PUBLIC HEALTH
Fifty years ago, when diseases such as polio and pneumonia took many lives, only a handful of today's high-performance pharmaceuticals existed. Through collaboration with biologists and clinicians, chemists and chemical engineers have helped change the course of medical history in the intervening years. Vaccines, antibiotics, and other pharmaceuticals can now save and prolong lives or improve the quality of life for those who suffer from illnesses. Today, more than ever, continuing advances
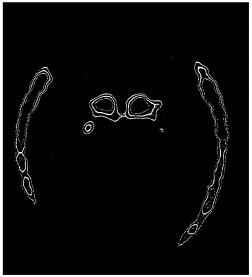
in research are helping to reduce and eradicate disease. For example, ivermectin is successfully used to treat and prevent river blindness, which affects or threatens 100 million people in some of the poorest areas of the world. Other spectacular success stories include the discovery of antiulcer drugs such as cimetidine and ranitidine, which not only reduce ulcer-related pain and morbidity but also decrease the need for expensive hospitalization.
The challenges to biomedical science in the last decade of the twentieth century are imposing; they include autoimmune diseases, mental illness, cancer, geriatric disorders, diabetes, and viral infections. In each case, major input must be obtained from chemists and chemical engineers if we are to meet these challenges. No current challenge is greater than that of AIDS, against which a concerted, multipronged attack is under way. The chemical synthesis of the life-extending drug AZT was an important first step in this battle.
Prostheses and Biomaterials: Medical Devices
Most likely, someone in your family has used a man-made device for the treatment of some medical condition: perhaps an inner ear implant, an artificial hip, or maybe just contact lenses. The development of synthetic replacements for joints, ligaments, lenses, and teeth is certain to become more important as the percentage of older people in our society increases. Battery-driven cardiac pacemakers now provide rhythm for defective biological control systems, other heart-assist devices aid in the recovery of patients with hearts damaged by disease or infarction, and polymerbased replacement parts ranging from small blood vessels to artificial hearts are on the horizon.
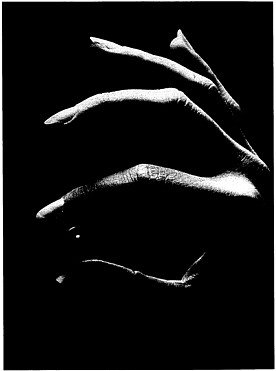
Contact lens technology, including the new, safer materials used in soft and extended-wear lenses, is the result of extensive research and development efforts by chemists and chemical engineers.
Hybrids of biological and man-made systems are also being explored. One example is the use of implanted
electrodes that electrochemically stimulate nerve cells and provide function for patients with spinal, vision, or hearing injuries. External treatments with novel materials and devices include kidney dialysis machines, support systems for open-heart surgery, and membrane blood oxygenators that can keep premature babies alive.
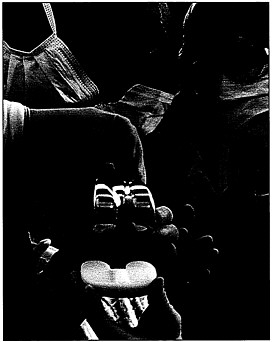
The synthetic materials developed for this total knee replacement system provide for an enhanced bond between the implant and bone cement used to hold it in place.
The design and synthesis of materials for use in the human body present major research challenges to chemists and chemical engineers. Successful use of these medical devices rests on understanding and controlling the way that man-made materials interact with the body. Today, the plastics and metals in such surgical implants as bone plates, stents (devices that compress plaque deposits against the walls of constricted arteries to result in improved blood flow), and drug infusion devices are almost never biodegradable. Over time, the inevitable tissue reaction to these materials can cause pain to the patient and failure of the device, requiring surgery to remove the device. Biodegradable materials could significantly reduce hospitalization time after surgery and the associated health care costs.
At the present time, organ transplants provide the only way to cure serious organ deficiency diseases, but the shortage of donor organs is overwhelming. For example, in the case of liver disease, donor organs are available for only a few percent of the 30,000 patients who suffer liver failure annually; for most liver-failure patients the prognosis is death. Scientists are trying to culture large numbers of cells supported on specially designed polymers, which must be nontoxic to cells and compatible with human tissue. If this line of research is successful, disease-free cells could be removed from a healthy subject, cultured externally, and then implanted into the patient.
