2
Potential Environmental and Human Health Implications of Pest-Protected Plants
This chapter begins with a discussion of risk assessment and its application to pest-protected plants and includes a review of the 1987 National Academy of Sciences white paper. It then considers the array of pest-protection traits and their possible use in transgenic pest-protected plants. The bulk of the chapter discusses potential environmental and human health impacts of conventional and transgenic pest-protected plants, such as human toxicity and allergenicity, nontarget effects, hybridization with weedy relatives, and evolution of pest adaptation to pest-protected plants. Scientific data on the potential for adverse environmental and health effects are presented and discussed. Scientific review in federal agencies is also discussed and will be covered in more detail in chapter 3.
2.1 RISK ASSESSMENT AND PEST-PROTECTED PLANTS
The 1987 National Academy of Sciences (NAS) white paper Introduction of rDNA-Engineered Organisms into the Environment stated that the “risks” posed by transgenic organisms are the “same in kind” as those associated with the introduction of unmodified organisms and organisms modified by other methods. Similar conclusions have been reached by international scientific organizations (FAO/WHO 1996; OECD 1993 and 1997). A clear definition of risk is needed if the committee is to interpret and evaluate that statement appropriately. This section clarifies the meaning of risk and related terms according to well-accepted definitions (NRC 1983).
Risk assessment consists of four steps: hazard identification, dose-
response evaluation, exposure assessment, and risk characterization. 1 The definitions of those and other terms in the National Research Council's (NRC's) “Red Book” (NRC 1983) are widely used and generally accepted.
Hazard identification is “the determination of whether a particular chemical is or is not causally linked to particular health effects” (NRC 1983). Hazard is usually determined experimentally in controlled experiments with known doses. In the case of pest-protected plants, hazard would be the effect of a gene product (such as Bacillus thuringiensis (Bt) toxin, or a secondary plant product, such as a glycoalkaloid) which is expressed or changed as a result of genetic modification. The effects of gene flow or the effects on nontarget organisms could be considered potential hazards for ecological risk assessments.
Dose-response assessment is the determination of the relationship between the magnitude of exposure and the probability of occurrence of the adverse effect in question. Dose-response assessment can address the potency or severity of the hazard. For example, many substances lead to adverse effects only at high doses and might be regarded as posing less severe hazards. The relationship between dose and adverse effects for a particular hazard is reflected in the dose-response curve. In the case of pest-protected plants, some proteinase inhibitors require very high concentrations to cause adverse health effects (Ryan 1990). On the other hand, some plant glycoalkaloids cause adverse health effects at relatively low doses. This allows toxicants to be ranked according to “relative hazard” which is not the same as “relative risk.” Overall risk is the product of the likelihood of an adverse consequence and the severity of that consequence. Hazard severity, and probability and magnitude of exposure all contribute to the overall risk. The risks that may be posed by proteinase inhibitors and glycoalkaloids could be similar depending on the probability and magnitude of exposure.
Exposure assessment is the determination of the extent of exposure to a toxicant under any stated set of circumstances. In the context of pest-protected crops, exposure of nontarget species to a plant-pesticide might be considered for ecological risk assessment, and exposure of humans to a plant-pesticide for human health risk assessment. Exposure assessment of pest-protected plants should deal with such questions as how much of the toxicant humans consume, concentrations in the edible portions of the crop, and how often and how much nontarget insects consume.
Risk characterization considers all the above and is often reported as a quantitative assessment of the probability of adverse effects under de-
1 |
Note that these essential steps may be categorized and/or termed differently in various risk assessment frameworks. |
fined conditions of exposure—for example, one in 10,000 humans will become ill given a certain set of circumstances. Hazard identification, dose-response assessment and exposure assessment are all essential elements of a risk assessment.
Standard toxicological human health risk assessment, despite problems of uncertainty and variability and the consequent difficulty in extrapolation, is science-based. Variability is the range of differences implicit in a natural population (such as the genetic variability in sensitivity to allergens); uncertainty is based on incomplete knowledge or data (such as inadequate surveys of genetic variability to allergens) or on measurement error.
Quantitative risk assessment is being used for not only cancer or toxicological risk assessment, but also for ecological risk assessment, microbial risk assessment, and other diverse types of assessment. In principle, quantitative risk assessment of transgenic pest-protected plants could be based on the methods of quantitative risk assessment if a hazard is detected. If adequate data were not available, the assessment could use uncertainty analyses, ranges of values, and extrapolation. However, until methods are adapted and applied to quantitative risk assessments for pest-protected plants, “relative hazard” ranking may be the best approach, recognizing that this is an interim solution and that quantitative risk assessment is the desired goal.
Because the fundamental elements of risk assessment, such as hazard identification, dose-response assessment, exposure assessment, and risk characterization, can also be applied to risk assessments for transgenic pest-protected plants, the committee found that
Health and ecological risk assessments of transgenic pest-protected plants do not differ in principle from the assessment of other health and ecological risks.
2.2 REVIEW OF PREVIOUS NATIONAL ACADEMY OF SCIENCES AND NATIONAL RESEARCH COUNCIL REPORTS
2.2.1 Introduction of Recombinant DNA-engineered Organisms Into the Environment (1987)
In 1987, the NAS published a summary of key issues related to the introduction of recombinant DNA-engineered (rDNA-engineered) organisms into the environment (NAS 1987). This brief white paper outlined the expected risks and benefits associated with all types of transgenic organisms, including bacteria, insects, fish, and crop plants. At the time, commercial field releases of transgenic organisms were still in the planning stages, and the impending “biotechnology revolution ” attracted en-
thusiastic support from some quarters and strong disapproval from others. To address the perception that rDNA techniques might be intrinsically dangerous, the report offered the following conclusions:
-
point 1 “There is no evidence that unique hazards exist either in the use of rDNA techniques or in the movement of genes between unrelated organisms.”
-
point 2 “The risks associated with the introduction of rDNA-engineered organisms are the same in kind as those associated with the introduction of unmodified organisms and organisms modified by other methods.”
-
point 3 “Assessment of the risks of introducing rDNA-engineered organisms into the environment should be based on the nature of the organism and the environment into which it is introduced, not on the method by which it was produced.”
Throughout this report, the committee describes various methods of both conventional and transgenic breeding methods in detail to provide relevant information about their similarities and differences. Some of the similarities and differences in properties of plants produced by varied genetic approaches are presented in box 2.1. Properties of conventional pest-protected plants are discussed, but the committee focuses on risks and benefits that may be posed by growing transgenic pest-protected plants commercially and on their regulatory oversight under the coordinated framework for regulation of genetically engineered organisms.
The 1987 NAS report noted that the risks associated with rDNA-engineered organisms are “the same in kind” as those associated with unmodified organisms and organisms modified by other methods. The committee agrees with that statement for pest-protected plants in that both transgenic and conventional plants may pose certain risks and the resulting plant phenotypes are often similar. Transgenic breeding techniques can be used to obtain the same resistance phenotype as conventional methods (for example resistance to microbial pathogens, nematodes, and insects). Because both methods have the potential to produce organisms of high or low risk, the committee agrees that
The properties of a genetically modified organism should be the focus of risk assessments, not the process by which it was produced (point 3).
In this regard, the committee found that
There is no strict dichotomy between, or new categories of, the health and environmental risks that might be posed by transgenic and conventional pest-protected plants.
BOX 2.1 Summary of Genetic Basis of Resistance Traits That Have Been Bred into Cultivated Plants Using Conventional and Transgenic Techniques
|
The committee recognizes that the magnitude of the risk varies on a product by product basis. The committee also agrees with points 1 and 2 in the sense that the potential hazards and risks associated with the organisms produced by conventional and transgenic methods fall into the
2 |
A molecular technique known as marker-assisted selection can speed the identification of polygenic or single-gene traits in the plant 's own genome, and rapid advances in genomics are expected to speed the identification of additional single-gene resistance traits in plants and other organisms. |
same general categories. As this report discusses, toxicity, allergencity, effects of gene flow, development of resistant pests, and effects on nontarget species are concerns for both conventional and transgenic pest-protected plants.
