Proc. Natl. Acad. Sci. USA
Vol. 94, pp. 8362–8369, August 1997
Colloquium Paper
This paper was presented at a colloquium entitled “Carbon Dioxide and Climate Change,” organized by Charles D. Keeling, held November 13–15, 1995, at the National Academy of Sciences, Irvine, CA.
A long marine history of carbon cycle modulation by orbital-climatic changes
T IMOTHY D. H ERBERT
Department of Geological Sciences, Brown University, Providence, RI 02912
ABSTRACT Pacing of the marine carbon cycle by orbital forcing during the Pliocene and Pleistocene Ice Ages [past 2.5 million years (Myr)] is well known. As older deep-sea sediment records are being studied at greater temporal resolution, it is becoming clear that similar fluctuations in the marine carbon system have occurred throughout the late Mesozoic and Tertiary, despite the absence of large continental ice sheets over much of this time. Variations in both the organic and the calcium carbonate components of the marine carbon system seem to have varied cyclically in response to climate forcing, and carbon and carbonate time series appear to accurately characterize the frequency spectrum of ancient climatic change. For the past 35 Myr, much of the variance in carbonate content carries the “polar” signal of obliquity [41,000 years (41 kyr)] forcing. Over the past 125 Myr, there is evidence from marine sediments of the continued role of precessional (˜21 kyr) climatic cycles. Repeat patterns of sedimentation at about 100, 400, and 2,400 kyr, the modulation periods of precession, persistently enter into marine carbon cycle records as well. These patterns suggest a non-linear response of climate and/or the sedimentation of organic carbon and carbonates to precessional orbital perturbations. Nonlinear responses of the carbon system may help to amplify relatively weak orbital insolation anomalies into more significant climatic perturbations through positive feedback effects. Nonlinearities in the carbon cycle may have transformed orbital-climatic cycles into long-wavelength features on time scales comparable to the residence times of carbon and nutrient elements in the ocean.
We tend to think that we live in the most exciting time. To a geologist, the most exciting time is the late Pleistocene world (the past 800,000 years), a period of unusually sensitive climate. Certainly, there are many surprises that have come from gathering paleoclimatic records over the past few million years (Myr) or so. These surprises include the demonstration that ice ages come and go with a regularity that can only come from pacing by changes in the Earth’s orbit ( 1 , 2 ); that these ice ages involve changes in sea level on the order of 125 m ( 3 ); that the deep ocean circulation rearranges itself with climatic state ( 4 , 5 and 6 ); and that the climatic changes, at least for the last two major glacial cycles, go hand in hand with variations in methane and carbon dioxide contents of the atmosphere ( 7 , 8 ).
The observed response of late Pleistocene climates to orbital perturbations raises a number of puzzles, many of which will reappear as we examine older climatic records. From a variety of proxy records, we have learned that seasonal anomalies enter strongly into the mean state of the climate system over the past few million years. This is seen clearly in the resemblance of the famous “Milankovitch” radiation curve of July temperatures at 65° north to the oxygen isotope record of ice volume and sea level. Were the insolation anomalies to be calculated on an annual basis, the ˜21,000-year (21-kyr) precessional component would vanish. In addition, a strong, ˜100-kyr cycle not present in the insolation curve runs through the oxygen isotopic record of sea level changes and other late Pleistocene climate records. The 100-kyr cycle dominates the ˜160-kyr-long greenhouse gas records of polar ice cores ( 7 , 8 ), although the Vostok methane record ( 8 ) seems to show particular sensitivity to the 21-kyr precessional forcing as well. It is difficult to explain the 100-kyr cycle of glaciation from the meager 100-kyr insolation anomaly directly due to eccentricity variations, although it may arise as a rectified response to the precessional cycle within a narrow band of the equator ( 9 ). Rather, most climate modelers invoke a large nonlinearity or linearities of the climate system to transfer energy from the precessional cycle, which is amplitude-modulated at the eccentricity periods of about 100, 400, and 2,400 kyr. Amplitude-modulated signals vary about a mean value according to a lower frequency function, which in the case of precession is the orbital eccentricity. The low frequency does not appear directly in the output of a linear response to such a forcing. Nonlinear systems tend to transform amplitude-modulated forcing into an outcome with significant energy at the modulating periods and, hence, could produce long-wavelength climatic cycles from the much shorter precessional perturbations ( 10 , 11 ).
In this contribution, I shall follow the variability of past climates, as it manifests itself in carbon records in deep-sea sediments over the past 125 Myr. This time span has seen major changes in the state of the Earth’s climate: changes from the warm, equable climates of the middle Cretaceous Period to the progressive cooling and glaciation of high latitudes during the Tertiary Period ( 12 , 13 , 14 and 15 ). The dynamics of the carbon records will be viewed with a particular lens: that of the spectral window tuned to the Milankovitch frequency band. By comparing variations on the same time scale, and with similar forcing, with those that alter late Pleistocene climate, I wish to make three points. First, the large-scale modulations of the marine carbon system, well characterized for the last 2.5 Myr, persist at similar frequencies into the more remote marine record. The prime recorder that will be used to measure these modulations is the calcium carbonate content of marine sediments. Second, in some cases, we may be able to assess whether the ancient marine climate–carbon connection was synchronized between hemispheres in ancient climates, as it has been in the more recent glacial cycles. And third, I will argue that marine carbon records suggest that pervasive nonlinearities in the climate system can translate relatively high frequency (˜20-kyr), seasonal, insolational variance into low frequency patterns that might be mistaken for tectonically driven changes in climate and ocean geochemistry.