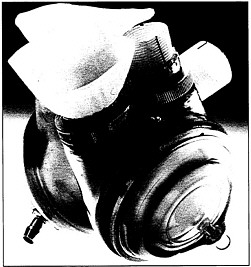
Designed as a temporary measure until a suitable organ can be located, this artificial heart has a tough outer case made from a solid block of polysulfone, a high-strength bio-compatible plastic with a unique ability to withstand repeated sterilizations at temperatures in excess of 270 degrees Fahrenheit.
Medical Diagnostics
The diagnosis of human disease increasingly relies on chemical analysis of extremely small specimens taken from the patient, a trend that ultimately will allow a physician to customize therapy to the patient 's needs. The technology for such diagnostic testing requires advances at the chemistry-biology interface, as illustrated by the antigen-antibody relationship. The binding of an antibody to a foreign substance is usually accompanied by a change in the antibody's molecular shape, which can be detected by chemical reporter systems. By precisely correlating the quantity of a given antigen with small, electronically measurable changes in the antibody's absorption of light, it would become possible to identify and measure minuscule amounts of antigens in a patient's bloodstream.
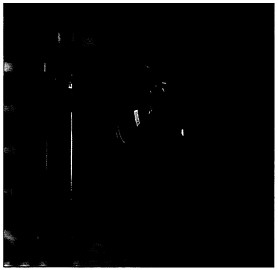
Phenomena such as fluorescence are used to study the structure and function of biological molecules. Here a scientist examines a peptide solution that exhibits red fluorescence when illuminated with light of a shorter wavelength.
With an eye toward developing new, noninvasive diagnostic systems that do not require, for instance, the drawing of blood, chemical engineers are trying to enhance the transport of molecules through tissues, using chemicals, ultrasound, or electricity. Someday, it may be possible to measure glucose or cholesterol by simply placing an adhesive patch on the patient's skin.
Chemical Synthesis of Drugs
Many of the drugs in current use were discovered through the isolation of pharmacologically active substances from natural sources, usually plants, marine organisms, or soil microorganisms. Some of these substances were known from folk medicine, and others have been found through pharmaceutical screening of a wide range of naturally occurring compounds. Once a promising lead is identified, chemists must design and synthesize novel molecules that have similar molecular structures but are safer, more effective, or both. The process increasingly relies on chemical insights achieved by using a series of powerful new tools that have evolved from basic research in chemistry: computer modeling of molecular structure, x-ray crystallography, and high-field nuclear magnetic resonance (NMR) spectroscopy.
The discovery of the dramatic effects of cortisone on patients incapacitated by rheumatoid arthritis provides one of the first examples of the importance
of chemical synthesis. Cortisone was first synthesized in 1946, but process research by chemists and later by chemical engineers was needed to make the 37-step conversion of cholic acid to cortisone practical on a commercial scale. They proved that esoteric reactions discovered in university laboratories could be reproduced effectively on a pilot-plant or even factory scale to manufacture molecules of great medical value.
During the mid-1950s, chemists synthesized the steroid 17-α-ethynyl-19-nortestosterone, which came to be of great importance because it is an orally effective birth control agent. That work serves as a persuasive reminder that chemistry can provide molecules with properties that profoundly affect not only medicine, but also societal behavior.
During the last 30 years the foundations of chemical synthesis have expanded markedly. In the 1960s, reliable approaches emerged for the synthesis of peptides—small fragments of proteins—but these methods were slow and cumbersome. In the 1970s and 1980s, chemists and chemical engineers developed automated methods that could synthesize grams of peptides in minutes. Three decades of research on the important hypothalamic hormones culminated in the isolation, characterization, and, finally, the synthesis of a series of peptide hormones during the 1980s. Human studies have shown that one of them, gonadoliberin, is useful in both the diagnosis and the treatment of infertility; analogs and antagonists of gonadoliberin may have both fertility and antifertility applications and are being used to treat prostate cancer.