The committee agrees with the 1987 NAS principles in that the magnitude of quantitative risk does not depend on the genetic-modification process. It depends on the new genes that are expressed in the plant. End points of risk (such as illness in humans and declines in nontarget species) can be the same regardless of whether a specific new gene was transferred by conventional or transgenic methods. For example, if the same alkaloid gene is transferred by sexual hybridization or Agrobacterium-mediated insertion, the risk should be similar. If a gene coding for a novel trait is transferred by transgenic methods, but cannot be transferred by conventional methods, it is the expressed trait that requires scrutiny, not the method of transfer. In summary,
The present committee found the three general principles to be valid within the scope of issues considered by the 1987 paper, and the present report further clarifies and expands on these principles.
Throughout the report, the committee expands on the 1987 principles by describing various methods of both conventional and transgenic plant breeding, and their potential consequences. The greater diversity of genes that can be transferred by transgenic methods, their enhanced effectiveness, and the ability to insert the same gene into many cultivated species have led to concerns about transgenic crops. Does the potential of transgenic methods to expand on the diversity of transferred genes mean that there is a greater chance for unintended risks from transgenic plants than those from conventionally bred plants? That question has been the subject of considerable debate and draws the question away from specific products. Some transgenic breeding results in pest-protective traits that are phenotypically indistinguishable from those conferred by conventional methods. In addition, transgenic methods are based on more complete knowledge of the genes that are being transferred into cultivated plants. In other cases, however, transgenic pest-protection traits may result in plants having new phenotypes, such as novel plant-produced toxins that could potentially affect human or animal health, nontarget organisms, or the weediness of crop relatives. Transgenic methods can also introduce extraneous traits when they involve marker genes, such as antibiotic resistance genes.
An up-to-date assessment of potential problems and advantages of transgenic methods is warranted (see section ES.2). Transgenic methods can improve the precision of plant breeding and lead to many advantages
over current pest control methods. With careful planning and appropriate regulatory oversight, commercial cultivation of transgenic pest-protected plants is not generally expected to pose higher risks and may pose less risk than other commonly used chemical and biological pest-management techniques. The committee concludes that
A major goal for further research and development of transgenic and conventional pest-protected plants should be to enhance agricultural productivity in ways that also foster more sustainable agricultural practices and enhance the preservation of biodiversity, and decrease the potential for health problems that could be associated with some types of pest-protected plants.
2.2.2 Field Testing Genetically Modified Organisms (1989)
To expand on the general principles outlined above, NRC published a more detailed report on how genetically modified plants and microorganisms should be regulated for small-scale, experimental field tests (NRC 1989). The recommendations proved useful and remain well-founded with regard to how federal agencies regulate field testing of genetically engineered organisms. One important and widely accepted conclusion of the 1989 report is that genetically engineered organisms should be evaluated case by case. The report also describes many of the same issues that apply to large-scale introductions, such as the potential to create weeds or insects that are resistant to Bt insecticides. However, because the 1989 report did not directly address health or environmental risks associated with commercialization, it has limited utility for providing guidelines for regulation of transgenic pest-protected plants.
2.3 FORMS AND MECHANISMS OF GENETICALLY CONTROLLED PEST-PROTECTION
Use of genetically controlled pest-protected germplasm for pest management is widely perceived as providing a number of benefits. First, crop losses or damage can be eliminated or minimized resulting in improvement of both yield and quality. Second, resistant germplasm constitutes a low-input option for pest management that often reduces the need for chemical pesticides and their associated financial costs. Third, by reducing the use of traditional pesticides, pest-protected plants can increase the safety of the food supply and reduce environmental impacts. An example of reduced pesticide use and costs as a direct result of planting conventional pest-protected crops is the case of winter wheat bred for resistance to eyespot disease caused by the fungus Pseudocercosporella
herpotrichoides. Resistant cultivars, which were introduced in 1988 and are now grown on nearly 1,000,000 hectares in the Pacific Northwest United States, have reduced midseason fungicide treatments to roughly half of that needed with susceptible cultivars (Jones et al. 1995). Estimates from 1994 indicate that genetic protection from eyespot disease reduced growers' production costs by $40 per hectare.
Plants with pest-protection properties can inhibit growth, reproduction, or survival of a particular pest or group of pests, or they may tolerate a pest infestation with minimal or acceptable levels of damage. Pest-protected plants that reduce pest populations can exhibit pest-protection characteristics through structural mechanisms. Trichomes on leaf surfaces, for example, present a structural barrier that reduces feeding activity of some insects. Pest-defense systems can also involve intracellular or biochemical mechanisms. These defense mechanisms can work through the action of preformed defensive compounds, and through induced defensive compounds, reactions, and signaling pathways that are triggered specifically or nonspecifically by an invading pest.
To understand the rationale of current and future directions of transgenic breeding for pest-protection and to assess risks of transgenic pest-protected plants relative to those that may be posed by conventional pest-protected plants, this section reviews mechanisms of conventional and transgenic resistance to insects and pathogens.
2.3.1 Natural Pest-protection Mechanisms
Preformed Chemical Defenses
Plants constitutively produce a variety of antimicrobial or insecticidal chemicals that are known or suspected to provide pest-protection (Mansfield 1983; Rosenthal and Berenbaum 1991). The chemicals are often sequestered in specialized cells or expressed in particular organs. Chemicals having antibiotic or suppressive activities against pathogens and insects include saponins, glycoalkaloids, terpenoids, and phenolic compounds. They can have acute or chronic toxic effects and some compounds can have behavioral effects on insects that reduce insect feeding, reproduction, or colonization. The saponin avenacin A-1, for example, is a glycosylated triterpene that is toxic to fungi by perturbing membrane structure and function (Osbourn 1996). It is found in the roots of some cereals. Avenacin A-1 in oats confers resistance to a number of root-infecting fungal pathogens, such as Gaeumannomyces graminis. Like other chemical defenses, avenacin A-1 is effective as an antibiotic in proportion to its accumulation in roots, the inherent sensitivity of the fungus, and the ability of the pathogen to detoxify the compound. Some compounds have relatively broad
specificities. Cyclic hydroxamic acids, such as 2,4-dihydroxy-7-methoxy-1,4-benzoxazin-3-one (DIMBOA), have been shown to confer protection against both fungal pathogens and insect pests (Frey et al. 1997).
Although many preformed chemicals, such as avenacin A-1 and DIMBOA, have been shown to provide pest-protection, the great majority of natural plant chemicals that have antibiotic properties in vitro have not been proved to be active defensive compounds in vivo. The array of compounds with potential defensive capability is vast, and it includes a large number of potential animal and human toxins. For example, 49 natural products or metabolites found in cabbage are known toxins in microbial or animal models (Ames et al. 1990a). Additionally, a number of natural products in the food supply do have acute human toxicity; the cholinesterase inhibitors solanine and chaconine in potato are well-documented examples. Ames et al. (1990b) estimated that the typical American consumes such compounds at roughly 1.5 g/day, primarily in fruits and vegetables, but diets rich in fruits and vegetables are associated with lower, not higher, risks of illnesses such as certain forms of cancer and heart disease (NRC 1982). Therefore, there is not necessarily a correlation between consumption of fruits and vegetables containing compounds with toxicity in experimental systems and adverse health effects.
Resistance Genes
Although the term resistance gene is sometimes used to describe any gene that encodes a plant-protection mechanism, it is most commonly applied to a gene that triggers a defense response to a specific pest or pathogen. In this report, these pathogen-specific resistance genes will be referred to as race-specific R genes, or simply, R genes. The more general term, defensive genes, will be used to describe natural plant genes specifying antibiotic or insecticidal factors that have broad specificity. The identification and deployment of R genes have been among the most important factors in the development of high-yielding conventional crop varieties. Genes have allowed the continued cultivation of many crops in areas where virulent pathogens and detrimental pests are common (for example, leaf stem, and stripe rust in wheat) (Knott 1989; Line 1995; McIntosh and Brown 1997). In many cases, the use of R genes has permitted a reduction in reliance on externally applied chemical pesticides (Jones et al. 1995).
