3
Theoretical Models of SMO Structure and Atmosphere
CURRENT STATUS OF MODELS
SMOs provide exotic laboratories for investigations of atmospheric physics and chemistry. Table 3.1 compares the bulk characteristics of Jupiter with those of a putative 5-Gy-old brown dwarf 50 times more massive. These characteristics approximately replicate those inferred from spectra of Gliese 229B, the first brown dwarf to be unambiguously detected and the only one bright enough and readily separable from its binary companion to allow for spectroscopic study of its structure.
TABLE 3.1 Bulk Characteristics of Jupiter and a Typical Brown Dwarf
Jupiter |
Brown Dwarf |
|
Mass (Jupiter masses) |
1 |
~50 |
Radius (km) |
71,500 |
~60,000 |
Photospheric gravity (m s−2) |
24.60 |
~12,000 |
Photospheric pressure (bar) |
~0.4 |
~10 |
Effective temperature (K) |
124 |
1,000 |
The brown dwarf and Jupiter, in sharing the same size, differ from one another dramatically in their “photospheric” gravities (i.e., the gravity at the radius corresponding approximately to unit optical depth in the atmosphere). The atmosphere of the brown dwarf can be pictured therefore as a thin layer, with a scale height six times greater than that of Jupiter.1 The temperatures measured in Gliese 229B exceed those observed for Jupiter by an order of magnitude, as does the “photospheric” pressure (i.e., the approximate pressure at the level in the atmosphere from which radiation is emitted). In addition, the flux emitted from Gliese 229B exceeds that of Jupiter by several orders of magnitude. Spectroscopic studies have identified many of Gliese 229B's major atmospheric constituents and set limits on its thermal profile and mass. Efforts are currently under way to determine the cloud structure of its atmosphere (see presentation by F. Allard in this chapter). The implications of these pioneering studies for atmospheric physics and chemistry are discussed below.
Observations of cool stars and brown dwarfs indicate molecular compositions dictated largely by atmospheric temperatures. Within hydrogen-rich atmospheres, carbon and nitrogen, for example, reside in NH3 and CH4 molecules at temperatures below ~1000 K (see presentation
1 |
For a recent review, see, for example, F. Allard et al., “Model Atmospheres of Very Low Mass Stars and Brown Dwarfs.” Annual Reviews of Astronomy and Astrophysics, 35: 137, 1998. |
by A. Burrows in this chapter). At temperatures exceeding ~1000 K, however, N2 and CO dominate. Jupiter's upper troposphere exhibits signatures of CH4 and NH3. This is in contrast to the dominance of CO and N2 in cool stars. Gliese 229B, with an effective temperature of 900 to 1000 K, lies in between these two regimes. It is just cool enough to contain CH4 in thermochemical equilibrium at photospheric levels. Yet Gliese 229B's effective temperature is near that required for equal abundances of N2 and NH3, with best estimates indicating a dominance of NH3 over N2. Spectra of Gliese 229B agree with thermochemical equilibrium calculations, displaying copious features of CH4 and H2O (see Figure 3.1). The weaker signatures of NH3 make it more difficult to observe and, in fact, it has not been detected yet.
Brown dwarfs allow us to examine the nature of atmospheric dynamics from those species that are not in equilibrium. It is possible that NH3 is depleted from the upper atmosphere of Gliese 229B as a result of convection: hot underlying gas transported to the photosphere at rates fast enough to quench in abundances of a deeper atmospheric level. The upper atmosphere would then be in disequilibrium and contain compounds native to this hotter and deeper level. Such a mechanism has been proposed to explain the trace amounts of CO in Jupiter' s upper troposphere—CO rapidly upwells from the level where temperatures are ~1100 K.
If, indeed, further investigation implicates convection as the source of excessive amounts of CO in the observable atmosphere, the NH3, N2, and CO abundances in Gliese 229B will serve as powerful probes of deep atmospheric dynamics. The composition of disequilibrium species may indicate the range of levels from which material is convected and perhaps even the nature of the convection itself.
