20
Chronobiology of the Immune System
Erhard Haus1
Introduction
The human organism at all levels of biologic organization shows an intricate time structure consisting of rhythmic variations in multiple frequencies of most biologic variables studied, which are superimposed on trends like development and aging. Many of the rhythms are genetically fixed. The inheritance of rhythm characteristics and genes and gene products determining the occurrence, the extent, and the timing of the rhythmic variations have been described (Feldmann, 1985; Konopka, 1979; Lakatua, 1994; Lee et al., 1996; Myers et al., 1996; Peleg et al., 1989; Reinberg et al., 1985; Rensing, 1997; Young, 1993; Young et al., 1985). Biologic rhythms of various frequencies were found in single cells and in cell organ cultures removed from the organism and studied in vitro (Edmunds, 1994; Milos et al., 1990; Morse et al., 1989; Schweiger et al., 1986). In vivo, these numerous oscillators are adjusted within the organism in their timing by pacemakers through humoral and/or neural
messages synchronizing the target cells or organs. In turn, many target cells provide feedback information in the form of humoral signals, for example, through cytokines or polypeptide hormones and neurotransmitters that act on the superimposed neuroendocrine structures and modify their response (Blalock, 1992, 1994). The interactions of the neuroendocrine and immune system are time dependent and follow rhythmic patterns in multiple frequencies. In some frequencies, the rhythms are adjusted in time (externally synchronized) by periodic environmental factors like light-darkness, social routine, the work schedule, and to some extent the time of food uptake. The spontaneous rhythms encountered are complemented by reactive, rhythmic response patterns to environmental stimuli, some of which may equally be genetically fixed (endogenous) in nature. Such rhythmic response patterns can be triggered by single stimuli (e.g., the introduction of an antigen) and in their timing relate to the time of stimulation and not to environmental rhythms, for example, the calendar week. Environmental stimuli may also change some rhythm parameters like timing or amplitude transiently for only as long as the environmental stimulus persists (''masking'' the rhythm).
The complexity of the human time structure and its adaptation to changing environmental conditions, presumably including geophysical factors (Breus et al., 1989; Lipa et al., 1976; Sitar, 1991), make it necessary to qualify the assumption that sampling at a fixed time of the day, the week, and/or the season will "control" rhythmic variables, and that the need for the observation of rhythms could be avoided.
The rhythmically changing physiologic state of the organism determines the response to environmental stimuli like physical exercise, pain perception, mental stress, toxic substances, bacterial and viral infections, antigenic stimulation, and drugs used in clinical medicine. The state of the host organism at the time of stimulation often determines critically the response to a stimulus in extent, and in some instances, in direction. This is shown dramatically in the response of mice to the injection of Escherichia coli endotoxin (Figure 20-1). Death or survival can be made experimentally a function of the time when the agent is injected.
The development of an immune response involves a series of interactions between lymphocytes and other mononuclear cells that may include cell-to-cell communication; generation of immunoreactive molecules; immunoglobulin synthesis and secretion; expression of cell surface markers that are not generally found on resting cells or changes in their receptor density and activity; and finally cell proliferation of immunocompetent cells. An effective immune response requires a balance in the function of lymphocyte subsets (e.g., helper and cytotoxic T-cells, and antibody-producing B-cell lymphocytes), macrophages, and natural killer (NK) cells, and a balance in the production of enhancing or inhibiting soluble factors or cytokines as expressed in the Th1 or Th2 response to immune stimulation. Each of these factors is directly or indirectly under the influence of neuroendocrine rhythmic variables directing
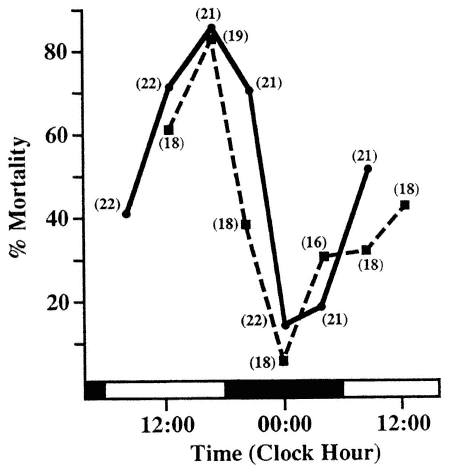
FIGURE 20-1
Circadian rhythm in susceptibility of Balb/C mice to intraperitoneal (i.p.) injection of Escherichia coli endotoxin. The same dose injected at different circadian stages into subgroups of comparable animals kept at a lighting regimen of Light-Dark (LD) 12:12 leads to dramatic differences in response (two studies show high degree of reproducibility). SOURCE: Adapted from Halberg et al. (1960) and Haus et al. (1974b).
components of the immune response and in turn provides signals to other immunocompetent cells or to the superimposed neuroendocrine centers via feedback regulation. Rhythmic variations in several frequencies are found in every step of the development of an immune response in the humoral as well as the cellular arm of the immune system. Rhythmic variations of the immune response in its different aspects have been studied most extensively in the circadian frequency range. Circadian rhythmic variations in hormone secretion or in the effect of humoral messengers on the target tissue caused by changes at the receptor level (Lakatua et al., 1986) or the interaction with other humoral factors can increase or decrease the number and functional activity of the immune-competent cells in the peripheral blood and/or in lymphoid and other tissues. These changes lead to differences in the immune response during certain stages of the circadian cycle, which may be of importance both for the response to a primary antigenic stimulation or to a challenge of the immunized organism. Disturbances of the usual temporal pattern in the function of the different cells of the immune system are found in disturbances in the immune response (e.g., in states of immunodeficiency) or may lead to autoantibody production with the development of a chronic autoimmune disease.
More recently, rhythms of about 7-day duration (circaseptan rhythms), synchronized or not by the environmental social week, have been recognized for their importance in the human time structure (Cornélissen et al., 1993; Levi and Halberg, 1982). Some of them appear to be endogenous in nature and may be found free running from known environmental time cues (Halberg and Hamburger, 1964). In addition to the spontaneously occurring circaseptan rhythms, a circaseptan response pattern characterizes the mammalian organism. After exposure to an environmental load, for example, to anoxic kidney damage (Hübner, 1967) or the exposure to an antigen (DeVecchi et al., 1981) or treatment with immunosuppressive agents (Hrushesky and März, 1994; Many and Schwartz, 1971), the response of the organism occurs in a rhythmic fashion with approximate (but not always exactly) 7-d periods. This response pattern is unrelated to the calendar day of the week but is triggered by the one-point stimulation at the time of exposure to the stimulus (e.g., the introduction of an antigen).
Seasonal variations as they are observed in the immune response may be due to environmental factors like differences in the length of the daily light span, temperature differences, and differences in exposure to a variety of antigens, including microorganisms. The environmental effects on the immune system may be mediated by seasonal changes in the function of the pineal gland (light-dark related), the thyroid gland (temperature related), or prolactin (PRL) (Arendt, 1994; Haus et al., 1980, 1988; Nicolau and Haus, 1994). However, rhythms of approximate duration of 1 year may in certain functions also be endogenous and presumably genetically fixed (so-called circannual rhythms). Circannual rhythms have been observed experimentally in animals, who for generations were kept under light- and temperature-controlled experimental laboratory conditions and have never been exposed to variations in day length or temperature (Haus et al., 1984, 1997; Haus M. et al., 1984). Figure 20-2 shows the circannual variations in cell proliferation as measured by the 3H-thymidine uptake in DNA of lymphatic organs and of the hematopoietic and lymphatic cells in the bone marrow in mice subgroups of which were studied over 2 years and measured during each season over a 24-h span with the circadian mean plotted in the figure. It is of interest that there appear to be phase differences between different lymphatic organs and the bone marrow. Other evidence about the endogenous nature of circannual variations is the observation of free-running circannual rhythms of body functions in human subjects (Haus and Touitou, 1994a).
The intricate web of neuroendocrine-immune regulation makes a separate discussion of some of its components artificial. However, experimental design and clinical studies can follow only a limited number of variables and end points. In presenting the results of studies of the time structure of certain parts of this complex system, it must be realized that each observation has to be understood in the context of its role within the framework of the system as a whole.
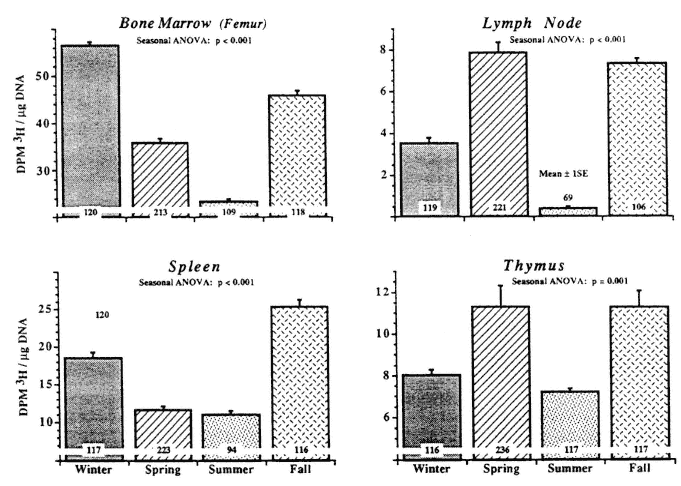
FIGURE 20-2
Circannual rhythm of 3H-thymidine uptake into DNA of lymphoid tissues and bone marrow of BDF1 male mice kept for several generations on artificial lighting (LD 12:12), under controlled temperatures, and fed the same mouse chow. The animals were studied over an entire 24-h span (six sampling times at 4-h intervals) in several studies per season during 2 consecutive years (results pooled). A circannual rhythm is found as a group phenomenon without known environmental seasonal variations. Note phase difference among organs. SOURCE: Adapted from Haus et al. (1997).
Biologic Rhythms in the Number and Function of Circulating White Blood Cells
The numbers of circulating white blood cells involved in the defense of the human body show high-amplitude circadian variations. Regularity, timing, and amplitude of these rhythms vary among the different cell types, and among different age groups and populations examined (e.g., Haus, 1959, 1994, 1996; Haus et al., 1983, 1988; Swoyer et al., 1989). In the peripheral blood, the periodic changes in the number of circulating leukocytes may be the result of multiple factors, such as distribution between the circulating and the marginal cell compartments, distribution among different tissues and organs of the body, influx from storage sites, proliferation of cells, release of newly formed cells into the circulation, and cell destruction and removal. Several or all of these factors may contribute to the number of circulating white blood cells, and these factors will vary in their importance from one cell type to the other, which may lead to differences in timing and amplitude of each circadian (or other) rhythm. In considering the relative importance of the number of circulating immune-
competent cells for defense reactions and the immune response of the body, it must be realized that with some cell types, only a very small percentage of the cells is circulating. For example, in lymphocytes, the circulating cells are fewer than 1 percent. The much larger fraction is found in the lymphatic organs, bone marrow, and other tissues (e.g., the lamina propria and submucosa of the intestinal and respiratory tract).
Figure 20-3 shows the circadian rhythms of the circulating white blood cells and platelets in 150 clinically healthy young adults (24 ± 10 years of age) following a diurnal activity pattern with rest during the night from approximately 23:00 to 06:30.
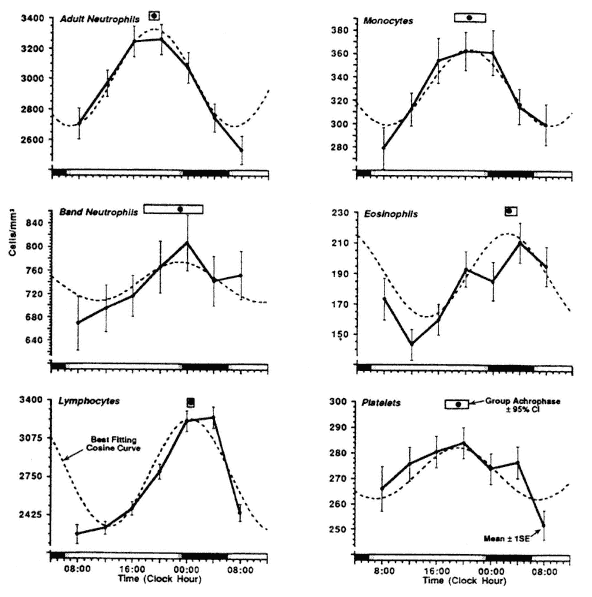
FIGURE 20-3
Circadian rhythm in circulating white blood cells in 150 clinically healthy adult subjects. Each subject sampled six times over a 24-h span. Data are shown with best fitting cosinor curve and circadian group-acrophase derived from cosinor analysis (Nelson et al., 1979). The small 95 percent confidence interval of the acrophase is due to group size and hides a substantial degree of individual variability. SOURCE: Adapted from Haus (1994, 1996); Haus et al. (1983, 1988).
The circadian variations in circulating white blood cells are consistent and highly reproducible as group phenomena. However, there is a substantial individual variation and in some of the 150 apparently clinically healthy subjects summarized as a group in Figure 20-3, the peak or trough of the values measured could be found at any clock hour of the day (Haus, 1996). The regularity and timing of the rhythms also varies by cell type. In neutrophil leukocytes, the location of the trough values is more consistent and reproducible than that of the peak values. Lymphocytes show a very regular rhythm with both peak and trough equally conspicuous. Circulating monocytes and eosinophils show more variability within and between individuals.
The circadian rhythm in circulating eosinophil leukocytes shows an apparent relation to the circadian rhythm in corticosteroid concentration, and exogenous corticosteroids and stress suppress the number of circulating eosinophils (Haus, 1959). The circadian change in the number of circulating eosinophils (rather than the absolute value) has been used in the past for the assessment of adrenal cortical function (Halberg and Stephens, 1959; Halberg et al., 1958; Haus, 1959), and the change in their number under stress conditions may still be of some interest, as an indicator (at least in part) of the peripheral response of a target tissue to corticosteroids.
TABLE 20-1 Peak-Trough Difference and Range of Change in Circulating Leukocytes (173 subjects) and Platelets (87 subjects) over a 24-h Span in Clinically Healthy Subjects
|
Peak-Trough Difference (cells/mm3) |
% Range of Change [(highest – lowest/lowest) × 100] |
||
|
Mean ± Standard Deviation |
Range |
Mean ± Standard Deviation |
Range |
Total white blood cells |
2,400 ± 1,000 |
400–6,100 |
41 ± 18 |
7–133 |
Total neutrophils |
1,840 ± 1,028 |
480–7,168 |
66 ± 42 |
20–346 |
Adult neutrophils |
1,567 ± 856 |
330–6,347 |
72 ± 43 |
18–344 |
Bands |
563 ± 350 |
56–1,790 |
152 ± 114 |
15–780 |
Lymphocytes |
1,616 ± 770 |
331–5,556 |
84 ± 41 |
14–234 |
Monocytes |
366 ± 167 |
80–974 |
277 ± 221 |
30–1,560 |
Eosinophils |
230 ± 110 |
40–816 |
292 ± 190 |
64–1,031 |
Basophils |
105 ± 57 |
0–390 |
315 ± 175 |
27–658 |
Platelets (× 103) |
54 ± 32 |
12–198 |
23 ± 15 |
3–83 |
The extent of the circadian variation encountered in 173 apparently clinically healthy subjects (irrespective of timing in each individual) is shown in Table 20-1. The within-day (circadian) peak-trough difference is expressed in absolute numbers at the left and as percent range of change at the right (the extent of change expressed in percent of the trough value). Also in the extent of the rhythms, there are very marked individual variations in an apparently clinically healthy population following a regular routine without known unusual stress or infection.
The variability in the absolute number of cells, and in the timing of peak, and trough, and extent of the circadian variation suggest a clinical usefulness for time-qualified reference values for the evaluation of single cell counts mainly in circulating lymphocytes (Figure 20-4). The reference value for the number of circulating lympocytes in blood drawn early in the morning is different from that in blood drawn at noon. However, the extent of change in the different cell types, which can occur spontaneously in a given individual within a 24-h span must be kept in mind for the evaluation of consecutive measurements in the same patient. Circadian periodicity has to be taken into account in studies in which circulating cell numbers serve as end points.
In clinically healthy subjects, the circadian rhythm in the number of circulating lymphocytes is a very regular and highly reproducible phenomenon. The peak values occur in most diurnally active human subjects during the night
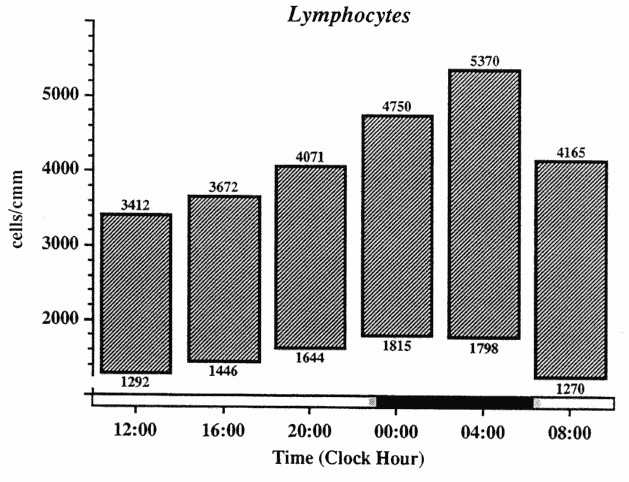
FIGURE 20-4
Circadian variation in usual range (expressed as fifth and ninety-fifth percentile) of numbers of circulating lymphocytes in 150 clinically healthy subjects studied over a 24-h span.
SOURCE: Adapted from Haus (1994, 1996) and Haus et al. (1983).
proportion as part of the total circulating lymphocyte population. The most consistent variations were found in the CD3+ (mature T-cells) and CD4+ hours, with the highest values found between midnight and 01:00. Also the subsets of lymphocytes show a circadian rhythm in their number and/or their (helper inducer cells), while in the CD8+ (suppresser cytotoxic cells) some investigators reported no significant circadian rhythm as a group phenomenon (Ritchie et al., 1983) or a 12-h rhythm only (Levi et al., 1985, 1988a). In this author's laboratory, a prominent circadian rhythm was evident also in the CD8+ cells, with an additional shorter frequency apparent in the men (Haus, 1994) (Figure 20-5). The circadian variation in the number of circulating lymphocytes persisted under conditions of sleep deprivation (Ritchie et al., 1983). A circadian rhythm in E-rosette-forming (T) cells was found in vitro in aliquots of
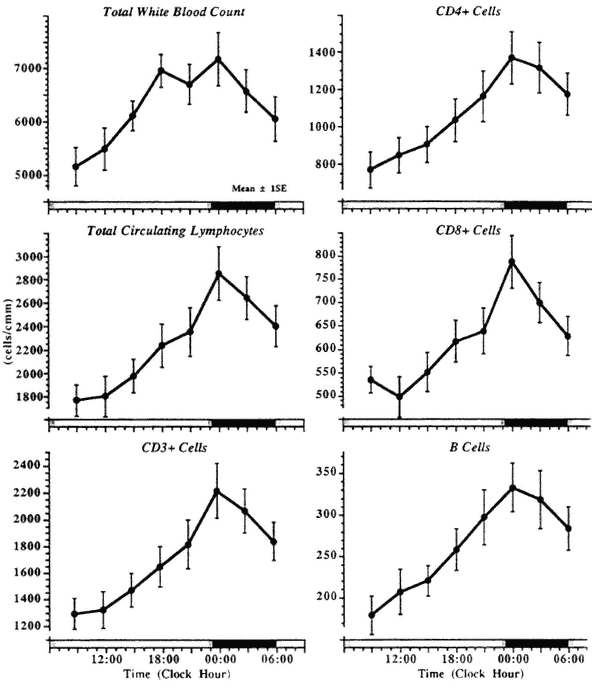
FIGURE 20-5
Circadian variation in lymphocyte subtypes in 10 clinically healthy subjects. (The double peak in "total white blood count" is due to the earlier circadian peak of neutrophils.)
blood samples studied over 24 hours and was reported to continue in 4-d-old cell cultures (Gamaleya et al., 1988). These in vitro studies suggest that the rhythmic fluctuations in at least some subpopulations of blood lymphocytes depend on circadian changes in the cells (a cellular oscillator) rather than on extracellular factors such as the circadian rhythm in plasma cortisol. However, cortisol may act as a synchronizer, or if given exogenously, it can transiently alter (mask) the circadian rhythm.
Not only the number of circulating lymphocytes but also several aspects of lymphocyte function show circadian periodicity. Circadian periodic variations in the response to external stimuli were recognized experimentally in changes of the number of cells in the peripheral blood (Figure 20-6) and bone marrow (Figure 20-7). In some studies, the T-cell response to phytohemagglutinin (PHA) was found to be circadian periodic with a remarkably large amplitude (Haus et al., 1974a, 1983; Tavadia et al., 1975) (Figure 20-8). However, there appears to be some variability in circadian timing of this rhythm, possibly due to seasonal or other factors that may not allow one to recognize a rhythm if sampling does not occur at the "right" times, for example, if only two time points are selected according to clock hour rather than according to the stage of the rhythm. Mixed lymphocyte reactions (MLRs) in PHA-activated T-cells and autologous non-T-cell activation showed statistically significant circadian variations with proliferation response of T-cells in both types of autologous MLRs, but with an apparent phase difference between them. The difference in timing of the two types of autologous MLRs may be due to the different types of cells responding (Damle and Gupta, 1982).
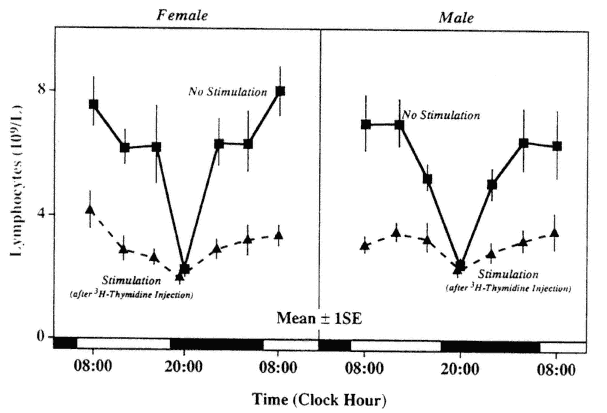
FIGURE 20-6
Circadian variation in lymphocytopenic response to handling and intraperitoneal injection of 3H-thymidine (1 µc 3H-thy in 0.2 ml saline/20 g body weight) in male and female Balb/C mice (sampling 2 hours after treatment).
