1
History and Context
DEFINITION OF PESTICIDE
The word pesticide and its more specific variants insecticide, fungicide, and herbicide have been in common parlance for over a century. Although there is general agreement as to their essential meaning, there is by no means widespread consistency in their use by the general public, by scientists, or by legislators and regulators. The literal meaning of pesticide is “pest-killer”, but common usage has restricted its meaning considerably. Although physical agents—such as heat, cold, and even shoe leather—have proved to be effective pest-killers over the years, they are rarely if ever considered pesticides. The entry of the terms into the language coincided with and was occasioned by the introduction and adoption of chemical agents and today these terms are used almost exclusively to refer to chemical agents that kill pests. It is important to define the terms precisely and to use them consistently; the definitions will affect the changing status of pesticides in regulatory contexts and their economic impact in the broader context of US agriculture.
The original definition of pesticide in the Federal Insecticide, Fungicide, and Rodenticide Act (FIFRA) came from earlier California law. The legal definition has since been modified at the federal and state levels. According to FIFRA, “a pesticide is any substance (or mixture of substances) intended for a pesticidal purpose, i.e., use for the purpose of preventing, destroying, repelling, or mitigating any pest or use as a plant regulator, defoliant, or desiccant, except that the term ‘pesticides' shall
not include any article that is a ‘new animal drug' within the meaning of section 201(w) of the Federal Food, Drug, and Cosmetic Act (31 USD 321 (w)), that has been determined by the Secretary of Health and Human Services not to be a new animal drug by a regulation establishing conditions of use for the article, or that is an animal feed.” What can be considered a pesticide is determined in part by what is specified as a pest. According to FIFRA, “an organism is declared to be a pest under circumstances that make it deleterious to man or the environment.” FIFRA also lays out the foundation for the regulation of pesticides by the federal government: according to the July 1, 1997, edition of FIFRA, as amended, “no person may distribute or sell any pesticide product that is not registered under the act except as provided in (152.20, 152.25 and 152.30).” Pesticides are regulated by three federal agencies: the US Department of Agriculture (USDA), the Environmental Protection Agency (EPA), and the Food and Drug Administration (FDA).
Although the legal definition of a pesticide might appear straightforward, its biological underpinnings are tenuous at best. Among the most important complications is the broad definition of pest itself, a term with no biological validity (Newsome, 1967). Simply put, a pest is any organism that appears in a place where it is unwelcome, and unwelcome is defined in strictly human terms. Pest status does not adhere to taxonomic lines; some families of insects, for example, have members that are pest species and others that are regarded as beneficial (the beetle family Coccinellidae, for example, includes economically damaging herbivorous crop pests and predaceous biological control agents). By the same token, some species are regarded as an economic boon in some localities and a bane in others. Even in the same locality, different constituencies might regard the same species from different perspectives. In some parts of the southern United States, for example, Johnsongrass is considered a noxious weed by crop producers and an important component of dove habitat by hunters. Clearly, the same species of plant can be a crop or a weed, depending on circumstances. The fact that little if any taxonomic consistency underlies pest status suggests that selectivity for pest species, generally considered a positive attribute of a pesticide, is unlikely to be achieved easily.
Confusion in the general public as to the nature and origin of pesticides is a reflection of inconsistencies in usage among various constituencies. According to FIFRA, substances that repel but do not kill pests and substances that mitigate pest problems are considered pesticides—a designation that is neither intuitive nor etymologically consistent. Moreover, in a legal context, origins serve as the basis of differentiation. According to EPA, for example, substances produced naturally by plants to defend against insects and pathogenic microbes—and the genetic material re-
quired for the production of such substances—are considered “plant-pesticides”. The term pesticide without modification implies a material synthesized by humans. There is a regulatory program in which incentives are provided for reduced-risk pesticides (EPA 1992), that is, pesticides with minimal impacts on nontarget species (including humans) and ecosystems. Such pesticides might include natural metabolites, which often are highly biodegradable and can be selective in their mode of action. The implication is that these materials are produced by living organisms as opposed to being synthesized industrially.