|
Abbreviations: kyr, thousand years; Myr, million years; Ma, million years ago; K/T, Cretaceous–Tertiary. |
|
© 1997 by The National Academy of Sciences 0027-8424/97/948362-8$2.00/0 PNAS is available online at http://www.pnas.org. |
The Pleistocene Precedent
Geologically measurable aspects of the marine carbon cycle trace important perturbations in the Earth’s climate; these measurements also suggest that the marine carbon cycle may have feedbacks that amplify climatic variance. One of the first convincing links between marine sediments and global climate change of the Pleistocene came from Arrhenius’ ( 16 ) study of the calcium carbonate content of sediment cores from the equatorial Pacific Ocean. Arrhenius correctly connected the variations in deep-sea sediment composition with ice age cycles known from the land and also noted the cyclical nature of the changes over time. It is now clear that over the past 2.5 My, nearly all aspects of the Earth’s climate have been paced by variations in the Earth’s orbital elements ( 17 , 18 and 19 ). In the deep sea, these changes include rearrangement of the deep circulation such that present differences in carbon chemistry between the abyssal Atlantic and Pacific nearly vanished during glacial stages ( 6 , 20 ). This restructuring is evidenced by changing lateral gradients in carbon isotopes recorded by benthic foraminifera ( 4 , 6 ), and by compensating changes in calcium carbonate content in Pacific and Atlantic sediments ( 21 ). During ice ages, carbonate microfossils tend to be better preserved in the Pacific and to show poorer preservation in the Atlantic. The carbonate variations trace important modulations in deep-water sources over time and, therefore, in the heat and salinity budgets of the global ocean circulation.
Carbon isotopic records measured from the skeletal remains of foraminifera indicate major changes in the carbon budget of the glacial world as compared with the present. A global shift to lighter isotopic compositions is best explained as a transfer of terrestrial organic matter (with mean isotopic composition of -25‰ to the ocean during ice ages after the destruction of forests and soils in the colder and drier glacial world ( 22 ). The mean oceanic shift in d13C of -0.4‰ ( 22 , 23 ) corresponds to the addition of some 450 × 1015 g of carbon to the ocean during glacial times. By itself, such a transfer would have tended to promote a higher CO2 content of the atmosphere. Ice core records, however, indicate the reverse; the paradox must be resolved by changes in the carbon cycle in the ocean that favor increasing carbon storage in the ocean during glacial periods. To date, hypotheses involving changes in the production of marine organic carbon in the glacial world ( 24 , 25 and 26 ) and in the alkalinity structure of the glacial ocean ( 27 ) have been proposed but have not produced a satisfying resolution.
As we look to time scales of millions of years, it becomes clear that the spectral content of Pleistocene carbon records mirrors that of other paleoclimatic indices. For example, the transition in ice volume frequency at ˜1 Myr ago from an earlier regime of 41 kyr (obliquity) to the late Pleistocene rhythm of large-amplitude, 100-kyr cycles, shows clearly in carbonate time series as well as in changes in oxygen isotope ratios ( 28 , 29 ). In both regimes, carbonate variations record in large measure the dance of deep-water sources over time. The faithfulness of marine carbonate records in replicating the mid-Pleistocene switch observed in oxygen isotopic data from 41- to 100-kyr-dominated spectra is significant for it indicates that nonlinearities in carbonate preservation and production are not so inherently large as to inevitably produce 100-kyr and longer cycles from precessional climate forcing alone (we will return to this point later). If changes in ocean alkalinity and nutrient structure are to be inferred for late Pleistocene, 100-kyr ice age cycles ( 27 ), then the early Pleistocene regime saw largely 41-kyr repeat cycles of high latitude origin in the oceanic carbon pump.
Organic carbon contents of marine sediments also vary at Milankovitch frequencies in the late Pleistocene ( 30 , 31 , 32 and 33 ). If these can be read as paleo-productivity records, then there is evidence for substantial modifications in the export of carbon from surface waters in soft form. It appears, however, that the patterns of organic carbon accumulation obtained from sediments are highly regional, and it is difficult at present to reconstruct a global mass balance for the export of organic matter during glacial periods.
Signatures of Orbital Cycles
A general understanding of the “fingerprint” of orbitally driven cycles is necessary if we are to follow the sensitivity of the climate system in general, and the carbon cycle in particular, to orbital perturbations in the more distant past. Direct insolation forcing comes from the obliquity cycle, with a current mean period of 41 kyr, and from precessional variations, with periods of 23 and 19 kyr. Considerations of tidal friction indicate that the periods of these orbital cycles should be shorter in the past; Berger and colleagues ( 34 ) estimate that the obliquity repeat time would be 38.75 kyr by 100 Ma (mid-Cretaceous) and that the precessional periods would lie at about 22 and 18 kyr, respectively. Eccentricity forcing is likely to remain at similar frequencies to the present day, that is, at a short cycle of ˜109 kyr actually comprised of components near 95 and 125 kyr, and nearly “line” frequencies of 413 and 2,400 kyr ( 35 , 36 ). These frequencies should primarily enter the climate system as modulations (the variance around the mean) of precession, as the direct radiative forcing due to eccentricity variations is minuscule. However, the importance of 100-kyr energy in the late Pleistocene record of ice volume tells us that Milankovitch climate theory may be missing a major component of climate variance.
Geologists have a number of tools to use as they examine older marine records for continuing evidence of orbitalclimatic forcing. By using multiple constraints, a convincing case can often be made that orbital frequencies are correctly identified. Criteria include repeat periods estimated by paleomagnetic and paleontological data consistent with orbital cycles, continuity of cyclic sedimentation, statistical evidence for periodicity from spectral analyses, successful correlation of patterns between different study locations, and internal consistency of the data viewed with the orbital model of sedimentation (for example, evidence of the expected amplitude modulating frequencies of precession when a precessional signal is identified). In most cases, the repeat times of carbon cycles can be estimated to a relative error of 10–20% over the past 84 Myr, the period of frequent, well-dated magnetic reversals. Good matches over long time periods between the repeat times of sedimentary cycles and expected orbital periods are powerful indications that a cause and effect connection has been found. Difficulties in dating older marine sediments accurately mean that other consistency arguments become more important in fingerprinting an orbital-climatic connection. One can also compare the character of sedimentary cycles to the expected forcing. For example, obliquity cycles are nearly sinusoidal in nature, whereas the precessional rhythm is strongly modulated by eccentricity cycles at about 100, 400, and 2,400 kyr. Such diagnostic features can often be observed in sedimentary time series.