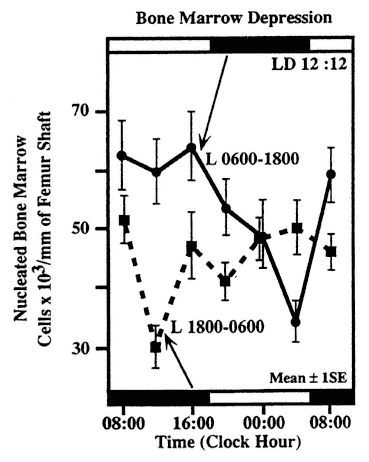
FIGURE 20-7
Circadian rhythm in sensitivity of nucleated cells in the bone marrow of Balb/C mice. Subgroups of mice were exposed at different circadian stages to 350 rad whole body x-irradiation. Half of the animals were kept on a lighting regimen of LD 12:12 with light from 06:00 to 18:00, the other half for 3 weeks prior to the study on LD 12:12 with light from 18:00 to 06:00. SOURCE: Adapted from Haus et al. (1974b, 1983).
For some investigators, the predominant B-cell activation by pokeweed mitogen (PWM) showed a circadian variation (Haus et al., 1983; Moldofsky et al., 1986), while for other investigators it did not (Indiveri et al., 1985). It appears that seasonal (Canon et al., 1986; Gamaleya et al., 1988) and perhaps other rhythmic and nonrhythmic variations may play a role in the modification of lymphocyte functions. Sleep deprivation of 40 hours led to a phase alteration of the circadian rhythm in the lymphocyte response to PWM but not to PHA (Moldofsky et al., 1989).
A circadian rhythm was reported for urinary neopterin excretion (Auzeby et al., 1988) which indicates T-cell activation (Huber et al., 1984). The peak of urinary neopterin (06:30) followed that of the circulating lymphocytes in the same clinically healthy subjects by about 2 hours (Levi et al., 1988a). Figure 20-9 shows the circadian rhythm in serum neopterin in patients with rheumatoid arthritis.
In a study that took samples at two time points, the frequency of sister chromatin exchange in human blood lymphocytes was significantly less at 09:00 than at 21:00 which suggests a circadian variation of this parameter (Slozina and Golovachev, 1986). This variation may be of importance for the lymphocyte
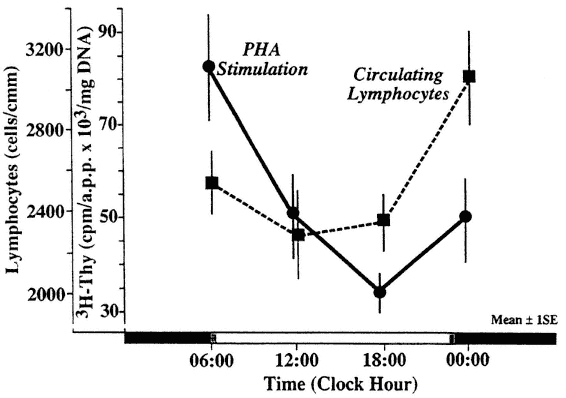
FIGURE 20-8
Circadian rhythm of lymphocytes in response to phytohemagglutinin (PHA). Lymphocyte cultures containing 1 to 2 × 106 cells/2 ml culture were set up with a 1:100 dilution of PHA. Five hours before the end of the 48-h incubation span, 50 λ (approximately 20 µCi, 1.9 Ci/µmole) 3H-thymidine was added to each culture tube. After 5 hours, the reaction was terminated and DNA was measured colorimetrically in triplicate cultures. An aliquot of the same sonicate was counted in a liquid scintillation counter. Counts per minute of acid-precipitable protein (a.p.p.) were adjusted for 1.0 × 106 of inoculated cells. NOTE: The rhythm in PHA response is out of phase with rhythm in the number of circulating lymphocytes. SOURCE: Adapted from Haus et al. (1983).
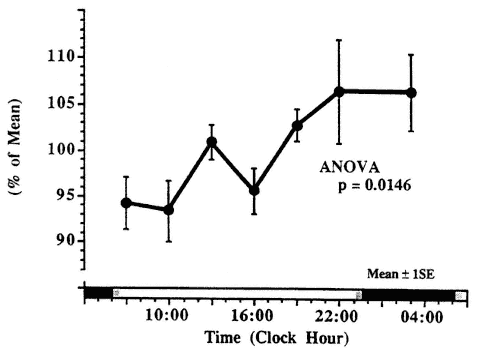
FIGURE 20-9
Circadian variation in serum neopterin concentration in 29 patients with rheumatoid arthritis. SOURCE: Adapted from Suteanu et al. (1995).
response to damage caused by a number of environmental stimuli, including radiation and certain carcinogenic agents.
Circadian variations in lymphocyte adrenoreceptor density were reported, with peak values around noon and a trough around midnight (Pangerl et al., 1986). Circannual variations of the same variable were found with high values in spring and summer and low values during winter (Haen et al., 1988; Pangerl et al., 1986). Disturbances in the expression and function of adrenoreceptors are thought to play a role in the pathobiology of, for example, hypertension and bronchial asthma (Pangerl et al., 1986), both of which show circadian and circannual chronopathology in their disease manifestations.
A circadian variation in the glucocorticoid receptor content of lymphocytes was reported with the values at 23:00 being 38 percent higher than at 08:00. The receptor affinity did not change over the 24-h span, and there was no seasonal variation in number and affinity of glucocorticoid receptors (Homo-Delarche, 1984).
The NK cell activity in the peripheral blood of clinically healthy adult subjects shows a reproducible circadian rhythm, with the activity being high in the early morning and declining to a minimum during the night hours (Abo et al., 1981; Angeli et al., 1992; Gatti et al., 1986, 1988; Moldofsky et al., 1986, 1989; Williams, et al. 1979). The NK cell activity is out of phase with the number of circulating lymphocytes of most other subtypes. There was a circadian rhythm in the response of NK cell activity to stimulation with interferon-gamma (INF-γ), which was synchronous with the spontaneous NK cell activity (acrophase2 around 04:00) (Angeli et al., 1992; Gatti et al., 1988). Corticosteroids are potent inhibitors of NK cell activity (Herberman and Callewaert, 1985; Richtsmeier, 1985). The maximum inhibitory effect by glucocorticosteroids was found earlier during the night preceding the peak in spontaneous NK cell activity (Angeli et al., 1992; Gatti et al., 1987).
The chronic administration of melatonin leads to a substantial increase in spontaneous NK cell activity and in the responsiveness of those cells to INF-γ (Angeli et al., 1992; Gatti et al., 1990). The decrease in melatonin production during aging may also contribute through this mechanism to the lower immunocompetence of the elderly. Investigations by Gatti et al. (1988) were suggestive of a seasonal (circannual) variation of NK cell activity.
Alterations of the circadian periodicity of human lymphocytes have been reported in patients with lymphoid tumors (Swoyer et al., 1975). Infection with the lymphotrophic human immunodeficiency virus (HIV) leads to alterations in the circadian rhythm of the circulating lymphocytes as an early event in the development of the AIDS related complex (ARC) and AIDS (Bourin et al., 1989; Martini et al., 1988a,b; Swoyer et al., 1990). Circadian periodicity in hemoglobin, hematocrit, and the number of circulating red blood cells is maintained with comparable timing, but with significantly lower amplitude in
HIV-infected patients (Swoyer et al., 1990). No day-night synchronized circadian rhythm was demonstrable as a group phenomenon in the HIV-infected patients in the number of circulating neutrophils, monocytes, total lymphocytes, CD3+, CD8+, and B1+ lymphocytes or in platelets. However, in individual HIV-infected patients, marked variations in the numbers of the circulating leukocytes over the 24-h span did persist but without the usual environmental synchronization (Haus, 1994, 1996; Swoyer et al., 1990). In the same patients, the circadian rhythm in circulating cortisol was maintained as a group phenomenon, with the circadian mean serum concentration and amplitude comparable to those of healthy controls (Haus, 1994, 1996). The circadian rhythm alterations in polymorphonuclear leukocytes and monocytes in HIV-infected subjects indicate a wider disturbance of the hematopoietic system than just in the lymphocyte series. Although the rhythm disturbances are more pronounced in the more severely affected patients, circadian rhythm disturbances appear to be an early consequence of HIV infection.
Circaseptan about 7-day variations have been found in many immune-related parameters (for reviews, see Haus and Touitou, 1994a, b; Haus et al., 1983, 1988; Levi and Halberg, 1982) and seem to represent a genetically fixed response pattern of the humoral as well as of the cell-bound immune system to an immunologic stimulus. Treatment with an immunosuppressive agent, melphalan, was shown to trigger a cyclic response pattern (with slightly longer than 7 day period) of immune regeneration in mice (Many and Schwartz, 1971). This response to a single stimulus points to the endogenous nature of the circaseptan organization of the immune system. Circaseptan rhythms are found in hematologic recovery after partial bone marrow suppression (Morley et al., 1970) and thus may develop during cancer chemotherapy. Circaseptan susceptibility rhythms of rodents to chemotherapeutic agents have been described (Bixby et al., 1982; Liu et al., 1986), but they need to be studied further in humans. Circaseptan variations in the numbers of circulating T- and B-cells were observed during the development of an antigenically triggered encephalomyelitis in guinea pigs. In this animal model, the peak in the number of circulating T-cells occurred at the time of the trough in the number of B-cells. The relapse occurred when the number of circulating T-cell lymphocytes was low (Raine et al., 1978). Nonimmunological stressors (such as a high salt diet in rats) can also lead to circaseptan response patterns (Uezono et al., 1987).
Circannual variations in the relative number of circulating T- and B-lymphocytes as determined by a bacterial adhesion test were described in clinically healthy human subjects, with the peak of the T-cells in late fall and of the B-cells in winter. The circannual variation was apparent in the subtypes only; the total number of lymphocytes in this study showed no statistically significant circannual variation (Bratescu and Teodorescu, 1981). Levi et al. (1988b) described circannual changes in the number and circadian rhythm characteristics of circulating total lymphocytes (with circannual acrophase of the circadian mean in November), Pan-T-cells (acrophase in March), suppressor-
cytotoxic T-cells (acrophase in December), and NK cells (acrophase in October).
Seasonal variations in the response of human (Shifrine et al., 1982a) and canine (Shifrine et al., 1980, 1982b) lymphocytes to PHA-induced transformation suggest a circannual cycle in cell-mediated immunity. The peak in PHA response was found during summer and the low response during winter.
Phase adaptation of hematologic rhythms is seen in human subjects after changes in their sleep-wakefulness pattern and their activity-rest cycles as encountered in shift workers or in subjects exposed to transmeridian flights over several time zones (Haus, 1994; Haus et al., 1988; Sharp, 1960). However, there is a considerable interindividual variation in shift-work adaptation. The phase adaptation to a 12-h difference in shift (e.g., change from night shift to day shift) may in some subjects require several weeks to adapt the timing of the circadian rhythms in plasma cortisol and in circulating lymphocytes to the changed light-dark and activity-rest schedule serving as environmental synchronizer (Haus and Touitou, 1994 a,b; Reinberg and Sindensky, 1994). A full-phase adaptation to the shift schedule may not occur at all in nonpermanent shift workers or in permanent shift workers if the subjects do not fully change their living habits during the week and return to a diurnal activity pattern on weekends (Haus et al., 1984).
The susceptibility-resistance cycles of the hematopoietic cells to agents used in the treatment of human malignancies including leukemia and solid tumors, are of considerable interest (Bjarnason and Hrushesky, 1994; Haus and Halberg, 1978; Haus et al., 1972, 1983; Hrushesky and März, 1994; Levi, 1994, 1996a, 1997; Levi et al., 1986, 1994; Rivard et al., 1993). Many chemotherapeutic agents are immunosuppressive, and circadian cycles of susceptibility and resistance to these toxic agents are also found in the immune system. A circadian rhythm of sensitivity of mice to whole body irradiation could be shown to parallel the number of surviving nucleated cells in the bone marrow studied 4 days after exposure to a single dose of 350 rads x-irradiation (Haus et al., 1974a, 1983) (Figure 20-7). In the same study, a phase alteration in the timing of maximum and minimum sensitivity could be obtained by a change in lighting regimen, which, however, after 2 weeks of exposure to the altered lighting regimen was not complete, indicating a longer time required to accomplish a phase shift of this nature. The myelotoxicity of recombinant human INF-α in mice, measured by a decrease in the number of white blood cells and the number of bone marrow myeloid precursors, was lowest when the agent was administered in the middle of the rest span. In contrast, the least toxicity of murine INF-γ was found during the mid-activity span (Koren and Fleischmann, 1993). Thus, different forms of interferon show a remarkable difference in the circadian timing of their effects. The susceptibility-resistance cycles to a large number of chemotherapeutic agents have been documented in nocturnal rodents with characteristic timing of the acrophase for various agents (Levi et al., 1986). For human application on a purely empirical basis, a 12-h
time difference was chosen as a guideline for the timing of chemotherapy in diurnally active human subjects. Thus far, this seems to have been successful, although the animal data on circadian timing in peripheral blood and bone marrow are sometimes at variance with the simplified assumption of a 12-h time difference (Haus et al., 1983).
Biologic Rhythms in Cytokines and Their Inhibitors
The central nervous system (CNS), endocrine, and immune systems respond to physiological and pharmacological stimuli in a coordinated manner with bidirectional communication between the systems (Beneviste, 1992; Blalock, 1992, 1994; Rivier and Rivest, 1993; Smith, 1992). The immune-competent cells recognize and respond to the introduction of antigens, to exposure to bacterial and other toxins, and to other tissue damage and initiate the appropriate defense. Signaling among the diverse forms of cells, including lymphocytes, macrophages, fibroblasts, and vascular endothelia, occurs through locally produced substances, including hormones and cytokines.
Cytokines are relatively small proteins or glycoproteins, most of which are characteristically multifunctional and exert their biologic activity in an autocrine and paracrine fashion in or close to the cells of origin. In addition, some cytokines are released into the circulation and exert endocrine effects on target tissues remote from the cell of origin, for example, on cells of the hematopoietic and immune systems, cells involved in inflammatory reactions, and a variety of mesenchymal (e.g., bone forming) and epithelial tissues (e.g., in breast, prostate). These circulating cytokines also act on certain hypothalamic centers and on cells of endocrine glands that in turn regulate cytokine production (Besedovsky and Del Rey, 1996; Spangelo and Gorospe, 1995).
The close interaction between cytokines and the neuroendocrine system leads, in some instances, to feedback regulation, for example, between the pro-inflammatory cytokines (especially interleukin [IL]-1), which are produced at the site of tissue injury, and the strongly anti-inflammatory response elicited in response to cytokine action on hypothalamic centers through the production and release of corticotropin-releasing factor (CRF), adrenocorticotrophic hormone (ACTH), and corticosteroids. Intravenous doses of IL-6 result in a dose-dependent increase in plasma ACTH levels. Antibodies to CRF block the rise in plasma ACTH induced by IL-1 and IL-6 (Naitoh et al., 1988). The increased release of hormones such as ACTH and corticosteroids in the course of immune challenges is considered important as a regulatory mechanism that provides negative feedback in response to overproduction of interleukins (Besedovsky and Del Rey, 1992; Rivier and Rivest, 1993). The immune system serves, through the production of cytokines, as a sensory organ that signals the exposure to antigen (e.g., bacterial or viral invasion, bacterial toxins) to the CNS and endocrine system, which then respond to the challenge and provide for the body's defense but at the same time limit the inflammatory and related
responses and prevent damage by overshoot of the inflammatory and immune defenses, leading to a balanced host response to environmental stimuli.
The circadian stage and the temporal sequence in which cytokines reach the hypothalamic oscillators in the CNS and/or endocrine organs may in part determine the particular response elicited and/or the alterations in the immune and endocrine systems as they are observed in some autoimmune diseases (Berczi et al., 1993). Little is known about rhythms in the auto- and paracrine action of cytokines at the sites of ongoing immune reactions, although in view of the multifrequency periodicities of the neuroendocrine regulation of inflammatory and immune reactions, and the periodicity found at the cellular level, rhythmic variations of these variables have to be assumed.
The circadian periodicity in the various components of the immune system also leads to circadian rhythmicity of cytokines and some of their soluble receptors or receptor antagonists released into circulation (Bourin et al., 1990; Sothern et al., 1995; Suteanu et al., 1995; Young et al., 1995). A circadian rhythm in IL-1 concentration was found in the serum of clinically healthy subjects (Bourin et al., 1990). Cytokines, including IL-1β and tumor necrosis factor (TNF), may be involved in the physiologic alternation of sleep and wakefulness (Krueger, 1990). Higher values of IL-1β and IL-6 were reported in clinically healthy subjects during nighttime sleep (Covelli et al., 1992; Gudewill et al., 1992). Peaks in serum IL-1 and IL-2-like activity occur shortly after sleep onset in healthy human subjects (Gudewill et al., 1992; Moldofsky et al., 1986).
A circadian rhythm has been described for the responsiveness of T-cells to IL-2 (Levi et al., 1991). In a whole blood assay, there was a large-amplitude circadian variation in tetanus toxoid and purified protein derivative of tuberculin (PPD)-stimulated production of INF-γ with highest values during the evening and nighttime (Petrovsky et al., 1994), which was about 12 ± 1 hours out of phase (by cosinor analysis) with the circadian variation in plasma cortisol. A seasonal variation of INF-γ production by mitogen-stimulated T-cells has also been reported (Katila et al., 1993).
IL-6 is a multifunctional cytokine that is produced by various types of lymphoid and nonlymphoid cells and that plays a central role in host defense mechanisms and in inflammation and immune responses (Mackiewicz et al., 1995) and exerts a protective role against lipopolysaccharide-induced (TNF-γ and IL-1 mediated) shock (Barton and Jackson, 1993), the mortality from which in animal experiments shows a very large amplitude circadian rhythm (Halberg et al., 1960; Haus et al., 1974a). The serum concentrations of IL-6 show a circadian rhythm in clinically healthy men, with high values during the night with a peak around 01:00 and a trough around 10:00. The range of change from the lowest to the highest value during a 24-h span ranged from 120 to 531 percent (Sothern et al., 1995). In the same subjects, Young et al. (1995) found a circadian rhythm in IL-2 with a peak during midday (12:48) and a range of change between 31 and 1,367 percent. In contrast, two daily peaks were observed for IL-10 (07:30 and 19:30; range of change between 8 and 266%) for
granulocyte macrophage-colony stimulating factor (GM-CSF) (13:30 and 19:30; range of change between 32 and 12,684%), and TNF-γ (07:30 and 13:30 with a range of change between 16 and 131%). Spontaneous in vitro secretion of IL-1, IL-6, and TNF-γ was higher when the lymphocytes were collected during the day (Zabel et al., 1990, 1993).
A circadian rhythm in soluble IL-2 receptor was found in clinically healthy subjects, with a peak value around 12:30 and a trough value around 04:15 (Lemmer et al., 1992). In patients with rheumatoid arthritis, circadian rhythms were found in IL-6 (Arvidson et al., 1994; Suteanu et al., 1995) and neopterin, a product of T-cell activation (Suteanu et al., 1995). Statistically significant within-day variation, presumably representing ultradian rhythms, were found in patients with rheumatoid arthritis in soluble IL-2 receptor, IL-1β, TNF-γ, and soluble TNF receptors I and II (Suteanu et al., 1995).
Human peripheral leukocytes infected by virus or treated with endotoxin will synthesize immunoreactive corticotropin and endorphins in addition to cytokines. The immunoreactive ACTH produced by the immunocompetent cells appears to be identical to pituitary ACTH, and appears to act on the same receptors in the target tissues and shows a steroidogenic response in mice. The production of ACTH both by pituitary cells and leukocytes in response to synthetic CRF is suppressed by dexamethasone in vitro and in vivo, which suggests that the production of ACTH and endorphins by leukocytes is controlled by CRF (Smith et al., 1986).
Biologic Rhythmicity of the Humoral Immune Response
Both the time of exposure of immunocompetent cells to an antigen (Fernandes et al., 1977, 1980; Pownall et al., 1979) and the response to challenge of the immunized organism after reintroduction of the antigen show circadian periodic variations in human subjects and in animals (Cove-Smith et al., 1978; Fernandes et al., 1976, 1977, 1980; Lee et al., 1977; Pownall et al., 1979). In animal experiments, the instensity of the humoral immune response to T-cell-dependent and independent antigens shows circadian periodicity (Fernandes et al., 1974, 1976; Pownall and Knapp, 1980). After immunization, circadian periodic variations in antibody titer and in the number of plaque-forming cells (PFC) were found in mice in response to sheep red blood cell (SRBC) antigen (Fernandes et al., 1974). The fewest PFC were seen when the antigen was injected close to the daily dark span, corresponding to the time of the highest corticosterone concentrations in nocturnal rodents (Calderon and Thomas, 1980; Fernandes et al., 1976).
The concentrations of serum proteins that are important for immune function, including the immunoglobulins and several other components of serum proteins, undergo circadian periodic changes (Halberg et al., 1977; Haus et al., 1983, 1988; Hayashi and Kikuchi, 1982; Reinberg et al., 1979; Scheving
et al., 1968, 1977). In clinically healthy subjects, the peak concentrations of all three major classes of immunoglobulins occurred in the early to late afternoon (e.g., Reinberg et al., 1979).
In patients with some clinical conditions, alterations in the circadian rhythms of the humoral immune system have been detected. In patients with allergic asthma, Cricchio et al. (1979) found statistically significant circadian rhythms only in immunoglobulins G (IgG) and M (IgM) with peak concentrations occurring at the same time as in clinically healthy subjects. In patients with allergic rhinitis, circadian rhythms were detected in all three immunoglobulins with a 3-h delay in the peaks of IgG and IgM as compared with healthy subjects (Cricchio et al., 1979) (Figure 20-10).
Surface IgA in the nasal secretions, a primary immune defense in the respiratory tract, shows a circadian rhythm (Hughes et al., 1977) that was found to be phase shifted in shift workers. Although peak and trough of the circadian rhythm in nasal secretory IgA (SIgA) coincide in diurnally active human subjects with those of plasma cortisol, suppression of the daily rise in plasma cortisol by a dexamethasone suppression test does not alter the concentration or the rhythm of SIgA (Menzio et al., 1980).