Recent developments in the production of pesticides might cloud that distinction further in the near future. For example, a biological step (using an esterase for chiral resolution) instead of a chemical catalysis is used in the synthesis of Pydrin® insecticide in Japan; however, this material is considered a synthetic pesticide, not a natural metabolite. In the pharmaceutical arena, pregnenolone and progesterone have been synthesized in yeast via deletion of one gene and insertion of five genes (Duport et al. 1998). The question arises as to how extensively organisms can be manipulated and still be considered to produce a natural metabolite. The reduction in restrictions for pesticidal natural metabolites offers a needed boost to companies seeking to market materials generally considered to be more environmentally compatible. This influx of new products will likely increase the diversity of materials available to pest-management specialists and create new confusion in the minds of customers.
Other considerations in defining pesticide are the mode of application and the intent of the applicator. Applied biological control involves human input, but the agents involved —natural enemies of pest species—are not regarded as pesticides by FIFRA. Classical biological control involves the intentional release of a beneficial, generally nonindigenous species to control a deleterious species. On occasion, the population of the beneficial species must be augmented by human intervention, often in the form of inundative numbers to flood a problem area rather than inoculative numbers to establish long-term populations. FIFRA does not regulate macroorganisms, such as parasitic wasps and nonpathogenic nematodes, and microorganisms are exempt from oversight if their vector is a nematode (NRC 1996). In general, biological control agents offer a high degree of specificity, but there is a discernible trend toward a pesticide application mentality with inundative use of these technologies. The use of microbial pesticides is an example of how nonchemical control techniques can have greater similarities to chemical control techniques than to other, more natural measures of pest control.
For the purposes of this report, we use the FIFRA definition of pesticide with a clear extension to encompass biopesticides (which include microbial pesticides and plant metabolites (box 1-1), but we will specifi-
cally exclude agents that are normally considered to be biological control agents and plant varieties that are produced by traditional breeding programs. We will extend the term to agents used in veterinary medicine to control insect and nematode pests.
Pesticides used in companion animals and livestock include pesticides that fit the FIFRA definition and that have been used widely in the treatment of insect infestations of animals. With the advent of the avermectin class of endectocides in the late 1970s—ivermectin, doramectin, milbemycin, and moxidectin—compounds that are active against both external insects and internal parasites became available (figure 1-1). Thus, some pesticides used on animals undergo approval through the FDA New Animal Drug Application (NADA) policy and do not undergo review and approval through FIFRA policy.
HISTORY OF PEST CONTROL
From their earliest days agriculturists have been beset by pests. Carvings dating back to 2300 BC in tombs in Egypt, one of the centers of plant domestication, depict locusts eating grain. Biblical passages allude to locusts (Exodus 10:3-6, 12-17, 19) and agricultural pests of other kinds (Joel 1:4, 7, 10-12, 17, 18, 2:19, 25; Deuteronomy 28:38, 39, 42; Amos 4:9,
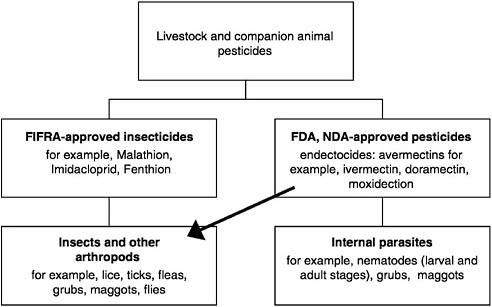
FIGURE 1-1 Relationship between FIFRA-approved insecticides and FDA NADA-approved pesticides used on companion animals and livestock.
19), including weeds (Jonah 2:5). Missing from the writings of the time, however, are descriptions of pest management. Little practical guidance was provided for doing anything other than surrendering to pests, possibly because infestations were often regarded as visitations by angry deities—condign retribution for impure thoughts or disobedience. The most common recourse in the case of infestation was to appeal to a deity for assistance.