Gliese 229B's spectrum reveals an extremely clear atmosphere devoid of high cloud decks. The clarity of a planet's or brown dwarf's atmosphere depends on its thermal structure and the saturation temperature of the major constituents. Jupiter's deep atmosphere is largely concealed by an upper deck of NH3 clouds at 0.3 to 0.6 bar, and below that by the condensation of H2O at 2 bar. Gliese 229B's atmosphere is warm enough to prevent these species from condensing and cool enough to keep the next suite of condensables from residing in the upper atmosphere. As a result, Gliese 229B's atmosphere allows visibility to depths at which the pressure is greater than 20 bar, well below the region observable in Jupiter by remote sensing, and the limit of the Galileo probe's in situ exploration. Spectra at visible wavelengths taken by the U.K. Infrared Telescope (UKIRT), Keck, and HST may allow us to identify the structure and composition of Gliese 229B's deep clouds, and hence probe the characteristics of the deep atmospheres of substellar objects.
Detailed atmospheric models of SMOs are also being used to interpret observations at the bottom of the main sequence of old, low-metallicity clusters (see presentation by G. Chabrier in this chapter). The ability of the models to reproduce the colors and luminosities of such objects over a range of metallicities (and hence contributions of molecules to the spectrum) attests to the utility of high-fidelity, but complex, models employing accurate molecular opacities. Information that can be extracted from such models includes mass functions of the clusters, the contribution of SMOs to dark matter in the galactic spheroid and dark halo, and even the validity of distance determinations from various techniques (e.g., Hipparcos versus ground-based data). Such models are time-consuming to develop and computationally demanding (see presentation by P. Hauschildt in this chapter); hence their success in interpreting both individual objects (Gliese 229B) and SMOs in clusters is gratifying.
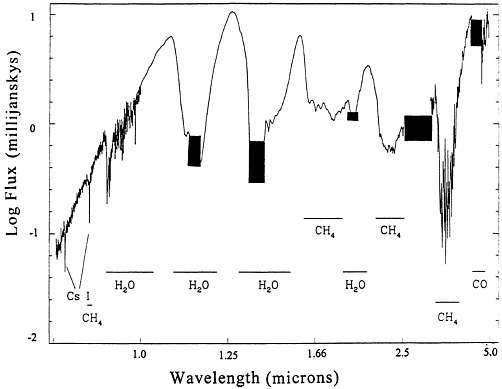
FIGURE 3.1 This spectrum of Gliese 229B demonstrates the enormous complexity of the atmosphere of a cool brown dwarf. The spectrum resembles that of Jupiter far more than any other celestial object because it contains deep absorption features due to methane (CH4) and water (H2O). In addition to methane, there is a small amount of carbon monoxide (CO), as well as features due to cesium (Cs). The spectrum of many extrasolar planets will, probably, bear a strong resemblance to this spectrum. The dark bands represent regions where the spectrum is unmeasurable from the ground because of absorption in Earth's atmosphere. This spectrum was made with the Near-Infrared Camera and the Low-Resolution Imaging Spectrometer at the W.M. Keck Observatory. Illustration adapted from B.R. Oppenheimer et al., “The Spectrum of the Brown Dwarf Giese 229B,” Astrophysical Journal, 502: 932, 1998.
AN HISTORICAL PERSPECTIVE ON THEORETICAL STUDIES OF BROWN DWARFS Peter Hauschildt University of Georgia I will give an overview of model calculations of the interiors, evolution, and atmospheres of brown dwarfs over the last 35 years. The simulations have become more and more sophisticated and accurate during this time, driven by improvements both in the input physics (equation of state, opacities, etc.) and in “enabling technologies” (computers, algorithms, etc.). For example, the development of detailed equations of state for the cool and dense interiors of brown dwarfs and the coupling of non-gray model atmospheres to evolutionary models has led to important breakthroughs. Modern parallel supercomputers and parallel algorithms allow us to include several hundred million molecular lines in brown dwarf model atmosphere calculations leading to the large grids required for detailed evolutionary sequences. These advances allow increasingly realistic simulations that have vastly improved our ability to understand the observational data. A number of discrepancies remain and point toward interesting physical effects that need to be included in the calculations, such as a better modeling of dust formation and opacity as well as improved molecular line lists for many molecules. All important feature of recent advances is that they are in part the result of synergy effects originating in other fields (e.g., supernovae and nova atmospheres) and, in turn, have led to spin-offs back into these fields. MODEL ATMOSPHERES AND SYNTHETIC SPECTRA OF BROWN DWARFS2 France Allard Wichita State University Accurate model atmospheres are necessary for the calculation of synthetic spectra and the quantitative derivation of the surface chemical composition. They form a necessary step in the transformation from observed colors to theoretical effective temperatures. A proper understanding of their colors is essential in the search for brown dwarfs. Photosphere models also serve as boundary conditions for calculations of both stellar interiors and chromospheres. The generation of