A circadian rhythm of serum IgE concentrations has been reported by some (Cricchio et al., 1978; Nye et al., 1975) but was not found by others (DeLeonardis and Brandi, 1984). Gaultier et al. (1987) compared the circadian
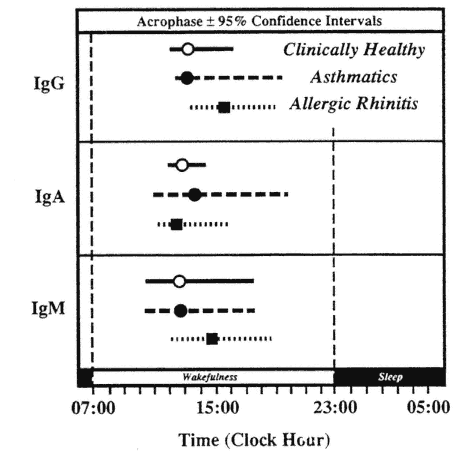
FIGURE 20-10
Circadian acrophase with 95 percent confidence interval of serum immunoglobulins in clinically healthy subjects and patients with allergic asthma or rhinitis.
SOURCE: Adapted from Cricchio et al. (1979).
variations in serum total IgE in clinically healthy children with that in asthmatic patients. No statistically significant circadian rhythm was validated in the healthy children, who showed low levels of IgE. In contrast, the asthmatic children who had substantially higher serum IgE concentrations showed a large-amplitude circadian rhythm with a double peak during daytime (presumably representing a 12-h rhythm) and a nocturnal trough.
Circadian variations in complement components, and in the activation of the alternate pathway in the complement response, were reported (Haus et al., 1983; Hoopes and McCall, 1977; Kim et al., 1980). The timing of the circadian rhythms of properdin was altered during starvation (Kim et al., 1980). In disease states like systemic lupus and rheumatoid arthritis, circulating immune complexes show circadian variations that may be of clinical importance (Isenberg et al., 1981).
Periodicities and/or response patterns that are not circadian are prominent in humoral immune phenomena. Rhythms with about 7-d periods (circaseptan rhythms) or longer (most frequently in multiples of the 7-d cycle, e.g., 14 or 21 days) occur in the function of several components of the immune system, for example, in the formation of antibodies in different experimental systems in a large number of animal species. Circaseptan rhythms in antibody-forming cells or antibody blood levels and in the hemagglutination response of rodents after immunization against sheep red blood cells or allogeneic thymic cells have been reported by Britton and Moller (1968), Grossman et al. (1980), Romball and Weigle (1973, 1976), Stimpfling and Richardson (1967), Weigle (1975), and others (for review, see Levi and Halberg, 1982).
Seasonal variations or circannual rhythms in serum immunoglobulins were reported in clinically healthy subjects with peak levels of IgG, IgA, and IgM occurring during midsummer to midautumn (Lu et al., 1979; Reinberg et al., 1979). In patients with atopic diseases, there were some differences in phase, with slightly earlier values for IgG and IgA but with a substantially different timing for IgM, which showed a peak in February.
Biologic Rhythms in Response to Vaccination
Circadian time dependency of the human response to inoculation with antigens is less well documented. Pöllman and Pöllman (1988) found a statistically significant lower antibody titer in subjects inoculated with hepatitis B antigen during the morning hours (07:00–09:00) as compared with subjects immunized during the afternoon (13:00–15:00). Also the side effects (pain and swelling around the injection site) were less after inoculation in the morning. The question was raised if these differences may be due to differences in the plasma cortisol concentration, which are higher in the morning and which already in physiologic concentrations show recognizable effects upon monocytes and T-lymphocytes.
Feigin et al. (1967a) gave attenuated Venezuelan equine encephalomyelitis (VEE) vaccine at 08:00 or at 20:00 to 40 healthy young men (20 at each time). A significant serologic response verifying infection was demonstrated in each subject. The number of subjects immunized at 08:00. who became ill was not statistically different from those immunized at 20:00, and there was no difference in the duration or severity of illness between the two groups (Alevizatos et al., 1967). However, the number of virus particles present in white blood cells reached its peak on day 2 after immunization at 08:00 and was delayed until day 6 in the subjects inoculated at 20:00.
There was a marked alteration in the circadian rhythm of whole blood amino acids (Feigin et al., 1967a, b) in all subjects sampled irrespective of time of inoculation. However, the rhythm alteration was apparent during days 1 through 4 in the group immunized at 08:00 and during days 2 through 8 in the group immunized at 20:00. Thus, both the disappearance of the virus particles from the circulating white blood cells and the disturbance of amino acid circadian periodicity occurred later after inoculation at 20:00 as compared with the inoculation at 8:00 A.M. These observations suggest that the effect of an infectious microorganism on the human host may depend to some degree on the time of exposure (Feigin et al., 1967a).
Langlois et al. (1995) reported data from two field trials of influenza vaccine (one conducted during summer, the other during fall). The vaccine given in both studies contained three antigens (A/Chile/83, A/Philippines 82, and B/USSR/83). The vaccine was administered to over 800 different patients between 08:30 and 17:00. Injection time-dependent differences in antibody response were detected in first-time inoculated subjects only in one of these studies and only for the A/Philippines antigen. The antibody response to this antigen was considerably larger than the response to the two others, which may explain these differences. However, the difference between the two studies could also be due to seasonal variations in the antibody response. Seasonal variations in the immune system have been reported in human subjects (Levi et al., 1988b; Shifrine et al., 1982a) and in experimental animals (Fernandes, 1994; Shifrine et al., 1982b).
The systemic reactions noted after first vaccination in these studies showed no circadian variation in occurrence or severity. In contrast, the local side effects after revaccination showed a statistically significant circadian variation even after correction for all known potentially confounding factors including age. The local reactions at the injection site (redness, hardness, and soreness) were most pronounced when the immunization took place around midafternoon. This finding was consistent in both studies and is in agreement with the observations by Pöllman and Pöllman (1988) using a different antigen (HB vaccine).
Effects of immunization on the circadian time structure of the immunized subjects were also observed. Westaway et al. (1995) studied the urinary excretion of cortisol in infants before and after immunization against diphtheria;
tetanus; pertussis; and haemophilus influenzae, type B. The immunization produced a significant increase of rectal temperature during the following night in all infants. Body temperature failed to fall during the night, although febrile levels were rarely obtained. Infants without an adult-like circadian body temperature pattern showed a significant increase in urinary cortisol excretion during the night and the morning after immunization. After an adult-like body temperature pattern had developed, immunization no longer significantly raised urinary cortisol output.
No such correlation was observed between the extent of temperature disturbance and the excretion of cortisol after the second immunization. These observations suggest significant developmental changes in the cortisol response to immunization. The change in the hypothalamic-pituitary-adrenal (HPA) axis from responding to not responding to the vaccines appeared to be more strongly related to the development of an adult-like body temperature pattern than to age. Since adult-like body temperature patterns develop at considerably different ages in different babies, it appears that some infants will remain in an infantile stage of their immunological development as far as cortisol excretion is concerned for much longer than others. The developing infant seems to undergo a series of changes that may radically affect the way in which it copes with infection. Individual differences in this developmental process may underlie differences in vulnerability among infants.
Data thus far available on the response of human subjects to vaccination support the following conclusions:
- Differences in immune response as a function of the circadian time of the introduction of antigen may occur as indicated by differences in antibody titer in clinically healthy subjects. These differences may be limited to certain antigens and/or to a certain degree of immune response and may also depend on other factors (such as seasonal variations), which at this time have not been systematically studied.
- Higher antibody titers can be achieved by vaccinating during midday or afternoon. However, the circadian differences described are quantitative in nature and all subjects studied achieved some degree of immunity irrespective of the time of vaccination.
- The circadian rhythms in antibody response described conceivably will be of some importance for the success of vaccinations, especially in subjects with poor antibody formation (Fletscher, 1993).
- Vaccination leads to a disturbance of the circadian rhythms of clinical (e.g., body temperature), endocrine (cortisol), and metabolic (e.g., blood amino acids) variables, which differ as a function of the time of inoculation and, in infants, as a function of the maturity of the circadian time structure.
- The most consistent findings of rhythmicity of the effects of antigen exposure in human subjects are the more pronounced local side effects after
- antigen inoculation at noon and during the afternoon in a previously sensitized individual.
- Vaccination response patterns other than those with circadian rhythmicity (e.g., circaseptan or circannual) have thus far not been explored.
Chronobiology in Cell-Mediated Immunity, Including Allograft Rejection
The development of cell-mediated immunity and the response to challenge, including the elimination of immunologically incompatible cells as seen in allograft rejection, is a complex process involving macrophages, cytotoxic T-cells, NK cells, cytokines, and other factors. Several of these factors are circadian periodic. In addition, many cell-mediated immune phenomena (e.g., rejection of tissue allografts) also show infradian (mostly circaseptan) response patterns.
The development of cell-mediated immunity and the response to reintroduced or persisting antigen are modified both by the time when the antigen is first encountered and by the time of challenge. In oxazolonesensitized, light-dark synchronized rats, the ear swelling after challenge with this agent showed a large-amplitude circadian rhythmicity. The mean response to challenge during the daily rest span of the animals was more than 8 times that seen in rats challenged at the time of onset of the daily activity (Knapp et al., 1981). A reversed light-dark cycle did reverse the timing of the peak and trough of the response to antigen challenge (Pownall et al., 1979).
Clinically healthy, diurnally active human subjects testing positive for PPD (due largely to BCG3 vaccination) showed, when injected intradermally with PPD during different circadian stages, a circadian variation in response with a maximum at 07:00 and a minimum at 22:00. The asymmetric biphasic pattern in this study suggests a superimposed ultradian rhythmicity (Cove-Smith et al., 1978).
In allograft rejection, although the immune-incompatible cells remain inside the host organism during development of the immune response, the time of the first encounter with the antigen modifies the intensity of the ensuing immune reaction. For example, the rejection of allogeneic skin in mice and of kidney grafts in rats was delayed significantly by grafting during the early dark span, the activity period of the animals that corresponds to the time of the circadian peak in serum corticosterone (Halberg et al., 1974; Ratté et al., 1973).
The more marked local response to PPD administered to sensitized human subjects during the early morning hours and the more frequent manifestation of the signs of renal allograft rejection at that time (Knapp et al., 1981) (the peak in both variables found to be around 07:00) suggests that the cell-mediated immune response is more vigorous during the late night and early morning
hours. This rhythm may be of interest for treatment with immunosuppressive agents that are given in current medical practice without chronotherapeutic considerations mainly during daytime. Sinusoidal infusions of cyclosporin with the maximal dose of the drug given at certain times of the day showed in dogs carrying kidney allografts (in comparison with a treatment with equal doses given throughout the 24-h span) a statistically significant circadian rhythm in response. Similarly, cyclosporin chronotherapy of pancreas-allotransplanted rats revealed circadian stage dependence of the dosing time to obtain an optimal gain in graft function (Liu et al., 1986).
Transplant studies in human subjects have shown, in addition to the circadian rhythm in the onset of the functional manifestations of renal transplant rejection, a circaseptan rhythm in the rejection of kidney allografts (DeVecchi et al., 1981). In 157 patients with kidney transplants, rejection episodes were clinically diagnosed when one of the biochemical variables of primary interest as a criterion of rejection (i.e., blood creatinine, blood urea, proteinuria) began to change. The occurrence of rejection episodes during a 2-mo observation after transplantation followed an infradian rhythm with a period longer than precisely 7 days (8.1 days). The fact that the period found in these patients is slightly but consistently different from the 7 days of the social week suggests that this period is endogenous in origin. The apparent 8.1-d periodicity was also different from the changes in corticosteroid regimen in those patients, which occurred at precisely 7-d intervals. Similar findings in human studies as well as in animal experiments have been reported also for heart and pancreas allografts (Liu et al., 1986).
The circaseptan rhythm in allograft rejection is of clinical interest since it appears to provide an opportunity to optimize antirejection therapy, both along the circaseptan and the circadian time scales. The timing of the best antirejection treatment schedule in diurnally active human subjects, with the maximal dose given during the dark span, is of interest in view of a similar timing found for the optimal cyclosporine effects in nocturnal rats bearing heart or pancreas allografts (Liu et al., 1986). A similarity of timing of duration of circadian rhythms in diurnally active humans and nocturnal rodents is found also in the circadian rhythm of melatonin and of some red cell parameters in the peripheral blood (Haus et al., 1983).
Time-Dependent Changes in Cutaneous Allergy and Allergic Asthma
The cutaneous response to histamine and to the antigens of ragweed, grass pollen antigen, and house dust, varies in a circadian rhythmic fashion. The greatest sensitivity is found 3 to 6 hours prior to the middle of the patient's sleep span, which in diurnally active human subjects usually corresponds to the hours between 18:00 and 23:00. The area of a reaction from a skin test carried out during this time is on the average about 2 to 3 times larger than that found for an
identical test on the same patient during the time of low reactivity (between 07:00 and 11:00) (Lee et al., 1977; Reinberg, 1967; Reinberg et al., 1965). In the application to patients with allergic diseases, testing with antigens or substances like histamine or acetylcholine early in the day may result in false negatives. In repeated testing of allergic patients, it is important to apply the antigen or other stimuli during the same circadian stage of cutaneous or bronchial reactivity. Only standardization of the time of testing in relation to the activity-rest pattern of the patient will yield reproducible results that are comparable from one test to the other (Reinberg and Sidi, 1966).
In bronchial asthma, the circadian times of the peak and trough of the hyperreactivity of the bronchial tree to histamine (DeVries et al., 1962) are almost identical with those found for the circadian rhythm in cutaneous reactivity to intradermal injections with histamine or skin allergens (Lee et al., 1977; Reinberg, 1967; Reinberg et al., 1963, 1965). A similar circadian rhythm was found in the hyperactivity of the bronchial response to the inhalation of acetylcholine (Reinberg et al., 1971). The time when asthmatics are exposed to a specific triggering agent appears to be almost as important as the quantitative aspects of the exposure. Asthmatic attacks, including deaths from severe asthmatic attacks, occur predominantly during the night hours primarily between 02:00 and 07:00, with peak incidence between 04:00 and 05:00 (Benatar, 1986; Dethlefsen and Repges, 1985; Hetzel et al., 1977; Turner-Warwick, 1988).
It appears that these rhythms are predominantly an expression of host sensitivity and are determined by endogenous circadian bioperiodicities in relationship to changes in the external environment during the 24-h day (Barnes, 1984a, b; Smolensky et al., 1981, 1986a, b). The times of greatest susceptibility to asthmatic attacks and the highest cutaneous sensitivity to antigen coincide with the trough of the circadian rhythm in the excretion of adrenocorticosteroids (Reinberg et al., 1963) and also coincide with the trough of the circadian rhythm in plasma catecholamines. Assumption of a causal relationship between the manifestations of cutaneous and bronchial allergy and the circadian rhythms in cortisol and catecholamines appears tempting. Although the circadian rhythms in plasma cortisol and catecholamines do not seem to be the only factor responsible for the nocturnal exacerbation of the chronic inflammatory processes, it could conceivably contribute to the day-night difference in sensitivity and thus be related to the risk and the frequency of asthma attacks. In addition to the inflammatory component of the disease, the bronchial tone is also strongly regulated by adrenergic mechanisms.
The circadian rhythm in serum IgE may also be related to the periodicity of allergic manifestations. Gaultier et al. (1987) found a fivefold greater mean concentration of IgE in asthmatics than in clinically healthy children, with a high-amplitude circadian rhythm equal to 30 percent of the 24-h mean in asthmatics, which was absent in the healthy subjects (Figure 20-11). In
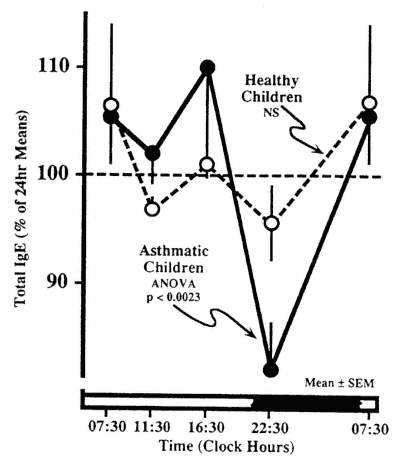
FIGURE 20-11
Circadian rhythm in serum immunoglobulin E (IgE) in children with allergic asthma is not evident in clinically healthy subjects. SOURCE Adapted from Gaultier et al. (1987).
asthmatics, the highest serum IgE levels occur around midday, and the lowest levels occur at night (Kunkel and Jusuf, 1984).
Successful management of asthmatic patients requires an understanding of the circadian features of the disease to achieve an effective chronotherapy for antiasthmatic medications (Reinberg, 1989; Reinberg et al., 1988a,b; Smolensky and D'Alonzo, 1994, 1997; Smolensky et al., 1986b, 1987).
The Rhythms of Sleep and Immunity
The alternation of sleep-wakefulness is a fundamental part of the human circadian time structure and coordinates numerous other neuroendocrine variables. Several immunologically active hormones and peptides influence the sleep-wake cycle of the brain and are involved in a bidirectional or multidirectional communication among neuroendocrine, immune, and CNS functions (Table 20-2). Most of them show circadian rhythmicity, and some are influenced by environmental factors acting as synchronizers or as masking agents. IL-1 and other cytokines play a regulatory role on the sleep-wake cycle (Opp et al., 1992) and interact in this process with various immunologically active neuroendocrine substances (Krueger, 1990). The physiologic regulation
TABLE 20–2 Immunologically Active Variables Affecting Sleep and/or Wakefulness
Promoting Sleep |
Reference |
|
Stimulating IL-1 and Sleep |
||
Insulin |
Krueger, 1990 |
|
Growth hormone |
Krueger, 1990 |
|
Growth hormone releasing factor |
Krueger, 1990 |
|
Melatonin |
Krueger, 1990 |
|
Somatostatin |
Krueger, 1990 |
|
Immunologically Active Peptides |
||
Interleukin-1 (IL-1) |
Krueger et al., 1983, 1984; Tobler et al., 1984 |
|
Interleukin-2 (IL-2) |
De Sarro et al., 1990 |
|
Interferon-α |
De Sarro et al., 1990 |
|
Tumor necrosis factor |
Shoham et al., 1987 |
|
Factor S |
Krueger et al., 1982 |
|
Muramyl peptides |
Krueger et al., 1984; Masek and Kadlec, 1983; Pappenheimer, 1983 |
|
Vasoactive intestinal peptide |
Jouvet, 1984; O'Dorisio et al., 1984 |
|
Delta sleep-inducing peptide |
Graf and Kastin, 1984; Yehuda et al., 1987 |
|
Promoting Wakefulness |
Reference |
|
Inhibiting IL-1 and Sleep |
||
Corticotropin-releasing factor |
Krueger, 1990 |
|
ACTH |
Krueger, 1990 |
|
α-MSH |
Krueger, 1990 |
|
Glucocorticoids |
Krueger, 1990 |
of the sleep-wakefulness rhythm requires a specific constellation of multiple natural sleep-promoting or wakefulness-promoting substances which are, in part, determined by their circadian rhythms and in part by environmental and behavioral conditions (Moldofsky, 1994). Periods of predisposition to sleepiness are separated by periods of resistance to sleep (Lavie and Weller, 1989).
Numerous immunologic variables are influenced by the sleep-wakefulness pattern and may be altered at times of environmentally enforced sleep irregularities and especially by sleep deprivation. For example, NK cell activity is related to sleep and declines during nocturnal (or other) sleep periods (Shahal et al., 1992). Sleep deprivation in experimental animals and in human subjects leads to an impairment of immune functions, which if prolonged, will lead to the death of the animals (Everson, 1993) and to the fatal familial insomnia syndrome of human subjects (Portaluppi et al., 1994). However, even much shorter periods of sleep deprivation lead to rhythm alterations and to marked impairment of immune function. In human subjects, Moldofsky et al. (1989) found during a single night of sleep deprivation increased plasma IL-1 and IL-2 concentrations with an alteration in the PMW response of B-lymphocytes and a depression or absence of the nocturnal decline in NK cell activity. The response to PHA remained unchanged. These changes appeared to be unrelated to the circadian rhythm in plasma cortisol. After 48 hours of sleep deprivation, Palmblad et al. (1979) also found a reduced PHA mitogen response. After 72 hours of sleep deprivation, there was an increase in plasma cortisol, an increase in interferon production by lymphocytes, and reduced phagocytosis by polymorphonuclear leukocytes (Palmblad et al., 1976).
The effect of sleep deprivation on the ability to respond to the introduction of an antigen has been studied in mice. Mice immunized against influenza virus were challenged, followed by a 7-h span of sleep deprivation. Whereas normally sleeping, immunized mice were able to clear the virus from the respiratory tract within 3 days of challenge, in immunized, sleep-deprived animals, the extent of viral recovery was almost the same as in nonimmunized animals (Figure 20-12) (Brown et al., 1989; Husband, 1993).
Phase shifts, as they are encountered in shift workers or after transmeridian flights, or rhythm disturbances in people under grossly irregular work schedules lead to internal desynchronization of immune-related circadian rhythms and to impairment of immune functions. In rats, weekly inversion of a light:dark (LD) 12:12 lighting regimen led after 2 months to a decreased cellular immune response as measured by mitogen stimulation with concanavalin A (ConA) and by a popliteal lymph node assay (Kort and Weijma, 1982). A rather severe immune impairment was found after 33 weeks of weekly phase shifting. Spot checks of adrenal function in these animals, measured in vitro and in vivo at a single time point only, did not reveal substantial changes. The latter result has to be qualified since single time point sampling does not allow an evaluation of the adrenal cycle. In studies of shift workers, Nakano et al. (1982) reported lower proliferative responses in lymphocytes as compared with regular daytime
workers. Since shift work is often accompanied by a certain degree of sleep deprivation, it is unclear whether the impaired immune function in shift workers is a consequence of circadian desynchronization, sleep deprivation, or both.