As we perform time series analyses of pre-Pleistocene deep-sea sediments, some of the questions we ask are as follows. (i) Is there evidence of continuing paleoceanographic sensitivity to orbital forcing since the Mesozoic, as seen through carbon records? (ii) If so, how does the climatic response to the possible mix of orbital components evolve over time? (iii) Is there evidence that Northern and Southern hemispheres continue to be synchronized to orbital-climatic cycles in the distant past? (iv) Do long-wavelength, eccentricity-driven cycles appear in pre-Pleistocene carbon records? (v) Can we deduce some features common to orbitally driven changes in the carbon cycle over the long late Mesozoic and Tertiary marine record?
Spectral Record of Tertiary Age Carbonate Sediments
Bloemendal and deMenocal ( 37 ) have documented a spectral shift in carbonate records at ˜2.6 million years ago (Ma) that seems coincident with the onset of significant Northern Hemisphere glaciations. They show that carbonate proxy records from the Oman margin record a growth in 41-kyr spectral power at the expense of 23-kyr precessional power across this transition. Further work has demonstrated that a similar transition occurs in the equatorial Atlantic. The change is believed to reflect the penetration of high latitude climate variations, which are most sensitive to obliquity forcing, into low latitudes. Although deMenocal ( 38 ) ascribes most of the variation in sediment carbonate content to dilution by atmospheric dust, the fact that a similar spectral transition occurs in nearly dust-free equatorial Pacific sediments ( 39 ) argues again for a coordinated shift in the frequency with which deep-water sources and carbonate alkalinity oscillated as climate evolved to cooler and more glaciated conditions.
As well sampled records of pre-Pliocene deep-sea sediments accumulate, it is becoming clear that the marine carbon cycle sensed orbital forcing in warmer climates of the middle and earlier Tertiary. Ocean drilling has provided glimpses of this sensitivity at a number of locations; these patterns have been supplemented by studies of pelagic sediments now exposed on land ( 40 , 41 , 42 and 43 ). One of the longest continuous records of orbitally mediated carbonate cycles comes from Ocean Drilling Program Leg 154 ( 44 ). Variations in carbonate content at Milankovitch frequencies were traced to middle Paleocene (˜55 Ma) times, with no suggestion of a halt in the Milankovitch response ( 44 ). Records from Oligocene age onward show evidence of obliquity (41 kyr) forcing, confirming a suggestion by Mead and colleagues ( 45 ) that this cycle has been an important paleoclimatic frequency since the inception of glaciation in the Southern Hemisphere at the end of the Eocene Epoch, ˜33.5 Ma.
Low Frequency Components in the Tertiary Carbon Cycle
The importance of 100-kyr cyclicity in the late Pleistocene is a puzzle in the Milankovitch theory of climate. Its disappearance in the earlier Pleistocene and latest Pliocene suggests a simpler world in which paleoclimatic patterns are more easily explained by a linear response to orbital forcing. However, there are a number of observations to suggest that eccentricity frequencies haunt earlier times as well. In some cases, prePleistocene time series show variance centered on the 100-kyr cycle in a manner similar to the last 1 Ma, but in others, there is good evidence for the 400-kyr component, the single largest term in the astronomical eccentricity series. Pliocene (3–4 Ma) carbonate records from the equatorial Pacific show a frequency structure almost identical to that of the late Pleistocene ( Fig. 1 ). An ˜100-kyr cycle of dissolution has about twice the amplitude attributable to obliquity and precessional periods. Deep-ocean carbon chemistry was clearly modulated by orbital forcing in a climate without large Northern Hemisphere ice sheets. Carbonate content of equatorial Pacific sediments fluctuated at a dominant period of 400 kyr in late Miocene times ( 46 ), another indication of a long history of eccentricity pacing of deep-ocean carbonate dissolution. This ˜400-kyr period is also seen clearly in carbon isotopic records of benthic foraminifera of mid-Miocene age ( 47 ) that imply considerable changes in the marine carbon budget. The authors attribute these changes to periods of enhanced burial of marine organic matter during climatic coolings. What is intriguing is the large amplitude of the long-wavelength variations (˜1‰ cycles in d13C), in view of the minimal direct radiative forcing at eccentricity frequencies.
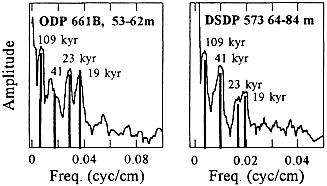
FIG. 1. Spectra of early Pliocene (3.5–4.5 Ma) carbonate variations at Deep Sea Drilling Site 573, equatorial Pacific, and at Ocean Drilling Program Site 661, tropical Atlantic. Frequency is plotted as a function of stratigraphic position; no “tuning” has been performed to achieve a match to an orbital model. Spectral peaks interpreted to correspond to the short cycle of eccentricity (109 kyr), the obliquity cycle (41 kyr), and the two components of precession (˜23 and 19 kyr) are indicated by vertical lines.
Interhemispheric Synchrony of Precessional Carbonate Cycles, Late Cretaceous
Deep-sea coring has given us a number of overlapping records in the South Atlantic and Indian oceans ( 48 , 49 ) of late Cretaceous and early Tertiary age that provide paleoceanographers with an unusual opportunity to develop a global picture of the dynamics of carbon cycling in the older ocean. In addition, intense interest in the Cretaceous–Tertiary (K/T) extinction level has generated detailed studies of land sections where former deep-sea sediments outcrop. Good independent dating of sections from magnetic reversal stratigraphy and from micropaleontological studies add confidence to the correlation of high frequency sedimentary variations between sites. These records allow us not only to document the pervasive orbital influence on marine carbon records, but also, using the unusually well documented time line at the K/T boundary, to assess whether carbon cycle variations may have been coordinated between hemispheres, as they have been in the Pleistocene.