The Greeks and Romans also had to deal with outbreaks of insects, weeds, and plant pathogens and similarly resorted to divine intervention for assistance. In the classical era, however, people began to take it upon themselves to rid themselves of pests, and pest-management practices are described in several of the more utilitarian writings that survive. From those texts, for example, Theophrastus' Enquiry into Plants, it is apparent that the ancients had a good grasp of many concepts of pest ecology—that herbivorous insects are host-specific, that population sizes depend on climate and geography, and that there is intraspecific variation in susceptibility to particular pests. Knowledge of life cycles was useful in designing pest-control programs; Aristotle's Generation of Animals was a seminal work in that regard. Pliny the Elder was the authoritative voice on pest control for centuries. Pliny was basically a politician with a penchant for writing—his Natural History encompassed 32 volumes and covered al
most all the knowledge of the day. Because he recorded everything dutifully, however, without commentary or criticism, it is difficult to differentiate between what was regarded as sound science and what was discredited as folk wisdom. Rightly or wrongly, his work influenced almost all later writings about pest control until the 17th century.
According to available sources, the Greeks and Romans relied on several approaches to pest control. Mechanical methods were used extensively, particularly during locust plagues. Systematic manual collecting was even legislated; in Cyrene, according to Pliny, locusts were collected by law three times a year, and in Lemnos the law required that each man take a specific quantity of locusts to the local magistrates (Beavis 1988). Biological methods of control were less easily regulated, but many classical authors noted that birds (including jays and jackdaws) feast on insects. Cultural control, too, was remarkably sophisticated for the era. Many authors mention intercropping cabbages with vetch or with squill to control cabbageworm; given the host specificity of Pieris brassicae and P. rapae, the European cabbageworms, such a practice would likely interfere with host-finding and reduce infestation (Tahavainen and Root 1972).
Chemical control was, for the most part, even more notably like today 's. Elemental sulfur, still in use today as an insecticide, was mixed with oil and used as an insect repellent and was boiled along with bitumen and olive oil leaves as a fumigant. As well, Greeks and Roman agriculturists relied heavily upon plant extracts for protecting cultivated plants from insects, weeds, and pathogens. Extracts could be made in water or oil, from fresh or burned plant material, sprinkled alone or in combination with other ingredients. A wide variety of plants were used, but the list is by no means a random one. Many of the plants specifically mentioned by classical authors as conferring protection against insect herbivores have been shown to contain highly insecticidal components. The recommendation of a solution of “dead larvae from another garden” (Beavis 1988) raises the possibility that alarm pheromones or insect pathogens were exploited for control purposes.
Europeans did little to advance the front of pest management after the collapse of the Roman Empire; classical authorities were dutifully copied and knowledge progressed slowly. What typified the era in general was not so much progress as regression; instead of relying on scientific observation, people tended to rely on divine intervention (of a sort) to deal with pest problems. There arose in the ninth century the curious and remarkably durable practice of prosecution, excommunication, or anathematization of insects and other animals in ecclesiastical courts of law. Historical records document at least 13 such cases in the 9th through 15th centuries. In the 100-year span of the 16th century, 18 trials were
conducted. They then began to taper off, but the practice continued well into the 19th century (Evans 1906).
As early as the 17th century, more scientific measures were being adopted to combat insect pests. Francis Bacon, lord chancellor of England in 1561–1626, is widely credited with introducing the scientific method. Although classical authorities continued to be acknowledged as sources, their accounts no longer took precedence over direct observation and experimentation. Moreover, throughout the 17th and 18th centuries, the stepped-up pace of world travel and exploration led to the discovery of new cultures, approaches, and materials— tobacco, for example, a New World plant known as a fumitory, also proved to be an exceptional insecticide. Most of the magical practices fell into abeyance and were virtually forgotten.
By the beginning of the 19th century, the pest controller's armamentarium was restricted primarily to botanical preparations, elemental sulfur, oil soaps and kerosene emulsions to combat insects, and lime and sodium chloride for weed control. By the end of that century, however, a fundamental change had taken effect, owing in large part to two major innovations. Classical biological control —the importation of natural enemies from the original area of an introduced pest—was popularized by the dramatic success of the release of Rodolia cardinalis, the vedalia beetle, to stop an outbreak of Icerya purchasi, cottony cushion scale, in California in 1873. By 1902, biological control was extended to weed management when efforts were launched to identify natural enemies of the weedy shrub Lantana camara for importation into Hawaii, where it was choking out native vegetation (Clausen 1978).
