6
Making the Case for Inquiry
Educators need evidence drawn from research to help them implement and justify inquiry-based approaches to teaching and learning science. Many science teachers, for example, question why they should reorient their teaching toward inquiry-based methods. School boards may want to know why they should support inquiry-based curricula and professional development. Preservice teachers may question the need for an inquiry approach in their courses. Parents may want to know why their sons and daughters need to learn so differently from the way they did. Indeed, everyone should want to know the basis for choices about teaching and learning.
Chapter 2 defined inquiry-based teaching as experiences that help students acquire concepts of science, skills and abilities of scientific inquiry, and understandings about scientific inquiry. That chapter also pointed out, as does the National Science Education Standards, that effective science teachers use many teaching strategies. For example, there are times when explicit or direct instruction is a more appropriate choice and will complement inquiry-based teaching, especially when students have already had a great deal of direct experience with a particular phenomenon.
This chapter closely examines the research base for inquiry-based teaching. It begins by looking at the research on learning and the kinds of learning environments that promote learning. This research is of particular interest because of the strong parallels between how research says students learn important science concepts and the processes of scientific inquiry that are used in inquiry-based teaching. The chapter then addresses research that is specifically focused on inquiry-based science teaching. Throughout, connections are made with the images and ideas discussed in previous chapters. Finally, the chapter describes the limitations of educational research in general.
Taken together, the research findings presented in this chapter build a powerful argument for inquiry-based teaching and learning of science.
HOW STUDENTS LEARN SCIENCE
A recent report of the National Research Council entitled How People Learn (Bransford et al., 1999) demonstrates a broad consensus about how learning occurs. The report synthesized research from a variety of fields, including cognition, child development, and brain functioning. It also drew from research across content areas, with important contributions from the research on science learning.
Several general findings from the study are presented below, with illustrations drawn from research on science learning. These findings are in turn connected to the definition of inquiry introduced in Chapter 2 and used throughout this volume.
Research Finding 1: Understanding science is more than knowing facts. The emphasis of recent research has been on learning for understanding, which means gaining knowledge that can be used and applied to novel situations. Research on people who have expertise in a field demonstrates that they (a) have a deep foundation of factual knowledge, (b) understand facts and ideas in the context of a conceptual framework, and (c) organize knowledge in ways that allow for retrieval and application (Donovan et al., 1999). They also have inquiry procedures available that help them solve new problems efficiently and effectively. Their extensive and well-organized bodies of knowledge affect what they notice and how they organize, represent, and interpret the information in their environments. In turn, this interaction with their environments affects their abilities to remember, reason, and solve prob
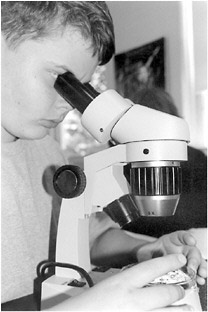
lems. For their knowledge to be usable in these ways, it must be connected and organized through important concepts. Experts must know the contexts in which knowledge is applicable and must be able to transfer that knowledge from one
context to another. What this means for science teaching is that for students to be able to use what they learn, they must understand the major concepts, build a strong base of supporting factual information, and know how to apply their knowledge effectively (Bransford et al., 1999).
Knowing science, however, is not only knowing scientific concepts and information. The research on learning indicates that students need to develop abilities to inquire similar to those in the Standards (and discussed in Chapter 2). All students need to learn strategies for scientific thinking (Linn et al., 1989). They should be able to describe a problem in detail before attempting a solution, determine what relevant information should enter the analysis of a problem, and decide which procedures can be used to generate descriptions and analyses of the problem (Glaser, 1992). Through scientific inquiry, students can gain new data to change their ideas or deepen their understanding of important scientific principles. They also develop important abilities such as reasoning, careful observing, and logical analysis (Minstrell, 1989; Rosebery et al., 1992). Thus the research on expertise confirms the importance of helping students understand major scientific concepts and related factual information, and develop a variety of inquiry abilities.