Genetic interactions between flax and the flax rust pathogen indicated that many R genes are effective against only particular races of a pathogen (or types of a pathogen with specific virulence properties) (Flor 1971). The races that are suppressed by a given R gene are known to
contain specific “avirulence” genes; races that are not suppressed lack a functional corresponding avirulence gene. In at least some cases, pathogen avirulence genes encode proteins that are required for infection of susceptible plant hosts (Kearney and Staskawicz 1990). The “gene-for-gene” concept was proposed to explain the interaction between a plant R gene and a pathogen avirulence gene, and this concept is used in agriculture to develop pest-protected crop varieties that are resistant to damage by pathogen races that have known virulence properties. A feature of race-specific R genes, and one of the major limitations associated with their use, is the occurrence of pathogen races that are unaffected by a given plant R gene; these can be pre-existing races that lack the corresponding avirulence genes or new races that have lost avirulence gene function. Study of numerous R genes isolated over the last few years has shown that many have a common evolutionary origin (Baker et al. 1997). Furthermore, race-specific R genes appear to function by triggering a cascade of molecular signaling and biochemical reactions that arrest pathogen spread at the initial site of infection, regardless of whether a particular R gene specifies resistance to a virus, fungus, or bacterium.
Several other types of disease-resistance genes that do not fit the gene-for-gene concept have also been identified. The HM1 gene of maize encodes a reductase that inactivates HC toxin, a cyclic tetrapeptide required for virulence of the fungus Cochliobolus carbonum race 1 (Johal and Briggs 1992). The recessive mlo gene in barley confers resistance to all races of the powdery mildew fungus, Erisyphe graminis f. sp. hordei, by priming the onset of several defense pathways (Buschges et al. 1997). Polygenic traits that confer quantitative pest-protection can also provide durable protection. Although the basis for this type of pest-protection is not entirely clear, cumulative effects of plant R genes that have been overcome by virulent pathogens might play a role in some systems (Li et al. 1999).
Genes for controlling insect and other invertebrate pests have also been identified and deployed, although they might be less common than plant R genes for viral, fungal, and bacterial pathogens. Some encode enzymes that catalyze synthesis of insecticidal or insect-deterrent compounds, whereas others trigger localized defense responses. Several nematode R genes are chemically related, or sequence-related, to race-specific pathogen R genes (Cai et al. 1997; Milligan et al. 1998); this suggests that the signaling mechanisms leading to resistance to nematode are similar to those for resistance to pathogens. The tomato Mi gene for resistance to the root-knot nematode, Meloidogyne incognita, also confers resistance to the potato aphid, Macrosiphum euphorbiae (Rossi et al. 1998; Vos et al. 1998); thus, some insect resistance genes could have broad specificity.
Induced Resistance Responses
A number of resistance responses by plants are induced by pathogen invasion or insect attack (Hutcheson 1998). The hypersensitive response (HR) results after R-gene-mediated, race-specific recognition of a pathogen. The HR in a natural infection is often limited to relatively few cells around the initial infection site. It can also be triggered nonspecifically by various elicitor compounds, such as fungal cell-wall components. The HR involves a cascade of reactions that result in production of reactive oxygen intermediates, antimicrobial compounds (termed phytoalexins), and degradative enzymes; alteration of cell membranes and cell walls; and ultimately cell death. The result of the HR in infected tissues is usually localized necrosis, inhibition of pathogen growth, and limitation of the disease. The HR can occur in plants that contain race-specific R genes effective against all types of viruses, fungi, and bacteria.
The HR leads to a number of other localized and systemic processes that result in increased generalized resistance to a wide array of pathogens. The systemic-acquired-resistance response results in activation of genes that encode defensive proteins, such as glucanases and chitinases, and antimicrobial biosynthetic pathways throughout the plant (Ryals et al. 1996). Defensive proteins can also be induced during the natural course of development of some plants; for example, pathogenesis-related proteins (such as several chitinases and osmotin) with antifungal activity are the predominant proteins that accumulate in the ripening fruit of grape plants (Salzman et al. 1998).
Insect herbivore activity can lead to a systemic defense response (Ryan 1990). This response can be triggered by biotic damage, such as that caused by chewing insects, or by mechanical damage. Insect feeding on a single leaf can result in production of defensive chemicals in all of a plant's leaves (Rosenthal and Berenbaum 1991). An important component of this wound-induced response is activation of genes that encode proteins, such as proteinase inhibitors, that have insecticidal activity. Proteinase inhibitors prevent digestion of plant material in the insect gut, and so result in starvation. Thus, plants exposed to chewing insects gain resistance to additional insect feeding through the wound response.
Viruses activate a defensive response that resembles post-transcriptional gene silencing (PTGS) (Carrington and Whitham 1998). PTGS response is adaptive in providing a customized antiviral response to each new virus that the plant encounters. Silencing in response to viruses with a RNA-based genetic code involves degradation of the genome itself. For viruses with a DNA-based genetic code, the PTGS results in degradation of the transcription products (mRNA). In either case this results in lower virus accumulation or in recovery of the plant. PTGS response can be
manipulated in transgenic plants to confer extreme immunity against viruses (Baulcombe 1996).
2.3.2 Transgenic Pest-protection Mechanisms
Plant biologists and breeders have developed a number of plants that have pest-protection conferred by transgenes. Transgenic pest-protection strategies generally depend on expression of novel genetic resources or transfer of natural plant resistance or defense genes. Transgenic pest-protection based on novel genetic traits involves the introduction of genes that interfere with a specific pest but that are derived from organisms in which the gene's natural function is not that of plant protection. The application of transgenic resistance should be most useful where natural conventional breeding has failed due to lack of resistance genes in sexually compatible plants or due to undesirable agronomic traits in conventional pest-protected crops. For example, the oat Pc-2 resistance gene which controls crown rust disease caused by the fungus Puccinia coronata is coinherited with a trait that confers sensitivity to an unrelated fungal pathogen, Cochliobolus victoriae, so it would not be useful to deploy this gene in oat cultivars by conventional breeding methods (Walton 1996). Transgenic pest-protection can also reduce the time required for cultivar development in some crops. Release of conventionally bred varieties of winter wheat that have the eyespot-disease-resistance gene Pch1 required 13 years from the initial crosses, mainly because of time-consuming selections of lines with acceptable agronomic and disease-resistance characters (Jones et al. 1995).
It is important to recognize that transgenic resistance programs do not displace traditional breeding because transgenes alone cannot currently provide the full spectrum of agronomic traits necessary in commercial varieties. Furthermore, use of transgenes for resistance does not circumvent the normal process of agronomic quality assurance and testing that occurs throughout a well-managed breeding program.
Genetically Engineered Pest-protection Based on Novel Genetic Resources
The most publicized examples of engineered resistance based on novel genetic resources involve use of Bacillus thuringiensis (Bt) delta endotoxins (Estruch et al. 1997). Specific Bt endotoxin proteins are toxic to lepidopteran or coleopteran insects—many of which are destructive plant pests (such as the corn earworm and the tobacco budworm on cotton). Bt proteins in fermentation mixtures and spores have been used for decades in microbial formulations and by fermentation of B. thuringiensis strains that produce Bt
crystalline proteins. Commercial transgenic varieties of corn, cotton, and potato that express Bt protein have been successful in reducing the incidence of pest damage and in reducing use of chemical pesticides in many cases (Robinson 1998; USDA 1999d; Gianessi 1999; Mullins and Mills 1999). These varieties may also be less susceptible to opportunistic pathogens that invade through wounds. The incidence of Fusarium ear rot and stalk rots in corn caused by several fungi may be significantly lower in Bt plants (Munkvold 1998). This would have the added benefit of lowering the exposure of humans and animals to fungal mycotoxins.