Almost contemporaneous with pioneering developments in biological control was the fortuitous discovery that compounds containing heavy metals have pesticidal properties (Ordish, 1976). Although Paris green, a component of paints that consists of a mixture of copper and arsenic acid, was applied initially to grape foliage in France to discourage thievery, its efficacy in reducing herbivore damage did not escape notice. Its effectiveness, coupled with that of Bordeaux mixture as a fungicide, stimulated extensive testing and adoption of a variety of inorganic compounds as pesticides. Copper sulfate was introduced for use against charlock in wheat fields (and remains in use today as an algicide) (Timmons 1969). Hydrocyanic acid came into use as a fumigant in 1886 against insects, and carbon bisulfide as a fumigant against weeds in 1906. Arsenicals were used for both insect and weed control—lead arsenate for insects in 1892 and sodium arsenite for annual weeds in terrestrial and aquatic systems. Accompanying the proliferation of pesticides was an intensified effort to design and manufacture equipment to increase application efficiency.
The heavy-metal compounds, making up the so-called first genera-
tion of chemical pesticides, also had some drawbacks. Their broad-spectrum toxicity extended to human applicators, and under particular environmental conditions they could cause extensive damage to crop foliage. Moreover, without regulation, unscrupulous manufacturers proliferated, offering for sale products with little or no active ingredient. In California, the extensive fraud led to the Insecticide Law of 1901, the nation 's first pesticide law, which standardized arsenic content in arsenicals. By 1901, California alone was spending over $250,000 on pesticides for food production (Stoll 1995).
Despite the problems associated with inorganic insecticides, they supplanted the use of biological control agents in most segments of the agricultural community. Thus, upon their discovery, the so-called second generation of insecticides, the synthetic organic compounds, found ready markets. Dichlorodiphenyl trichloroethane (DDT) had been synthesized by Otto Ziedler in 1874, but its insecticidal properties were not discovered until 1939, when Paul Muller tested it as part of an extensive screening study. DDT was cheap, persistent, and extraordinarily toxic to a wide variety of arthropods. Its utility in suppressing a typhus epidemic in Italy in 1943-1944 enhanced its appeal, as did its use in reducing wartime casualties in the Pacific Theater from malaria and other insectborne diseases. At the end of World War II, DDT became available for public use and was widely adopted (Dunlap 1981). Its astonishing efficacy led to the development of a variety of chlorinated hydrocarbons, such as gamma-lindane and toxaphene. Another product of wartime research was the organophosphates, which, despite their greater toxicity to mammals and other nontarget species, enjoyed considerable popularity because of their broadspectrum efficacy and low cost (Casida and Quistad 1998).
Herbicides developed in parallel with insecticides during this era. Inorganic agents predominated in the early part of the 20th century. Sodium chlorate was used to control deep-rooted perennial weeds in noncrop areas and in small patches of field bindweed in cultivated fields; borates were found to control weeds without the flammability of the chlorates and are still used under asphalt to prevent weed growth (Timmons 1969). The compound that in 1942 dramatically illustrated the value of synthetic organic compounds for weed control was 2,4-dichlorophenoxyacetic acid (2,4-D); this compound was widely and enthusiastically embraced because of its selectivity for broadleaf weeds. Introduced soon after were substituted ureas and uracils, S-triazines and triazoles, and phenoxyethyl and acid sulfate compounds. From 1950 to 1969, the number of herbicides registered and released for use tripled (Timmons 1969).