Research Finding 2: Students build new knowledge and understanding on what they already know and believe. Students have conceptions about natural phenomena, and those conceptions influence their learning. When consistent with ideas accepted by the scientific community, this “prior” or “informal” knowledge forms a strong base on which to build deeper understandings. Many learners’ preconceptions, however, are inconsistent with accepted, extant science knowledge. These preconceptions are generally ideas that are reasonable and appropriate in a limited context, but students inappropriately apply them to situations where they do not work (Anderson and Smith, 1987; Driver et al., 1985; 1994). Students often hold tenaciously to these ideas, and their preconceptions can be resistant to change, particularly using conventional teaching strategies (Wandersee et al., 1994). For example, many students continue to believe that the earth is hotter in the summer because it is closer to the sun, even after being “taught” the correct reason. In Chapter 3, Mr. Gilbert uncovered and worked with his students’ preconceived ideas about the moon’s phases as did Mr. Hull with his students’ conceptions of forces on stationary objects. In Chapter 5, Lezlie comments about recognizing her own “misconcep-
tions” about physics, which made her pay more attention to those of her students. The research on students’ conceptions of science principles is substantial, addressing a wide range of scientific areas (Driver et al., 1985; 1994; Minstrell, 1989; 1992; Novak, 1987).
Research Finding 3: Students formulate new knowledge by modifying and refining their current concepts and by adding new concepts to what they already know (Driver et al., 1985; 1994). The research on conceptual change indicates that students change their ideas when they find these ideas to be unsatisfactory, that is, when their present ideas do not sufficiently describe or explain an event or observation. Further, they change their ideas when they discover alternatives that seem plausible and appear to be more useful (Hewson and Thorley, 1989). This is what happened with students in Ms. Flores’s elementary classroom as they considered why the trees grow differently, illustrated in Chapter 3, and Lillian’s college students, whose understanding of electrical circuits grew substantially as they were challenged with more complex phenomena, described in Chapter 5. Other research suggests that whether and how learners change their ideas depends on what they view as evidence for or against a competing idea (Duschl and Gitomer, 1991). This relates to students’ views of science and scientific explanations. Students often think of science as a collection of facts to be memorized and explanations as reports of isolated events. When this is true, there is less likelihood that students will actively
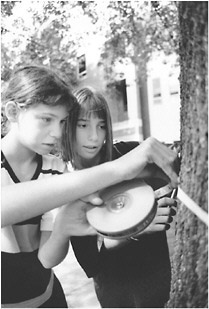
seek evidence for different explanations, think about why one set of evidence is stronger than another, and make good decisions about which explanation has the most support. Their ideas about natural phenomena are unlikely to change on the basis of sound scientific reasoning (Songer and Linn, 1991).
Research Finding 4: Learning is mediated by the social environment in which learners interact
with others. Saying that learners construct their own knowledge does not imply that they do so alone. Research indicates that learners benefit from opportunities to articulate their ideas to others, challenge each others’ ideas, and, in doing so, reconstruct their ideas (Rosebery et al., 1992). Students in every vignette in Chapter 3 had all these opportunities as they developed explanations for basic observations like dying trees, moon phases, and murkiness of lake water. Teachers in Chapter 5 similarly experienced and then recognized the benefits of collaboration to their learning of both science and pedagogy.
Research Finding 5: Effective learning requires that students take control of their own learning. Students need to learn to recognize when they understand and when they need more information. They need to be able and know when to ask: What kinds of evidence do I need in order to believe particular claims? How can I build my own theories of phenomena and test them effectively (White and Frederiksen, in press)? Good learners articulate their own ideas, compare and contrast them with those of others, and provide reasons why they accept one point of view rather than another. They are “metacognitive,” that is, they are aware and capable of monitoring and regulating their thoughts and their knowledge (American Psychological Association, 1993). Students in all four Chapter 3 vignettes worked hard to devise clear arguments for their conclusions; Mr. Gilbert’s students went further by reflecting on how good the models were that they used to explain moon phases and how they needed to account for the models’ deficiencies. In Chapter 5, Sandy and her teacher colleagues shared student work and videos of their teaching to reflect on how what they were doing did or did not help their students learn. Research underscores the value of student self-assessment in developing their understanding of science concepts, as well as their abilities to reason and think critically (Black and Wiliam, 1998b; Duschl and Gitomer, 1997). As Black and Wiliam (1998b) note, it is only when students are trained in and given opportunities for self-assessment that “they can understand the main purposes of their learning and thereby grasp what they need to do to achieve.” (p. 143)
Research Finding 6: The ability to apply knowledge to novel situations, that is, transfer of learning, is affected by the degree to which students learn with understanding. In order to use what they learn, learners must achieve an initial threshold of knowledge, practice using the knowledge in a variety of contexts, and then get feedback on how well they did. To be able to use
their learning in the future, people need time during their learning to grapple with specific information, explore underlying concepts, and make connections to what they already know. They need tasks that are challenging but not frustrating and social opportunities to see the usefulness of what they are learning and to see its impact on others. Finally, they are more apt to apply what they know to novel situations if they have learned to extract the underlying themes and principles from their learning experiences (Bransford et al., 1999; Bruer, 1993; Byrnes, 1996). Students in Ms. Idoni’s class, for example, were called on to apply their learning to a hypothetical situation of a fish kill, which was quite different from what they had observed in the lake. They needed to apply their understanding of the nature and consequences of pollution to this new challenge. Several teachers in Chapter 5, for example, Steve in his physics teaching and Lezlie with her kindergarten classes, took the ideas they learned through professional development directly into their classrooms.