Pathogen-derived resistance involves the use of genes from a known pathogen in ways that result in protection from that pathogen (Beachy 1997; Sanford and Johnston 1985). The resistance can occur through a number of mechanisms. Expression of a normal or altered form of a pathogen protein in transgenic plants can disrupt the pathogen 's normal pattern or timing of expression of that protein, or interfere with the interaction between a host and the pathogen. Coat protein-mediated resistance to viruses (Baulcombe 1996; Lomonossoff 1995) is the best-known example of pathogen-derived resistance and has been developed commercially in a number of crops. Expression of viral coat protein in plants interferes with uncoating of the viral genome and thereby prevents or delays the establishment of infection. Expression of multiple coat-protein genes confers resistance to multiple viruses (Tricoli et al. 1995). Expression of other types of viral genes that code for replicases and other proteins required for virus replication or movement in plants, has also been demonstrated to confer resistance in many cases (Baulcombe 1996; Lomonossoff 1995).
Pathogen-derived resistance can also trigger mechanisms that initiate or intensify natural plant-protection processes. For example, introduction of functional or nonfunctional viral transgenes into a plant often results in activation PTGS that suppresses expression of the transgene (Baulcombe 1996). The PTGS mechanism involves sequence-specific recognition and degradation of RNA in the cytoplasm (Grant 1999). Plants that activate PTGS to suppress a transgene invariably are highly resistant or immune to infection by the virus in which the transgene originated. In fact, PTGS of transgenes closely resembles the natural silencing response of plants to viruses, which can result in a recovery from the initial symptoms of infection (Al-Kaff et al. 1998; Ratcliff et al. 1997).
Genetically Engineered Resistance Based on Transfer of Natural Pest-Protection Mechanisms
The isolation of natural plant R and defensive genes provides the resources to transfer resistance from one plant species to another. Many
of the known R genes, particularly those which confer protection from pathogen and nematode pests, are highly conserved in structure and function (Baker et al. 1997); that is, an R gene from one plant species will often function after transfer to another plant species. The N gene for resistance to tobacco mosaic virus in Nicotiana tabacum, for example, functions well after transfer to tomato (Whitham et al. 1996), and the Cf-9 gene for race-specific protection of tomato from the fungus Cladosporium fulvum is functional when transferred to tobacco and potato, as the gene triggers HR specifically in response to the C. fulvum avr9 avirulence protein (Hammond-Kosack et al. 1998).
Cloned R genes and pathogen avirulence genes make it possible to engineer natural resistance responses to a wide array of pathogens and pests. For example, combining an R gene with a corresponding avirulence gene under the control of appropriate regulatory genetic elements in transgenic pest-protected plants can facilitate activation of defense responses against pathogens that are normally not limited by that particular R gene.
Transfer of defense genes for specific degradative enzymes and inhibitors can also confer pest-protection. For example, constitutive or localized expression of a variety of genes that encode proteinase inhibitors, chitinases, and lectins in transgenic plants can provide protection against some chewing insects, sucking insects, or nematodes (Johnson et al. 1989; Kramer and Muthukrishnan 1997; Rao et al. 1998; Ryan 1990; Urwin et al. 1997). Transgenic modification of the production of defensive chemicals also will affect resistance to pests and pathogens (for example, Melanson et al. 1997).
Future Directions
Research focused on developing new ways to produce both conventional and transgenic pest-protected plants, is some of the most exciting in the field of plant biology. Through wide crosses and other nontransgenic techniques, plant resistance genes will continue to be transferred to crop species from species at greater and greater taxonomic distances. A number of genomics projects with model and crop plants are yielding data from which information about new R and defense genes can be obtained. That information could lead to identification and manipulation of resistance factors with unique specificities against important pests and pathogens. The signaling mechanisms whereby resistance responses are triggered by insects and pathogens are being unraveled. It might soon be possible to engineer plants with altered signaling components that result in resistance being triggered by a broader array of pests. Understanding how defensive secondary compounds and defense proteins are produced
and regulated should allow design of resistant plants in which the active compound is expressed in defined locations within the plant. New Bt endotoxins with different specificities or targets are on the horizon, as are a variety of novel or pathogen-derived resistance strategies that have high efficiency and specificity.
A major goal of future development of pest-protected plants should be to decrease the potential for ecological and health risks that may be posed by some types of pest-protected plants (section 2.2.1). This work could include using marker-assisted breeding to avoid selecting varieties with inadvertently high levels of potential toxins and limiting expression of transgenes that have potential adverse nontarget effects to nonedible plant tissues. Development of strategies that enhance the effective life span, or durability, of transgenic pest-protection mechanisms is also of vital importance.
2.4 POTENTIAL HEALTH EFFECTS OF DIVERSE GENE PRODUCTS AND BREEDING METHODS
Sections 2.1 and 2.2 discussed standard risk-assessment terminology for GMPP plants and the 1987 NAS principles. One of the conclusions from those sections was that quantitative risk would vary case by case and depend on the gene product (hazard), its potency (hazard severity and dose-response relationship), and magnitude of exposure levels (exposure assessment). The following two subsections discuss the potential of various gene products (discussed in section 2.4.1) to cause adverse health effects and the potential of various genetic modification techniques (discussed in section 2.4.2) to cause indirect effects regardless of the intended gene product.
2.4.1 Health Effects Associated with Different Types of Gene Products
Different types of transgenic pest-protected plants that might be developed have the potential to cause adverse health effects. The degree of risk is related to the chance that potentially hazardous toxic or allergenic compounds are produced and to the magnitude of exposure of such compounds. The chance that hazardous compounds will be produced by either intended or unintended modifications is related largely to the specific type of transgene used.
Race-specific and Other Naturally Occurring Pest-Protective Genes
Pathogen race-specific pest-protective genes
Plants contain hundreds of pathogen race-specific pest-protective genes that are often referred to as R genes (see section 2.3). Most of these genes are evolutionarily conserved in structure and, most likely, in function. R genes for protection against pathogens are routinely transferred between plants by conventional breeding. There are no known toxic or nontarget effects of R gene products aside from their role in triggering localized and systemic defense responses in the presence of specific pathogens. Transfer of race-specific R-gene from a nonedible plant species to an edible species would result in new exposure of consumers and nontarget species to a specific R-gene product, although not to a new class of proteins. Because plants expressing R genes are not thought to mount a defense response unless encountered by pathogens, the chances of unintended health effects due to pleiotropic effects are remote. Compared with R-gene transfer by conventional breeding, introduction of an R gene via a transgenic method should result in fewer unintended effects, because of the lack of introduction of non-R gene DNA into the new variety.
Other pest-protective genes
Familiarity with the structure and function of race-nonspecific and other types of pathogen-protective genes is less than that with the major class of race-specific R genes, although their history of use in conventional breeding suggests that few hazards exist. Most of these pathogen-protective genes are probably conserved among different species of plants. It is unlikely that exposures to new classes of genes or gene products will result from transfer of these genes between plants, particularly if the source is an edible plant. However a protective gene may encode a protein that increases the concentration of one or more plant compounds with potential nontarget toxicity, thus leading to a potential hazard.
Defense Genes Encoding Pest-Degradation or Inhibitor Functions
A number of genes that code for degradative or hydrolytic functions, such as glucanases and chitinases, with pest-protective activities can be induced by infection (section 2.3.1). These types of enzymes are ubiquitous in plants and are common constituents of foods. Transfer of such genes from one edible plant to another is unlikely to cause a problem. However, if these genes were expressed at relatively high constitutive
levels, were derived from nonedible plants or nonplant sources, or were engineered in such a way as to increase stability or alter structure substantially, novel exposures might result. The potential for adverse affects depends on the likelihood of increased toxicity or allergenicity of a novel product. The extent to which those properties are altered is partially predictable and testable.
