , Merritts, D. M. & Amundson, R. G. ( 1994 ) Biogeochemistry 24 , 115–127 .
50. Jenkinson, D. J. , Adams, D. E. & Wild, A. ( 1991 ) Nature (London) 351 , 304–306 .
51. Kirschbaum, M. U. F. ( 1995 ) Soil Biol. Biochem. 27 , 753–760 .
52. Harte, J. , Torn, M. S. , Chang, F.-R. , Feifarek, B. , Kinzig, A. P. , Shaw, M. R. & Shen, K. ( 1995 ) Ecol. Appl. 5 , 132–150 .
53. Scharpenseel, H. W. , Becker-Heidmann, P. , Neue, H. U. & Tsutsuki, K. ( 1989 ) Sci. Total Environ. 81/82 , 99–110 .
54. O’Brien, B. J. ( 1986 ) Radiocarbon 28 , 358–362 .
55. Goh, K. M. , Stout, J. D. & O’Brien, B. J. ( 1984 ) N. Z. J. Soil Sci. 35 , 69–72 .
56. Scharpenseel, H. W. , Schiffmann, H. & Becker-Heidmann, P. ( 1984 ) Radiocarbon 26 , 367–383 .
57. Scharpenseel, H. W. & Becker-Heidmann, P. ( 1992 ) Radiocarbon 34 , 541–549 .
58. Trumbore, S. E. , Bonani, G. & Wolfi, W. ( 1990 ) in The Rates of Carbon Cycling in Several Soils from AMS 14C Measurements of Fractionated Soil Organic Matter , ed. Bouwman, A. F. ( Wiley , New York ), pp. 405–414 .
59. Harden, J. W. , Trumbore, S. E. & O’Neill, K. ( 1997 ) J. Geophys. Res. , in press.
60. Schlesinger, W. H. , Reynolds, J. F. , Cunningham, F. L. , Huenneke, L. F. , Jarrell, W. M. , Virginia, R. A. & Whitford, W. G. ( 1990 ) Science 247 , 1043–1048 .
61. Amundson, R. A. , Chadwick, O. , McFadden, L. , Trumbore, S. , Wells, S. & DeNiro, M. ( 1994 ) Earth Planet. Sci. Lett. 125 , 385–405 .
62. Trumbore, S. E. , Davidson, E. A. , deCamargo, P. B. , Nepstad, D. C. & Martinelli, L. A. ( 1995 ) Global Biogeochem. Cycles 9 , 515–528 .
63. Goh, K. M. , Stout, J. D. & Rafter, T. A. ( 1977 ) Soil Sci. 123 , 385–391 .
64. Hsieh, Y.-P. ( 1993 ) Soil Sci. Soc. Am. J. 57 , 1020–1022 .
65. Harkness, D. D. , Harrison, A. F. & Bacon, P. J. ( 1986 ) Radiocarbon 28 , 328–337 .
66. Jenkinson, D. S. , Harkness, D. D. , Vance, E. D. , Adams, D. E. & Harrison, A. F. ( 1992 ) Soil Biol. Biochem. 24 , 295–308 .
67. Trumbore, S. E. ( 1993 ) Global Biogeochem. Cycles 7 , 275–290 .
68. Trumbore, S. E. , Vogel, J. S. & Southon, J. R. ( 1989 ) Radiocarbon 31 , 644–654 .
69. Balesdent, J. , Mariotti, A. & Guillet, B. ( 1987 ) Soil Biol. Biochem. 19 , 25–30 .
70. Cerri, C. C. , Eduardo, B. P. & Piccolo, M. C. ( 1991 ) in Use of Stable Isotopes in Soil Organic Matter Studies ( International Atomic Energy Agency , Vienna ), pp. 247–259 .
71. Desjardins, T. , Andreux, F. , Volkoff, B. & Cerri, C. C. ( 1994 ) Geoderma 61 , 103–118 .
72. Balesdent, J. & Mariotti, A. ( 1996 ) in Mass Spectrometry of Soils , eds. Boutton, T. & Yamasaki, S. ( Dekker , New York ), pp. 83–112 .
73. Trumbore, S. E. & Druffel, E. R. M. ( 1995 ) in Carbon Isotopes for Characterizing Sources and Turnover of Nonliving Organic Matter , ed. Sonntag, R. G. Z. A. C. ( Wiley , New York ), pp. 7–21 .
74. Nepstad, D. C. , de Carvalho, C. R. , Davidson, E. A. , Jipp, P. H. , Lefebvre, P. A. , Negreiros, G. H. , Silva, E. D. D. , Stone, T. A. , Trumbore, S. E. & Vieira, S. ( 1994 ) Nature (London) 372 , 666–669 .
75. Harrison, K. G. & Broecker, W. S. ( 1993 ) Science 262 , 725–729 .
76. Schlesinger, W. H. ( 1977 ) Annu. Rev. Ecol. Sys. 8 , 51–81 .
77. Eswaran, H. , Berg, E. V. D. & Reich, P. ( 1993 ) Soil Sci. Soc. Am. J. 57 , 192–194 .
78. Davidson, E. A. & Lefebvre, P. A. ( 1993 ) Biogeochemistry 22 , 107–131 .
79. Sombroek, W. G. , Nachtergaele, F. O. & Hebel, A. ( 1993 ) Ambio 22 , 417–426 .
80. Post, W. M. , Anderson, D. W. , Dahmke, A. , Houghton, R. A. , Huc, A.-Y. , Lassiter, R. , Najjar, R. G. , Neue, H.-U. , Pedersen, T. F. , Trumbore, S. E. & Vaikmae, R. ( 1995 ) in What Is the Role of Nonliving Organic Matter Cycling on the Global Scale , eds. Zepp, R. G. & Sonntag, C. ( Wiley , New York ), pp. 155–174 .
81. Schimel, D. S. , Braswell, B. H. , McKeown, R. , Ojima, D. S. , Parton, W. J. & Pullman, W. ( 1996 ) Global Biogeochem. Cycles 10 , 677–692 .
82. Buol, S. W. , Sanchez, P. A. , Kimble, J. M. & Weed, S. B. ( 1990 ) in Predicted Impact of Climate Warming on Soil Properties and Use , ed. Kimball, J. M. ( American Soil Association , Madison ), Special Publication No. 53 , pp. 71–82 .
83. Raich, J. W. & Potter, C. S. ( 1995 ) Global Biogeochem. Cycles 9 , 23–36 .
84. Townsend, A. R. , Vitousek, P. M. & Holland, E. A. ( 1992 ) Clim. Change 22 , 293–303 .
85. Trumbore, S. E. & Harden, J. W. ( 1997 ) J. Geophys. Res. Atmosph. , in press.
86. Goreham, E. ( 1991 ) Ecol. Appl. 1 , 182–195 .
87. Billings, W. D. ( 1987 ) Q. Sci. Rev. 6 , 165–177 .