These findings from research into learning connect in important ways with the definition of inquiry presented earlier. The Standards stress understanding major science concepts and building abilities to “do” science. These are the capacities recognized in experts, who have a well-structured understanding of the major ideas in their field and inquiry abilities that help them solve new problems efficiently and effectively (Finding 1). The research suggests that to develop expertise requires achieving both kinds of outcomes specified in the Standards: learning subject matter as well as the thinking strategies needed to use and inquire more deeply into those concepts.
Inquiry focuses on a scientifically-oriented question, problem, or phenomenon, beginning with what the learner knows and actively engaging him or her in the search for answers and explanations (Findings 2, 3). This search involves gathering and analyzing information; making inferences and predictions; and actively creating, modifying, and discarding some explanations (Finding 3). As students work together to discuss the evidence, compare results, and, with teacher guidance, connect their results with scientific knowledge, their understanding broadens (Findings 3, 4). As they develop their abilities to question, reason, and think critically about scientific phenomena, they take increasing control of their own learning (Finding 5). They can use their broadened science knowledge and inquiry abilities to address other questions and problems and to develop or test explanations for other phenomena of interest (Finding 6). In this way, effective learning involves the reorganization of the deep struc-
ture of one’s thought processes. The learner comes to own a new idea or new way of thinking. Without this, school learning becomes a transitory experience with little application to future thought and action.
EFFECTIVE LEARNING ENVIRONMENTS AND EXPERIENCES
Research on student learning leads to a question of great practical importance: What kinds of learning experiences and learning environments promote science learning? The research synthesized in How People Learn (Bransford et al., 1999) suggests that effective teachers employ strategies that attend to four elements: learners, knowledge, assessment, and community.
Learner-centered environments pay careful attention to the knowledge, skills, attitudes, and beliefs that learners bring to the educational setting. Accomplished teachers respect and understand their students’ prior experiences and understandings and use these as a foundation on which to build new understandings (Duckworth, 1987; American Psychological Association, 1993). For example, in Chapter 3, Ms. Flores and Mr. Gilbert both elicited students’ knowledge before launching into their new topics and used what they learned to focus student inquiries. In Chapter 5, Joanna and her teacher colleagues at the science museum were carefully supported to begin with what they knew and pursue questions of interest in order to deepen and broaden their understandings.
Research on students who are learning English as a second language points clearly to the need for teachers’ attention to what these students bring to the science classroom (Fradd and Lee, 1999; Rosebery et al., 1992).
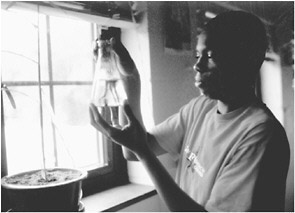
Students from diverse language backgrounds vary greatly in their abilities to express, communicate, discuss, and demonstrate their understandings of science and of scientific concepts by virtue of their developing language abilities (CCSSO, 1999). Further, like all students, they vary in what they understand of science; this is complicated by the fact that their home cultures may not have exposed them to science as generally taught in schools. As Fradd and Lee (1999) note, “the norms and values of science
are more familiar to students from the mainstream middle-class than to students from diverse languages and cultures (p. 15).” Therefore, learner-centered environments in which teachers build new learning on the knowledge, skills, attitudes, and beliefs that students bring to the classroom, are critical to science learning of English language learners.