Some inhibitor proteins, such as proteinase inhibitors, are effective defense proteins that are naturally induced by wounding or attack by chewing insects. Proteinase inhibitors are also present in relatively high concentrations in some food plants, such as potato. Animals can suffer adverse effects if foods high in proteinase inhibitors are consumed (Ryan 1990). Some naturally occurring lectins that have pest-protection attributes are also known to be toxic to humans and animals. Foods that are high in proteinase inhibitors and lectins, such as potatoes and beans, are usually cooked, and cooking destroys inhibitor and toxic activity. Depending on the protein, a plant modified to express high concentrations of inhibitors in edible tissues can cause adverse health effects if the plant is consumed raw, and such a risk can be reduced by designing transgenes that are expressed only in nonedible plant parts.
Genes Encoding Enzymes that Alter Secondary Metabolites or Natural Products
The wide variety of plant chemicals with potential pest-protection characteristics suggests that modification, transfer, or overexpression of genes that control natural-product biosynthesis can result in new types of pest-protected plants (sections 2.3.1 and 2.3.2). It is reasonable to predict that manipulation of those pathways can enhance resistance to insects and pathogens. The known toxicity of many protective natural products to nontarget organisms, however, means that such strategies could pose a risk. Furthermore, alteration of enzymes in one pathway might alter flux through other pathways. For example, Fray et al. (1995) demonstrated that constitutive overexpression of phytoene synthase, an enzyme required for carotenoid biosynthesis, in tomato had the unintended consequence of causing a dwarf phenotype, most likely due to decreases in gibberellin hormone and phytol (chlorophyll side chain) biosynthesis. Modulation of pathways for production of pest-protection chemicals could result in new exposures to potentially toxic compounds. That risk might be minimized by engineering transgenes with regulatory control elements that result in localized expression in nonedible tissues and plant parts. The risk might also be lowered through increased understanding of potentially hazardous compounds in commercial crop plants. Up-to-date and easily accessed databases with qualitative and quantitative descriptions of known or suspected toxicants would be particularly valu-
able in assessing inadvertent risks (sections 2.5.2 and 3.2.4) These databases could be used to catalog potential toxicants and their concentrations in edible portions of prominent cultivars grown under standardized conditions. New cultivars, regardless of how they were produced, could be tested for known or suspected toxicants and compared with established cultivars that are already being consumed.
Pathogen-Derived Protective Genes
Virus-derived transgenes
Because viruses of edible plants are common components of the food supply and no associations between such viral infections and adverse health effects have emerged, transgenic plants that express parts of viral genomes are generally considered not to represent an important human health risk because there is little chance of exposure to a novel virus gene product. The concentration of some viral gene products might be higher in a transgenic plant than in a naturally infected plant; but in the case of coat-protein-expressing plants, the concentrations will likely be lower.
Plants containing virus-derived transgenes that confer protection from pests because of activation of gene silencing generally produce very low concentrations of transgene-encoded protein, because the transgene mRNA is inactivated (section 2.3.2). From the standpoint of exposure to new or enhanced levels of viral protein, transgenic plants that contain silenced transgenes have little chance of causing problems.
Other pathogen-derived or pest-derived protective genes.
Experience with pathogen-derived or pest-derived protection from organisms other than viruses is sparse. It is difficult to assess this class of potential protective genes with regard to risk. New exposures could result, depending on the pathogen or pest, and potential toxicity to non-target organisms is conceivable. Those examples will require relative-risk assessments case by case.
Genes from Sources Other than Plants, Plant Pathogens, or Pests
The various genes from novel genetic resources that confer pest-protection cannot be grouped from the standpoint of health risks. Transgenes that encode protective compounds from nonplant sources, such as Bt delta endotoxin, will probably present novel exposures and must be assessed on a case by case basis.
2.4.2 Indirect Effects Associated with Different Breeding Methods
To understand the risks posed by genetically modified pest-protected plants, it is important to understand that, in addition to the direct effects of the pest-protective gene (section 2.4.1), breeding can lead to indirect effects, such as the effects of extraneous genes linked to the protective genes and pleiotropic effects caused by the protective genes.
The potential for inadvertent changes caused by the addition of extraneous genes that are physically linked to protective genes depends on the breeding method used and the source of the protective gene. The breeding method and the source of genes used for breeding determine the amount of new DNA moved into the cultivar and the number of novel genes linked to the pest-protective gene. Therefore, genetic modification methods, both conventional and transgenic, are discussed below with regard to their potential for adding novel extraneous genes and their potential for causing unanticipated pleiotropic effects.
Conventional Breeding Methods that Involve Sexual Hybridization
The choice of parents used in the crosses and the mating structure of the plant species are important in determining the potential for inadvertent health effects associated with the progeny (hybrid, inbred line, or population).
Intraspecific hybrids of two cultivars
In crosses, or sexual hybrids, the amount of DNA transferred to the progeny can be immense. Depending on the mating design, a parent 's contribution can range from very small (less than 1% for the donor parent in backcrossing) to very large (over 99% for the recurrent parent in backcrossing). In a cross between two parents that have been previously cultivated (that is, cultivars), each parent contributes one half its DNA to the progeny. In bread wheat, each parent contributes 16 billion base pairs to the progeny (see chapter 1, table 1.1). To put that into perspective, each wheat parent contributes roughly 55 times the total amount of DNA found in Arabidopsis thaliana. At first glance, the potential for inadvertent changes that could create new allergens or toxic compounds might seem high. However, through the long history of wheat improvement, wheat has remained a staple food consumed safely by much of the world (people suffering from celiac disease constitute a notable exception). The same is true of most major food crops.
In most conventional plant breeding, the goal is to create new genotypes that combine the favorable alleles from two cultivars into a superior
progeny, which is selected. For cultivated crops without a history of alterations in antinutritional, allergenic, or toxic properties, the creation of new antinutritional, allergenic, or toxic properties due to crosses between two existing cultivars would be extremely rare.
Intraspecific hybrids of a cultivar and a wild relative, or interspecific hybrids (crosses between species in the same genus)
Within a species that includes a cultivar, there might be weedy relatives that can be used as a source of pest-protective genes (section 2.7.2). The potential for unexpected health effects in crosses between a cultivar and a weedy relative in the same species is similar to the potential for health effects associated with interspecific crosses described below.
Species in the same genus have a common ancestry and have numerous related or similar genes. Interspecific crosses are usually between a cultivar and a wild species that has a pest-protective gene of interest. Inasmuch as most of the wild relative's genes are removed by backcrossing, with the exception of those genes linked to the selected protective gene (known as a linkage block), the creation of a new antinutritional, allergenic or toxic constituent in the hybrid cultivar will be rare.
The size of the linkage block associated with the protective gene depends on the rate and type of recombination between the wild relative 's chromosomes and those of the cultivated parent and on the number of backcrosses. Formulas used to estimate the size of the linkage block when there is normal recombination can be found in many papers (for example, Hanson 1959a,b; Muehlbauer et al. 1988). The practical outcome of moving a block of linked genes with the protective gene is that additional genes are carried with the protective gene (for example, Zeven et al. 1983). If homologous recombination is decreased because of poor chromosome pairing, the linkage block will be larger. Poor chromosome pairing occurs in interspecific and, more commonly, in intergeneric crosses, which are discussed below. However, as just mentioned, the species have a common ancestry and similar genes, so most of the genes in the linkage block will be related to those of the cultivar.
Intergeneric hybrids (crosses between genotypes of two genera)
In this case, there is greater divergence in the genetic ancestry, there are more genes that have never previously been combined, and the usually poorer chromosome pairing leads to larger linkage blocks. The genetic divergence is very important in the outcomes of these crosses. For example, bread wheat and rye (Secale cereale L.) are in different genera, but they share a relatively recent progenitor in evolutionary time. Hence,
as in interspecific crosses, bread wheat and rye have many similar genes. The similarity in gene function between the two genera is illustrated by the great success of many bread-wheat cultivars that contain whole chromosome arms from rye that replaced the equivalent chromosome arms of wheat (Zeller and Hsam 1983; Lukaszewski 1990). The ability to replace a chromosome arm without decreasing productivity indicates that many of the genes in the two genera are equivalent.