The virtues of many of the synthetic organic insecticides proved in the long term to be environmental liabilities (Metcalf, 1980). Persistence
reduced costs of application but also increased the probability of the evolution of resistance. Moreover, persistence and broad-spectrum toxicity led to widespread nontarget effects. Predators and parasites were especially vulnerable because of biomagnification, increases in concentration as material moves up a food chain. Unintended devastation of natural enemies led to the appearance of secondary pests, species that, before extensive insecticide use, were kept in check by their enemies. Biomagnification also led to concern about human health effects of repeated long-term exposure to residual pesticides as environmental and dietary contaminants.
Momentum for reassessments of pesticide safety and efficacy increased through the late 1950s. Public concern about pesticides crystallized with the publication of the book Silent Spring by Rachel Carson in 1962. The thesis of the book was that current patterns of pesticide use were harmful and that environmentally compatible alternatives to pesticides were available and should be used more extensively. Although Carson did not advocate a total ban on pesticide use (rather, she argued for their intelligent use), reaction in the chemical community was swift and critical. Nonetheless, her well-written arguments won her a substantial constituency. In the years following, environmental awareness grew among the public. By the end of the 1960s, a major effort to reevaluate the role of pesticides in US agriculture emerged; it culminated in the creation of the Environmental Protection Agency, a ban on DDT (and later other organochlorine insecticides) for all agricultural uses, and passage of legislation regulating the production and use of pesticides. Since 1971, many synthetic organic insecticides have been canceled or restricted because they posed health or environmental risks (table 1-1).
In the wake of Silent Spring, alternatives to synthetic organic insecticides have received more attention in the research community. In 1959, the first insect pheromone, bombykol, was characterized; by 1966, the sex pheromone of the cabbage looper Trichoplusia ni, a pest of several row crop species, was identified and ushered in an era in which pheromones have been exploited for trapping, mating disruption, and monitoring of pests. Advances in insect physiology allowed the development of control chemicals targeted at disrupting hormones that regulate insect growth, such as methoprene, a compound that works as a juvenile hormone analogue to interfere with maturation. The advantages of these so-called third-generation and fourth-generation pesticides were numerous; among them were that they offered specificity and environmental degradability. Nonchemical alternatives also proliferated, notably, control techniques based on microbial pathogens.
By the 1990s a new approach to pesticide development was made possible by advances in molecular biology and genetic engineering.
TABLE 1-1 EPA Regulatory Actions and Special Review Status of Selected Pesticides Used in Field-Crop Production, 1972-June 1995
Pesticides |
Regulatory Action |
Date |
Alachlor |
Uses restricted and label warning, under EPA review for groundwater contamination |
1987 |
Aldicarb |
Use canceled on bananas, posing dietary risk |
1992 |
Aldrin |
All uses canceled except termite control |
1972 |
Captafol |
All uses canceled |
1987 |
Chlordimeform |
All uses canceled, use of existing inventory until 1989. |
1988 |
Cyanazine |
Manufacturers voluntarily phasing out production by 2000, but stock can be used until 2003 |
1995 |
DDT* |
All uses canceled (except control of vector diseases, health quarantine, and body lice) |
1972 |
Diazinon |
All uses on golf courses and sod farms canceled |
1990 |
Dimethoate |
Dust formulation denied and label changed |
1981 |
Dinoseb |
All uses canceled |
1989 |
EBDC** |
Protective-clothing and wildlife hazard warning |
1982 |
(Mancozeb, Maneb, Metiram, Nabam, Zineb) |
||
Endrin |
All uses canceled |
1985 |
Ethalfluralin |
Benefits exceeded risks; additional data required |
1985 |
Heptachlor |
All uses canceled except homeowner termite control |
1988 |
Linuron |
No regulatory action needed |
1989 |
Methyl bromide |
Annual production and use limited to 1991 levels; use to be terminated in 2001 |
1993 |
Mevinphos |
Voluntary cancellation of all uses |
1994 |
Monocrotophos |
All uses canceled |
1988 |
Parathion |
Use on field crops only, under EPA review with toxicity data requested |
1991 |
Propargite |
Registered use for 10 crops canceled, use for other crops remains legal |
1996 |
Toxaphene |
Most uses canceled except emergency use for specific insect infestation of corn, cotton, and small grains |
1982 |
Trifluralin |
Restrictions on product formulation |
1982 |
2,4-D*** (2,4-DB, 2,4-DP) |
Industry agreed to reduce exposure through label change and user education |
1992 |
*dichlorodiphenyltrichloroethane **ethylene bisdithiocarbamic acid ***2,4-dichlorophenoxyacetic acid Sources: EPA, 1998; Lin et al., 1995. |
Casida and Quistad (1998) highlighted the actual and potential contributions of genetic engineering to pesticide productivity
-
Enhancement of pesticide manufacturing capabilities (via cell-culture techniques).