Knowledge-centered environments help students develop well-organized bodies of knowledge and organize that knowledge so that it supports planning and strategic thinking. In these kinds of environments, students “learn their way around” a discipline. Like experts, they are able to make connections among ideas. In these kinds of learning environments, teachers help students think about the general principles or “big” ideas in a subject. When they learn new knowledge, students also learn where it applies and how. They have opportunities to practice using it in novel situations. Their learning environments promote the sort of problem-solving behavior observed in experts (Bransford et al., 1999). All of the Chapter 3 vignettes showed students attacking problems using their firsthand observations and science knowledge from other sources to build new general ideas. In Chapter 5, Gabe’s and Steve’s field experiences, Joanna’s experience in the science museum, and Lezlie’s experience in the physics laboratory created opportunities to learn science through firsthand observations gained from “doing” science.
Assessment-centered environments help students learn to monitor and regulate their own learning. They learn to question why they believe what they believe and whether there is sufficient evidence for their beliefs (White and Frederiksen, in press). These environments provide students with opportunities for feedback and revision. Assessment-centered environments also help teachers shape classroom activities, diagnose students’ ideas and products, and guide teachers’ decisions (Duschl and Gitomer, 1997; Gitomer and Duschl, 1995). As Black and Wiliam (1998b) note from their extensive review of the research on classroom assessment, “there is a body of firm evidence that formative assessment is an essential component of classroom work, and that its development can raise standards of achievement.” (p. 148) Assessment plays a major role in the classrooms depicted in Chapter 3, as elaborated in Chapter 4.
Community-centered environments require students to articulate their ideas, challenge those of others, and negotiate deeper meaning along with other learners. Such environments encourage people to learn from one another. They value the search for understanding and acknowledge that mistakes are a necessary ingredient if learning is to occur. Studies of
effective environments for learning science “emphasize the importance of class discussions for developing a language for talking about scientific ideas, for making students’ thinking explicit to the teacher and the rest of the class, and for learning to develop a line of argumentation that uses what one has learned to solve problems and explain phenomena and observations.” (Bransford et al., 1999, p. 171) Further, such environments are open to new ideas and ways of thinking, as the community members are both encouraged and expected to provide each other with feedback and work to incorporate new ideas into their thinking. The development of community and use of community as both stimulus and context for learning is well illustrated in the Chapter 3 vignettes and in the teachers’ stories of their own collaborative learning in Chapter 5.
A number of studies have examined learning environments that incorporate all four of these elements. In their studies of high school physics teaching and learning, Minstrell (1982, 1989, 1992) assessed the following research-based instructional techniques: making students’ thinking visible; bridging from students’ preconceptions to scientifically-based conceptions; and facilitating students’ ability to restructure their own knowledge. The approach depicts the teacher’s role as coach in developing student understanding of major ideas in physics such as force and motion, rather than as a dispenser of facts.
In their studies of young Haitian students’ development of scientific ideas, Rosebery et al. (1992) describe classrooms in which students explore their own questions, design studies, collect information, analyze data and
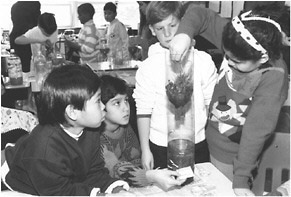
construct evidence, consult experts and literature to help them interpret their test results, and debate the conclusions they derive. The teacher’s role is to guide and support them as they explore problems, define questions, and build and argue about theories. The learning environment these researchers describe incorporates all the features discussed above.
Many research studies of environments in which students learn for understanding use standardized measures of student achievement, although these measures do not emphasize the kinds of deep understanding on which the research is focused. According to the National
Research Council (Bransford et al., 1999), “in some cases there is evidence that teaching for understanding can increase scores on standardized measures (e.g., Resnick et al., 1991); in other cases, scores on standardized tests are unaffected, but the students show sizable advantages on assessments that are sensitive to their comprehension and understanding rather than reflecting sheer memorization (e.g., Carpenter et al., 1996; Secules et al., 1997)” (p. 177).