The amount of DNA contained on an average chromosome arm for wheat would be about 380 million base pairs (16 billion base pairs in the haploid genome divided by 42 chromosome arms). In this example, short chromosome arms were replaced, so they would have fewer than the estimated number of DNA base pairs in an average chromosome arm. However, each chromosome arm would be as large as or larger than the Arabidopsis haploid genome. Historically, intergeneric crosses are usually used with other breeding methods (such as backcrossing) that remove most of one genus's genes from the commercial product. However, because of poor chromosome pairing, there is often little homologous recombination, and large linkage blocks of DNA are retained in the progeny (for example, the whole chromosome arms mentioned above). However, alien introgressions3 into food plants have been common (for example, Friebe et al. 1996) and have been associated with little history of inadvertent problems. The example given above emphasizes rye introgressions into wheat where both plants are food crops, but many gene blocks introgressed into wheat are from grassy relatives whose grain is not consumed by humans.
New methods are constantly being developed to overcome interspecific and intergeneric hybridization barriers. As these barriers are overcome, the overall genetic difference between parents becomes larger. For example, it is now possible to create oat lines that contain corn genes through oat-corn hybridization. If an oat line with a gene for an allergen from corn were released, an oat consumer with corn allergy might no longer know which oat products were safe to eat.
Conventional and Transgenic Genetic Modification Methods that Do Not Involve Sexual Hybridization
Mutagenesis
Mutations include chromosome rearrangements (such as translocations, deletions, and transposable elements) and DNA changes (such as
3 |
Integration of new blocks of DNA or new traits not previously found in the species. |
single base changes, insertions and deletions). The typical variation we see in the traits of organisms generally involves naturally occurring mutations. Natural mutations and mutations induced by chemicals and radiation have been used to produce many commonly used cultivars. In general, few genes are modified and most of the genome is unaffected. Most mutations are from an active form to a less active form and would pose a problem only when the active form is needed to remove an antinutritional, allergenic, or toxic constituent.
Somaclonal variation
Somaclonal variation is considered to be a form of induced mutagenesis occurring during the tissue culture process. The reason for interest in somaclonal variation is that it increases the genetic variation in plants regenerated from tissue culture; one of the general procedures used to develop transgenic plants. Its potential for unfamiliar health effects would be similar to that of mutagenesis.
Somatic-cell fusion
Somatic-cell fusion has the potential to combine whole genomes from genotypes of widely divergent genera. However, it is rare for a somaticfusion hybrid from widely divergent genera to be directly commercialized. More likely, the genes contributed from one genus would be reduced with another breeding technique or genetic manipulation (such as backcrossing). According to the rationale described above for sexual hybridization, somatic-cell fusion involving cells from the same species (intraspecific hybrids) would have a lower potential for adverse health effects than interspecific hybrids, which would have a lower potential than intergeneric hybrids.
Transgenic methods
Introduction of transgenes into plants typically involves random integration of DNA into the nuclear genome and the use of tissue culture, which can lead to somaclonal variation. If integration of a transgene occurs within or near a gene, there is a potential for unintended consequences. Disruption of a gene can lead to its down-regulation or inactivation. If the gene is essential, viable plants will not be recovered after the transformation or transgene introduction. If the gene is not essential for growth and development, viable transgenic plants will be recovered, but they might have unexpected traits.
Introduction of a transgene can also result in activation or up-
regulation of an adjacent gene. In this case, the regulatory regions of the transgene stimulate a nearby resident gene, and potentially cause increased expression of that gene. It has been argued that one unintended consequence of this process is up-regulation of genes for biosynthesis of plant toxins. The potential for overproduction of hazardous compounds by this random integration of transgenes is likely to be similar to that for mutations, transposable-element activation, and other processes that yield genomic variation. The potential for adverse effects can be minimized through characterization of the locus of transgene insertion. Plants with transgenes that insert relatively close to genes known to affect production of potentially toxic compounds can be avoided.
It is important to point out, however, that these pleiotropic effects are not peculiar to transgenic plants. Crops resulting from conventional breeding and other nontransgenic methods can contain potentially hazardous concentrations of naturally occurring toxic compounds, as has been documented in new or established varieties. The introduction of whole chromosomes or chromosome arms from wild, nonedible relatives presents risks that are proportional to the number of alien genes added to the commercial cultivar. With sufficient testing, the risks posed by the introduction of inadvertent, potentially hazardous concentrations of known or suspected toxins into commercial transgenic or conventionally bred cultivars can be reduced.
2.5 POTENTIAL HUMAN HEALTH EFFECTS
In the United States, the EPA proposes to assess the health effects of pest-protected plants under the FIFRA and FFDCA (section 1.5). The FDA will regulate food safety and quality under FFDCA (P.L. 104-170). This section discusses EPA's scientific review of potential human health effects and general scientific issues surrounding those effects.
Although human health risks associated with transgenic pest-protected plants tend to be potential rather than apparent, some regard these potential risks as important and have expressed their views on appropriate testing and controls (OECD 1993a; Goldburg and Tjaden 1990). Potential food safety concerns for transgenic pest-protected plants (and other transgenic plant products) have been identified and articulated by EPA and FDA (FDA 1992) and international organizations (OECD 1993; FAO/ WHO 1996; OECD 1997b). These key food safety considerations have served as a basis of the food safety reviews for the products currently in the market.
The potential risks of transgenic pest-protected plants to human health are generally related to the possibility of introducing new allergens
or toxins into food-plant varieties, the possibility of introducing new allergens into pollen, or the possibility that previously unknown protein combinations now being produced in food plants will have unforeseen secondary or pleiotropic effects. The use of antibiotic-resistance marker genes has also given rise to concern4.
In the regulation of recently approved transgenic pest-protected plant products (that is plant products with Bt and viral coat proteins), the emphasis has not been on detailed assessments of safety for humans or domestic animals. Rather, it has been on explaining the scientific basis for why there is probably no appreciable risk and justifying the tests which are required. Although the assumption of no appreciable risk from the recently reviewed transgenic pest-protected plants (for example, plants containing Cry1A and Cry3A Bt proteins and viral coat proteins) appears reasonable, it is important that the tests that are performed be rigorous, logical and scientifically sound. In most cases, the tests have these qualities. However, specific suggestions for improving the testing protocols can be found in sections section 2.5.1, section 2.5.2, and section 3.1.3).
A number of traditional chemical pesticides are considered human carcinogens (Hodgson and Meyer 1997). Others have been linked to human health problems, such as Parkinson's disease (Fleming et al. 1994). Therefore,
Human health benefits could arise from reductions in the application of chemical pesticides due to the commercial production of certain transgenic pest-protected plants.
But it is not necessarily true that all traditional chemical pesticides pose a risk to human or domestic-animal health, so the benefits will depend on the particular pesticides that transgenic pest-protected plants replace and the effects of the transgenic pest-protected plant on pest control practices.
The proposed human health testing for EPA, and the testing for FDA consultation, fall into the categories outlined in box 2.2. Those categories are general, and considerable variation in methodology is possible. There is evidence that this variation has occurred under the current guidelines (see discussion below). Even though the EPA rule is not final, more specific testing protocols should be developed and adopted (see section 4.3.5 and section 4.3.7).
4 |
Issues surrounding antibiotic resistance, although mentioned, were not analyzed in this report (section ES.1 and ES.2). |
BOX 2.2 Categories of Human Health Testing for EPA and FDA Health-effects assessment (general testing for potential hazards)
Human safety assessment (more specific assessments) Food safety
Nonfood safety (only EPA)
|
2.5.1 Toxicity and Allergenicity Tests During EPA Review
To provide a cross section of recent activity under the general guidance that EPA informally provides to prospective registrants, the committee examined EPA pesticide fact sheets and data provided by the registrant for three registered Bt toxins that are regulated as plant-pesticides: Bt Cry3A delta endotoxin in potato (EPA 1995a), Bt subs. kurstaki Cry1Ac delta endotoxin in cotton (EPA 1995b), and Bt Cry1Ab delta endotoxin in corn (EPA 1997a and 1998a). The committee also reviewed EPA pesticide fact sheets for Bt subs. tolworthi Cry 9C protein in corn (EPA 1998c).