Changes in Immune System-Related Variables During the Menstrual Cycle
Gonadal steroids contribute to the regulation of immune function (Da Silva and Hall, 1992; H. S. Fox, 1995b; Hasty et al., 1994; Mortin and Courchay, 1994; Schuurs and Verheul, 1990). Receptors for steroid hormones are present in circulating lymphocytes (Bellido et al., 1993; Daniel et al., 1983; Stimson,
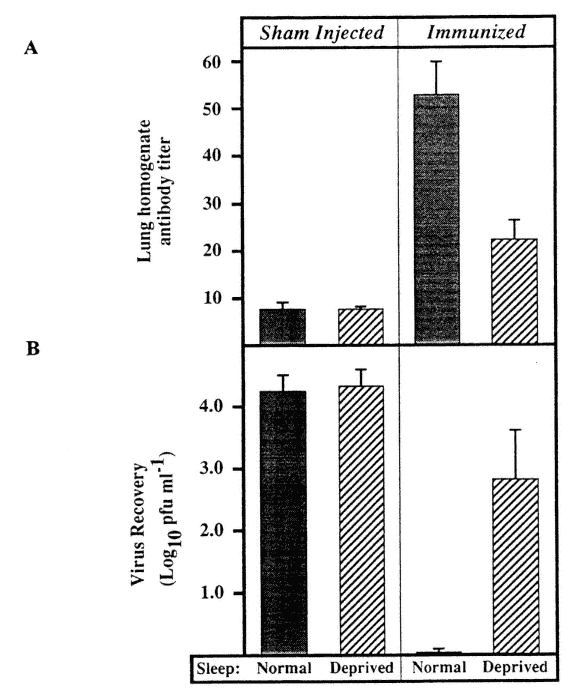
FIGURE 20-12
Effect of sleep deprivation on ability to respond to challenge with antigen (influenza virus) in immunized mice. Challenge followed by 7 hours of sleep deprivation. Sleep deprivation leads to impairment of antibody response (A) and of elimination of antigen (B). SOURCE: Adapted from Brown et al. (1989).
1988). No or only minor changes in the number of circulating blood cells were reported during the menstrual cycle (Eichler and Keiling, 1988; Fox et al., 1991). In a comparison between day 6 and day 22 of the menstrual cycle, there were no consistent changes in the proportion of lymphocyte subtypes, nor were there any significant changes in the absolute numbers or in the circadian rhythmicity in total white blood cell count, granulocytes, lymphocytes, T- and B-cells, T-helper and suppressor cells, and NK cells (Dixon-Northern et al., 1994). The only apparent difference as a function of the day of the menstrual cycle was found in the circadian periodicity of monocytes, for which the trough was found at 12 noon for day 6 and at midnight for day 22 (Dixon-Northern et al., 1994). The limited sampling and the small number of subjects in these studies do not allow the drawing of final conclusions.
In contrast, the plasma IL-1 activity (Cannon and Dinarello, 1985) is consistently increased after ovulation in the luteal phase of the menstrual cycle simultaneously with the elevation in body temperature. In view of the thermogenic effect of IL-1, this may be related and may also be consistent with the observation that progesterone and estrogen increase the macrophage production of IL-1 (Olsen and Kovacs, 1996).
Related to differences in the human immune response during different stages of the menstrual cycle may be the clinical observation of a greatly reduced overall and recurrence-free survival in women with breast cancer who were operated on during days 3 to 12 after onset of menstruation as compared with women operated on between days 0 through 2 or days 13 through 32 (Badwe et al., 1991; Hrushesky and März, 1994; Senie et al., 1991).
Seasonal Changes and/or Circannual Variations in Immune Response
Changes of immune-related variables during different seasons may be caused by climatic differences, including day length, temperature, and related factors such as exposure to allergens and microorganisms, but they may also be the consequence of endogenous circannual rhythms that may or may not be synchronized by environmental (climatic and other) stimuli (Haus and Touitou, 1994b). Circannual rhythms have been demonstrated in animals kept for their entire life, and in some instances for generations, in environments with a standardized lighting regimen and constant temperature (Figure 20-12) (Brock, 1983, 1991; Haus et al., 1974a, 1984, 1997; Haus M. et al., 1984), and free-running circannual rhythms have been described in human subjects (Haus and Touitou, 1994a). The seasonal rhythmicity in lymphocyte blastogenic response persists in young mice kept in a constant environment (Brock, 1983). Mortality in the young animals varied in a circannual fashion, with the peak occurring when the proliferation rates of both T- and B-cells were low (Brock, 1987). In contrast in old animals, the amplitude of the circannual rhythm of T- and B-cells
was markedly decreased, and mortality was consistently higher throughout the year (Brock, 1987, 1991).
Levi et al. (1988b) found seasonal modulation of the circadian rhythms of circulating T- and NK-lymphocyte subsets in healthy volunteers. These studies suggested a rhythm with a period of about 6 months for circulating T-helper cells. There appeared to be circadian desynchronization of circulating lymphocytes during spring, which corresponded in its timing to the peak incidence of viral infections and of Hodgkin's disease (Levi et al., 1988b). Pati et al. (1987) described in mice a 6-mo rhythm in the proliferative mitogen response of splenic B-lymphocytes and a circannual rhythm for NK cell activity. Shifrine et al. (1980, 1982a, b) found seasonal variations in cell-mediated immunity in dogs and in humans. Studying the whole-blood, lectin-induced, lymphocyte mitogen response, they observed a peak in summer and poor response to mitogens in winter.
A circannual variation of rubella antibody titer was noted in a large number of patients who were followed for 7 years (Rosenblatt et al., 1982). In Finland, a country with marked climatic differences between the seasons, Katila et al. (1993) found a seasonal variation in the interferon-producing capacity of leukocytes from clinically healthy subjects, with low values during the summer months. In contrast, no seasonal differences in this variable were found in Japan (Kishida et al., 1987), a country with lesser seasonal climatic changes.
Deficiency in erythrocyte adenosine aminohydrolase activity has been reported in patients with severe combined immunodeficiency disease (Giblett et al., 1972), and alterations in the activity of this enzyme have been associated with immunologic loads (Tritsch et al., 1975) and with neoplasia (Zimmer et al., 1975). In a study extending over 2 years, Nechaev et al. (1977) showed a circannual variation of erythrocyte adenosine aminohydrolase in healthy subjects and in patients with a variety of neoplastic diseases of various stages and treated with a variety of chemotherapeutic agents. In healthy subjects and in patients with cancer, the circannual variation resulted in relatively low enzyme levels between April and June and higher levels between October and February.
Seasonal modifications in immune response must be considered in clinical trials and in the planning of immunotherapeutic treatment strategies for various diseases.
Modification of Immune-Related Rhythms by Exercise
Physical exercise alters the circadian periodicity of several components of the immune system and of related neuroendocrine variables (Shephard et al., 1995; Winget et al., 1994). With moderate exercise, these changes are short in duration and may only transiently mask the circadian rhythm of, for example, catecholamines, the numbers of polymorphonuclear leukocytes, and lymphocytes. Physical performance and performance tolerance vary
rhythmically. Maximal performance (e.g., in grip strength or diverse sport activities) is reached in diurnally active human subjects during the late afternoon (Lundeen et al., 1990; Winget et al., 1994).
Exercise induces changes in the T-cell number, in the relation of CD4+ cells to CD8+ cells, the sensitivity of T-cell β-adrenoceptors, NK cell activity, interleukin production, and cell proliferation. While moderate physical exercise and training may improve certain immune functions, severe and exhaustive exercise is immunosuppressive (for review, see Shephard et al., 1995). Severe and exhaustive exercise can also mark many of the neuroendocrine rhythms that are instrumental in regulating the rhythmicity and, to some degree, the integrity of the immune system (Opstad, 1991, 1992). However, no investigations of the effects of circadian and other periodicities in performance on the response of the immune system to exercise have been found in the available literature. Investigations of the periodicity in exercise effects (acute strenuous as well as endurance) on the neuroendocrine-immune system network might be of considerable interest for military and sports medicine.
Modification of Immune-Related Rhythms by Time of Food Uptake
The activity-rest and sleep-wakefulness pattern is not the only synchronizer acting on the mammalian circadian system. If a large proportion of the daily caloric intake is taken as one major meal at a certain circadian phase, the timing of food uptake may mask the circadian rhythms. If such intake persists for a prolonged time, it may become the dominant synchronizer of some circadian periodic functions. In animal studies, many circadian rhythms related directly or indirectly to immune functions can be adjusted in their timing by time-restricted meal feeding at certain circadian stages (Halberg and Stephens, 1959; Lakatua et al., 1983; Nelson et al., 1975; Scheving et al., 1976). Other rhythms, however, will remain synchronized to the light-dark regimen in experimental animals or to the activity-rest pattern in humans. A number of endocrine and hematologic rhythms may show, under the influence of the ''competing'' synchronizers, some changes in their usual timing without the apparent predominance of either synchronizer.
In human subjects, time adaptation of rhythms by timed food uptake is much less pronounced and can be achieved only by exposure to rigorous feeding schedules that would be uncommon in everyday life. Clinically healthy, young adult volunteers following a diurnal activity pattern with sleep during the night (typically between 23:00 and 06:30) who received all their calories in the form of a single meal as breakfast or as dinner were studied over a 24-h span at the end of 3 weeks on that regimen. The circadian rhythms of plasma insulin, serum iron, and blood urea nitrogen concentration had adapted their timing to the time of food uptake, irrespective of the activity pattern of the subjects. In contrast, the timing of the circadian rhythms in growth hormone (GH), plasma
cortisol, serum chlorides, and total leukocyte count had shifted only slightly, while the rhythm in circulating lymphocytes was not statistically significantly different between the (same) subjects studied on both dietary regimens and on a three-meal eating schedule (Halberg et al., 1995; Haus et al., 1984, 1988). In a shorter study in which all calories were given either as breakfast or as dinner, Sensi et al. (1984) found after only 3 days, marked phase shifts in the circadian rhythms of respiratory quotient and variables related to carbohydrate metabolism and lipid oxidation. In urinary catecholamines, the evening mealonly schedule delayed the circadian peak for over 5 hours. Cortisol did not seem to be influenced by the short period of meal timing, and sodium and potassium excretion did not show consistent differences between the two diet regimens. After 18 days on the altered schedule of calorie uptake, plasma cortisol and thyroid-stimulating hormone (TSH) circadian rhythms were similar, as seen with a three-meal diet. The circadian rhythm in PRL was detectable when the single daily meal was given at 18:00 but disappeared when the meal was given at 10:00.
From these and other studies in human subjects (Goetz et al., 1976; Sensi et al., 1984) and in animals (Lakatua et al., 1983; Scheving et al., 1976), it appears that for the circadian rhythms related to digestive and metabolic functions, including those of the endocrine and exocrine pancreas and of the gastrointestinal tract epithelia, the time of food uptake acts as the dominant synchronizer. Also the circadian rhythms of many liver enzymes are synchronized primarily by the time of food uptake (Fuller and Snoddy, 1968). In human subjects, some of the most stable rhythms (such as plasma cortisol and circulating lymphocytes), which directly relate to the regulation of immune functions, are apparently synchronized predominantly by the activity-rest schedule and the related light-dark regimen. However, with prolonged exposure to unusual feeding schedules, the changes observed in GH and insulin may well lead to changes in the timing of certain immune-related variables, although experimental evidence for such a relationship is thus far lacking.
It appears that the phase adaptation of a number of circadian rhythms of physiologic importance in shift workers can be reinforced, delayed, or even prevented by the timing of food uptake in relation to the activity-rest pattern. Internal desynchronization brought about by the independent alteration of two competing synchronizers may lead to functional or even organic disturbances, as has been suggested by a variety of problems, including the appearance of gastric ulcers in shift workers exposed to physiologically unacceptable alterations of their activity-rest pattern and the time of food uptake (Carandente and Halberg, 1976; Gibinski et al., 1979; Tarquini, 1980).
Biologic Rhythmicity in Neuroendocrine-Immune Interactions
The rhythmicity observed in the immune system is in part regulated by the multifrequency neuroendocrine rhythms that characterize the mammalian time structure and in some frequencies are environmentally synchronized (for reviews, see, e.g., Touitou and Haus, 1994; Van Cauter and Turek, 1995). Interactions between the endocrine and the immune system are found at every level from the hypothalamic nuclei to the receptors in the cells of the target organs (for reviews, see Auernhammer and Strasburger, 1995; Besedovsky and Del Rey, 1996; Blalock, 1992; Daynes et al., 1995; Fabris et al., 1995; Maestroni, 1993; Murphy et al., 1995; Olsen and Kovacs, 1996; Weigent and Blalock, 1995; Wilder, 1995).
Neuroendocrine hormones that act as immune modulators include CRF, ACTH, cortisol, dehydroepiandrosterone (DHEA), and DHEA-sulfate (-S), estrogen, progesterone, testosterone, melatonin, PRL, GH, vasopressin, β-endorphin, and other proopiomelanocortin (POMC) peptides, thyrotropin-releasing hormone (TRH), and TSH (Blalock, 1989, 1992; Weigent and Blalock, 1995).
Immune competent cells, including both T- and B-lymphocytes, carry high-affinity receptors for the POMC peptides including ACTH (Clarke and Bost, 1989) and opioid peptides (Carr, 1991). The same classes of receptors as found in neural tissue have been identified on cells of the immune system (Carr, 1991). ACTH and endorphins inhibit antibody production (Johnson et al., 1982) and modulate many aspects of immune cell function (Carr, 1992; Johnson et al., 1982, 1992).
In turn, mouse and human lymphocytes produce POMC-derived peptides, including ACTH, which are biologically active (Blalock, 1992, 1994), as shown by the rise in corticosterone found in hypophysectomized animals exposed to infectious agents (Bayle et al., 1991; Smith et al., 1982). This effect was abolished in chicken if B-lymphocytes were deleted by bursectomy (Bayle et al., 1991). Although the lymphocyte-produced hormones usually do not reach concentrations in the circulation that allow their measurement, their local production in an auto- or paracrine manner by cells of the immune system at the site of an immune process or inflammation can lead to immunomodulation (Johnson et al., 1992). At the present time, no information is available on the periodicity of hormone production by activated cells of the immune system, although some of these cells seem to respond to humoral neuroendocrine controls like CRF or ACTH.
Glucocorticoids exert their powerful anti-inflammatory actions in part by downregulating the transcription of inflammatory mediators (Russo, 1992), including the cytokines IL-1 (Kern et al., 1988), IL-2 (Kelso and Munck, 1984), IL-3 (Homo-Delarche et al., 1991), IL-6 (Besedovsky and Del Rey, 1996), IL-8 (Mukaida et al., 1993), GM-CSF, TNF-α (Homo-Delarche et al., 1991), and
INF-γ (Kelso and Munck, 1984). The large-amplitude circadian rhythm in plasma cortisol concentration in humans with peak values in the early morning hours in diurnally active subjects has been related to changes in immune response, with the minimum in response corresponding to the peak in cortisol concentrations (Lee et al., 1997; Reinberg et al., 1963). This same association has been observed for the circadian rhythm in plasma corticosterone in nocturnal rodents, which exhibit peak corticosterone values at the end of the daily light span and the beginning of the dark span.
The anti-inflammatory action of cortisol is balanced at any one time by the immunostimulatory hormones, including GH, PRL, and melatonin (Hooghe et al., 1993; Maestroni et al., 1986), which all peak during the night hours. The circadian rhythmicity in immune response appears to be, to a large extent, the net result of the balance at any one time between the immunosuppressive effect of glucocorticoids and the effect of immunostimulant hormones modulated by cytokine feedback and peripheral hormone production by cells of the immune system (Weigent and Blalock, 1995).
Immunomodulation also occurs at the target tissue level with selectivity of hormone effects and temporal variations due to the activity of specific enzyme systems metabolizing and transforming hormones at the level of the target cells. This seems to be the case for the formation of DHEA from its prehormone DHEA-S in lymphoid tissues (Daynes et al., 1995). DHEA modulates the immune response in lymphoid tissue after antigen exposure (Daynes et al., 1995). Its decreased production (e.g., with aging or the decreased liberation from its prehormone DHEA-S) leads to immunosenescence, which can be overcome by the administration of DHEA. The poor response of immunosenescent elderly subjects to vaccination (Schwab et al., 1989) is thought to be a consequence of the decrease in production of DHEA (Daynes et al., 1995). When aged animals were given oral supplementation with DHEA-S, the usual mature adult pattern of inducible T-cell lymphokines was restored, and a solid humoral immune response was obtained (Daynes and Araneo, 1992; Garg and Bondada, 1993). Similarly, the direct incorporation of DHEA or DHEA-S into a protein vaccine formulation was found to promote the development of a strong immune response in age-compromised hosts (Araneo et al., 1993). There is a high-amplitude circadian rhythm of DHEA, which in its timing is comparable to that of cortisol (Haus et al., 1995). In contrast, DHEA-S in humans characteristically shows a more flat circadian variation, with a peak later during the day (Haus et al., 1988, 1995). DHEA-S also shows a circannual rhythm, which in its amplitude may be equal to or higher than that of the circadian rhythm (Haus et al., 1988; Nicolau et al., 1984). No data are available relating these rhythmic variations in adrenal androgens to circadian or circannual changes in the immune system.
The serum PRL concentration shows a large-amplitude circadian rhythm, with peak values during the night hours (Haus et al., 1980). This rhythm is modulated by a marked seasonal variation found in women but not in men
(Haus et al., 1980, 1988; Touitou et al., 1983). Also ethnic-geographic differences in the circadian and circannual amplitudes of PRL concentrations have been reported (Haus et al., 1980). The circadian variation in plasma PRL concentrations is modulated by sleep but to a lesser degree than that of growth hormone (hGH).
PRL is a major immunoregulating hormone. CD4+ and CD8+ cells and macrophages express PRL receptors (Gala and Shevach, 1993). Nuclear PRL receptors play an important role in IL-2 induced T-cell proliferation in vitro (Clevenger et al., 1990), which can be inhibited by PRL antibodies (Hartmann et al., 1989). Lymphocytes and macrophages also seem to produce PRL or a "PRL-like" factor (Gala and Shevach, 1994), and PRL has been suggested as a growth factor for lymphoid cell proliferation (Russell et al., 1988).
In animal experiments, hypophysectomy or suppression of PRL secretion by the dihydroxyphenylalanine (DOPA) agonist bromocryptine leads to a state of immunodeficiency with decreased resistance to infection, which can be reversed by treatment with PRL (Bernton et al., 1988; Nagy et al., 1983). Cyclosporine, an immunosuppressive fungal peptide, inhibits PRL binding to T-lymphocytes (Hiestand et al., 1986), competing in a dose-dependent fashion for receptor sites. Stimulation of PRL secretion can reverse the immunosuppression induced by cyclosporine (Hiestand et al., 1986), suggesting that the immunosuppressive effect of cyclosporine, which in its extent is circadian, circaseptan, and circannual periodic, may be mediated by the displacement of PRL from binding sites on lymphocytes (Liu et al., 1986).
The unbalanced prevalence of proinflammatory hormones like PRL and GH over the anti-inflammatory regulators like the hormones of the HPA axis is thought to favor the development of chronic inflammatory and autoimmune diseases like rheumatoid arthritis (Berczi et al., 1993). Improvement of autoimmune uveitis occurred in patients treated (for unrelated conditions) with bromocryptine (Hedner and Bynke, 1985), an observation that seemed to be supported by observations in an animal model (Palestine et al., 1987). The relapse in patients with rheumatoid arthritis often seen after childbirth may be related to the high serum PRL concentrations encountered at this time (Wilder, 1995). Events that affect PRL secretion, like chronic stress and sleep deprivation as experienced in military situations, and also the administration of many pharmacotherapeutic agents that are known to alter the secretion of PRL (e.g., phenothiazines, opiates, neuroleptics, L-DOPA, and bromocryptine) may alter immunologic function and show undesirable and sometimes unexpected side effects.
GH shows a marked circadian rhythm that is largely but not exclusively related to sleep, with a major spike in hormone secretion after sleep onset and a decrease in peak height reported during aging. Sleep deprivation and irregular sleep-wakefulness patterns alter this rhythm through the "masking" effect of sleep. Receptors for GH have been found on immunocompetent cells, many of which also show receptors for growth hormone-releasing hormone (GHRH) (for
review, see Auernhammer and Strasburger, 1995); GHRH in low (but not in high) concentrations enhances PHA-stimulated lymphocyte proliferation, IL-2 production in human peripheral blood mononuclear cells (PBMC) (Valtora et al., 1991), and antigen responses (Mercola et al., 1981). Conversely, in vitro stimulation of human PBMC by the T-cell mitogens PHA and ConA or by IL-2 produces a marked rise in GH secreted by PBMC (Hattori et al., 1990; Varma et al., 1993), which suggests bidirectional interactions within a somatotropic immune axis (Walker, 1994). Many of the effects of GH on the immune system are not direct, but they may be mediated through insulin-like growth factor (IGF)-I or may be due to high-affinity binding of GH to the PRL receptor. IGF-I shows a low-amplitude circadian rhythm (Haus et al., 1988) and exerts proliferative effects both on T-cells (Clark et al., 1993) and on B-cell progenitors (Gibson et al., 1993; Landreth et al., 1992). Both GH and IGF-I induce immunoglobulin production and proliferation of human plasma cell lines (Kimata and Yoshida, 1994). The local production of hGH and IGF-I, with the autocrine and paracrine effects of these factors on the immune system, may be at least as important as the circulating hormone concentrations.
Melatonin acts as messenger providing information on environmental light and darkness and shows a high-amplitude circadian variation that depends to a large extent on the presence and/or absence of photic stimulation. Melatonin represents an important endogenous immunomodulating agent (Maestroni, 1993) that has been shown to amplify the immune effects of IL-2 and reduce its toxicity (Lissoni et al., 1994) and to modulate TNF toxicity and biologic activity (for review, see Kopp et al., 1994). Melatonin activates human monocytes and induces their cytotoxic properties along with IL-1 secretion (Morrey et al., 1994). The immunostimulating properties of melatonin depend on activated CD4+ cells, which upon melatonin stimulation, show an increased synthesis and/or release of opioid peptides, IL-2 and IL-4, and INF-γ (Maestroni, 1993, 1995). Especially the Th2 variant of T-helper cells is a target of the circadian melatonin signal. Th2 cells are sensitive to INF-γ, which selectively inhibits their proliferative response (Del Prete et al., 1994). INF-γ in turn stimulates melatonin production in the pineal gland (Withyachumnarnkul et al., 1990), while IL-4 inhibits INF-γ production by activated T-cells (Banchereau, 1991), contributing to the balance of pro- and anti-inflammatory and immunostimulating factors. Immune competent cells like macrophages and their predecessors, monocytes, can also synthesize melatonin and serotonin from tryptophan in peripheral blood and tissues (Finocchiaro et al., 1988, 1991), forming a peripheral immunomodulary circuit also involving INF-γ (Finocchiaro et al., 1988). This bidirectional interaction leads to a complex pineal-immune axis, in which melatonin-induced Th2 cytokines, such as IL-4, exert a feedback on Th1 cytokines, such as INF-γ, and restrain their immunostimulating effect on melatonin synthesis. The pineal-immune axis helps to maintain the Th1-Th2 balance, which is instrumental in developing a successful and balanced immune response.