Another important challenge in new drug synthesis is the design of genespecific DNA and RNA binding agents. For example, the target for many anticancer drugs is DNA, the genetic material itself, or the RNA that translates this genetic information into various processes of the living cell. Oligonucleotides, small nucleic acid molecules, offer novel opportunities for therapeutic intervention. The sequence of the naturally occurring nucleic acid carries the sense of the genetic message, but the synthetic oligonucleotide has an opposite sequence and is therefore described as “antisense.” An oligonucleotide with an antisense structure exactly complements a particular DNA or RNA sequence and can bind to it with high specificity. Such molecules are being explored in new treatments for disorders ranging from viral herpes infections to cancer and heart disease. The first step is synthesizing an antisense molecule that is complementary to the genetic sequence responsible for a disease state in a cell. Developing efficient methods to synthesize these molecules on a large scale will be essential to enabling new therapies that can be employed at acceptable costs. The next step is delivering the antisense molecule to the infected cell, where it can bind to the nucleic acid sequence of the RNA or DNA and inhibit the chemical processes that cause the illness. Delivering enough of the molecule to the cell also represents a significant chemical and engineering challenge.
Computer-aided Drug Design
As a rule, drugs are small molecules, consisting of only tens or a few hundreds of atoms, whereas their protein targets may have many thousands of atoms arranged in elegant and complex three-dimensional structures. The protein's structure enables the drug to bind to it in a highly specific manner, inducing changes in the chemical properties of the protein. Because a successful drug must be able to fit comfortably into the protein's binding site, designing a new drug is facilitated by visualizing that site. Chemical research over the last decade has shown that neither the protein nor the drug has a single, unique shape. Instead they undergo a variety of breathing and flexing motions over time. As a result, correlation of the optimal three-dimensional structures of the protein and of the potential drug with the desired biological activity is extremely complicated. Fortunately, the magnitude of this problem, although overwhelming to a human, is ideally suited to the latest generation of supercomputers.
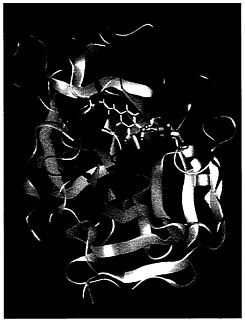
Computer graphics depict an antiemphysema drug candidate bound to the human leukocyte elastase enzyme. The large elastase molecule is shown in yellow, green, and purple; the small drug candidate is shown as the red-and-white stick figure embedded in the elastase.
Today, computational chemistry and molecular modeling enable studies on complex systems that were unthinkable just a few years ago. Armed with the insights such studies can provide into the molecular features needed for a possible drug, research chemists can design candidates for preliminary evaluation on the computer. The most likely candidates can then be synthesized by and tested in real biological systems.
Genetic Engineering and Recombinant Human Proteins
Discoveries in basic science in the late 1960s and early 1970s made possible the development of the U.S. biotechnology industry. Methods were first discovered for isolating pieces of DNA that, when inserted into bacteria, caused them to produce biologically active proteins. Novel chemical methods were then developed for DNA sequencing that enabled active genes to be directly read and therefore more easily manipulated and chemically produced. The resulting recombinant-DNA technology offered the possibility of producing replacement human proteins for medical treatment, but even a
milligram, just one thousandth of a gram, was hard to prepare with existing laboratory techniques. Enter the chemical engineers with practical experience in fermentation and chemical process technologies, who quickly adapted procedures originally developed by the brewing, pharmaceutical, and chemical manufacturing industries.
What are the genetically engineered proteins that currently benefit human health? Human insulin is now used to treat diabetes, replacing the hormone from pigs or calves that sometimes induced unfavorable immunological responses. Human growth hormone is available for children whose own hormone production is deficient; human growth hormone also has potential as an anti-aging and antiobesity drug. These powerful hormones, normally made in minute amounts in the body, were previously difficult to obtain in sufficient quantity for essential chemical and medical research; current levels of availability permit their medical exploration in ways unimaginable a decade ago.
Recently, clot-dissolving proteins have come into widespread use in hospital emergency rooms to save the lives of heart attack victims. The genetically engineered form of clotting factor VIII is now used to treat bleeding in hemophiliacs, whose lives are threatened by otherwise minor injuries.