Cores recovered by the Deep Sea Drilling project from the South Atlantic display continuous carbonate cyclicity from Santonian (˜85 Ma) to mid-Paleocene (˜60 Ma) time. Drill sites come from two subsiding rises on opposite sides of the South Atlantic that lay at about 30° south at the time of deposition. Variations in carbonate content can be logged by reflectance spectroscopy calibrated to discrete measurements of calcium carbonate content ( Fig. 2 ). Carbonate cycles are present at sites that ranged in paleodepth from 0.5 to 3.5 km. Carbonate variations are substantial (lows of 35%, highs of 85%) and highly structured. The mean repeat time of the cycles estimated from paleomagnetic stratigraphy is 23.3 ± 4 kyr, a good match to the expected precessional period ( 48 ). Just as importantly, the cycles display the typical hierarchy of modulation expected of precessional anomalies. Nodes of low amplitude occur at 4–5 cycles, 20–25 cycles ( Fig. 2A ), and 100–120 cycles ( Fig. 2B ). These almost certainly match eccentricity modulation at about 100-, 400-, and 2,300-kyr repeat times. The cycles appear to be directly correlative between sites, indicating a basin-wide climatic control on sedimentation.
Along with the amplitude modulation expected of a direct response to precessional seasonal anomalies in insolation, the South Atlantic carbonate cycles contain evidence for cycles in accumulation that cannot be so readily explained. Changes in sediment accumulation rate can be estimated by following the spacing of the carbonate cycles over time. Intervals of thicker
cycles, an indication of higher sediment fluxes, coincide with intervals of high amplitude oscillations in carbonate content. The most evident modulations in sedimentation rate occur at intervals of about 400 and 2,500 kyr. A linear response of carbonate sedimentation to precessional forcing would not generate long-wavelength anomalies in deposition because the increase in deposition rate during one phase of a precessional cycle would be precisely canceled by the succeeding decrease, i.e., the precessional signal itself varies at any given latitude about a constant mean. Therefore, the low frequency energy envelope of precessional variations must have been transferred in this portion of the geological record into low frequency changes in oceanic carbonate deposition through an unidentified nonlinear mechanism.
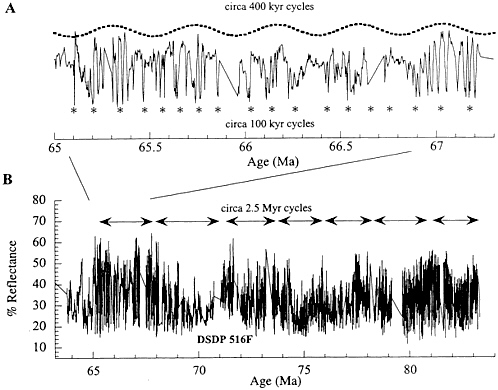
FIG. 2. Time series of late Cretaceous (Campanian through Maastrichtian) deep-sea carbonate variations from South Atlantic Deep Sea Drilling Project Site 516F (DSDP 516F). Reflectance measurements at 700 nm logged every 4 cm by a visible light spectophotometer have been used as a proxy for carbonate content. Time control comes from magnetic reversal stratigraphy correlated to the Berggren et al. ( 50 ) time scale. Carbonate variations are modulated at a number of scales, the longest of which is close to 2.5 Myr, the long nodal cycle of eccentricity (B). The detail above shows the organization of individual precessional carbonate cycles into bundles of 100- and 400-kyr spacing (A).
The late Cretaceous carbonate variations show a South Atlantic Ocean dominated almost entirely by low latitude (precessional) climate variations. Because the cycles appear in both shallow (paleodepth = 1 km) and deeper locations, it seems likely that they reflect in large part changes in the productivity of calcareous organisms rather than in the alkalinity of deep waters. Simple models of sediment accumulation suggest that the carbonate cycles reflect increases both in carbonate accumulation (high carbonate beds) and in dilution by terrigenous material during the antiphase (dark, low carbonate concentration layers) of the 20-kyr cycle.
Late Cretaceous deep-sea sediments now exposed in Spain give us the unusual chance to test whether precessional variations in the marine carbon cycle may have been synchronized between hemispheres. As in the South Atlantic, high frequency oscillations in carbonate have been detected in a number of outcrops of Maastrichtian age sampled by ten Kate and Sprenger ( 51 ). Although magnetic reversal stratigraphy has not been successful in each study site, the lithological patterns are similar enough between sites that time lines can be drawn quite confidently between the Spanish sections. I believe that it is possible to correlate the distinctively modulated patterns of carbonate variations in the 500 kyr before the K/T boundary between the North and South Atlantic with high confidence ( Fig. 3 ). Tie lines between the sites include the K/T boundary, the position of the magnetic reversal boundary separating polarity zone C29R from the normal polarity zone C30N, and the node of low amplitude carbonate variations that I attribute to the 400-kyr eccentricity amplitude envelope. Surprisingly, the carbonate oscillations seem to be in phase between hemispheres. In every South Atlantic section, the K/T boundary occurs at the top of the high carbonate phase of the precessional cycle. Precisely the same pattern occurs in Spain. The studied sections were situated in subtropical locations (about 30° south and north) on opposite sides of the late Cretaceous equator. It appears likely, then, that carbonate variations in both hemispheres, at least in the Atlantic basins, were somehow synchronized in a manner similar to the late Pleistocene. (Recall that insolation anomalies due to precession are out of phase between hemispheres; synchrony of ocean chemical changes on opposite sides of the equator implies the dominance of one hemisphere.) The most plausible means of linking the carbonate records between the North and South Atlantic is through a deep-ocean circulation climatically weighted to
one hemisphere. Changes in carbonate content could have been effected by some combination of varying nutrient inventories and varying corrosiveness of deep waters to carbonate minerals.