Pinealectomy or functional pinealectomy by constant light or by the β-adrenergic blocker propanolol leads to a decreased ability to mount a primary antibody response (Maestroni and Pierpaoli, 1981). Melatonin can augment the immune response and correct these immunodeficiency states and those that may follow acute stress, viral diseases, or immunosuppressive drug treatment and those found during the process of aging (Maestroni and Conti, 1993). In experimental autoimmune disorders, melatonin acts as an immune stimulant during the development of the disease and accentuates its severity (Hansson et al., 1992; Mattsson et al., 1994).
The effects of melatonin and of drugs acting on melatonin synthesis are strictly circadian time dependent. In animal experiments, the immune suppression by the β-adrenergic blocker propanolol occurred only after evening administration, but not after morning administration of the drug, and could be reversed only by evening administration of melatonin (Maestroni et al., 1987a). Similarly, immune enhancement by melatonin in intact animals was found only when melatonin was injected during the late afternoon or evening (Maestroni et al., 1988). Melatonin given in the morning was either ineffective or even immunosuppressive (Maestroni et al., 1987b).
TSH shows a high-amplitude circadian rhythm and seasonal variation, presumably at least in part as a response to environmental temperature (Haus et al., 1988). Both TRH and TSH interact with the immune system. TSH was reported to augment both T-dependent and T-independent antibody production (Blalock, 1992), to increase the proliferative response of mouse lymphocytes to lectin mitogens, and to stimulate IL-2-induced NK cell activity (Provinciali et al., 1992), apparently acting on both T- and B-cells. TRH induces TSH production by lymphocytes and its effect on the immune system may be mediated by this mechanism (Kruger et al., 1989).
Prolonged, hard, physical exercise combined with sleep and energy deprivation as experienced, for example, during military training courses may lead to rhythm alterations of numerous neuroendocrine variables interacting with the immune system (Opstad, 1991, 1992). Severe physical exercise together with sleep deprivation maintained throughout a span of 5 days leads to partial and, for some of the neuroendocrine variables, complete disappearance of circadian rhythmicity as a group phenomenon (Opstad, 1994). This observation may be due to actual disappearance of a recognizable circadian rhythm due to the severe stress (masking) or due to a desynchronization among the members of the group studied. Some changes (e.g., in the circadian mean of plasma cortisol) were still present 4 to 5 days after termination of the exposure to the combined-exercise sleep deprivation stress. Since optimal function in the human body depends on the "right" sequence of events as expressed by a certain phase relation ("internal timing") of the biologic rhythms within a frequency range, a disruption of this sequence that results in the right metabolite not being provided at the right time may lead to malfunction and immune deficiencies, as seen during exposures to phase shift, sleep deprivation, severe stress, and other
factors. Some of these exposures may be unavoidable in a military setting, but they should be recognized as a problem for soldiers and should be included, wherever feasible, in the planning for military deployment and action.
Chronopharmacology and Chronotherapeutics
The rhythmicity of events in the immune system and in related functions provides an opportunity to predict the time when certain events are most likely to occur and allows the recognition of transient risk states for immune-related phenomena (e.g., the circadian peak in sensitivity to allergen exposure or the circaseptan response pattern in allograft rejection). The prediction of such risk states allows one to initiate preventive measures or to treat an immune-related disorder at a time when optimal effects can be obtained. Both the time-dependent changes in the pharmacokinetics of an agent (chronopharmacokinetics) and the time-dependent changes in the host response (chronopharmacodynamics) must be considered in the design of a chronotherapeutic treatment schedule (Wood and Hrushesky, 1994). Timing of treatment with INF-α, according to the circadian rhythm in susceptibility and/or tolerance of the patient, has led to improved therapeutic effects and decreased toxicity (Depres-Brummer et al., 1991). Timing of treatment with cytokines and/or their inhibitors (including soluble receptors and receptor antagonists) is also expected to be effective in the immunotherapy of cancer (Kopp et al., 1994; Lissoni et al., 1990, 1993, 1995; Soiffer et al., 1996), in preventing allograft rejection in several forms of critical illness (Souba, 1994), in the treatment of rheumatoid arthritis (Breedveld, 1994; D. A. Fox, 1995a; Maini et al., 1994; Rankin et al., 1995; Wendling et al., 1993), and for numerous other conditions.
Immunosuppression by corticosteroids in patients with kidney allografts was most effective and accompanied by the fewest side effects if the dosing occurred in the morning, as compared with groups of patients taking the drug in the evening or throughout the day (Knapp et al., 1980). The toxic effects of immunosuppressive drugs are still a major obstacle for use in human subjects (Shaw et al., 1996). If a treatment with immunotoxic agents must be used, for example, in cancer chemotherapy, the time of maximal resistance of the relevant rhythm in the immune system of the host can be chosen to achieve the therapeutic effect with a minimum of undesirable side effects (for reviews, see Hrushesky, 1994; Hrushesky and März, 1994; Lemmer, 1989; Levi, 1996b; Levi et al., 1988a; Reinberg et al., 1991).
Seasonal as well as circadian variations in the toxicity of chemotherapeutic agents were found for epirubicin and for doxorubicin (Mormont et al., 1988), THP (4'-0-tetrahydropyranyladriamycin) (Levi et al., 1988a), and other agents (Hrushesky and März, 1994). Manipulation of the feeding schedule in mice was shown to alter the circadian rhythm in toxicity of adriamycin (Halberg, 1974) and of the immunosuppressant chemotherapeutic agent, methotrexate (Song et al., 1993) regardless of the light-dark regimen.
In animal experiments, the timing of cyclosporine treatment, according to the circadian, circaseptan, and circannual rhythm of the toxicity of this agent, led to a substantial improvement of the toxic-therapeutic ratio and of the survival of the allografted animals (Liu et al., 1986). In human subjects, cyclosporine shows a circadian rhythm in its pharmacokinetics after oral dosing (Ohlman et al., 1993; Sabate et al., 1990; Venkataramanan et al., 1986) and during continuing infusion (Heifets et al., 1995; Ohlman et al., 1993). Peak plasma concentrations were found in the early morning hours, between 03:30 07:30, and the lowest values between 15:30 and 19:30 (Heifets et al., 1995). The lowest rate of drug clearance occurred early in the morning, and the highest rate occurred in the afternoon. Monitoring of drug levels was recommended during the morning hours (around 07:00–08:00), which correspond to the nadir phase of the circadian variation of drug effect (Heifets et al., 1995). The importance of these findings for the therapeutic effect of cyclosporine in patients needs to be explored. The treatment with immunosuppressive agents can be timed for the stages in the circadian or other rhythms in the immune system either to achieve maximal immunosuppression with a minimal dose of the agent or to minimize immunosuppression if other effects of the agents are desired.
Further exploration of the time structure of the immune system will provide the background for numerous chronotherapeutic applications to treatment with growth factors, cytokines, and their agonists and antagonists. Immunoenhancement as well as immunosuppression can gain from chronotherapeutic consideration in dosing times (Halberg and Halberg, 1980; Knapp et al., 1980; Liu et al., 1986).
Author's Conclusions
The following conclusions may be drawn from this review:
- The neuroendocrine and immune system form a complex web of interactions and controls at all levels of integration. Most of the variables encountered are not stationary but show rhythms in multiple frequencies (circadian, circaseptan, circannual, and others).
- Some of the rhythms affecting the immune system are genetically fixed (endogenous), but in certain frequencies (e.g., circadian) the rhythm may be adjusted in its timing by periodic environmental factors (synchronizers, entraining agents, "zeitgeber"), such as light-darkness, activity-rest pattern, temperature, and for some parameters in human subjects less than in experimental animals, the time of food uptake.
- Rhythmic changes in the functional stage of cell and organ systems are predictable in their timing if (1) the rhythm is known to be synchronized by a rhythmic environmental factor (e.g., light-darkness, activity-rest), (2) if the rhythm can be monitored directly (e.g., the response of lymphocytes to mitogens), or (3) if the rhythm has a fixed phase relation to a variable that can
- be studied as time reference or so-called marker rhythm (e.g., sleep-wakefulness pattern, body temperature, activity, salivary solutes, circulating leukocytes).
- Environmental factors, as they are encountered in daily life and especially in the military climate, can transiently alter (mask) the expression of rhythms (e.g., plasma catecholamines or cortisol) without necessarily changing the underlying rhythmic mechanisms.
- Factors that affect neuroendocrine rhythms also affect immune functions (e.g., sleep and sleep deprivation; physical exercise, moderate or exhaustive; phase shifts; or other rhythm disturbances, including shift work).
- The rhythmic variations in immune-related parameters must be taken into account in any studies and testing procedures. For some of these parameters, the amplitude of these rhythms may be quite large. Experimental designs in animal as well as in human studies can take advantage of the relative predictability of rhythmic variations and detect these rhythm alterations (e.g., a phase shift). Failure to do so may lead to misleading results.
- Rhythms in immune-related functions may determine differences in the primary immune response and in the response to antigenic challenge depending on the stage of the rhythm in which the exposure occurs.
- Rhythms in immune-related functions can lead to time-dependent differences in the response to vaccination. In the circadian range, a stronger immune response was noted in diurnally active subjects after vaccination in the afternoon (hepatitis B vaccine) or in subjects vaccinated at noon or during the afternoon (influenza vaccine). In the latter study this temporal difference in vaccination effect was observed for some antigens, but not for others. The clearance of the antigen (viral particles) and the metabolic response showed differences in extent and duration as a function of the time of vaccination (Venezuelan equine encephalitis attenuated viral vaccine), with more pronounced changes in subjects vaccinated at 20:00 as compared with 08:00.
The local response to vaccination and/or revaccination was most pronounced after antigen exposure in the afternoon. However, the observed changes were quantitative, and all subjects in these studies did achieve immunity irrespective of the circadian time of vaccination. Nevertheless, some investigators suggest that the timing of vaccination may have some importance in subjects with poor antibody formation.
- Rhythmic infradian (most frequently circaseptan) response patterns are found during tissue regeneration, the regeneration of organs of the immune system after immunosuppression, and in the development of humoral- and cell-mediated immunity after introduction of an antigen. Similar reaction patterns can be triggered by metabolic loads. It is not known if exposure to prolonged stress as experienced in the military climate leads to the manifestation of rhythmic reaction patterns.
- Allograft rejection is characterized by rhythmicity of its clinical manifestations, with circadian and circaseptan periods observed. The relative predictability of these rhythmically occurring events may allow preventive
- treatment at the time when needed and no treatment or decreased dosage at times when rejection reactions are unlikely, thus decreasing undesirable side effects and cost. The feasibility of such an approach has been shown in animal experiments.
- Timing of administration according to circadian or infradian rhythm stages can be of help in treatment with growth factors and cytokines, or with their agonists or antagonists, and in treatment with immunosuppressive or chemotherapeutic agents in which immunosuppression is an undesirable side effect. Timing of treatment according to the stages of a sensitivity or resistance cycle may attain optimal effects with a minimal dose of an agent and/or the desired effects with minimal toxicity (e.g., cancer chemotherapy). These possibilities have been widely explored in animal experiments and recently have also been applied to clinical medicine where they are expected to gain much wider use in the near future.
- In disorders of the immune system (e.g., AIDS), changes in the rhythmic variations of some of its components may be of importance and may precede the clinical manifestations of the disease.
References
Abo, T., T. Kawate, K. Itoh, and K. Kumagai. 1981. Studies on the bioperiodicity of the immune response. I. Circadian rhythms of human T-, B-, and K-cell traffic in the peripheral blood. J. Immunol. 126:1360-1363.
Alevizatos, A.C., R.W. McKinney, and R.D. Feigin. 1967. Live attenuated equine encephalomyelitis virus vaccine. I. Clinical response of man to immunization. Am. J. Trop. Med. Hyg. 16:762-768.
Angeli, A., G. Gatti, M.L. Sartori, and R.G. Masora. 1992. Chronobiological aspects of the neuroendocrine immune network. Regulation of human natural killer (NK) cells as a model . Chronobiologia 19:93-110.
Araneo, B.A., M.L.I. Woods, and R.A. Daynes. 1993. Reversal of the immunosenescent phenotype by dehydroepiandrosterone: Hormone treatment provides an adjuvant effect on the immunization of aged mice with recombinant hepatitis B surface antigen. J. Infect. Dis. 167:830-840.
Arendt, J. 1994. The pineal. Pp. 348-362. In Biologic Rhythms in Clinical and Laboratory Medicine, Y. Touitou and E. Haus, editors. 2nd ed. Heidelberg: Springer-Verlag.
Arvidson, N.G., B. Gudbjornson, L. Elfman, and A.C. Ryden. 1994. Circadian rhythm of serum interleukin 6 in rheumatoid arthritis. Ann. Rheum. Dis. 53(8):521-524.
Auernhammer, C.J., and C.J. Strasburger. 1995. Effects of growth hormone and insulin-like growth factor I on the immune system. Eur. J. Endocrinol. 133:635-645.
Auzeby, A., A. Bogdan, Z. Krosi, and Y. Touitou. 1988. Time dependence of urinary neopterin, a marker of cellular immune activity. Clin. Chem. 34:1866-1867.
Badwe, R.A., W.M. Gregory, M.A. Chaudary, and M.A. Richards. 1991. Timing of surgery during the menstrual cycle and survival of the premenopausal woman with operable breast cancer. Lancet 337:1261-1264.
Banchereau, J. 1991. Interleukin-4. Pp. 119-149 in The Cytokine Handbook, A. Thomson, ed. London: Academic Press.
Barnes, P.J. 1984a. Autonomic control of the airways in nocturnal asthma. Pp. 69-73 in Nocturnal Asthma, P.J. Barnes and J. Levy, eds. Oxford: Oxford University Press.
Barnes, P.J. 1984b. Nocturnal asthma: Mechanisms and treatment. Br. Med. J. 288:1397-1398.
Barton, B.E., and J.V. Jackson. 1993. Protective role of interleukin-6 in the lipopolysaccharide-galactosamine septic shock model. Infect. Immun. 61:1496-1499.
Bayle, J.E., M. Guellati, F. Ibos, and J. Roux. 1991. Brucella abortus antigen stimulates the pituitary-adrenal axis through the extrapituitary B-lymphoid system. Prog. Neuroendocrinol. Immunol. 4:99-105.
Bellido, T., G. Girasole, G. Passeri, X.P. Yu, H. Mocharla, R.L. Jilka, A. Notides, and S.C. Manolagas. 1993. Demonstration of estrogen and vitamin D receptors in bone marrow-derived stromal cells: Upregulation of the estrogen receptor by 1,25-dihydroxyvitamin D3. Endocrinology 133:553-562.
Benatar, S.R. 1986. Fatal asthma. N. Engl. J. Med. 314:423-429.
Beneviste, E.N. 1992. Cytokines: Influence on glial cell gene expression and function. Pp. 106-153 in Neuroimmunoendocrinology: Chemical Immunology, 2d ed., J.E. Blalock, ed. Basel: Karger.
Berczi, I., F.D. Baragar, I.M. Chalmers, E.C. Keystone, E. Nagy, and R.J. Warrington. 1993. Hormones in self tolerance and autoimmunity: A role in the pathogenesis of rheumatoid arthritis. Autoimmunity 16:45-56.
Bernton, E., M.S. Meltzer, and J.W. Holaday. 1988. Suppression of macrophage activation and T-lymphocyte function in hypoprolactinemic mice. Science 239:401-404.
Besedovsky, H.O., and A. del Rey. 1992. Immune-neuroendocrine circuits: Integrative role of cytokines [Review]. Front Neuroendocrinology. 13(1):61-94.
Besedovsky, H.O., and A. del Rey. 1996. Immune-neuro-endocrine interactions: Facts and hypothesis. Endocr. Rev. 17:64-102.
Bixby, E.K., F. Levi, R. Haus, F. Halberg, and W. Hrushesky. 1982. Circadian aspects of murine nephrotoxicity of cisdiamminedichloroplatinum (II). Pp. 339-347 in Toward Chronopharmacology, R. Takahashi, ed. Oxford, New York: Pergamon Press.
Bjarnason, G.A., and W.J.M. Hrushesky. 1994. Cancer chronotherapy. Pp. 241-263 in Circadian Cancer Therapy, W.J.M. Hrushesky, ed. Boca Raton, Fla.: CRC Press.
Blalock, J.E. 1989a. A molecular basis for bidirectional communication between the immune and neuroendocrine systems. Physiol. Rev. 69:1-32.
Blalock, J.E. 1989b. A production of peptide hormones and neurotransmitters by the immune system. Pp. 1-19 in Neuroimmunoendocrinology: Chemical Immunology, 2d ed., J.E. Blalock, ed. Basel: Karger.
Blalock, J.E. 1994. The immune system: Our sixth sense. Immunologist 1994:8-15.
Bourin, P., F. Levi, I. Mansour, C. Doinel, and M. Joussemet. 1990. Circadian rhythm of interleukin-1 (IL-1) in serum of healthy men. Ann. Rev. Chronopharmacol. 7:201-204.
Bourin, P., I. Mansour, F. Levi, J.M. Villette, R. Roue, J. Fiet, P. Rouger, and C. Doinel. 1989. Perturbations precoces des rhythmes circadiens des lymphocytes T et B au cours de l'immunodeficience humaine (VIH). CR Acad. Sci. (Paris) 308:431-436.
Bratescu, A., and M. Teodorescu. 1981. Circannual variations in the B-cell/T-cell ratio in normal human peripheral blood. J. Allergy Clin. Immunol. 68:273-280.
Breedveld, F. 1994. Tenidap: A novel cytokine-modulating antirheumatic drug for the treatment of rheumatoid-arthritis. Scand. J. Rheumatol. 100:31-44.
Breus, T.K., F.I. Komarov, M.M. Musin, I.V. Naborov, and S.I. Rapoport. 1989. Heliogeophysical factors and their influence on cyclical processes in biosphere. Itogi Nauki i Techniki: Medicinskaya Geografica 18:138-142.
Britton, S., and G.H. Moller. 1968. Regulation of antibody synthesis against Escherichia coli endotoxin. I. Suppressive effects of endogenously produced and passively transferred antibodies. J. Immunol. 100:1326-1334.
Brock, M.A. 1983. Seasonal rhythmicity in lymphocyte blastogenesis persists in a constant environment. J. Immunol. 130:2586-2588.
Brock, M.A. 1987. Age-related changes in circannual rhythms of lymphocyte blastogenic response in mice. Am. J. Physiol. 252:R299-R305.
Brock, M.A. 1991. Chronobiology of aging. J. Am. Geriatr. Soc. 39:74-91.
Brown, R., G. Pang, A.J. Husband, and M.C. King. 1989. A suppression of immunity to influenza virus infection in the respiratory tract following sleep deprivation. Regul. Immunol. 2:321-325.
Calderon, R.A., and D.B. Thomas. 1980. In vivo cyclic change in B-lymphocyte susceptibility to T-cell control . Nature (Lond.) 285:662-664.
Cannon, J.G., and C.A. Dinarello. 1985. Increased plasma interleukin-1 activity in women after ovulation. Science 227:1247-1249.
Canon, C., F. Levi, Y. Touitou, J. Sulon, E. Demey-Ponsart, A. Reinberg, and G. Mathe. 1986. Variations circadienne et saisonniere du rapport inducteur: Suppresseur (OKT4 + OKT8+) dans la sang veineux de l'homme adulte sain. C.R. Acad. Sci. III 302:519-524.
Carandente, F., and F. Halberg. 1976. Chronobiologic view of shift-work and ulcers. Pp. 273-283 in NIOSH Research Symposium, Shift-Work and Health, Cincinnati, P.G. Rentos and R.D. Shepard, eds. Cincinnati: National Institute for Occupational Safety and Health.
Carr, D.J.J. 1991. The role of endogenous opioids and their receptors in the immune system. Soc. Exp. Biol. Med. 198:710-720.
Carr, D.J.J. 1992. Neuroendocrine peptide receptors on cells of the immune system. Pp. 49-83 in Neuroimmunoendocrinology: Chemical Immunology, 2d ed., J.E. Blalock, ed. Basel: Karger.
Clark, R., J. Strasser, S. McCabe, K. Robbins, and P. Jardieu. 1993. Insulin-like growth factor-1 stimulation of lymphopoiesis. J. Clin. Invest. 92:540-548.
Clarke, B.L., and K.L. Bost. 1989. Differential expression of functional adrenocorticotropic hormone receptors by subpopulations of lymphocytes. J. Immunol. 143:464-469.
Clevenger, C.V., D.H. Russell, P.M. Appasamy, and M.B. Prystowsky. 1990. Regulation of interleukin-2 driven T-lymphocyte proliferation by prolactin. Proc. Natl. Acad. Sci. USA 87:6460-6464.
Cornélissen, G., T.K. Breus, C. Bingham, R. Zaslavskaya, M. Varshitsky, B. Mirsky, M. Teibloom, B. Tarquini, E. Bakken, and F. Halberg. 1993. Beyond circadian chronorisk: Worldwide circaseptan-circasemiseptan patterns of myocardial infarctions, other vascular events, and emergencies. Chronobiologia 20:87-115.
Cove-Smith, J.R., P. Kabler, R. Pownall, and M.S. Knapp. 1978. Circadian variation in an immune response in man. Br. Med. J. 2(6132):253-254.
Covelli, V., F. Massari, C. Fallarca, I. Munno, F. Jirrilo, S. Savastano, A. Tommaselli, and G. Lombardi. 1992. Interleukin-1β and β-endorphin circadian rhythms are inversely related in normal and stress-altered sleep. Int. J. Neurosci. 63:299-305.
Cricchio, I., G. Arcara, G. Abbate, and M.F. Cannonito. 1978. Aspetti cronobiologia delle immunoglobuline sieriche. Folia Allergol. Immunol. Clin. 24:470.
Cricchio, I., G. Aracara, G. Abbate, T. Ferrar, R. Tarantino, and S. Romano. 1979. Circadian rhythms of serum immunoglobulins in patients affected with allergic rhinitis, atopic asthma and chronic urticaria. Pp. 55-63 in Recent Advances in the Chronobiology of Allergy and Immunology, M.H. Smolensky, A. Reinberg, and J.P. McGovern, eds. Oxford: Pergamon Press.