-
Improvement in rates of discovery of novel compounds (via novel screening techniques).
-
Facilitation of development of transgenic crops that produce their own defenses.
-
Facilitation of development of transgenic natural enemies that can withstand exposure to conventional pesticides.
-
Evolution of new pesticides, in terms of mode of action, structure, specificity and origins.
GOALS OF AGRICULTURE
Pesticides have been an integral part of US agriculture since its earliest days. The goals of US agriculture have historically been to
-
Ensure an adequate supply of high-quality safe food and other agricultural products to meet the nutritional needs of consumers.
-
Sustain a competitive food and agricultural economy.
-
Enhance quality of life and economic opportunity for rural citizens and society as a whole.
-
Maintain a quality environment and natural-resource base (USDA 1999).
The use of pesticides in the past has been motivated and justified by their perceived effectiveness in allowing the agricultural sector to achieve its goals. Agriculture and related activities are evaluated by their contribution to the well-being of the nation (Just et al. 1982) which can be thought of as the sum of the well-being of members of a society. Historically, various agencies in the public sector (such as the US Department of Agriculture, the Food and Drug Administration, and land grant universities) have been extensively involved in
-
Research, development, and testing of pest controls.
-
Oversight of those controls (chemical and, increasingly, biological and genetic) for safety and efficacy.
-
Transfer of the control technologies to producers.
-
Education of the public about pest management.
Public support for research has traditionally been justified by the public-good argument for research that does not lead to commercial innovations. Research that does not generate marketable products that will repay the research is of high priority for public support. It can be research that leads to ecological and evolutionary biological information, to new cultural practices, or to biological control techniques for pest management. Studies in biochemistry, physiology, genomics, and genetics may also contribute to enrichment of these disciplines such that embodied pest control innovations that are marketable may eventually be developed.
Today public-sector researchers face concerns about their continued participation in this enterprise. Federal funding of pesticide research has historically had a very narrow base, evidenced by the pattern of Agricultural Research Service (ARS) funding (table 1-2). Table 1-2 provides a summary of the funding of 85 chemical-pesticide research projects in the USDA ARS in 1999. Most ARS chemical-pesticide research is directed to field studies and efficacy (including postharvest, laboratory efficacy research, and pest-management predictive models). Of the $20,764, 416 that funds the 85 projects, 57% is in that category. The three categories of field studies and efficacy (11), environmental fate (7), and residue analysis (15) are funded at $18.4 million, about 89% of the total for the 85 projects. The more basic chemical-pesticide research (categories 1-7) received about $5.3 million, about 25% of the total; categories 8-15 make up the remaining $15.5 million. Environmental-fate research receives 77% of the funding in the basic categories; field studies-efficacy receives 76% of the funds supporting the more applied research. It is important to note that little ARS funding is directed toward basic research (for example, toxicology or mode-of-action research) that supports development of new chemical pesticides.
Agencies other than USDA contribute to public sector support of pesticide research, albeit at a lower level. Table 1-3 shows National Science Foundation (NSF) awards for the divisions of environmental biology and chemistry for 1995–1999. The table reveals that only 11% of the funded studies involved agriculture, and less than 4 % involved agricultural pests or pesticides.
Advances in science that provide alternatives to chemical controls and that lead to greater understanding of how chemical controls work have changed the atmosphere in which public research decisions are made. Also, as a consequence of those advances, public agencies sensitive to how the scientific basis of criteria for determining pesticide use can change are redefining pesticide use and acceptable amounts of residues in food products. The increasing availability of alternatives to chemical pest controls appears to be emerging as a consideration in their decision-making.