A side benefit of producing such proteins in bacteria is the avoidance of potential contamination by such infectious agents as the AIDS virus. Erythropoietin, the hormone that stimulates red blood cell development, has also been produced by genetic engineering; now used to treat kidney disease, it may also have applications for treating AIDS-associated anemia and blood loss in deep invasive surgery. Granulocyte-macrophage colony-stimulating factors are proteins with a number of possible therapeutic uses that include regenerating bone-marrow blood precursor cells in cancer patients undergoing radiotherapy, chemotherapy, or bone-marrow transplants, fighting life-threatening bacterial infections, and treating immuno-compromised AIDS patients.
Nearly all these recombinant proteins, and many more currently in the biopharmaceutical pipeline, owe their existence to the 30-year investment by the United States in the basic health-related sciences; a significant portion of this research was carried out at universities under government funding. The ideas, the technologies, and the trained scientists and engineers critical to the development of the biotechnology industry have had their genesis in university and government research laboratories across the country. The technology next moved into small biotechnology start-up companies and finally into large, multinational pharmaceutical companies. No other national effort in support of chemistry and medicine has achieved comparable success or more effectively channeled scientific discovery toward practical applications in human health care.
Gene Therapy
Chemists and biochemists are making key contributions to advances in the health sciences, including gene therapy. An underlying genetic component is present in many diseases, including not only the well-known examples of cystic fibrosis and muscular dystrophy, but also complex disorders such as cancer and Alzheimer's disease. If the chemical alterations in chromosome structure responsible for such diseases could be readily detected—and then corrected by direct DNA replacement therapy—many human diseases could be cured outright.
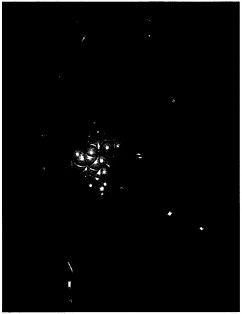
This computer-generated image shows a potential drug (yellow) binding to HIV protease, an enzyme (purple and red ribbons) encoded by the AIDS virus.
Through computational research studies of protein-drug interactions, chemists hope to learn what molecular features are needed for a drug that can bind to this enzyme and inhibit replication of the virus.
How could this be done at a practical level? Major advances by chemists and molecular biologists have produced DNA diagnostic technology, especially the polymerase chain reaction (PCR) method of amplifying small segments of DNA. PCR allows selective sequence amplification even in single DNA molecules, permitting convenient, rapid, inexpensive typing of the genetic makeup of any individual at a molecular level. If, then, an appropriate corrected or chemically modified segment of the gene sequence could be inserted into a patient's DNA, a cure for the genetic deficiency would result. Techniques for delivering and inserting genetic material for this purpose have been developed based on modifying the DNA of a viral vector that carries engineered human genes. Infection of the patient with such a modified virus vector provides a mechanism for curing the original disease. Clinical trials employing this technology are currently under way for a variety of genetic diseases ranging from cystic fibrosis to familial elevated cholesterol.
An alternate, possibly safer and more efficient method of gene delivery that circumvents the need for engineered viruses has recently been developed. It uses chemically designed complexes of receptor-binding proteins and human DNA that can target the human gene to be efficiently taken up by cells having the appropriate receptor.
AIDS, which has generated a major worldwide health crisis, is one focus of research on gene therapy. Recently, chemists, using the methods of protein crystallography and high-field NMR, have begun to obtain detailed structural information about the proteins encoded by the HIV virus that causes AIDS. This information has been used to attempt the rational computer-aided design of drugs able to inhibit these enzymes, obstruct the infectious process, and ameliorate the progressive loss of the immune defense system.
An alternative approach involves gene therapy based on engineering the immune system to recognize and then kill AIDS virus-infected cells that display HIV coat proteins on their cell surfaces. By engineering natural killer
cells (T-lymphocytes, one kind of white blood cell) to selectively kill HIV-infected lymphocytes early in the infective process, the infected cells could be eliminated while preserving the bulk of the T-lymphocytes and allowing the patient's immune response to remain normal. Both the gene therapy and rational drug design approaches are being explored by scientists in the biotechnology and pharmaceutical industries to find a more effective therapy for this terrible disease.