In general, oral toxicity testing for Bt endotoxins is based on the presumption that there is unlikely to be a problem inasmuch as a number of Bt toxins have been widely used for many years in microbial sprays without human toxicity. A variety of Bt proteins have been subjected to toxicological testing, starting with testing conducted on microbial Bt products, which typically contain multiple Bt proteins. This testing included acute, subchronic and chronic toxicology testing of products during the 1960s and 1970s (EPA 1988b; McClintock et al. 1995). However, it should be noted that most previous field uses resulted in minimal toxin ingestion by humans because sprayed microbial Bt toxin only remains effective for an average of 1.5 days (largely because of ultraviolet degradation), and therefore, residues are rare on fruits and vegetables. Also,
sprayed microbial Bt toxins are protoxins, while some Bt plants produce activated toxins.
Information in peer reviewed studies indicates that plant-expressed Bt proteins are probably without human health risk. Nevertheless, a minimal number of properly defined tests are needed to determine if based on plant modification of the proteins, or if based on use of more novel Bt toxins or Bt toxins not found in currently registered microbial products (for example Cry9C), there is a potential impact on human health. Post-transcriptional modification is known to occur in plants and such characteristics as the degree of glycosylation might also affect stability and other physiochemical properties of proteins. Tests should preferably be conducted with the protein as produced in the plant (see also section 3.1.3). However, the committee recognizes that it is often difficult to obtain enough plant-expressed protein; in these cases, the committee recommends that
The EPA should provide clear, scientifically justifiable criteria for establishing biochemical and functional equivalency when registrants request permission to test non plant-expressed proteins in lieu of plant-expressed proteins.
The strong likelihood that gene products currently found in commercial transgenic pest-protected plants are not allergens does not remove the need for a minimum of properly planned and executed tests. For example, allergenicity is assumed to be unimportant for many Bt endotoxins, more because of the common characteristics of food allergens than because of rigorous testing. The Cry 1Ab pesticide fact sheet (EPA 1998a) states that
current scientific knowledge suggests that common food allergens tend to be resistant to degradation by heat, acid and proteases, are glycosylated and present at high concentrations in the food. The delta endotoxins are not present at high concentrations, are not resistant to degradation by heat, acid and proteases, and are apparently not glycosylated when produced in plants.
In the case of Cry3A in potatoes (EPA 1995a), the company demonstrated that the endotoxin is not a major component of the food, is apparently not glycosylated in plants, and is digested by gastric enzymes. However, Cry9C toxin, unlike the Cry1A and 3A toxins, does not degrade rapidly in gastric fluids and is relatively more heat-stable (EPA 1998c); these characteristics of Cry9C raise concerns of allergenicity. It is important to note that levels of gastric enzymes may vary among individuals and that those variations may need to be considered.
Although the standard tests indicate nonallergenicity for Cry3A, they
were not all carried out on the endotoxin produced in potatoes, and none involved testing the immune system itself. Allergenicity is difficult to test, in part because prior exposure is a prerequisite to an allergic reaction, but tests for allergenicity ideally should involve the immune system or use an allergic endpoint. Useful guides to protein allergenicity include a supplement to Critical Reviews in Food Science and Nutrition (Metcalfe 1996a) and the proceedings of a workshop held at the 1998 Society of Toxicology annual meeting and recently published (Kimber et al. 1999). They make it clear that food allergy is relatively common and can have numerous clinical manifestations, some of which are serious and life-threatening. Furthermore, it is well established that allergenic proteins can be found in many food plants, of which some, such as soybeans and potatoes, have been genetically modified for pest-protection, and many others are or will be candidates for this type of genetic modification.
Those two sources also summarize the problems in protein allergenicity testing. Some, such as the double-blind placebo-controlled food challenge and the skin-prick test, although they involve adverse toxicologic endpoints and are carried out directly on human volunteers, nevertheless provide questionable results, in that they require volunteers who are already sensitized and that they are difficult to implement with novel proteins that have not traditionally been consumed. Tiered tests involving protein homology and stability comparisons with known food allergens and immunoassays for specific classes of antibodies are also proposed in these documents and are currently used by the agencies as a screen for allergenicity (figure 2.1). However, the tests in figure 2.1 either are indirect, do not involve adverse effects, or are otherwise problematic for testing of novel proteins that have not previously been components of the food supply. Indeed, figure 2.1 starts with a decision based on whether or not the protein is derived from a source that is known to be allergenic. This decision can usually be made clearly if the source is a food plant. For transgenic proteins such as Bt endotoxins making such an assessment would be complicated. If we conservatively choose the “yes” decision, then it would be extremely difficult to complete all of the tests listed because test materials and previously exposed human subjects are not readily available.
It is clear from the report of Nordlee et al. (1996) on the expression of Brazil nut protein in soybeans that genetic engineering can result in the expression of an allergenic protein in a food plant, but this is not known to be the case with any commercialized transgenic pest-protected plant. However, some testing of pest-protected plants and purified gene products is appropriate in many cases inasmuch as allergenicity is one of the possible adverse effects. The possibility that proteins in spore-crystal formulations of Bt can interact with the human immune system was suggested by a recent study on workers in crops topically treated with Bt
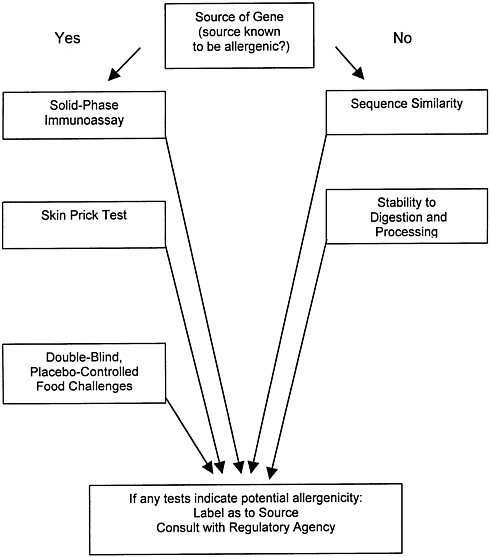
FIGURE 2.1 Tests for Potential Allergenicity. Source: Adapted from Metcalfe (1996b).
sprays (Bernstein et al. 1999). The response seen in this study could have been due to proteins other than the Bt toxins in the formulation, so similar studies should be performed with purified Bt toxins.
2.5.2 Questions Concerning Health Impacts
The potential for transgenic pest-protected plants to pose a threat to human or animal health must be considered against the background of
existing information. To date no such effects have been shown with commercialized transgenic crop plants. The work of Ewen and Pusztai (1999) hints of some possible interaction between a lectin expressed in potato and alterations in the potato caused by the genetic engineering process. According to the study, diets containing genetically engineered potatoes expressing the lectin, Galanthus nivalis agglutinin (GNA), showed some effects on different parts of the rat gastrointestinal tract. Those effects fell into two categories, ones caused by the GNA transgene itself and others caused by pleiotropic effects of expressing the transgene. However, analysis of the work of Ewen and Pusztai by the Royal Society (Royal Society 1999) and by Kuiper et al. (1999) indicates that the study lacked scientific rigor. For example, data concerning the biochemical composition of the potatoes used in the study show that the nontransgenic variety differed significantly from the transgenic variety. These differences could be attributable to natural variations in potato lines and are not necessarily due to the genetic modification (Kuiper et al. 1999).
It is important to ask whether any such threats have resulted from more conventional genetic modification of agricultural crop plants (conventional pest-protected plants) and, if so, whether they can serve as examples for assessing the risks of transgenic pest-protected plants. The questions outlined below can be asked, with equal validity, of transgenic pest-protected or conventional pest-protected plants. It should be noted, however, that conventional breeding usually selects for endpoints that are almost always controlled by several genes. It could be reasoned that such selection because of genetic linkage would be more likely to select simultaneously and inadvertently for an additional undesirable characteristic than would the introduction of a single gene or even a small number of genes with transgenic techniques (section 2.4.2). Both animal and plant breeding have yielded examples of inadvertent selection of undesirable characteristics.