frequency-averaged opacities is a natural by-product of these calculations, and such opacities are valuable for several fields of research, including star and planet formation; the formation, structure, and evolution of accretion disks; stellar evolution; and stellar pulsation and dynamics. The properties of dust grains, which are present in the atmospheres of cool dwarfs as well as the interplanetary and interstellar media, play a critical role in many of these processes. While ongoing ground-based and infrared space-based surveys of unprecedented faintness and precision continue to reveal cool substellar objects, the presence of first molecules and then condensed particulates greatly complicates their physical properties. Crystals of, for example, Fe, Ni, Al2O3, CaTiO3, and various calcium silicates form in their cool photospheres ( Tgas ≤1800 K). As a result, the absorption bands of the TiO, FeH, and CaH molecules, still
|
strong in a dM8 star, disappear gradually from the optical spectra of the faintest red dwarfs. A fraction of these dust grains form haze and cloud layers that remain suspended in the photosphere, and that heat up their atmosphere. Water-vapor bands, shaping their infrared spectral distribution, saturate as a result, and convey extreme infrared colors (J-K #DXGT#1.2) to these objects. Pressure-induced H2 bands show briefly through the K-band flux maximum. And, eventually, in cooler, evolved brown dwarfs such as Gliese 229B (Teff≈900 K), most grains have rained or sedimented out below the photosphere, letting methane bands finally emerge in the 2.5-and 1.6-μm spectral windows. Their photosphere becomes essentially radiative through relief of the tremendous blocking pressures exerted by the stronger TiO and H2O opacities in hotter, fully convective, stellar objects. Models that should predict and reproduce quantitatively the spectroscopic and photometric properties of substellar objects must therefore address the following principal issues:
INTERIOR AND EVOLUTIONARY MODELS OF BROWN DWARFS Adam Burrows University of Arizona Doppler spectroscopy has recently revealed ~20 giant planets and brown dwarfs orbiting nearby stars, including τ Bootis, 51 Pegasi, υ Andromedae, 55 Cancri, ρ Coronae Borealis, 70 Virginis, 16 Cygni B, and 47 Ursae Majoris. In addition, the brown dwarf Gliese 229B has been directly detected at wavelengths from 0.65 to 10 μm. Gliese 229B's discovery is a watershed, since it has methane spectral features and an inferred effective temperature (Teff ~ 950 K) that is unambiguously below the solar-metallicity main sequence edge. In parallel with these recent planetary and brown dwarf discoveries have been observations in the optical and the near-infrared of hot young brown dwarfs and late-M dwarfs that bracket the substellar limit. However, the study of the atmospheres, spectra, colors, compositions, and evolution of extrasolar giant planets (EGPs) and brown dwarfs is still in its infancy. Despite this, we can summarize some of their properties. By definition, brown dwarfs do not burn hydrogen stably on the main sequence, but they can, nevertheless, have thermonuclear phases. Objects more massive than 0.07 M◉ (70 Jupiter masses), but less massive than the ~0.075 M◉ that marks the solar-metallicity stellar edge, can derive many tens of percent of their luminosity from the truncated p-p chain before they sputter out and cool to blackness. Objects more massive than 0.013 M◉ can consume their stores of “primordial” deuterium. During this phase, which lasts from 106 to 108 years, the object's luminosity can be approximately three times larger than it would have been had its cooling not been partially stabilized by the thermonuclear energy source. Stars more massive than ~0.06 M◉ burn their stock of lithium, leading to the lithium test now being profitably employed. |
Brown dwarf and EGP cores contain liquid metallic hydrogen/helium mixtures and have densities from ~1 to 2000 gm cm−3 and temperatures that can approach 2.7 × 106 K. Surface gravities for the family range from 2 × 103 to 3 × 105 cm s−2, and their radii hover around 0.1 R◉. After a Hubble time, their luminosities can range from ~10−5 to 10−10 L◉ and their effective temperatures are below ~1700 K. Even without central energy sources, these objects are convective throughout, save for the thin (~10−5 by mass) radiative atmospheres that serve as valves to space. At low temperatures and high gravities, their atmospheres are composed predominantly of molecules such as H2, H2O, CH4, CO, N2, and NH3, though many other molecular species, including silicates, chlorides, sulfides, and phosphorus-bearing compounds, should survive in interesting amounts, some producing hazes and clouds. Water and ammonia clouds form at effective temperatures below ~400 K and ~200 K, respectively. All of the above depends sensitively upon metallicity. In particular, the edge mass and luminosity vary from 0.074 M◉ and 6 × 10−5 L◉ at solar metallicity to 0.092 M◉and 1.3 × 10−3 L◉ at zero metallicity. However, the