Drug Delivery Systems
Despite the many wonders that they work, modern drugs still have limitations. A drug may never reach its target organ; the blood-brain barrier, for instance, prevents many substances in the bloodstream from entering the brain. Or it may be difficult to achieve the necessary level of the drug in the blood. Because most drugs are metabolized by the body, a large dose may be needed to ensure that an effective concentration in the bloodstream remains long enough to have the desired action; however, large doses can result in serious side effects. Some drugs are metabolized very rapidly, lasting only a short time in the body. Such drugs must be given by infusion or in very frequent doses and thus can be nearly impossible to take.
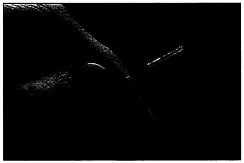
Certain medicinal agents produce a cytotoxic effect in cancer cells when exposed to a specific wavelength of laser light. This hand-held treatment fiber directs the light to the affected area, thus minimizing damage to healthy cells.
To address these problems, chemists and chemical engineers are designing new drug delivery systems. A pill has been engineered with a plastic coating in which a laser has made a single perforation; when the pill is swallowed, fluid flows through the coating, and the drug is pumped through the perforation at a precise and constant rate. Such a system is now being used to deliver a powerful heart medication, keeping the blood concentration at a low and steady level and avoiding side effects. Another improved oral system has a novel design for delivery of the antiasthma drug theophylline; microcapsules of the drug are embedded in a degradable plastic matrix that breaks down and releases the drug at a steady rate, again minimizing side effects.
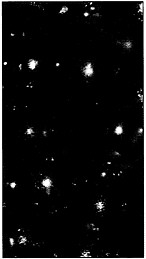
Biodegradable polymeric microspheres can release a drug into the bloodstream at a controlled rate, thus increasing the safety and efficacy of the drug. This research photograph shows microspheres containing an orange dye.
The transdermal patch has been introduced within the last few years. This system represents a novel engineering feat that combines five separate layers: a backing layer through which the drug cannot penetrate, the drug reservoir, a rate-controlling membrane, an adhesive layer that is eventually placed on the skin, and a peel strip cover that is
removed from the adhesive prior to application. The resulting ultrathin patch may be less than one hundredth of an inch thick. The drug contained in the patch slowly passes through the skin and into the bloodstream over periods ranging from a day to a week. This approach offers convenience and avoids oral administration, which can sometimes result in destruction of the drug by enzymes in the digestive system or liver. Transdermal patches are now being used for delivery of nitroglycerin to patients with angina, estradiol for postmenopausal disorders, medication for motion sickness, and nicotine to smokers who want to quit.
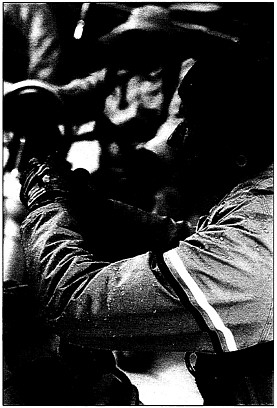
The fisherman in this scene is wearing a transdermal patch behind his ear that delivers a drug to prevent motion sickness.
For drugs such as peptides or proteins that are too big or do not have the right chemical properties to pass through the skin, chemists and chemical engineers have begun to develop new delivery systems. These larger molecules can almost never be taken orally and must be injected, but even then are often destroyed in just minutes by the body's metabolic processes. Implantable, degradable plastics permit the drug molecules to be slowly released over days or even years, providing a steady level of the drug in the body. Such long-acting delivery systems, now being used to treat prostate cancer and endometriosis, may become even more important in the future. Perhaps someday diabetics will take insulin shots once a month or once a year, thereby avoiding both the inconvenience and the risk of daily injections.
Once a drug is administered, it travels throughout the body, where it can harm normal cells and organs as well as diseased cells. The problem is particularly serious in cancer treatment, which uses powerful chemotherapeutic agents to destroy tumors. In a promising approach to tumor therapy, an anticancer drug is attached to an antibody that has an affinity for particular cancer cells.