Da Silva, J.A., and G.M. Hall. 1992. The effect of gender and sex hormones on outcome in rheumatoid arthritis. Clin. Rheumatol. 6:196-219.
Damle, N.K., and S. Gupta. 1982. Autologous mixed lymphocyte reaction in man. III. Regulation of autologous MLR by theophylline-resistant and sensitive human T-lymphocyte subpopulations. Scand. J. Immunol. 15:493-499.
Daniel, L., G. Souweine, J.C. Monier, and S. Saez. 1983. Specific estrogen binding sites in human lymphoid cells and thymic cells. Esteroid Biochem. 18:559-563.
Daynes, R.A., and B.A. Araneo. 1992. Prevention and reversal of some age-associated changes in immunologic responses by supplemental dehydroepiandrosterone sulfate therapy. Aging Immunol. Infect. Dis. 3:135-154.
Daynes, R.A., B.A. Araneo, J. Hennebold, E. Enioutina, and H.M. Hong. 1995. Steroids as regulators of the mammalian immune response. J. Invest. Dermatol. 105:14S-19S.
Del Prete, G., E. Maggi, and S. Romagnani. 1994. Human Th1 and Th2 cells: Functional properties, mechanisms of regulation and role in disease. Lab. Invest. 70:299-307.
DeLeonardis, V., and M.L. Brandi. 1984. Total IgE serum levels: A chronobiologic approach. Pp. 395-398 in Chronobiology 1982-1983, E. Haus and H.F. Kabat, eds. Basel: Karger.
Depres-Brummer, P., F. Levi, M. Palma, A. Beliard, P. Lebon, S. Marion, C. Jasmin, and J. Misset. 1991. A phase I trial of 21-day continuous venous infusion of α-interferon at circadian rhythm modulated rate in cancer patients. J. Immunother. 10:440-447.
DeSarro, G.B., Y. Masuda, C. Ascioti, M.G. Audino, and G. Nistico. 1990. Behavioral and ECoG spectrum changes induced by intracerebral infusion of interferons and interleukin-2 in rats are antagonized by naloxone. Neuropharmacology 29:167-179.
Dethlefsen, U., and R. Repges. 1985. Ein neues Therapieprinzip bei nachtlichem Asthma. Med. Klin. 80:40-47.
DeVecchi, A., F. Halberg, R.B. Sothern, A. Cantaluppi, and C. Ponticelli. 1981. Circaseptan rhythmic aspects of rejection in treated patients with kidney transplant. Pp. 339-353 in Chronopharmacology and Chemotherapeutics, C.A. Walker, C.M. Winget, and K.F.A. Soliman, eds. Tallahassee, FL: A and M University Foundation.
DeVries, K., J.T. Goei, H. Booy-Noord, and N.G.M. Orie. 1962. Changes during 24 hours in the lung function and histamine hyperreactivity of the bronchial tree in asthmatic and bronchitic patients. Int. Arch. Allerg. 20:93-101.
Dixon-Northern, A.L., S.M. Rutter, and C.M. Peterson. 1994. Cyclic changes in the concentration of peripheral blood immune cells during the normal menstrual cycle. Proc. Soc. Exp. Biol. Med. 207:81-88.
Edmunds, L.N.J. 1994. Cellular and molecular aspects of circadian oscillators: Models and mechanisms for biological timekeeping. Pp. 35-54 in Biologic Rhythms in Clinical and Laboratory Medicine, 2d ed., Y. Touitou and E. Haus, eds. Heidelberg: Springer-Verlag.
Eichler, F., and R. Keiling. 1988. Variations in the percentages of lymphocyte subtypes during the menstrual cycle in women. Biomed. Pharmacother. 42:285-288.
Everson, C.A. 1993. Sustained sleep deprivation impairs host defense. Am. J. Physiol. 265:R1148-R1154.
Fabris, N., E. Mocchegiani, and M. Provinciali. 1995. Pituitary-thyroid axis and immune system: A reciprocal neuroendocrine-immune interaction. Horm. Res. 43:29-38.
Feigin, R.D., R.F. Jaeger, R.W. McKinney, and A.C. Alevizatos. 1967a. Live, attenuated Venezuelan equine encephalomyelitis virus vaccine. II. Whole-blood amino-acid and fluorescent-antibody studies following immunization. Am. J. Trop. Med. Hyg. 16:769-777.
Feigin, R.D., A.S. Klainer, and W.R. Beisel. 1967b. Circadian periodicity of whole-blood amino acids in adult men. Nature 215:512-514.
Feldmann, J.F. 1985. Genetic and physiological analysis of a clock gene in Neurospora crassa. Pp. 238-245 in Temporal Order, L. Rensing and N.I. Jaeger, eds. Berlin, Heidelberg, and New York: Springer.
Fernandes, G. 1994. Chronobiology of immune functions: Cellular and humoral aspects. Pp. 493-503 in Biologic Rhythms in Clinical and Laboratory Medicine, Y. Touitou and E. Haus, eds. Heidelberg: Springer-Verlag.
Fernandes, G., F. Halberg, and R.A. Good. 1980. Circadian dependent chronoimmunological responses of T-, B-, and natural killer cells. Allergology 3:164-170.
Fernandes, G., F. Halberg, E. Yunis, and R.A. Good. 1976. Circadian rhythmic plaque-forming cell response of spleens from mice immunized by SRBC. J. Immunol. 117:962-966.
Fernandes, G., E.J. Yunis, and F. Halberg. 1977. Circadian aspect of immune response in the mouse. Pp. 233-249 in Chronobiology in Allergy and Immunology, J.P. McGovern, M.H. Smolensky, and A. Reinberg, eds. Springfield, Ill.: Charles C Thomas.
Fernandes, G., E.J. Yunis, W. Nelson, and F. Halberg. 1974. Differences in immune response of mice to sheep red blood cells as a function of circadian phase. Pp. 329-338 in Chronobiology. Proceedings of the International Society for the Study of Biological Rhythms, L.E. Scheving, F. Halberg, and J.E. Pauly, eds. Stuttgart and Tokyo: Thime/Igaku Shoin.
Finocchiaro, L.E., E. Nahmod, and J.M. Launay. 1991. Melatonin biosynthesis and metabolism in peripheral blood mononuclear leukocytes . Biochem. J. 280:727-732.
Finocchiaro, L.M.E., E.S. Arzt, S. Fernandez-Castelo, M. Criscuolo, S. Finkielman, and V.E. Nahmod. 1988. Serotonin and melatonin synthesis in peripheral blood mononuclear cells: Stimulation by interferon-γ as part of an immunomodulatory pathway. J. Interferon Res. 1988:705-716.
Fletscher, B. 1993. Zirkadiane Variation der Antikorperbildung nach Hepatitis-B-Impfung. Deutsch. Med. Wschr 118:999.
Fox, D.A. 1995a. Biological therapies: A novel approach to the treatment of autoimmune disease. Am. J. Med. 99:82-88.
Fox, H.S. 1995b. Sex steroids and the immune system [Review]. CIBA Foundation Symposium. 191:203-211.
Fox, H.S., B.L. Bond, and T.G. Parslow. 1991. Estrogen regulates the INF-γ promoter. J. Immunol. 146:4362-4367.
Fuller, R. and H. Snoddy. 1968. Feeding schedule alteration of daily rhythm in tyrosine α-ketoglutaride transaminase in rat liver. Science 159:738.
Gala, R.R., and E.M. Shevach. 1993. Identification by analytical flow cytometry of prolactin receptors on immunocompetent cell population in the mouse. Endocrinology 133:1617-1623.
Gala, R.R., and E.M. Shevach. 1994. Evidence for the release of a prolactin-like substance by mouse lymphocytes and macrophages. Proc. Soc. Exp. Biol. Med. 205:12-19.
Gamaleya, N.F., E.D. Silisko, and A.P. Cherny. 1988. Preservation of circadian rhythms by human leukocytes in vitro. Byull. Eksper. Biol. Med. 106:598-600.
Garg, M., and S. Bondada. 1993. Reversal of age-associated decline in immune response to pnu-immune vaccine by supplementation with the steroid hormone dehydroepiandrosterone. Infect. Immun. 61:2238-2241.
Gatti, G., R. Cavallo, M.L. Sartori, R. Carignola, R. Masera, D. Delponte, A. Salvadori, and A. Angeli. 1988. Circadian variations of interferon-induced enhancement of human natural killer (NK) cell activity. Cancer Detect. 12:431-438.
Gatti, G., R. Cavallo, M.L. Sartori, D. Delponte, R.G. Masera, A. Salvadori, R. Carignola, and A. Angeli. 1987. Inhibition by cortisol of human natural killer (NK) cell activity. Steroid. Biochem. 26:49-58.
Gatti, G., R. Cavallo, M.I. Sartori, C. Marinone, and A. Angeli. 1986. Cortisol at physiological concentrations and prostaglandin E2 are additive inhibitors of human nature killer cell activity. Immunopharmacology 11:119-128.
Gatti, G., R.G. Masera, R. Carignola, M.L. Sartori, E. Margro, and A. Angeli. 1990. Circadian-single-dependent enhancement of human natural killer cell activity by melatonin. J. Immunol. Res. 2:108-116.
Gaultier, C., G. DeMontis, A. Reinberg, and Y. Motohashi. 1987. Circadian rhythm of serum total immunoglobulin E (IgE) in asthmatic children. Biomed. Pharmacother. 41:186-188.
Gibinski, K., A. Nowak, J. Rybicka, and K. Czarnecka. 1979. An endoscopic study on the natural history of gastroduodenal ulcer disease . Mater. Med. Pol. 11:265-269.
Gibson, L.F., D. Piktel, and K.S. Landreth. 1993. Insulin-like growth factor-I potentiates expansion of interleukin-7-dependent pro-B-cells. Blood 82:3005-3011.
Gilblett, E.R., J.E. Anderson, F. Cohen, B. Pollara, and H.J. Meuwissen. 1972. Adenosine deaminase deficiency in two patients with severely impaired cellular immunity. Lancet II:1067-1069.
Goetz, F., J. Bishop, F. Halberg, R. Sothern, R. Brunning, B. Senske, B. Greenberg, D. Minors, P. Stoney, I.D. Smith, G.D. Rosen, D. Cressey, E. Haus, and M. Apfelbaum. 1976. Timing of single daily meal influences relations among human circadian rhythms in urinary cyclic AMP and hemic glucagon, insulin, and iron. Experientia (Basel) 32:1081-1084.
Graf, M.V., and A.J. Kastin. 1984. Delta-sleep-inducing peptide (DSIP): A review. Neurosci. Biobehav. Rev. 8:83-93.
Grossman, Z., R. Asofsky, and C. Delisi. 1980. The dynamics of antibody secreting cell production, regulation of growth, and oscillations in the response to T-independent antigens. J. Theor. Biol. 84:49-92.
Gudewill, S., T. Pollmacher, H. Vedder, W. Schreuber, K. Fassbender, and F. Holsboer. 1992. Nocturnal plasma levels of cytokines in healthy men. Eur. Arch. Psych. Clin. Neurosci. 242:53-56.
Haen, E., I. Langenmayer, A. Pangerl, B. Liebl, and J. Remien. 1988. Circannual variation in the expression of β2 adrenoceptors on human peripheral mononuclear leukocytes (MNLs). Klin. Wschr. 66:579-582.
Halberg, E., and F. Halberg. 1980. Chronobiologic study design in everyday life, clinic, and laboratory. Chronobiologia 7:95-120.
Halberg, F. 1974. From iatrotoxicosis and iatrosepsis towards chronotherapy. Pp. 1-34 in Chronobiologic Aspects of Endocrinology, J. Aschoff, F. Ceresa, and F. Halberg, eds. Stuttgart: Schattaner-Verlag.
Halberg, F., and C. Hamburger. 1964. 17-ketosteroids and volume of human urine. Weekly and other changes with low frequency. Minn. Med. 47:916-925.
Halberg, F., and A.N. Stephens. 1959. Susceptibility to ouabain and physiologic circadian periodicity. Proc. Minn. Acad. Sci. 27:139-143.
Halberg, F., C.P. Barnum, R. Silber, and J.J. Bittner. 1958. 24-hour rhythms at several levels of integration in mice on different lighting regimens. Proc. Soc. Exp. Biol. Med. 97:897-900.
Halberg, F., D. Duffert, and H. Von Mayersbach. 1977. Circadian rhythm in serum immunoglobulins of clinically healthy young men [abstract]. Chronobiologia 4:114.
Halberg, F., E. Haus, and G. Cornélissen. 1995. From biologic rhythms to chronomes relevant for nutrition. Pp. 361-372 in Not Eating Enough, Overcoming
Underconsumption of Military Operational Rations, B.M. Marriott, Ed. Committee on Military Nutrition Research, Food and Nutrition Board, Institute of Medicine. Washington, D.C.: National Academy Press.
Halberg, F., E.A. Johnson, B.W. Brown, and J.J. Bittner. 1960. Susceptibility rhythm to E. coli endotoxin and bioassay. Proc. Soc. Exp. Biol. Med. 103:142-144.
Halberg, J., E. Halberg, W. Runge, J. Wicks, L. Cadotte, E. Yunis, G. Katinas, O. Stutman, and F. Halberg. 1974. Transplant chronobiology. Pp. 320-328 in Chronobiology. Proceedings of the International Society for the Study of Biological Rhythms, L.E. Scheving, F. Halberg, and J.E. Pauly, eds. Stuttgart and Tokyo: Thieme/Igaku Shoin.
Hansson, I., R. Holmdahl, and R. Mattsson. 1992. The pineal hormone melatonin exaggerates development of collagen-induced arthritis in mice. J. Neuroimmunol. 39:23-30.
Hartmann, D.P., J.W. Holaday, and E.W. Bernton. 1989. Inhibition of lymphocyte proliferation by antibodies to prolactin. FASEB J. 3:2194-2202.
Hasty, L.A., J.D. Lambris, B.A. Lessey, K. Pruksananonda, and C.R. Lyttle . 1994. Hormonal regulation of complement and receptors throughout the menstrual cycle. Am. J. Obstet. Gynecol. 170:168-175.
Hattori, N., A. Shimatsu, M. Sugita, S. Kumagai, and H. Imura. 1990. Immunoreactive growth hormone (GH) secretion by human lymphocytes: Augmented release by exogenous GH. Biochem. Biophys. Res. Commun. 168:396-401.
Haus, E. 1959. Endokrines system und Blut. Pp. 181-286 in Handbuch der gesamten Hämatologie, L. Heilmeyer and A. Hittmair, eds. Munich: Urban und Schwarzenberg.
Haus, E. 1994. Chronobiology of circulating blood cells and platelets. Pp. 504-526 in Biologic Rhythms in Clinical and Laboratory Medicine, 2d ed., Y. Touitou and E. Haus, eds. Heidelberg: Springer Verlag.
Haus, E. 1996. Biologic rhythms in hematology. Path. Biol. 44(6):618-630.
Haus, E., and F. Halberg. 1978. Cronofarmacologia della neoplasia con speciale riferimento alla leucemia. Pp. 29-85 in Farmacologia Clinica e Terapia, A. Bertelli, ed. Turin: Edizioni.
Haus, E., and Y. Touitou. 1994a. Chronobiology in laboratory medicine. Pp. 673-708 in Biologic Rhythms in Clinical and Laboratory Medicine, 2d ed., Y. Touitou and E. Haus, eds. Heidelberg: Springer-Verlag.
Haus, E., and Y. Touitou. 1994b. Principles of clinical chronobiology. Pp. 6-34 in Biologic Rhythms in Clinical and Laboratory Medicine, 2d ed., Y. Touitou and E. Haus, eds. Heidelberg: Springer-Verlag.
Haus, E., L. Dumitriu, G.Y. Nicolau, D.J. Lakatua, H. Berg, E. Petrescu, L. Sackett-Lundeen, and R. Reilly. 1995. Time relation of circadian rhythms in plasma dehydroepiandrosterone and dehydroepiandrosterone-sulfate to ACTH, cortisol, and 11-desoxycortisol-#107 [abstract]. Biol. Rhythm Res. 26:(4)399.
Haus, E., F. Halberg, J.F.W. Juhl, and D.J. Lakatua. 1974a. Chronopharmacology in animals. Chronobiologia 1:122-156.
Haus, E., F. Halberg, M.K. Loken, and Y.S. Kim. 1974b. Circadian rhythmometry of mammalian radiosensitivity. Pp. 435-474 in Space Radiation Biology, C.A. Tobias and P. Todd, eds. New York: American Institute of Biological Science Academic Press.
Haus, E., F. Halberg, L.E. Scheving, J.E. Pauly, S. Cardoso, J.F.W. Kuhl, R.B. Sothern, R.N. Shiotsuka, and D.S. Hwang. 1972. Increased tolerance of leukemic mice to arabinosyl cytosine with schedule adjusted to circadian system. Science 177:80-82.
Haus, E., D.J. Lakatua, F. Halberg, E. Halberg, G. Cornélissen, L. Sackett, H. Berg, T. Kawasaki, M. Ueno, K. Uezono, M. Matsouka, and T. Omae. 1980.
Chronobiological studies of plasma prolactin in women in Kyushu, Japan, and Minnesota, U.S.A. J. Clin. Endocrinol. Metab. 51:632-640.
Haus, E., D.J. Lakatua, L. Sackett-Lundeen, and J. Swoyer. 1984. Chronobiology in laboratory medicine. Pp. 13-82 in Clinical Aspects of Chronobiology, W.J. Rietveld, ed. The Netherlands: Meducation Service Hoechst.
Haus, E., D.J. Lakatua, L. Sackett-Lundeen and M. White. 1984. Circannual variation of intestinal cell proliferation in BDF male mice on three lighting regimens. Chronobiol. Int. 1:185-194.
Haus, E., D.J. Lakatua, J. Swoyer, and L. Sackett-Lundeen. 1983. Chronobiology in hematology and immunology. Am. J. Anatomy 168:467-517.
Haus, E., G.Y. Nicolau, D.J. Lakatua, and L. Sackett-Lundeen. 1988. Reference values for chronopharmacology. Chronopharmacology 4:333-424.
Haus, M., L. Sackett-Lundeen, D. Lakatua, and E. Haus. 1984. Circannual variation of 3H-thymidine uptake in DNA of lymphatic organs irrespective of relative length of light and dark span [abstract]. J. Minn. Acad. Sci. 49(2):19.
Hayashi, O.K., and M. Kikuchi. 1982. The effects of the light-dark cycle on humoral and cell-mediated immune responses of mice. Chronobiologia 9:291-300.
Hedner, L., and G. Bynke. 1985. Endogenous iridocyclitis relieved during treatment with Bromocriptine. Am. J. Ophthal. 100:618-619.
Heifets, M., G.F. Cooney, L.M. Shaw, and G. Lebetti. 1995. Diurnal variation of cyclosporine clearance in stable renal transplant recipients receiving continuous infusion. Transplantation 60:1615-1617.
Herberman R.B., and D.H. Callewaert, eds. 1985. Mechanisms of Cytotoxicity by NK Cells. Orlando, Fla.: Academic Press.
Hetzel, M.R., T.J.H. Clark, and M.A. Branthwaite. 1977. Asthma: Analysis of sudden deaths and ventilatory arrest in hospital. Br. Med. J. 1:808-811.
Hiestand, P.C., P. Mekler, R. Nordmann, A. Grieder, and C. Permmongkol. 1986. Prolactin as a modulator of lymphocyte responsiveness provides a possible mechanism of action for cyclosporine. Proc. Natl. Acad. Sci. USA 83:2599-2603.
Homo-Delarche, F. 1984. Glucocorticoid receptors and steroid sensitivity in normal and neoplastic human lymphoid tissue: A review. Cancer Res. 44:431-437.
Homo-Delarche, F., F. Fitzpatrick, N. Christeff, E.A. Nunez, J.F. Bach, and M. Dardenne. 1991. Sex steroids, glucocorticoids, stress, and autoimmunity. J. Steroid Biochem. Mol. 40:619-637.
Hooghe, R., M. Delhase, P. Vergani, A. Malur, and E.L. Hooghe-Peters. 1993. Growth hormone and prolactin are paracrine growth and differentiation factors in the haemopoietic system. Immunol. Today 14:212-214.
Hoopes, P.C., and C.E. McCall. 1977. The effect of cobra venom factor (CVF) on activation of the complement cascade on leukocyte circadian variation in the rat. Experientia 33:224-226.
Hrushesky, W.J.M., ed. 1994. Circadian Cancer Therapy. Boca Raton, Fla.: CRC Press.
Hrushesky, W.J.M., and W.J. März. 1994. Chronochemotherapy of malignant tumors: Temporal aspects of antineoplastic drug toxicity. Pp. 611-634 in Biologic Rhythms in Clinical and Laboratory Medicine, 2d ed., Y. Touitou and E. Haus, eds. Heidelberg: Springer-Verlag.
Huber, C., J.R. Batchelor, D. Fuchs, A. Hauser, A. Lang, D. Niederwieser, G. Reitnegger, P. Swetly, J. Troppmair, and H. Wachter. 1984. Immune response associated production of neopterin. Release from macrophages primarily under control of interferon-γ. J. Exp. Med. 160:310-316.
Hübner, K. 1967. Kompensatorische Hypertrophie, Wachstum und Regeneration der Rattenniere. Ergebn Allg. Path. Path. Anat. 48:1-80.
Hughes, E.C., R.L. Johnson, and C.W. Whitaker. 1977. Circadian rhythmic aspects of secretory immunoglobulin A. Pp. 216-232 in Chronobiology in Allergy and Immunology, J.P. McGovern, M.H. Smolensky, and A. Reinberg, eds. Springfield, Ill.: Charles C Thomas.
Husband, A.J. 1993. Role of central nervous system and behavior in the immune response. Vaccine 11:805-816.
Indiveri, F., I. Pierri, S. Rogna, A. Poggi, P. Montaldo, R. Romano, A. Pende, A. Morgano, A. Barabino, and S. Ferrone. 1985. Circadian variations of autologous mixed lymphocyte reactions and endogenous cortisol. J. Immunol. Meth. 82:17-24.
Isenberg, D.A., A.J. Guisp, W.J.W. Morrow, D. Newham, and M.L. Snaith. 1981. Variations in circulating immune complex levels with diet, exercise, and sleep: A comparison between normal controls and patients with systemic lupus erythematosis. Ann. Rheum. Dis. 40:466-469.