Research on effective learning and learning environments has interesting parallels to the process of scientific inquiry itself (Duschl, 1992). Both learner and scientist actively construct knowledge through confrontation with a new question, problem, or phenomenon, gathering information, and creating explanations. Throughout the process of inquiry, both constantly evaluate and reevaluate the nature and strength of evidence and share and then critique their explanations and those of others. A classroom in which students use scientific inquiry to learn is one that resembles those that research has found the most effective for learning for understanding. This consequence strengthens the argument for inquiry-based teaching.
RESEARCH ON INQUIRY-BASED SCIENCE TEACHING
The final line of research supporting the use of inquiry in teaching and learning involves the study of specific science programs. In the 1960s and 1970s, a number of curriculum projects, including the Biological Sciences Curriculum Study (BSCS) programs in biology, the Physical Sciences Study Committee (PSSC) materials in physics, and the Science Curriculum Improvement Study (SCIS) and Elementary Science Study (ESS) units for elementary school science, incorporated approaches to teaching and learning that today would fall, at least in part, under the heading of inquiry. The term “inquiry” was used explicitly in studies of various NSF-funded curriculum projects (Shymansky et al., 1983). These studies examined teaching techniques such as “inquiry-discovery” (Wise and Okey, 1983), project-based science instruction (Blumenfeld, 1994; Krajcik et al., 1994; Ladewski et al., 1994; Marx et al., 1994), and newer technology-enhanced curriculum (White and Frederiksen, in press). Although this research suffers from the lack of a shared, precise definition of inquiry, it is possible to look for patterns that show up across studies.
In the 1980s, several meta-analyses were done of the original research projects, in which the individual projects are re-analyzed as a whole to yield broader results than any one study alone can produce. In general, these meta-analyses show that inquiry-based teaching produces positive,
although in some cases modest, results across a variety of indicators. For example, studies of inquiry-oriented curriculum programs (Shymansky et al., 1983; Shymansky et al., 1990; Mechling and Oliver, 1983) demonstrated significant positive effects on various quantitative measures, including cognitive achievement, process skills, and attitudes toward science. (However, there was essentially no correlation between positive results and expert ratings of the degree of inquiry in the materials.) Wise and Okey (1983) showed a positive effect for what they called inquiry-discovery teaching for cognitive outcomes. Although Lott (1983) found only small differences between inductive and deductive approaches, the differences were in favor of the inductive approach, which incorporates elements of inquiry teaching and learning. Other meta-analyses conducted independently at approximately the same time, such as those by Weinstein et al. (1982) and Bredderman (1982), produced similar positive results. Studies in particular subject areas, such as biology (Hurd, 1998), also generally favored inquiry-based approaches.
Other studies have demonstrated a range of other specific outcomes from inquiry-based teaching, including vocabulary knowledge and conceptual understanding (Lloyd, 1988), critical thinking (Narode, 1987), inquiry abilities and physics understanding (White and Frederiksen, in press), and positive attitudes toward science (Shymansky et al., 1983). In studies of underrepresented and underserved populations, inquiry-oriented strategies enhanced scientific ways of thinking, talking, and writing for language learners and helped them to acquire English and reasoning skills (Rosebery et al., 1992).
David Haury (1993) has provided a brief, but thorough, summary of the above research. His review concludes that inquiry-oriented teaching can result in outcomes that include scientific literacy, familiarity with science processes, vocabulary knowledge,
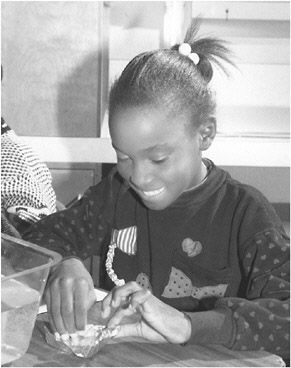
conceptual understanding, critical thinking, and positive attitudes toward science. Another review from Flick (1995) addresses research on explicit instruction as well as inquiry-oriented instruction. He notes that explicit teaching can produce major gains in student achievement on selected kinds of instructional objectives, but goes on to point out that “The high levels of teacher supervision implied by explicit teaching models may not foster the kinds of thinking required for instruction with complex and more ill-structured tasks” (p. 17).