Medical Imaging
The correct diagnosis of a patient's medical condition is the essential first step in prescribing a treatment. The rapidly growing technologies of diagnostic imaging provide direct information about the structure of bone, soft-tissue organs, and tumors. Virtually everyone has had diagnostic tests with x rays, and it is increasingly likely that you or a member of your family has benefited or will benefit from newer techniques that include magnetic resonance imaging (MRI), ultrasound, positron emission tomography (PET), single photon emission computed tomography (SPECT), and other nuclear medicine techniques. With MRI, PET, and SPECT, three-dimensional images can now be displayed on a computer screen with remarkably high resolution and detail. The information provided by these imaging technologies is now rapidly moving beyond structure to provide measures of biological function. PET can show levels of metabolism in various regions of the brain and determine blood flow through coronary arteries or the heart. MRI is beginning to be used in measuring blood flow in various regions of the brain.
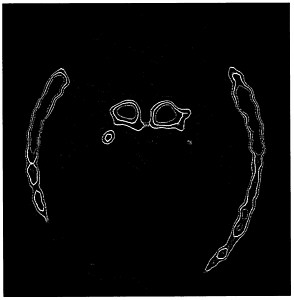
Positron emission tomography (PET) image of the brain of a patient with Parkinson's disease shows substantially reduced uptake of [18F]fluorodopamine in the central part of the brain (red areas).
These diagnostic techniques have evolved from the research results of chemists, engineers, physicists, biologists, and mathematicians. MRI is an outgrowth of work in NMR spectroscopy by chemists and has been aided by advances in magnet technology. PET usually uses radioisotopes of very short lifetime, requiring on-site preparation and subsequent incorporation into organic compounds by advanced synthesis techniques. Images obtained from MRI studies are also frequently enhanced by chemical contrast agents. For each of these technologies, advances in medical diagnosis and treatment will be predicated on further research in chemistry and the other basic sciences.
ANGIOTENSIN-CONVERTING ENZYME INHIBITORS
Angiotensin-converting enzyme (ACE) converts the biologically inactive peptide angiotensin I to angiotensin II, a highly active component in the complex biochemical system for regulating blood pressure. Some individuals develop imbalances in this system that lead to hypertension, an important medical issue that affects one in three adults at some time in their lives. The health care costs associated with hypertension were $13.7 billion in 1991.
Following the isolation of these two peptides in the United States, scientists in Brazil and Japan discovered a series of peptides in snake venoms that lowered blood pressure in people. These seemingly unrelated studies were linked when biochemists purified ACE and found that ACE is inhibited by these same snake-venom peptides—inhibitors of ACE apparently restore the balance to the system that regulates blood pressure. The stage was then set for the use of the snake-venom peptides as a starting point for the design and synthesis of clinically useful inhibitors of ACE. The development of these drugs has had an important influence on the treatment of both hypertension and chronic heart failure.
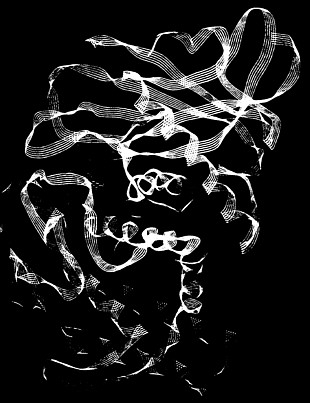
The ACE-inhibitor problem. Because the 3-dimensional structure of angiotensin-converting enzyme has not been fully determined, computational chemists have studied the related protein thermolysin. This computer-generated image depicts the binding of an inhibitor (red-orange) to thermolysin.
Scientists discovered that zinc is a key component in the ACE enzyme. This discovery allowed them to deduce molecular properties that could permit inhibition of the enzyme's activity. Combining this information with knowledge of the snake-venom inhibitors, chemists designed and synthesized the inhibitor captopril and demonstrated its ability to lower blood pressure in animals and in humans. Because occasional human side effects such as rashes and loss of taste were reminiscent of those from penicillamine, chemists suspected that the similarities resulted from specific sulfur atoms that are common to both molecules. A worldwide search began for a new inhibitor that would retain both the zinc-binding ability and the biological activity. Chemists found an ingenious solution to this problem by accepting a partial loss of potency from replacement of a key sulfur atom that was offset by the potency-increasing effect of modifying another portion of the molecule that enhances its binding to the ACE molecule. The end result was the highly effective inhibitor, enalapril.
All in all, the ACE story is a beautiful illustration of successful interaction among chemists, biochemists, and biologists in both academic and industrial environments. It set the stage for the discovery of other highly specific enzyme inhibitors such as lovastatin, which effectively and safely lowers cholesterol levels.