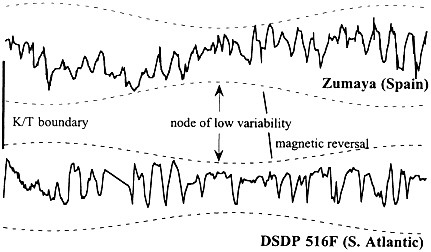
FIG. 3. Comparison of latest Cretaceous carbonate cycles from the Southern Hemisphere [Deep Sea Drilling Project Site 516F (DSDP 516F)] and from the Northern Hemisphere (Zumaya, Spain). Similar modulations are seen in both records; note the node of low variability that is correlated to the ˜400-kyr eccentricity modulation of precessional amplitude. The K/T extinction boundary occurs in both hemispheres at the peak of a high carbonate cycle. Spanish data are from ten Kate and Sprenger ( 51 ).
The 100- and 400-kyr Cycles in Carbonate and Organic Carbon Deposition, Middle Cretaceous
The middle Cretaceous (Aptian through Cenomanian stages) is widely believed to have had the warmest global climate of the last 125 Myr ( 12 , 52 ). One common feature of deep-sea deposits of this age are organic-carbon enriched “black shales,” which have been found throughout the Mediterranean–Atlantic basins, and into the high latitude Indian Ocean ( 39 , 53 ). Modern deep-sea sediments generally have organic carbon contents of 0.5% or less; therefore, the frequent occurrence of layers with 1–25% organic carbon in the middle Cretaceous pelagic record may indicate unusual oceanic circulation during the thermal maximum. The data presented below indicate that the biological productivity of warm Cretaceous oceans was highly variable; warmer temperatures apparently did not make for a less dynamic environment. Detailed studies from one location, a continuous core drilled through Aptian and Albian deep-sea sediments of central Italy ( 54 ) can be applied to other mid-Cretaceous ocean basins because studies of pelagic sediments outcropping in the Mediterranean region and recovered by deep-sea drilling show similar patterns ( 40 ).
Time series sampling demonstrates that organic carbon-enriched beds fall in a consistent position in a hierarchy of sedimentary oscillations linked to orbital forcing. Black shales occupy ˜5-cm-thick intervals of low carbonate in the curves presented in Fig. 4. Typical deposition rates for the intervals studied are ˜0.5 cm/kyr, so the dark–light, low–high carbonate pair has a period of ˜20 kyr. However, there are longer term oscillations of ˜50 cm (100-kyr) long, in which intervals of two to three oscillations of high carbonate content alternate with two to three pairs of lower carbonate cycles. It is in the troughs of this 50-cm cycle that black shales occur. A further hierarchy of cyclicity occurs at 2–2.5 m (400–500 kyr). This wavelength makes an “envelope” on the amplitude of the 50-cm, 100-kyr cycle. At nodal points, carbonate variations are small, and black shales are usually absent. Spectral analysis of the middle Cretaceous lithological records clearly demonstrates the presence of an important periodicity at ˜100 kyr ( 55 ) with much greater amplitude than at the precessional periods. Simple “tuning” assumptions used to sharpen spectra in fact detect two line components whose frequency ratio precisely matches the beat frequencies of the 100-kyr cycle (95 and 125 kyr) in the Cretaceous carbonate records ( 55 ).
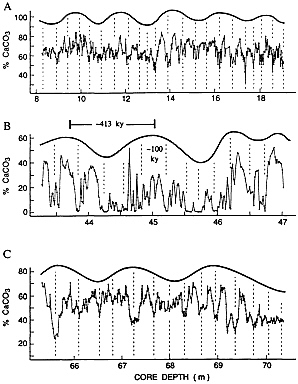
FIG. 4. Middle Cretaceous series of carbonate variations driven by a combination of precession and eccentricity cycles. The series in A comes from strata at ˜102 Ma; the series in B, from strata at ˜110 Ma; and the series in C, from strata at ˜115 Ma. The average sedimentation rate is just under 0.5 cm/kyr. Organic carbon-rich beds occur in 5-cm-thick bands of low carbonate content.
Geochemical work demonstrates that the basic alternation of lithologies is a simple mixing of biogenic skeletal components (both siliceous and calcareous) and terrigenous material ( 54 ). The organic carbon-enriched layers are actually impoverished in the remains of hard parts of the marine plankton. The covariance of both siliceous and carbonate deposition makes it unlikely, therefore, that the carbonate oscillations reflect changes in the saturation state of the deep basin waters. Instead, the evidence favors several-fold variations in the productivity of the planktonic ecosystem at periods of 20, 100, and 400 kyr. Large variations in productivity could in turn generate large changes in deposition rate, which could skew the interpretation of frequency spectra collected as a function of depth. This time–depth distortion could explain the observation that the individual ˜20-kyr carbonate oscillations are poorly resolved in frequency analysis ( 55 ).
If we use the bedding cycles as time markers, it is possible to develop a reasonable approximation to the dynamics of accumulation of both biogenic and terrigenous materials in the middle Cretaceous ocean. This reconstruction does indeed show the fingerprint of a precessional cycle highly modulated by 100- and 400-kyr cycles of eccentricity. The orbital chronometer works as follows. If we assume that each ˜10-cm cycle in carbonate content has approximately the same time value (in fact, the precessional period varies by ˜12% around its mean), and we pick cycle maxima and minima objectively, then it is possible to total the accumulation of carbonate, biogenic silica, organic matter, and terrigenous matter per unit time (one 20-kyr cycle). It is then possible to assess the relative variances of the different components and to detect modulations in the accumulation rate that might be otherwise masked. Fig. 5 shows bed-by-bed (10-kyr increment) totals of carbonate and aluminum (a proxy for clay content) for an upper Albian (˜102 Ma) time series. The variance of the carbonate accumulation is about twice that of the terrigenous component, reinforcing the earlier hypothesis of a strong control on deposition rate by fluctuations in carbonate production (a conclusion further strengthened by micropaleontological data from ref. 56). Carbonate accumulation patterns also show significant periodicities centered at about 100 and 400 kyr (dashed curves indicated in Fig. 5 ). These variations could only arise if eccentricity modulations of precession enter in some way directly into deposition rate. A weak oscillation in aluminum flux is present at the 100-kyr period, essentially out of phase with carbonate (and silica) deposition. But a quite regular, large amplitude cycle occurs at the 400-kyr period. The flux of clays and other terrigenous materials, therefore, covaried with carbonate with about equal amplitude and phase at the 400-kyr cycle. This covariance mostly masks the 400-kyr signal when only weight percent measures of the sediment are inspected.