In the final analysis, review of the research on the effectiveness of inquiry-based teaching and learning leads to a discussion of one’s objectives for science education. If one accepts the full sweep of content in the National Science Education Standards, including conceptual understanding of science principles, comprehension of the nature of scientific inquiry, development of the abilities for inquiry, and a grasp of applications of science knowledge to societal and personal issues, this body of research clearly suggests that teaching through inquiry is effective.
Research on inquiry is continuing. Some studies are directed at special student populations. For example, research on teachers’ roles in promoting science inquiry with students from diverse language backgrounds, although in its infancy, has pointed to the need to consider carefully how best to design and structure inquiries for these students (Fradd and Lee, 1999). Research by Delpit (1995) suggests the importance of students receiving explicit instruction in the skills they need to engage in science inquiry and learn from inquiry experiences. Other research by Rosebery et al. (1992), as noted earlier, indicates that students learning English can successfully engage in science inquiry and learn science concepts as well as the language in culture of science. In their research on students with learning disabilities, Scruggs et al. (1993) found significantly higher learning with an inquiry-oriented approach. Studies continue in other countries as well. A study in university-level biochemistry in Turkey (Basaga et al., 1994) found higher achievement for students using an inquiry-oriented approach than those in a traditional approach. Another university-level study in Ireland (Heywood and Heywood, 1992) found similar results on pupil tests for students in discovery and expository approaches, but greater student motivation with discovery approaches. A pattern of general support for inquiry-based teaching continues to emerge from the research.
THE LIMITATIONS AND CONTRIBUTIONS OF EDUCATIONAL RESEARCH REGARDING DECISIONS ABOUT POLICY
In addition to examining the research base for inquiry, it is important to understand what research can and cannot provide. As Hiebert (1999) points out in his discussions of the research support for the national mathematics standards, the question about the strength of that research is fair, even though it does not have a simple answer. Simple answers, in fact, do not provide the credibility necessary to support a substantially different approach to teaching and learning.
Research has several limitations. First, research cannot determine goals or standards, which are primarily a reflection of values (Hiebert, 1999). The standards being written by some states and districts are largely lists of factual information to be memorized. These reflect a different set of values than those behind the National Science Education Standards, which focus on major concepts in science and on learning for understanding. The methods of teaching most appropriate for these different kinds of standards vary as well. Inquiry-based teaching that encourages questioning, developing alternative explanations, challenging each others’ ideas, and conducting open-ended, long-term projects may not be most appropriate if the goal is for students to memorize information.
Second, research alone cannot establish what is best. Education is a very complicated enterprise, and most outcomes are influenced by more factors than can be identified, let alone controlled.
Third, research cannot prescribe a curriculum or pedagogical approach for all students and for all times. Such decisions must always be made within a given context, and the level of confidence with which they are made changes with new information and new conditions.
This said, there are several things that research can do (Hiebert, 1999). It can be used to make decisions that are based on probabilities that a certain outcome will ensue. Thus, research can inform decisions but not guarantee that they are right for all circumstances. By reviewing many studies done under a variety of conditions and looking for patterns in the results, decision-makers can increase the possibility of success. Indeed, looking at a variety of studies can sensitize decision-makers to the complexities involved in a decision and to the crucial issues involved.
Research also can help prevent mistakes. It can show that some goals, however lofty, are unattainable. And it can probe below the surface to indicate why certain results occur: why certain programs do not work as
expected or certain goals are not achieved. Of particular interest when student learning is being assessed is the nature of the opportunities students had to learn and achieve the outcomes.
Research can also show what is possible and what looks promising. It can illuminate what students are capable of, what improvements are feasible, and what parts of reform visions are reasonable. In this respect, research can suggest what is not known and could benefit from some additional examination. For example, given the importance of formative assessment established in Chapter 4, research has begun to focus on listening and feedback in science classrooms.
THE CASE FOR STANDARDS-BASED INQUIRY
The research on inquiry-based teaching and learning comes from a number of sources. The research base on learning and on effective learning environments makes a strong case for inquiry-based approaches. Research on programs and materials that incorporate inquiry also shows positive influences on many critical dimensions of student learning. Although the research demands a clearer definition of terms and falls short of illuminating all the complexities of teaching for understanding, the evidence from several streams of research is both positive and promising.
Effective science teachers take a number of approaches to teaching. However, as this chapter has argued, their use of inquiry can have a powerful influence on their students’ science learning.