TABLE 1-2 USDA Agricultural Research Service Funding of Chemical-Pesticide Research, 1999
Category |
Research subject |
Funding Dollars |
Percentage of Total |
1 |
Natural products |
392,285 |
1.89 |
2 |
Pest biochemistry |
75,468 |
0.36 |
3 |
Structure-activity relations, molecular modeling |
62,952 |
0.30 |
4 |
Toxicology, mode of action |
0 |
0.00 |
5 |
Metabolism: pest, host, and mammalian |
62,952 |
0.30 |
6 |
Pest resistance (to pesticides) |
693,827 |
3.34 |
7 |
Environmental fate (includes uptake and transport in plants and environmental degradation |
4,051,198 |
19.51 |
8 |
Pesticide-screening bioassays |
0 |
0.00 |
9 |
Application-delivery technology |
850,640 |
4.10 |
10 |
Disposal technology |
0 |
0.00 |
11 |
Field studies-efficacy (includes postharvest, laboratory efficacy research, and pest-management predictive models) |
11,838,807 |
57.02 |
12 |
Resistance management |
182,978 |
0.88 |
13 |
Farmworker protection |
0 |
0.00 |
14 |
Human exposure |
0 |
0.00 |
15 |
Residue analysis (includes chemical detection and methods for residue analysis) |
2,553,309 |
12.30 |
TOTAL1 |
20,764,416 |
100.00 |
|
1Includes salaries and operating expenses in addition to research. Source: Pesticide funding data from Nancy Ragsdale, USDA-ARS, 1999. |
In light of shifting priorities in pesticide research and management policies, the USDA and EPA requested the National Research Council convene a committee of experts to address the future role of pesticides in agriculture and the nature of research that would be required to support development and use of new chemical pesticides.
In its charge, the committee was asked to:
-
Identify the circumstances under which chemical pesticides may be required in future pest management.
-
Determine what types of chemical products are the most appropriate tools for ecologically based pest management.
TABLE 1-3 National Science Foundation Award Data Relevant to Pesticide Research (1995–1999)
Key Words |
No. of Projectsa |
% of Projects |
CHEMISTRY |
||
Agriculture |
12 |
0.62 |
Agriculture + chemical |
5 |
0.26 |
Pesticide |
4 |
0.21 |
Insecticide |
2 |
0.10 |
Herbicide |
3 |
0.15 |
Fungicide |
0 |
0.00 |
Combinatorial chemistry |
14 |
0.72 |
Combinatorial chemistry + pesticide |
0 |
0.00 |
Structure activity |
9 |
0.46 |
Mechanism of action |
4 |
0.21 |
Novel target |
0 |
0.00 |
DIVISION OF ENVIRONMENTAL BIOLOGY |
||
Agriculture |
174 |
11.04 |
Pest |
128 |
8.12 |
Agriculture + pest |
49 |
3.11 |
Insect + pest |
74 |
4.70 |
Pathogen |
54 |
3.43 |
Weed |
20 |
1.27 |
Resistance |
139 |
8.82 |
Pest + resistance |
27 |
1.71 |
Pesticide |
13 |
0.82 |
Insecticide |
7 |
0.44 |
Pesticide + resistance |
6 |
0.38 |
Ecology + pest |
95 |
6.03 |
Biological control |
40 |
2.54 |
Ecosystem |
529 |
33.57 |
Ecosystem + pest |
79 |
5.01 |
LTERb |
48 |
3.05 |
LTERb + agriculture |
10 |
0.63 |
LTERb + agriculture + field crop |
1 |
0.06 |
LTERb + forest |
19 |
1.21 |
aTotal number of projects awarded after in 1995–1999 is 42,850. Of them, less than 1%were related to agriculture and pesticides. Three keyword combinations were used to search for projects that were related to agriculture and pesticides: agriculture, pest + agriculture, and pesticide. Numbers of projects found were 325 (.076% of the total), 88 (.021%), and 99 (.023%) respectively. There are two subdivisions within NSF where such projects would most likely be categorized: Chemistry (CHE) and the Division of Environmental Biology (DEB). CHE accounts for 1,936 (4.52%) of total NSF projects related to agriculture and pesticides. DEB accounts for 1,576 (3.68%). Within the two entities, projects are further divided by keywords used to search for them, as the table shows. bLTER=long term ecological research. Source: NSF, 1999. |
-
Explore the most promising opportunities to increase benefits and reduce health and environmental risks of pesticide use.