FIG. 5. Comparison of accumulation rates of carbonate and aluminum over an estimated 1.6 Myr of middle Cretaceous history from central Italy. A 20-kyr repeat time for carbonate cycles has been assumed, consistent with independent estimates of sedimentation rate, and with spectral analysis of geochemical time series. Geochemical fluxes were estimated by binning components in sequential 10-kyr half-cycles defined by maxima and minima in carbonate content. Sinusoids of about 100 and 400 kyr (dashed lines) have been drawn to highlight the dominant patterns of carbonate accumulation. Note that the variability of carbonate fluxes is larger than that for aluminum at the 100-kyr period but that the two components covary at the 400-kyr period. The ˜3-fold changes in carbonate accumulation on 100-kyr time scales are believed to result from changes in the productivity of calcareous plankton.
We are left then, with a picture of large variations in the flux of both biogenic and terrigenous materials to many Cretaceous ocean basins. The shortest recurrences were paced by precessional insolation anomalies. It appears that at the 20- and 100-kyr wavelengths, the high marine productivity episodes alternated with low productivity intervals in which the clay accumulation was enhanced. As the dominant mode of clay flux to the sheltered basins of the Italian region was presumably by eolian deposition, the evidence favors a synchronization between wetter continental conditions, with low clay influx and higher marine producitivity, and drier conditions, with enhanced transport of clays to the deep sea and concomitantly lower productivity in the study area. The changes in productivity could be caused by large changes in the supply of nutrients to the ocean basins with changes in climate, or, more probably, by changing patterns of exchange between ocean basins. Switches from “estuarine” to “lagoonal” configurations ( 57 ) could have altered the carbon dynamics on the Milankovitch time scale. The Cretaceous organic, carbon-rich intervals correspond to extremes in precessional forcing, in a manner strikingly similar to analogous deposits from the Eastern Mediterranean over the past 0.5 Myr ( 58 ).
Models that use Cretaceous geography and orbital end member geometries do in fact suggest monsoonal sensitivity of the Cretaceous climate that could explain the sedimentary variations ( 52 , 59 ). The largest depositional anomalies in the Italian sequence, however, coincide with eccentricity periodicities that would be absent from a direct analysis of radiative forcing. As observed in late Pleistocene records, geologic data
suggest that climatic nonlinearities can translate high frequency variations into longer period geochemical flux anomalies by mechanisms still unknown. One consequence of the climate–sediment nonlinearities may be to transfer depositional anomalies of carbon and nutrient storage from time scales shorter than the residence times of these elements to 100-kyr, 400-kyr, and even longer scales, which could well affect their mean oceanic inventories. We also suspect, although we do not know yet, that the terrigenous input of carbon to the Cretaceous oceans may have also varied in phase with the clay accumulation cycles observed in the deep sea.
Are There Common Threads?
It would be simplistic to assume that all variations in carbonate and organic carbon time series from the deep sea represent precisely the same climatic response to orbital forcing. The spectral mix of frequencies observed in sediment records in fact varies on time scales of millions of years. It does appear, however, that variations in the deposition of both carbon phases have always been climatically sensitive at the orbital frequencies. If the Pleistocene–Pliocene precedent holds for earlier times, spectra of carbonate time series in marine records give a good representation of the frequency mix of paleoclimatic energy in the entire climatic system. Precessional forcing appears to be a constant in the accumulation of organic and inorganic carbon in the deep sea, and in the influx of terrigenous materials. Obliquity signals appear strongly only after the cooling of the high latitude Southern Hemisphere began.
The persistence of periodicities associated in some way with eccentricity forcing is one of the major surprises that come from looking at older records. Energy at about 100-, 400-, and in some cases, 2,400-kyr wavelengths is especially clear before the onset of large-scale Southern Hemisphere glaciation in the earliest Oligocene, but it also appears in many later carbon records. The dominance of the 41-kyr obliquity cycle in long Oligocene, early Miocene, and early Pleistocene carbon records make it clear that 100-kyr cyclicity is not inherent in the carbonate recorder itself. Rather, eccentricity-related cycles in carbonate content and in carbon isotopic composition most likely reflect real changes in ocean and atmospheric circulation at these frequencies. One of the unresolved puzzles of late Pleistocene climate, the dominance of an orbital term with little direct insolation forcing, therefore grows in importance with a longer time perspective.
What we do not know yet is the extent to which anomalies in marine carbon deposition related to climate change represent not only passive tracers of changes in ocean circulation but also active feedback responses that could modify climate sensitivity. As tracers, the aspects of the carbon system that can be monitored geologically include changes in the path and sources of deep-water formation, changes in wind stress and nutrient inventories to the surface biota, and transfers of carbon between terrigenous and marine reservoirs. Feedbacks implied by ancient carbon cycles range from variable uptake by the ocean of atmospheric carbon dioxide due to changes in temperature and circulation, to anomalies in the global rates of organic and carbonate carbon deposition rate that could affect the long-term greenhouse gas content of the atmosphere. It is particularly important to understand the origin of long-wavelength marine carbon cycles as these could potentially alter factors such as sedimentary storage of nutrient elements and the carbon dioxide content of the atmosphere for long enough periods of time to affect weathering cycles on land and evolution and biogeochemical cycling in the ocean. If the data presented above are representative of ocean history for the past 125 Myr, the gap between the relatively short duration (˜20 and 41 kyr) orbital insolation and tectonic perturbations to climate and biogeochemical cycles may be bridged by nonlinear climate–carbon connections. These may cause changes in the oceanic output of carbon and associated elements on time scales normally considered to fall within the tectonic window (cf. ref. 60).