Johnson, H.M., M.O. Downs, and C.H. Pontzer, 1992. Neuroendocrine peptide hormone regulation of immunity [Review]. Chemical Immunology 52:49-83.
Johnson, H.M., E.M. Smith, B.A. Torres, and J.E. Blalock. 1982. Neuroendocrine hormone regulation of in vitro antibody production. Proc. Natl. Acad. Sci. USA 79:4171-4174.
Jouvet, M. 1984. Neuromediateurs et facteurs hypnogenes. Rev. Neurol. 140:389-400.
Katila, H., K. Cantell, B. Appelberg, and R. Rimon. 1993. Is there a seasonal variation in the interferon-producing capacity of healthy subjects? J. Interferon Res. 13:233-234.
Kelso, A., and A. Munck. 1984. Glucocorticoid inhibition of lymphokine secretion by alloreactive T-lymphocyte clones. J. Immunol. 133:784-791.
Kern, J.A., R.J. Lamb, J.C. Reed, R.P. Daniele, and P.C. Nowell. 1988. Dexamethasone inhibition of interleukin-1β production by human monocytes. Postranscriptional Mechanisms. J. Clin. Invest. 81:237-244.
Kim, Y., M. Pallansch, F. Carandente, G. Reissmann, E. Halberg, and F. Halberg. 1980. Circadian and circannual aspects of the complement cascade--new and old results, differing in specificity? Chronobiologia 7:189-204.
Kimata, H., and A. Yoshida. 1994. Differential effect of growth hormone and insulin-like growth factor-I, insulin-like growth factor-II, and insulin on Ig production and growth in human plasma cells. Blood 83:1569-1574.
Kishida, T., M. Kita, M. Yamaji, E. Kaneta, and T. Teramatsu. 1987. Interferon-producing capacity in patients with liver diseases, diabetes mellitus, chronic diseases, and malignant diseases. Pp. 435-441 in The Biology of the Interferon System 1986, K. Cantel and H. Schellekens, eds. Dordrecht: Martinus Nijhoff Publishers.
Knapp, M.S., N.P. Byron, R. Pownall, and P. Mayor. 1980. Time of day of taking immunosuppressive agents after renal transplantation. Br. Med. J. 281:1382-1385.
Knapp, M.S., R. Pownall, and J.R. Cove-Smith. 1981. Circadian variations in cell-mediated immunity and in the timing of human allograft rejection. Pp. 329-338 in Chronopharmacology and Chronotherapeutics, C.A. Walker, C.M. Winget, and K.F.A. Soliman, eds. Tallahassee, Fla.: A&M University Foundation.
Konopka, R.J. 1979. Genetic dissection of the Drosophila circadian system. Fed. Proc. 38:2602-2605.
Kopp, W.C., J.T. Holmlund, and W.J. Urba. 1994. Immunologic monitoring and clinical trials of biological response modifiers. Cancer Chemother. Biol. Response Modif. 15:226-286.
Koren, S., and W.R. Fleischmann. 1993. Circadian variations in myelosuppressive activity of interferon-α in mice: Identification of an optimal treatment time associated with reduced myelosuppressive activity. Exp. Hematol. 21:552-559.
Kort, W.J., and J.M. Weijma. 1982. Effect of chronic light-dark shift stress on the immune response of the rat. Physiol. Behav. 29:1083-1087.
Krueger, J.M. 1990. Somnogenic activity of immune response modifiers. Trends Pharmacol. Sci. 11(3):122-126.
Krueger, J.M., C.A. Dinarello, and L. Chedid. 1983. Promotion of slow wave sleep (SWS) by a purified Interleukin-1(IL-1) preparation [abstract]. Fed. Proc. 42:356.
Krueger, J.M., J.R. Pappenheimer, and M.L. Karnovsky. 1982. The composition of sleeppromoting factor isolated from human urine. J. Biol. Chem. 25:1664-1669.
Krueger, J.M., E.J. Walter, C.A. Dinarello, S.M. Wolff, and L. Chedid. 1984. Sleeppromoting effects of endogenous pyrogen (Interleukin-1). Am. J. Physiol. 246:R994-R999.
Kruger, T.E., L.R. Smith, D.V. Harbour, and J.E. Blalock. 1989. Thyrotropin: An endogenous regulator of the in vitro immune response. J. Immunol. 142:744-747.
Kunkel, G., and L. Jusuf. 1984. Theoretical and practical aspects of circadian rhythm for theophylline therapy. Pp. 149-155 in New Perspectives in Theophylline Therapy, M. Turner-Warwick and J. Levy, eds. London: Royal Society of Medicine.
Lakatua, D.J. 1994. Molecular and genetic aspects of chronobiology. Pp. 65-77 in Biologic Rhythms in Clinical and Laboratory Medicine, 2d ed., Y. Touitou and E. Haus, eds. Heidelberg: Springer-Verlag.
Lakatua, D.J., E. Haus, K. Labrosse, C. Veit, and L. Sackett-Lundeen. 1986. Circadian rhythm in mammary cytoplasmic estrogen receptor content of Balb/C female mice with and without pituitary isografts. Chronobiol. Int. 3(4):213-219.
Lakatua, D.J., M. White, L.L. Sackett-Lundeen, and E. Haus. 1983. Change in phase relations of circadian rhythms in cell proliferation induced by time-limited feeding in Balb/C × D8A/2F1 mice bearing a transplantable Harding-Passey tumor. Cancer Res. 43:4068-4072.
Landreth, K.S., R. Narayanan, and K. Dorshkind. 1992. Insulin-like growth factor I regulates pro-B-cell differentiation . Blood 80:1207-1212.
Langlois, P.H., M.H. Smolensky, W.P. Glezen, and W.A. Keitel. 1995. Diurnal variation in responses to influenza vaccine. Chronobiol. Int. 12:28-36.
Lavie, P., and B. Weller. 1989. Timing of naps: Effects on post-nap sleepiness levels. EEG Clin. Neurophysiol. 72:218-224.
Lee, C., P. Vaishali, T. Itsukaichi, B. Kiho, and I. Edery. 1996. Resetting the Drosophila clock by photic regulation of PER and a PER-TIM complex. Science 271:1740-1744.
Lee, R.E., M.H. Smolensky, C.S. Leach, and J.P. McGovern. 1977. Circadian rhythms in the cutaneous reactivity to histamine and selected antigens, including phase relationship to urinary cortisol excretion. Ann. Allergy 38(4):231-236.
Lemmer, B., ed. 1989. Chronopharmacology, Cellular, and Biochemical Interactions. New York: Marcel Dekker.
Lemmer, B., U. Schwulera, A. Thrun, and R. Lissner. 1992. Circadian rhythm of soluble interleukin-2 receptor in healthy individuals. Eur. Cytokine Netw. 3:335-336.
Levi, F. 1994. Chronotherapy of cancer: Biological basis and clinical application. Pathol. Biol. (Paris) 42:338-341.
Levi, F. 1996a. Chronotherapy for gastrointestinal cancers. Curr. Opin. Oncol. 8:334-341.
Levi, F. 1996b. Chronopharmacologie et chronothérapie des cancers. Path. Biol. 44:631-644.
Levi, F. 1997. Chronopharmacology of Anticancer Agents. Pp. 299-332 in Physiology and Pharmacology of Biologic Rhythms. Handbook of Experimental Pharmacology. Volume 125, P.H. Redfern and B. Lemmer, eds. Heidelberg: Springer-Verlag.
Levi, F., and F. Halberg. 1982. Circaseptan (about 7 day) bioperiodicity--spontaneous and reactive--and the search for pacemakers. Ric. Clin. Lab. 12:323-370.
Levi, F., I. Blazsek, and A. Ferle-Vidovic. 1988a. Circadian and seasonal rhythms in murine bone marrow colony-forming cells affect tolerance for the anti-cancer agent 4-0-tetrahydropyranyl adriamycin (THP). Exp. Hematol. 16:696-701.
Levi, F., N.A. Boughattas, and I. Blazsek. 1986. Comparative murine chronotoxicity of anticancer agents and related mechanisms. Ann. Rev. Chronopharmacol. 4:283-331.
Levi, F., C. Canon, J.P. Blum, M. Mechkouri, A. Reinberg, and G. Mathe. 1985. Circadian and/or circahemidian rhythms in nine lymphocyte-related variables from peripheral blood of healthy subjects. J. Immunol. 134:217-222.
Levi, F., C. Canon, M. Dipalma, I. Florentin, and J.L. Misset. 1991. When should the immune clock be reset? From circadian pharmacodynamics to temporally optimized drug delivery. Ann. N.Y. Acad. Sci. 618:312-329.
Levi, F., C. Canon, Y. Touitou, A. Reinberg, and G. Mathe. 1988b. Seasonal modulation of the circadian time structure of circulating T- and natural killer lymphocyte subsets from healthy subjects. J. Clin. Invest. 81:407-413.
Levi, F.A., R. Zidani, J.M. Vannetzel, B. Perpoint, C. Focan, R. Faggiuolo, P. Chollet, C. Garufi, M. Itzhaki, and L. Dogliotti. 1994. Chronomodulated versus fixed-infusion-rate delivery of ambulatory chemotherapy with oxaliplatin, fluorouracil, and folinic acid (leucovorin) in patients with colorectal cancer metastases: a randomized multi-institutional trial. J. Natl. Cancer Inst. 86(21):1608-1617.
Lipa, B.J., P.A. Sturrock, and E. Rogot. 1976. Search for correlation between geomagnetic disturbances and mortality. Nature 259:302-304.
Lissoni, P., S. Barni, C. Archili, G. Cattaneo, F. Rovelli, A. Conti, G.J.M. Maestroni, and G. Tancini. 1990. Endocrine effects of a 24-hour intravenous infusion of interleukin-2 in the immunotherapy of cancer. Anticancer Res. 10:753-758.
Lissoni, P., S. Barni, G. Tancini, A. Ardizzoia, G. Ricci, R. Aldeghi, F. Brivio, E. Tisi, R. Rescaldani, G. Quadro, and G.J.M. Maestroni. 1994. A randomized study with subcutaneous low dose interleukin-2 alone vs. interleukin-2 plus the pineal neurohormone melatonin in advanced solid neoplasms other than renal cancer and melanoma. Br. J. Cancer 69:196-199.
Lissoni, P. S. Barni, G. Tancini, A. Ardizzoia, F. Rovelli, M. Cazzaniga, F. Brivio, A. Piperno, R. Aldeghi, D. Fossati, D. Characiejus, L. Kothari, A. Conti, and G.J.M. Maestroni. 1993. Immunotherapy with subcutaneous low dose interleukin-2 and the pineal indole melatonin as a new effective therapy in advanced cancers of the digestive tract. Br. J. Cancer 67:1404-1407.
Lissoni, P., S. Barni, G. Tancini, E. Maini, F. Piglia, G.J.M. Maestroni, and A. Lewinski 1995. Immunoendocrine therapy with low-dose subcutaneous interleukin-2 plus melatonin of locally advanced or metastatic endocrine tumors. Oncology 52:163-166.
Liu, T., M. Cavallini, F. Halberg, G. Cornélissen, J. Field, and D.E.R. Sutherland. 1986. More of the need for circadian, circaseptan, and circannual optimization of cyclosporine therapy. Experientia 42:20-22.
Lu, M.C., M.H. Smolensky, B. Hsi, and J.P. McGovern. 1979. Seasonal changes in immunoglobulin and complement levels in atopic and non-atopic persons. Pp. 261-272 in Recent Advances in the Chronobiology of Allergy and Immunology, M.H. Smolensky, A. Reinberg, and J.P. McGovern, eds. Oxford: Pergamon Press.
Lundeen, W., G.Y. Nicolau, D.J. Lakatua, L. Sackett-Lundeen, E. Petrescu, and E. Haus. 1990. Circadian periodicity of performance in athletic students. Progr. Clin. Biol. Res. 341B:337-343.
Mackiewicz, A., A. Kof, and P.B. Sehgal, eds. 1995. Interleukin-6-type cytokines. New York: New York Academy of Science. Pp. 1-522.
Maestroni, G.J.M. 1993. The immunoneuroendocrine role of melatonin. J. Pineal Res. 14:1-10. 1995. T-helper-2 lymphocytes as a peripheral target of melatonin. J. Pineal Res. 18:84-89.
Maestroni, G.J.M., and A. Conti. 1993. Melatonin in relation with the immune system. Pp. 229-311 in Melatonin: Biosynthesis, Physiological Effects, and Clinical Applications, Y. Hing-Su and R.J. Reiter, eds. Boca Raton, Fla.: CRC Press.
Maestroni, G.J.M., and W. Pierpaoli. 1981. Pharmacological control of the hormonally mediated immune-response. Pp. 405-425 in Psychoneuroimmunology, R. Ader, ed. New York: Academic Press.
Maestroni, G.J.M., A. Conti, and W. Pierpaoli. 1986. Role of the pineal gland in immunity. Circadian synthesis and release of melatonin modulates the antibody response and antagonizes the immunosuppressive effect of corticosterone. J. Neuroimmunol. 13:19-30.
Maestroni, G.J.M., A. Conti, and W. Pierpaoli. 1987a. The pineal gland and the circadian opiatergic immunoregulatory role of melatonin. Ann. N.Y. Acad. Sci. 496:67-77.
Maestroni, G.J.M., A. Conti, and W. Pierpaoli. 1987b. Role of the pineal gland in immunity. II. Melatonin enhances the antibody response via an opiatergic mechanism. Clin. Exp. Immunol. 68:384-391.
Maestroni, G.J.M., A. Conti, and W. Pierpaoli. 1988. Pineal melatonin: Its fundamental immunoregulatory role in aging and cancer. Ann. N.Y. Acad. Sci. 521:140-148.
Maini, R.N., M. Elliott, F.M. Brennan, R.O. Williams, and M. Feldmann. 1994. Targeting TNF-α for the therapy of rheumatoid arthritis. Clin. Exp. Rheumatol. 12(11):S63-S66.
Many, A., and R.S. Schwartz. 1971. Periodicity during recovery of the immune response after cyclophosphamide treatment. Blood 37:692-695.
Martini, E., J.Y. Muller, C. Doinel, C. Gastal, H. Roquin, L. Douay, and C. Salmon. 1988a. Disappearance of CD4 lymphocyte circadian cycles in HIV-infected patients: Early even during asymptomatic infection. AIDS 2:133-134.
Martini, E., J.Y. Muller, C. Gastal, C. Doinel, M.C. Meyohas, H. Roquin, J. Frottier, and C. Salmon. 1988b. Early anomalies of CD4 and CD20 lymphocyte cycles in human immunodeficiency virus. Presse Med. 17:2167-2168.
Masek, K., and O. Kadlec. 1983. Sleep factor, muramyl peptides, and the serotoninergic system [letter]. Lancet 2(8336):1277.
Mattson, R., I. Hannsson, and R. Holmdah. 1994. Pineal gland in autoimmunity: Melatonindependent exaggeration of collagen-induced arthritis in mice. Autoimmunity 17:83-86.
Menzio, P., B. Morra, A. Sartoris, R. Molino, M. Bussi, and G. Cortesina. 1980. Nasal secretory IgA circadian rhythm: A single-dose suppression. Ann. Otol. 89:173-175.
Mercola, K.E., M.J. Cline, and D.W. Golde. 1981. Growth hormone stimulation of normal and leukemic human T-lymphocyte proliferation in vitro. Blood 58:337-340.
Milos, P., D. Morse, and J.W. Hastings. 1990. Circadian control over synthesis of many Gonyaulax proteins is at a translational level. Naturwissenschaften 77:87-89.
Moldofsky, H. 1994. Central nervous system and peripheral immune functions and the sleepwake system. J. Psychiatr. Neurosci. 19(5):368-374.
Moldofsky, H., F.A. Lue, J.R. Davidson, J. Jephthah-Ochola, K. Carayanniotis, and R. Gorczynski. 1989. The effect of 64 hours of wakefulness on immune functions and plasma cortisol in humans. Pp. 185-187 in Sleep '88, J. Horne, ed. New York: Gustav Fischer Verlag.
Moldofsky, H., F.A. Lue, J. Eisen, E. Keyston, and R.M. Gorczynski. 1986. The relationship of interleukin-1 and immune functions to sleep in humans. Psychosom. Med. 48:309-318.
Morley, A., E.A. King-Smith, and F.J. Stohlman. 1970. The oscillatory nature of hemopoiesis. Pp. 3-14 in Symposium on Hemopoietic Cellular Proliferation, F. Stohlman Jr., ed. New York: Grune and Stratton.
Mormont, M.C., R. von Roemeling, R.B. Sothern, J.S. Berestka, T.R. Langevin, M. Wick, and W.J.M. Hrushesky. 1988. Circadian rhythm and seasonal dependence in the toxicologic response of mice to epirubicin. Invest. New Drugs 6:273-283.
Morrey, M.K., J.A. McLachlan, Serkin C.D., and O. Bakouche. 1994. Activation of human monocytes by the pineal hormone melatonin. J. Immunol. 153:2671-2680.
Morse, D., P.M. Milos, E. Roux, and J.W. Hastings. 1989. Circadian regulation of bioluminescence in Gonyaulax involves translational control. Proc. Natl. Acad. Sci. USA 86:172-176.
Mortin, R., and G. Courchay. 1994. Pregnenolone and dehydroepiandrosterone as precursors of native 7-hydroxylated metabolites which increase the immune response in mice. J. Steroid Biochem. Mol. Biol. 50:91-100.
Mukaida, N., G.L. Gussella, T. Kasahara, Y. Ko, C.O. Zachariae, T. Kawai, and K. Matsushima. 1993. Molecular analysis of the inhibition of interleukin-8 production by dexamethasone in a human fibrosarcoma cell line. Immunology 75:674-679.
Murphy, W.J., H. Rui, and D.L. Longo. 1995. Effects of growth hormone and prolactin immune development and function. Life Sci. 57:1-14.
Myers, M.P., K. Wager-Smith, A. Rothenfluh-Hilfiker, and M.W. Young. 1996. Light-induced degradation of TIMELESS and entrainment of the Drosophila circadian clock. Science 271:1736-1740.
Nagy, E., I. Berczi, G.E. Wren, S.L. Asa, and K. Kovacs. 1983. Immunomodulation by bromocriptine. Immunopharmacology 6:231-243.
Naitoh, Y., J. Fukata, T. Tominaga, Y. Nakai, S. Tamai, K. Mori, and H. Imura. 1988. Interleukin-6 stimulates the secretion of adrenocorticotrophic hormone in conscious, freely moving rats. Biochem. Biophys. Res. Commun. 155:1459-1463.
Nakano, Y., T. Miura, I. Hara, H. Aono, N. Miyano, and K. Miyajima. 1982. The effect of shift work on cellular immune function. J. Hum. Ergol. 11(suppl.):131-137.
Nechaev, A., F. Halberg, A. Mittelman, and G.L. Tritsch. 1977. Circannual variation in human erythrocyte adenosine aminohydrolase . Chronobiologia 4:191-198.
Nelson, W., L. Scheving, and F. Halberg. 1975. Circadian rhythms in mice fed a single daily ''meal'' at different stages of LD 12:12 lighting regimen. J. Nutr. 105:171-184.
Nelson, W., Y.L. Tong, J.K. Lee, and F. Halberg. 1979. Methods for cosinor rhythmometry. Chronobiologia 6:305-323.
Nicolau, G.Y., and E. Haus. 1994. Chronobiology of the hypothalamic-pituitary-thyroid axis. Pp. 330-347 in Biologic Rhythms in Clinical and Laboratory Medicine, 2d ed., Y. Touitou and E. Haus, eds. Heidelberg: Springer-Verlag.
Nicolau, G.Y., D.J. Lakatua, L. Sackett-Lundeen, and E. Haus. 1984. Circadian and circannual rhythms of hormonal variables in elderly men and women. Chronobiol. Int. 1:301-319.
Nye, L., T.G. Merret, J. Landon, and R.J. White. 1975. A detailed investigation of circulating IgE levels in a normal population. Clin. Allergy 5(1):13-24.
O'Dorisio, M.S., C.L. Wood, and T.M. O'Dorisio. 1984. Vasoactive intestinal peptide and neuropeptide modulation of the immune response. J. Immunol. 135:792S-796S.
Ohlman, S., A. Lindholm, H. Hagglund, J. Sawe, and B.D. Kahan. 1993. On the intraindividual variability and chronobiology of cyclosporine pharmacokinetics in renal transplantation. Eur. J. Clin. Pharmacol. 44:265-269.
Olsen, N.J., and W.J. Kovacs. 1996. Steroids and immunity. Endocr. Rev. 17:369-384.
Opp, M.R., H. Kapas, and L.A. Toth. 1992. Cytokine involvement in the regulation of sleep. Proc Soc Exp Biol Med. 201:16-27.
Opstad, P.K. 1991. Alterations in the morning plasma levels of hormones and the endocrine responses to bicycle exercise during prolonged strain. The significance of energy and sleep deprivation. Acta Endocrinol. (Cophenh.) 125:14-22.
Opstad, P.K. 1992. Androgenic hormones during prolonged physical stress, sleep, and energy deficiency . J. Clin. Endocrinol. Metab. 74:1176-1183.
Opstad, P.K. 1994. Circadian rhythm of hormones is extinguished during prolonged physical stress, sleep, and energy deficiency in young men. Eur. J. Endocrinol. 131:56-66.
Palestine, A.G., C.G. Muellenberg-Coulombre, M.K. Kim, M.C. Gelato, and R.B. Nussenblatt. 1987. Bromocriptine and low dose cyclosporine in the treatment of experimental autoimmune uveitis in the rat. J. Clin. Invest. 79:1078-1081.
Palmblad, J., K. Cantell, H. Strander, J. Froberg, C.G. Karlsson, L. Levi, M. Granstom, and P. Unger. 1976. Stressor exposure and immunological response in man: Interferon-producing capacity and phagocytosis. J. Psychosom. Res. 20:193-199.
Palmblad, J., B. Petrini, J. Wasserman, and T. Akerstedt. 1979. Lymphocyte and granulocyte reactions during sleep deprivation. Psychosom. Med. 41:273-278.
Pangerl, A., J. Remien, and E. Haen. 1986. The number of β-adrenoceptor sites on intact human lymphocytes depends on time of day, on season, and on sex . Ann. Rev. Chronopharmacol. 3:331-334.