radii of substellar objects are only weakly dependent on metallicity and mass, varying by only ~50% at later times and shrinking to below 0.2 R◉ within 106 years. The peak radius of an old giant planet occurs near 4 Jupiter masses. At the densities achieved in such substellar objects, the Coulomb interaction is still quite important in the equation of state, but the objects are mostly electron degenerate and can be viewed as inhabiting a low-mass, hydrogen-rich extension of the white dwarf family. There are a few major aspects of the atmospheres of SMOs that bear listing and that uniquely characterize them. For effective temperatures below 1300 K, the dominant equilibrium carbon molecule is CH4, not CO, and below 600 K the dominant nitrogen molecule is NH3, not N2. The major opacity sources are H2, H2O, CH4, and NH3. Collision-induced absorption of H2 partially suppresses emissions longward of ~10 μm. The holes in the opacity spectrum of H2O that define the classic telluric infrared bands also regulate much of the emission from EGP/brown dwarfs in the near infrared. The windows in H2O and the suppression by H2 conspire to force flux to the blue for a given effective temperature. The upshot is an exotic spectrum enhanced relative to the blackbody value in the J and H bands (~1.2 μm and 1.6 μm, respectively) by as much as 2 to 10 orders of magnitude, depending on the effective temperature. In addition, as the effective temperature decreases below ~1000 K, the flux in the M band (~5μm ) is progressively enhanced relative to the blackbody value. Hence, the J,H, and M bands are the premier bands in which to search for cold substellar objects. Even though K band (~2.2 μm) fluxes are generally higher than blackbody values, H2 and CH4 absorption features in the K band decrease its importance relative to J and H. As a consequence of the increase in atmospheric pressure with decreasing effective temperature, the anomalously blue J-K and H-K colors get bluer, not redder. Preliminary Conclusions Our recent theoretical studies have led to some interesting preliminary conclusions:
|
In support of the myriad direct searches now being conducted around the globe and in an effort to better understand this problematic substellar branch, theory should address at least the following questions. As it does, the two allied disciplines of planetary science and astronomy could be better integrated into a new synthesis, with substellar-mass objects as its nexus. Theoretical Questions
|
THEORY AND OBSERVATION OF KINEMATICALLY OLD, LOW-METALLICITY OBJECTS Gilles Chabrier Ecole Normale Superieur de Lyon We first examine the characteristic properties of metal-depleted low-mass stars and brown dwarfs, compared to their solar-metallicity counterparts: central and effective temperature, luminosity, radius. We present the metallicity dependence of the mass/effective-temperature relationship and of the hydrogen-burning limit. We give the characteristic magnitudes of the brown dwarf limit for subsolar metal abundances in various optical and infrared filters. We examine the expected photometric signatures of substellar objects, which stem from their very physical properties, in these filters. Theory is then compared with observation in different (optical and infrared) color-magnitude diagrams (CMD) for various globular clusters with different metallicities observed with the Hubble Space Telescope (HST), including the most recent observations with the Near-Infrared Camera and Multi-Object Spectrometer (NICMOS). This yields the derivation of the characteristic bolometric luminosity functions for these clusters and of their present-day and initial mass functions. These latter are well represented by slowly rising power-law functions down to the bottom of the main sequence. We present the predicted luminosity functions for these clusters in the brown dwarf domain, which should be in reach with ongoing infrared projects. This yields the determination of the stellar and brown dwarf densities in various globular clusters. We then turn to field high-velocity low-mass stars, characteristic of the spheroid (stellar halo) and dark halo populations. We first determine the metallicity dispersion of these objects from their photometric signature in CMD and the luminosity functions obtained from ground-based observations and from HST standard and deep-field observations. We transform these luminosity functions into mass functions. This yields the determination of the halo stellar density and halo/disk normalization down to the brown dwarf limit. Extrapolation into the substellar regime yields the maximum contribution of brown dwarfs to the spheroid and dark halo populations and to the galactic dynamical mass. We examine the prospects for the search of stellar and substellar kinematically old objects with ongoing or near-future projects like the European Southern Observatory's (ESO) Imaging Survey (EIS), and the “Son of ISAAC” (SOFI) 1- to 2.5μm imager and spectrometer for ESO's New Technology Telescope (NTT). |