If we are to look for common threads over time, it is necessary to look for robust mechanisms that do not depend, for example, on the existence of extensive glaciation (for example, see ref. 24), or on other boundary conditions not appropriate for the span of late Mesozoic and Tertiary time. A simple model should also explain why different variables might be coupled in a response to orbital forcing. I suggest that the interaction between radiative forcing, marine sea surface temperatures, and the hydrological cycle may give a rich array of climatic responses at Milankovitch frequencies over long periods of geological time. For the carbon cycle, small changes in the density structure of deep waters can modulate the basin–basin fractionation of alkalinity and nutrients (cf. ref. 57) and explain dissolution patterns imposed on many sediment carbonate records. The existence of carbonate dissolution cycles in basinal deposits during most of the Tertiary and late Mesozoic suggests that there have been at least two regions of deep-water formation, with different initial dissolved inorganic carbon characteristics, throughout this time.
The density differences between potential deep-water-forming areas may be extremely small and would be sensitive to changes in both sea surface temperature and salinity. The Mediterranean may be a small-scale example of this phenomenon. It seems likely that the present evaporative water balance shifted at a number of time in the past to fresher conditions in response to enhanced African monsoons, those regulated by the precessional cycle ( 58 ). During such periods, the exchange of waters between the Atlantic and Mediterranean may have been reversed, leading to a nutrient-trap configuration and the development of anoxic bottom waters and organic-carbon-rich sediment layers ( 61 ).
Because water vapor is such a potent greenhouse gas, changes in sea surface temperature could amplify global temperature anomalies. On land, changes in the water balance could promote or disfavor the storage of organic carbon in soils and biomass. The signs of terrestrial climate change may be imprinted in cycles in the carbon isotopic composition of sea water, and in the variations in deposition rate of terrigenous dust in deep-sea sediments. Geological records hint that all of these responses happen commonly, and that they have important nonlinear aspects that enhance modest initial forcings.
I thank S. D’Hondt, E. Erba, A. G. Fischer, C. D. Keeling, J. Park, and I. Premoli Silva for many helpful discussions, and two anonymous reviewers who helped improve the final manuscript. Portions of this work were funded by the Petroleum Research Foundation of the American Chemical Society and by grants from the National Science Foundation.
1. Hays, J. D. , Imbrie, J. & Shackleton, N. J. ( 1976 ) Science 194 , 1121–1132 .
2. Imbrie, J. , Hays, J. D. , Martinson, D. G. , McIntyre, A. , Mix, A. C. , Morley, J. J. , Pisias, N. G. & Shackleton, N. J. ( 1984 ) in Milankovitch and Climate , eds. Berger, A. , Imbrie, J. , Hays, J. , Kukla, G. & Saltzman, B. ( Reidel , Hingham ), pp. 269–305 .
3. Fairbanks, R. G. ( 1989 ) Nature (London) 342 , 637–642 .
4. Shackleton, N. J. , Imbrie, J. & Hall, M. A. ( 1983 ) Earth Planet. Sci. Lett. 65 , 233–244.
5. Duplessy, J. C. , Shackleton, N. J. , Fairbanks, R. G. , Labeyrie, L. , Oppo, D. & Kallel, N. ( 1988 ) Paleoceanography 3, 343–360 .
6. Raymo, M. E. , Ruddiman, W. F. , Shackleton, N. J. & Oppo, D. W. ( 1990 ) Earth Planet. Sci. Lett. 97 , 353–368 .
7. Barnola, J. M. , Raynaud, D. , Korotkevich, V. S. & Lorius, C. ( 1987 ) Nature (London) 329 , 408–414 .
8. Chappellaz, J. , Barnola, J. M. , Raynaud, D. , Korotkevich, Y. S. & Lorius, C. ( 1990 ) Nature (London) 345 , 127–131 .
9. Short, D. , Mengel, J. G. , Crowley, T. J. , Hyde, W. T. & North, G. R. ( 1991 ) Quat. Res. 35 , 157–173 .
10. Wigley, T. M. L. ( 1976 ) Nature (London) 264 , 629–631 .
11. Imbrie, J. & Imbrie, J. Z. ( 1980 ) Science 207 , 943–953 .
12. Savin, S. M. ( 1977 ) Annu. Rev. Earth Planet. Sci. 5 , 319–355 .
13. Wolfe, J. A. ( 1978 ) Am. Sci. 66 , 694–703 .
14. Spicer, R. A. & Parrish, J. T. ( 1990 ) J. Geol. Soc. (London) 147 , 329–341 .
15. Barron, E. J. ( 1983 ) Earth Sci. Rev. 19 , 305–338 .
16. Arrhenius, G. ( 1952 ) Repts. Swed. Deep Sea Exped. 5 , 1–227 .
17. Hovan, S. A. , Rea, D. K. , Pisias, N. G. & Shackleton, N. J. ( 1989 ) Nature (London) 340 , 294–296 .
18. Shackleton, N. J. , Berger, A. & Peltier, W. R. ( 1990 ) Trans. R. Soc. Edinburgh Earth Sci. 81 , 251–261 .
19. Tiedemann, R. , Sarnthein, M. &. Shackleton, N. J. ( 1994 ) Paleoceanography 9 , 619–638 .
20. Oppo, D. , Fairbanks, R. G. , Gordon, A. L. & Shackleton, N. J. ( 1990 ) Paleoceanography 5 , 43–54 .
21. Berger, W. H. ( 1973 ) J. Foraminiferal. Res. 3 , 187–195 .
22. Shackleton, N. J. ( 1977 ) in The Fate of Fossil Fuel CO2in the Oceans , eds. Andersen, N. R. & Malahoff, A. ( Plenum , New York ), pp. 401–427 .