Pappenheimer, J.R. 1983. Induction of sleep by muramyl peptides. J. Physiol. 335:1-2.
Pati, A.K., I. Florentin, V. Chung, M. DeSousa, F. Levi, and G. Mathe. 1987. Circannual rhythm in natural killer activity and mitogen responsiveness of murine splenocytes. Cell. Immunol. 108:227-234.
Peleg, L., M.N. Nesbitt, and I.E. Ashkenazi. 1989. Strain dependent response of circadian rhythms during exposure to continuous illumination. Life Sci. 44:893-900.
Petrovsky, N., P. McNair, and L.C. Harrison. 1994. Circadian rhythmicity of interferon-γ production in antigen-stimulated whole blood. Chronobiologia 21:293-300.
Pöllman, L., and B. Pöllman. 1988. Variations of the efficiency of hepatitis B vaccination. Annual Rev. Chronopharmacol. 5:45-48.
Portaluppi, F., P. Cortelli, P. Avoni, and L. Vergnani. 1994. Progressive disruption of the circadian rhythm of melatonin in fatal familial insomnia. J. Clin. Endocrinol. Metab. 78(5):1075-1078.
Pownall, R., and M.S. Knapp. 1980. Immune responses have rhythms. Are they important? Immunol. Today 1:7-10.
Pownall, R., P.A. Kabler, and M.S. Knapp. 1979. The time of day of antigen encounter influences the magnitude of the immune response. Clin. Exp. Immunol. 36:347-354.
Provinciali, M., G. DiStefano, and N. Fabris. 1992. Improvement in the proliferative capacity and natural killer cell activity of murine spleen lymphocytes by thyrotropin. J. Immunopharmacol. 14:865-870.
Raine, C.S., V. Traugott, and S.H. Stone. 1978. Suppression of chronic allergic encephalomyelitis: Relevance to multiple sclerosis. Science 201:445-448.
Rankin, E.C., E.H. Choy, D. Kassimos, and G.H. Kingsley. 1995. The therapeutic effects of an engineered human anti-tumor necrosis factor-α antibody (CDP571) in rheumatoid arthritis. Br. J. Rheumatol. 34(4):334-342.
Ratté, J., F. Halberg, and J.F.W. Kuhl. 1973. Circadian variation in the rejection of rat kidney allografts. Surgery 73:102-108.
Reinberg, A. 1967. The hours of changing responsiveness or susceptibility. Perspect. Biol. Med. 11:111-128.
Reinberg, A. 1989. Chronopharmacology of corticosteroids and ACTH. Pp. 137-167 in Chronopharmacology, Cellular, and Biochemical Interactions, B. Lemmer, ed. New York: Marcel Dekker.
Reinberg, A., and E. Sidi. 1966. Circadian changes in the inhibitory effects of an antihistaminic drug in man. J. Invest. Dermatol. 46:415-419.
Reinberg, A., and M.H. Smolensky. 1994. Night and Shift Work and Transmeridian and Space Flights. Pp. 243-255 in Biologic Rhythms in Clinical and Laboratory Medicine, 2d ed., Y. Touitou and E. Haus, eds. Heidelberg: Springer-Verlag.
Reinberg, A., P. Gervais, M. Morin, and C. Abulker. 1971. Human circadian rhythm in threshold of the bronchial response to acetylcholine. C.R. Acad. Sci. Hebd. Seances Acad. Sci. D. 272:1879-1881.
Reinberg, A., J. Ghata, and E. Sidi. 1963. Nocturnal asthma attacks: Their relationship to the circadian adrenal cycle. J. Allerg. 34:323-330.
Reinberg A., G. Labreque, and M.H. Smolensky, eds. 1991. Chronobiologie et Chronotherapeutique. Heme Optimale d'Administration des Medicamcuts. Paris: Flamarion-Medecine-Sciences.
Reinberg, A., E. Schuller, J. Clench, and M.H. Smolensky. 1979. Circadian and circannual rhythms of leukocytes, proteins, and immunoglobulins. Pp. 251-259 in Recent Advances in the Chronobiology of Allergy and Immunology, M.H. Smolensky, A. Reinberg, and J.P. McGovern, eds. Oxford: Pergamon Press.
Reinberg, A., E. Sidi, and J. Ghata. 1965. Circadian reactivity rhythms of human skin to histamine or allergen and the adrenal cycle. J. Allerg. 36:273-283.
Reinberg, A., M.H. Smolensky, G.E. D'Alonzo, and J.P. McGovern. 1988a. Chronobiology and asthma. III. Timing corticotherapy to biological rhythms to optimize treatment goals. J. Asthma 25:219-248.
Reinberg, A., Y. Touitou, M. Botbol, P. Gervais, D. Chaouat, F. Levi, and A. Bicakova-Rocher. 1988b. Oral morning dosing of corticosteroids in long term treated cortico-dependent asthmatics: Increased tolerance and preservation of the adrenocortical function. Ann. Rev. Chronopharmacol. 5:209-212.
Reinberg, A., Y. Touitou, A. Restoin, C. Migraine, F. Levi, and H. Montagner. 1985. The genetic background of circadian and ultradian rhythm patterns of 17-hydroxycorticosteroids: A cross twin study. J. Endocrinol. 105:247-253.
Rensing L. 1997. Genetics and molecular biology of circadian clocks. Pp. 55-77 in Physiology and Pharmacology of Biologic Rhythms. Handbook of Experimental Pharmacology, Volume 125, P.H. Redfern and B. Lemmer, eds. Heidelberg: Springer-Verlag.
Richtsmeier, W.S. 1985. Interferon. Present and future prospects. CRC Crit. Rev. Clin. Lab. Sci. 20:57-93.
Ritchie, A.W.S., I. Oswald, H.S. Micklem, J.E. Boyd, R.A. Elton, E. Jazwinska, and K. James. 1983. Circadian variation of lymphocyte subpopulations: A study with monoclonal antibodies. Br. Med. J. 286:1773-1775.
Rivard, G.E., C. Infante-Rivard, M.F. Dresse, J.M. Leclerc, and J. Champagne. 1993. Circadian time-dependent response of childhood lymphoblastic leukemia to chemotherapy: A long-term follow-up study of survival. Chronobiol. Int. 10:201-204.
Rivier, C., and S. Rivest. 1993. Mechanisms mediating the effects of cytokines on neuroendocrine functions in the rat. Ciba Found. Symp. 172:204-220.
Romball, C.G., and W.O. Weigle. 1973. A cyclical appearance of antibody producing cells after a single injection of serum protein antigen. J. Exp. Med. 138:1426-1442.
Romball, C.G., and W.O. Weigle. 1976. Modulation of regulatory mechanisms operative in the cyclical production of antibody. J. Exp. Med. 143:497-510.
Rosenblatt, L.S., M. Shifrine, N.W. Hetherington, T. Paglierioni, and M.R. Mackenzie. 1982. A circannual rhythm in rubella antibody titers. J. Interdiscipl. Cycle Res. 13:81-88.
Russell, D.H., K.T. Mills, F.J. Talamantes, and H.A. Bern. 1988. Neonatal administration of prolactin antiserum alters the development pattern of T- and B-lymphocytes in the thymus and spleen in Balb/C female mice. Proc. Natl. Acad. Sci. USA 85:7404-7407.
Russo, M.F. 1992. Macrophages and the glucocorticoids. J. Neuroimmunol. 40:281-286.
Sabate, I., J.M. Grino, A.M. Castelao, B. Arranz, C. Gonzalez, E. Guillin, C. Diaz, J. Huguet, and S. Gracia. 1990. Diurnal variations of cyclosporine and metabolites in renal transplant patients. Transplant. Proc. 22:1700-1701.
Scheving, L.E., E. Burns, J.E. Pauly, S. Tsai, and F. Halberg. 1976. Meal scheduling, cellular rhythms, and the chronotherapy of cancer [abstract]. Chronobiologia 3:80.
Scheving, L.E., E.L. Kanabrocki, F. Halberg, and J.E. Pauly. 1977. Circadian variations in total and electrophoretically fractioned serum proteins of presumably healthy man. Pp. 204-214 in Chronobiology in Allergy and Immunology, J.P. McGovern, M.H. Smolensky, and A. Reinberg, eds. Springfield, Ill.: Charles C Thomas.
Scheving, L.E., J.E. Pauly, and T. Tsai. 1968. Circadian fluctuation in plasma proteins of the rat. Am. J. Physiol. 215:1096-1101.
Schuurs, A.H., and H.A. Verheul. 1990. Effects of gender and sex steroids on the immune system. J. Steroid Biochem. 25:157-172.
Schwab, R., C.A. Waiters, and M.E. Weksler. 1989. Host defense mechanisms and aging. Semin. Oncol. 16:20-27.
Schweiger, H.G., R. Hartwig, and M. Schweigher. 1986. Cellular aspects of circadian rhythms. J. Cell Sci. 4(suppl.):181-200.
Senie, R.T., P.P. Rosen, P. Rhodes, and M.L. Lesser. 1991. Timing of breast cancer excision during the menstrual cycle influences duration of disease-free survival. Ann. Intern. Med. 115:337-342.
Sensi, S., E. Haus, G.Y. Nicolau, F. Halberg, D.J. Lakatua, A. DelPonte, and M.T. Guagnano. 1984. Circannual variation of insulin secretion in clinically healthy subjects in Italy, Romania, and the U.S.A. Riv. Ital. Biol. Med. 4:1-8.
Shahal, B., F.A. Lue, C.G. Jiang, A. MacLean, and H. Moldofsky. 1992. Circadian and sleepwake related changes in immune functions. J. Sleep Res. 1(suppl.):210.
Sharp, G.W.G. 1960. Reversal of diurnal leukocyte variations in man. J. Endocrinol. 21:107-114.
Shaw, L.M., B. Kaplan, and D. Kaufman. 1996. Toxic effects of immunosuppressive drugs: Mechanisms and strategies of controlling them. Clin. Chem. 42:1316-1321.
Shephard, R.J., S. Rhind, and P.N. Shek. 1995. The impact of exercise on the immune system: NK cells, interleukins-1 and -2, and related responses. Exerc. Sport Sci. Rev. 23:215-241.
Shifrine, M., A. Garsd, and L.S. Rosenblatt. 1982a. Seasonal variation in immunity of humans. J. Interdiscipl. Cycle Res. 12:157-165.
Shifrine, M., L.S. Rosenblatt, N. Taylor, N.W. Hetherington, F.J. Mathews, and F.D. Wilson. 1982b. Seasonal variations in lectin-induced lymphocyte transformation in Beagle dogs. J. Interdiscipl. Cycle Res. 13:151-156.
Shifrine, M., N. Taylor, L.S. Rosenblatt, and F. Wilson. 1980. Seasonal variation in cell-mediated immunity of clinically normal dogs. Exp. Hematol. 8:318-326.
Shoham, S., D. Davenne, A.B. Cady, C.A. Dinarello, and J.M. Krueger. 1987. Recombinant tumor necrosis factor and interleukin-1 enhance slow wave sleep. Am. J. Physiol. 253:R142-R149.
Sitar, J. 1991. Correlation of some parameters of solar wind and sudden cardiovascular deaths. Cas Lek Cesk. 130:44-47.
Slozina, N.M., and G.D. Golovachev. 1986. The frequency of sister chromatin exchanges in human lymphocytes determined at different times within 24 hours . Citologia 28:127-129.
Smith, E.M. 1992. Hormonal activities of cytokines. Chem. Immunol. 52:154-169.
Smith, E.M., W.J. Meyer, and J.E. Blalock. 1982. Virus-induced increases in corticosterone in hypophysectomized mice: A possible lymphoid adrenal axis. Science 218:1311-1313.
Smith, E.M., A.C. Morrill, W.J.I. Meyer, and J.E. Blalock. 1986. Corticotropin-releasing factor induction of lymphocyte-derived immunoreactive ACTH and endorphins. Nature 321:881-882.
Smolensky, M.H., and G.E.D. D'Alonzo. 1994. Nocturnal asthma: mechanisms and chronotherapy. Pp. 453-469 in Biologic Rhythms in Clinical and Laboratory Medicine, 2d ed., Y. Touitou and E. Haus, eds. New York: Springer-Verlag.
Smolensky, M.H., and G.E.D. D'Alonzo. 1997. Progress in the chronotherapy of nocturnal asthma. Pp. 205-250 in Physiology and Pharmacology of Biologic Rhthyms. Handbook of Experimental Pharmacology, Volume 125, P.H. Redfern and B. Lemmer, eds. Heidelberg: Springer-Verlag.
Smolensky, M.H., P.J. Barnes, A. Reinberg, and J.P. McGovern. 1986a. Chronobiology and asthma. I. Day-night differences in bronchial patency and dyspnea and circadian rhythm dependencies. J. Asthma 23:321-343.
Smolensky, M.H., G.E. D'Alonzo, G. Kunkel, and P.J. Barnes. 1987. Circadian rhythmadapted theophylline chronotherapy for nocturnal asthma. Chronobiol. Int. 4:301-466.
Smolensky, M.H., A. Reinberg, and J.T. Queng. 1981. The chronobiology and chronopharmacology of allergy. Ann. Allergy 47:237-252.
Smolensky, M.H., P.H. Scott, P.J. Barnes, and J.H.G. Jonkman. 1986b. The chronopharmacology and chronotherapy of asthma. Ann. Rev. Chronopharmacol. 2:229-273.
Soiffer, R.J., C. Murray, C. Shapiro, H. Collins, S. Chartier, S. Lazo, and J. Ritz. 1996. Expansion and manipulation of natural killer cells in patients with metastatic cancer by low-dose continuous infusion and intermittent bolus administration of interleukin-2. Clin. Cancer Res. 2:493-499.
Song, J.G., S. Nakano, S. Ohdo, and N. Ogawa. 1993. Chronotoxicity and chronopharmacokinetics of methotrexate in mice: Modification of feeding schedule. Japan J. Pharmacol. 62:373-378.
Sothern, R.B., B. Roitman-Johnson, E.L. Kanabrocki, J.G. Yager, M.M. Roodell, J.A. Weatherbee, M.R. Young, B.M. Nemchausky, and L.E. Scheving. 1995. Circadian characteristics of circulating interleukin-6 in men. J. Allergy Clin. Immunol. 95:1029-1035.
Souba, W.W. 1994. Cytokine control of nutrition and metabolism in critical illness. Curr. Probl. Surg. 31(7):577-652.
Spangelo, B.L., and W.C. Gorospe. 1995. Role of the cytokines in the neuroendocrine immune system axis. Front. Neuroendocrinol. 16:1-22.
Stimpfling, J.H., and A. Richardson. 1967. Periodic variations of the hemagglutinin response in mice following immunization against sheep red blood cells and alloantigens. Transplantation 5:1496-1503.
Stimson, W.H. 1988. Oestrogen and human T-lymphocytes: Presence of specific receptors in the T-suppressor cytotoxic subset. Scand. J. Immunol. 28:345-350.
Suteanu, S., E. Haus, L. Dumitriu, G.Y. Nicolau, E. Petrescu, H. Berg, L. Sackett-Lundeen, I. Ionescu, R. Reilly. 1995. Circadian rhythm of pro- and anti-inflammatory factors in patients with rheumatoid arthritis-#226 [abstract]. Biological Rhythm Research 26:(4)446.
Swoyer, J., P. Irvine, L. Sackett-Lundeen, L. Conlin, D.J. Lakatua, and E. Haus. 1989. Circadian hematologic time structure in the elderly. Chronobiol. Int. 6:131-137.
Swoyer, J., F. Rhame, W. Hrushesky, L. Sackett-Lundeen, R. Sothern, H. Gale, and E. Haus. 1990. Circadian rhythm alterations in HIV-infected patients. Pp. 437-449 in Chronobiology: Its Role in Clinical Medicine, General Biology, and Agriculture, Proceedings of the XIX International Conference of the International Society for Chronobiology, Bethesda, Md., June 20-24, 1989. New York: Wiley-Liss, Inc.
Swoyer, J., L.L. Sackett, E. Haus, D.J. Lakatua, and L. Taddeini. 1975. Circadian lymphocytic rhythms in clinically healthy subjects and in patients with hematologic malignancies [abstract]. Pp. 62-63 in International Congress on Rhythmic Functions in Biological System, Vienna: Egerman.
Tarquini, B. 1980. Physiopathology of peptic ulcer: A new view. Rass. di Medicina Sperimentale 27(5):279.
Tavadia, H.B., K.A. Fleming, P.D. Hume, and H.W. Simpson. 1975. Circadian rhythmicity of human plasma cortisol and PHA-induced lymphocyte transformation. Clin. Exp. Immunol. 22:190-193.
Tobler, I., A.A. Borbely, M. Schwyzer, and A. Fontana. 1984. Interleukin-1 derived from astrocytes enhances slow wave activity in sleep EEG of rat. Eur. J. Pharmacol. 104:191-192.
Touitou, Y., A. Carayon, A. Reinberg, A. Bogdan, and H. Beck. 1983. Differences in the seasonal rhythmicity of plasma prolactin in elderly subjects. Detection in women but not in men. J. Endocrinol. 96:65-71.
Touitou, Y., and E. Haus. 1994. Aging of the human endocrine and neuroendocrine time structure. Ann. N.Y. Acad. Sci. 719:378-397.
Tritsch, G.L., A. Nechaev, and A. Mittelman. 1975. Adenosine aminohydrolase as an indicator of lymphocyte-mediated cytolysis: Possible role of cyclic adenosine monophosphate. Clin. Chem. 21:984.
Turner-Warwick, M. 1988. Epidemiology of nocturnal asthma. Am. J. Med. 85(suppl. 1B):6-8.
Uezono, K., L. Sackett-Lundeen, T. Kawasaki, T. Omae, and E. Haus. 1987. Circaseptan rhythm in sodium and potassium excretion in salt sensitive and salt resistant Dahl rats. Pp. 297-307 in Advances in Chronobiology, Part A, J.E. Pauly and L.E. Scheving, eds. New York: Alan R. Liss, Inc.
Valtora, A., A. Moretta, R. Maccario, M. Bozzola, and F. Severi. 1991. Influence of growth hormone-releasing hormone (GHRH) on phytohemagglutinin-induced lymphocyte activation: comparison of two synthetic forms. Thymus 18:51-59.
Van Cauter, E., and F.W. Turek. 1995. Endocrine and other biologic rhythms. Pp. 2487-2548 in Endocrinology, Degroot, L.J. ed. Philadelphia: W.B. Saunders Company.
Varma, S., P. Sabharwal, J.F. Sheridan, and W.B. Malarkey. 1993. Growth hormone secretion by human peripheral blood mononuclear cells detected by an enzyme-linked immunoplaque assay. J. Clin. Endocrinol. Metab. 76:49-53.
Venkataramanan, R., S. Yang, G.J. Burckard, R.J. Ptachcinski, D.H. Van Thiel, and T.E. Starzl. 1986. Diurnal variation in cyclosporine pharmacokinetics. Ther. Drug Monit. 8:380-381.
Walker, A.M. 1994. Phosphorylated and non-phosphorylated prolactin isoforms. Endocrinol. Metab. 5:195-200.
Weigent, D.A., and J.E. Blalock. 1995. Associations between the neuroendocrine and immune systems. J. Leukocyte Biol. 58:137-150.
Weigle, W.O. 1975. Cyclic production of antibody as a regulatory mechanism in the immune response. Adv. Immunol. 21:87-111.
Wendling, D., E. Racadot, and J. Wijdeness. 1993. Treatment of severe rheumatoid arthritis by anti-interleukin-6 monoclonal antibody. J. Rheumatol. 20(2):259-262.
Westaway, J., C.M. Atkinson, T. Davies, S.A. Petersen, and M.P. Wailoo. 1995. Urinary excretion of cortisol after immunization. Arch. Dis. Child. 72:432-434.
Wilder, R.L. 1995. Neuroendocrine-immune system interactions and autoimmunity. Ann. Rev. Immunol. 13:307-338.
Williams, R.M., L.J. Kraus, D.P. Dubey, E.J. Yunis, and F. Halberg. 1979. Circadian bioperiodicity in natural killer cell activity of human blood [abstract]. Chronobiologia 6:172.
Winget, C.M., M.R.I. Soliman, D.C. Holley, and J.S. Meylor. 1994. Chronobiology of Physical Performance and Sports Medicine. Pp. 230-242 in Biologic Rhythms in Clinical and Laboratory Medicine, 2d ed., Y. Touitou and E. Haus, eds. Heidelberg: Springer-Verlag.
Withyachumnarnkul, B., K.O. Nonaka, C. Santana, A.M. Attia, and R.J. Reiter. 1990. Interferon-γ modulates melatonin production in rat pineal gland in organ culture. J. Interferon Res. 10:403-411.
Wood, P.A., and W.J.M. Hrushesky. 1994. Chronopharmacodynamics of hematopoietic growth factors and antitumor cytokines. Pp. 185-207 in Circadian Cancer Therapy, W.J.M. Hrushesky, ed. Boca Raton, Fla.: CRC Press.
Yehuda, S., B. Shredny, and Y. Kalechman. 1987. Effects of DSIP, 5-HTP, and serotonin on the lymphokine system: A preliminary study. Int. J. Neurosci. 33:185-187.
Young, M.R.I., J.P. Matthews, E.L. Kanabrocki, R.B. Sothern, B. Roitman-Johnson, and L.E. Scheving. 1995. Circadian rhythmometry of serum interleukin-2, interleukin-10, tumor necrosis factor-α, and granulocyte-macrophage colony stimulating factor in men. Chronobiol. Int. 12:19-27.
Young, M.W., ed. 1993. Molecular Genetics of Biological Rhythms. New York: Dekker.
Young, M.W., F.R. Jackson, H.S. Shin, and T.A. Bargiello. 1985. A biological clock in Drosophila. Cold Spring Harbor Symp. Quant. Biol. 50:865-875.
Zabel, P., H. Horst, C. Kreiber, and M. Schlaak. 1990. Circadian rhythm of interleukin-1 production of monocytes and the influence of endogenous and exogenous glucocorticoids in man. Klin Wochenschr. 68:1217-1221.
Zabel, P., K. Linnemann, and M. Schlaak. 1993. Circadian rhythm in cytokines. Immun. Infekt. 20:38-40.
Zimmer, J., A.S. Khalifa, and J.J. Lightbody. 1975. Decreased lymphocyte adenosine deaminase activity in acute lymphocytic leukemic children and their parents. Cancer 35(1):68-70.