-
Recommend an appropriate role for the public sector in research, product development, product testing and registration, implementation of pesticide use strategies, and public education about pesticides.
REFERENCES
Beavis, I.C. 1988. Insects and Other Invertebrates in Classical Antiquity. Devon: University of Exeter.
Carson, R. 1962. Pp. 161–172 in Silent Spring. Boston: Houghton Mifflin.
Casida, J.E., and G.B. Quistad 1998. Golden age of insecticide research; past present or future? Annu. Rev. Entomol. 43:1–16.
Clausen, C.P., ed. 1978. Introduced Parasites and Predators of Arthropod Pests and Weeds: A World Review. Agriculture Handbook/USDA no. 480. Washington, DC: US Dept. of Agriculture, Agricultural Research Service.
Dunlap, T.R. 1981. DDT: Scientists, Citizens, and Public Policy. NJ: Princeton University Press.
Duport, C., R. Spagnoli, E. Degryse, and D. Pompon. 1998. Self-sufficient biosynthesis of pregnenolone and progesterone in engineered yeast. Nature Biotech. 16(2):186–189.
EPA (US Environmental Protection Agency). 1992. Incentives for Development and Registration of Reduced Risk Pesticides . Federal Register. 57(139):32140–32145.
EPA (US Environmental Protection Agency). 1998. Status of Pesticides in Registration, Reregistration, and Special Review (Rainbow Report). Washington, DC: EPA, Office of Pesticide Programs.
EPA (US Environmental Protection Agency). 1999. What are biopesticides. Office of Pesticide Program. [Online]. Available: http://www.epa.gov/oppbppd1/biopesticides/what_are_biopesticides.htm
Evans, E.P. 1906. The Criminal Prosecution and Capital Punishment of Animals. Reprint London: Faber and Faber 1986.
Just, R.E., D.L. Hueth, and A. Schmitz. 1982. Applied Welfare Economics and Public Policy. Englewood Cliffs, NJ: Prentice Hall.
Lin, B.-H., M. Padgitt, L. Bull, H. Delvo, D. Shank, and H. Taylor. 1995. Pesticide and Fertilzer Use and Trends in US Agriculture. Agricultural Economic Report Number 717. Washington, DC: US Department of Agriculture, Economic Research Service.
Metcalf, R.L. 1980. Changing role of insecticides in crop protection. Ann. Rev. Entomol. 25:219–256.
Newsome, L.D. 1967. Consequences of insecticide use on nontarget organisms. Am. Rev. Ent. 12:257–258.
NRC (National Research Council). 1996. Ecologically Based Pest Management: New Solutions for a New Century . Washington, DC: National Academy Press
NSF (National Science Foundation). 1999. Award search results. [Online]. Available: http://www.nsf.gov/verity/srchawdf.
Ordish, G. 1976. The Constant Pest: A Short History of Pests and their Control. New York: Charles Scribner and Sons.
Stoll, S., 1995. Insects and institutions: university science and the fruit business in California. Ag. Hist. 69:216–269.
Tahavainen, J., and R.B. Root. 1972. The influence of vegetational diversity on the population ecology of a specialized herbivore, Phyllotreta cruciferae (Coleoptera: Chrysomelidae). Oecologia 10:321–346.
Timmons, F.L. 1969. A history of weed control in the United States and Canada. Weed Sci. 17:294–307.
USDA (US Department of Agriculture). 1999. Agricultural Research Service mission. [Online]. Available: http://www.ars.usda.gov/afm/mr.html.