23. Curry, W. B. & Crowley, T. J. ( 1987 ) Paleoceanography 2 , 489– 517 .
24. Broecker, W. S. ( 1982 ) Prog. Oceanogr. 11 , 151–197 .
25. Sarmiento, J. L. & Toggweiler, J. R. ( 1984 ) Nature (London) 308 , 621–624 .
26. Martin, J. H. ( 1990 ) Paleoceanography 5 , 1–14 .
27. Boyle, E. A. ( 1988 ) J. Geophys. Res. 93 , 15701–15715 .
28. Ruddiman, W. F. , Raymo, M. & McIntyre, A. ( 1986 ) Earth Planet. Sci. Lett. 80 , 117–129 .
29. Park, J. & Maasch, K. ( 1993 ) J. Geophys. Res. 98 , 447–461 .
30. Muller, P. J. & Suess, E. ( 1979 ) Deep Sea Res. 26A , 1347–1362 .
31. Pedersen, T. F. ( 1983 ) Geology 11 , 16–19 .
32. Lyle, M. ( 1988 ) Nature (London) 335 , 529–532 .
33. Sarnthein, M. , Winn, K. , Duplessy, J.-C. & Fontugne, M. R. ( 1988 ) Paleoceanography 3 , 361–399 .
34. Berger, A. , Loutre, M. F. & Dehant, V. ( 1989 ) Paleoceanography 4 , 555–564 .
35. Berger, A. & Loutre, M. F. ( 1991 ) Quat. Sci. Rev. 10 , 297–317 .
36. Berger, A. , Loutre, M. F. & Laskar, J. ( 1992 ) Science 255 , 560–566 .
37. Bloemendal, J. & deMenocal, P. ( 1989 ) Nature (London) 342 , 897–900 .
38. deMenocal, P. B. ( 1995 ) Science 270 , 53–59 .
39. Herbert, T. D. & Mayer, L. A. ( 1991 ) J. Sediment. Petrol. 61 , 1089–1108 .
40. Cotillon, P. & Rio, M. ( 1984 ) Init. Rep. Deep Sea Drill. Proj. 77 , 339–378 .
41. Schwarzacher, W. ( 1987 ) Earth Planet. Sci. Lett. 84 , 22–26 .
42. Hilgen, F. J. ( 1991 ) Earth Planet. Sci. Lett. 107 , 349–368 .
43. Gale, A. S. ( 1995 ) in Orbital Forcing Timescales and Cyclostratigraphy , Geol. Soc. Special Publication 85 , eds. House, M. R. & Gale, A. S. ( Geol. Soc. , London ), pp. 177–197 .
44. Shackleton, N. J. , Curry, W. B. & Richter, C. ( 1995 ) Proc. Ocean Drill. Prog. Init. Repts. 154 , 1–1111 .
45. Mead, G. A. , Tauxe, L. & LaBreque, J. L. ( 1986 ) Paleoceanography 1 , 273–284 .
46. Moore, T. C., Jr. , Pisias, N. G. & Dunn, D. A. ( 1982 ) Mar. Geol. 46 , 217–233 .
47. Woodruff, F. & Savin, S. M. ( 1991 ) Paleoceanography 6 , 755–806 .
48. Herbert, T. D. & D’Hondt, S. L. ( 1990 ) Earth Planet. Sci. Lett. 99 , 263–275 .
49. Huang, Z. , Boyd, R. & O’Connell, S. ( 1992 ) Proc. Ocean Drill. Prog. Sci. Res. 122 , 259–277 .
50. Berggren, W. A. , Kent, D. V. , Swisher, C. C., III & Aubry, M.-P. ( 1995 ) in Geochronology, Time Scales and Global Stratigraphic Correlation , Soc. Econ. Paleontol. Mineral. Spec. Publ. 54 , eds. Berggren, W. A. , Kent, D. V. , Aubry, M.-P. & Hardenbol, J. ( Soc. Econ. Paleontol. Mineral. , Tulsa, OK ), pp. 129–212 .
51. ten Kate, W. G. H. & Sprenger, A. ( 1993 ) Sediment. Geol. 87 , 69–101 .
52. Barron, E. J. , Arthur, M. A. & Kauffman, E. G. ( 1985 ) Earth Planet. Sci. Lett. 72 , 327–340 .
53. Barker, P. F. & Kennett, J. P. ( 1988 ) Proc. Ocean Drill. Prog. Init. Rep. 113 , 293–328 .
54. Herbert, T. D. , Stallard, R. F. & Fischer, A. G. ( 1986 ) Paleoceanography 1 , 495–506 .
55. Park, J. & Herbert, T . D . ( 1987 ) J. Geophys. Res. 92 , 14027– 14040 .
56. Premoli Silva, I. , Ripepe, M. & Tornhaghi, M. E. ( 1989 ) Terra Nova 1 , 443–448 .
57. Berger, W. H. ( 1970 ) Geol. Soc. Am. Bull. 81 , 1385–1402 .
58. Rossignol-Strick, M. ( 1985 ) Palaeogeogr. Palaeoclimatol. Palaeoecol. 49 , 237–263 .
59. Oglesby, R. & Park, J. ( 1989 ) J. Geophys. Res. 94 , 14793–14816 .
60. Lasaga, A. C. , Berner, R. A. & Garrels, R. M. ( 1985 ) in The Carbon Cycle and Atmospheric CO2: Natural Variations Archean to Present , eds. Sundquist, E. T. & Broecker, W. S. ( American Geophysical Union , Washington, DC ), Monogr. 32 , pp. 397–411 .
61. Sarmiento, J. L. , Herbert, T. D. & Toggweiler, J. R. ( 1988 ) Global Biogeochem. Cycles 2 , 427–444 .