Is the Transgene Expressed in the Edible Part of the Plant?
If so, what are the potential effects on humans, domestic animals, and other nontarget animals? Toxicity testing can be carried out on the gene product or the edible part of the plant, and testing to predict potential effects on humans is carried out on laboratory animals and extrapolated to humans. Toxicity testing of chemicals that are macronutrients, such as proteins, has unique problems. The maximal tolerated dose, as determined in short term tests, is usually very high and palatability problems unrelated to toxicity can interfere with tests. Given the high dose, it is difficult to feed enough of the plant material to the test subjects without making substantial dietary changes. Suitable controls are difficult to de-
vise because the control diet should have the same nutritional characteristics as the control diet.
It has been suggested (for example, Health Canada 1994) that in cases of foods where the concentration of substances to be tested cannot be increased, it would be useful to test the plant material in longterm feeding experiments with animals whose natural diets consist of large quantities and the type of plant material being tested. Research on longterm feeding of transgenic pest-protected plants to those animals (for example, grain and forage crops to livestock) might provide information relevant to human health effects (see also section 3.4). Such testing has been shown to be practical with herbicide-tolerant soybean (Hammond et al. 1996), and with Bt corn (Jackson et al. 1995). Livestock that are normally fed on the crop in question can be fed on the genetically altered variety from weaning until a termination time consistent with normal agricultural practice. The genetically closest variety can be used as a control. These types of studies would make use of the natural diet of the test organism to test large quantities of the whole plant. Nonmodified plant varieties that were identical to the genetically modified plant before the modification occurred should be used as controls. Most toxicity testing is conducted using the purified plant-pesticide (section 3.1.3), and therefore pleiotropic effects of the genetic modification cannot be monitored. If proper controls are used, feeding whole plants to the test animals might allow for the detection of potential toxicity due to pleiotropic effects.
However, there will be a need to carefully assess the relevance of such testing to human health. Humans and ruminants have different digestive systems, as humans are mongastric and ruminants have a four-chambered stomach that can serve as a buffer from the effects of some proteins. Feeding studies using monogastric animals, such as hogs, whose natural diets consist of the transgenic crop in question may provide more relevant information. While the finding of negative effects in such livestock tests would certainly raise concerns, the finding of no effects on these animals is hard to interpret because we lack sufficient information on their biochemical similarities to humans.
Is the Physiology of the Plant Changed by the Genetic Modification?
Potential pleiotropic effects of genetic modification on plant physiology and biochemistry are discussed below. The committee concludes that
It is important to monitor for physiological and biochemical changes during the development of transgenic pest-protected plants.
However, there is lack of data on the natural levels of endogenous plant compounds in both transgenic and conventional pest-protected plants and on how these levels vary with the plant's environment (see section 2.4.1, section 3.2.4, and section 3.4.1).
In addition, any changes in the use of traditional pesticides may be considered in parallel to these pleiotropic effects, as the benefits of decreased use of toxic pesticides may offset the risks of increased toxicity due to genetic modification.
Changes in the levels of toxic secondary plant chemicals
Secondary plant chemicals (chemical compounds that are not required for normal growth and development of the plant) can be toxic to humans and other mammals (Senti and Rizek 1974), and the concentrations of these chemicals can be changed, either purposely or inadvertently, by conventional or transgenic genetic modifications. For example, potatoes, a major source of starch and good-quality protein, contain toxic glycoalkaloids to which humans appear to be more sensitive than other mammals. The modes of action seem to be cholinesterase inhibition in the nervous system and disruption of cell membranes in other organ systems (Friedman and McDonald 1997). This is similar to the mode of action of organophosphate and carbamate insecticides. Many factors, both genetic and environmental, affect glycoalkaloid concentrations in the potato and distribution to different plant parts.
A number of confirmed cases of human poisoning have been caused by potatoes with high glycoalkaloid concentrations (Friedman and McDonald 1997). The conventionally-bred Lenape variety of potato is an example of why great caution must be exercised in the development of new varieties of food plants that contain human toxins. The Lenape variety was developed by crossing Solanum tuberosum and S. chacoense to incorporate resistance to certain pests based on leptines (Sturckow and Low 1961). This variety was released for commercial use in 1967 (Akeley et al. 1968), but was soon withdrawn from the market (Sinden and Webb 1972). The new variety was indeed resistant to pests and had other desirable characteristics, but there were reports of illness, caused by ingesting tubers with high glycoalkaloid content (Zitnak and Johnson 1970). In Sweden, a popular commercial potato variety, Magnum Bonum, was withdrawn from the market for similar reasons (Hellenas et al. 1995).
Another problem of potential importance, the appearance of toxins that were not present in the parental lines, also has been demonstrated in potato. Somatic hybrids between Solanum brevidens and S. tuberosum contained the steroidal alkaloid demissine, not found in either parental line. Laurila et al. (1996) advanced the plausible hypothesis that the hydroge-
nase enzyme of S. brevidens that produces tomatidine from the precursor teinamine by hydrogenation of the double bond at position 5 also produces demissidine by hydrogenating the corresponding double bond in solanidine, a compound that is found in S. tuberosum but not in S. brevidens.
Celery is another example of where conventional plant breeding methods produced an unwanted result. Furanocoumarins are bioactive components of celery and are known to interact with DNA, are mutagenic and carcinogenic, are reproductive toxicants and cause contact dermatitis in humans. A celery line with resistance to Fusarium, but with concentrations of linear furanocoumarins high enough to cause severe contact dermatitis in field workers, was almost released for commercialization (Diawara and Trumble 1997; Trumble et al. 1990 and in press).
Transgenic pest-protected varieties commercialized to date in the United States have not been shown to have elevated levels of certain toxic compounds. For discussion during FDA consultation, companies monitor for changes in the levels of certain endogenous plant compounds. For example, ringspot-resistant transgenic pest-protected papaya lines were shown by chemical analysis to have no more of the suspected human toxicant benzyl isothiocyanate than nontransgenic varieties (University of Hawaii 1997).
Changes in the distribution of secondary plant chemicals so that they are expressed in edible parts of the plant
The “edible” part of a plant varies with the species and the consumer in question. In the human diet, the part eaten can also vary with the cultural background of the consumer. Overall increases in the concentrations of secondary plant chemicals in the total plant might cause toxic chemicals that are normally present only in trace amounts in edible parts to be increased to the point where they pose a toxic hazard. In some cases, genes transferred by conventional breeding can also change the distribution of secondary plant compounds among plant parts. For example, cucumber was bred to produce a bitter protective compound, cucurbitacin, in the leaves and stems of the plant but not in the fruits. A single gene controls the restriction of the compound to the leaves and stems (Barham 1953).
Changes in the chemical or physical properties of the plant surface or edible parts in ways that affect its contact allergenicity or food allergenicity, respectively
Some plants cause either contact allergic responses or food allergies. However, only a few documented examples involving contact allergens-
(Trumble et al. 1990 and in press) show that allergens can be inadvertently changed during conventional breeding, and there is no substantial body of information on this possibility. There are some examples of assessments of endogenous allergens in transgenic plants (Burks and Fuchs 1995; Metcalfe et al. 1996a).
2.5.3 Summary
The above questions can be used to guide the review process for the potential health effects of transgenic pest-protected plants. In reviewing toxicity testing relevant to human health for currently commercialized transgenic pest-protected plant products (that is, Bt toxins and viral proteins), the committee found that,
When the active ingredient is a protein, short-term oral toxicity and potential allergenicity testing are currently appropriate, inasmuch as the testing protocols are the ones currently available. However, testing protocols, particularly for allergenicity, should be improved with additional research.
The committee recommends that
When the active ingredient of a transgenic pest-protected plant is a protein and when health effects data are required, both short term oral toxicity and potential for allergenicity should be tested. Additional categories of helath effects testing (such as for carcinogenicity) should not be required unless justified.
Additional categories of toxicity testing do not appear justified for currently commercialized products (such as products with Cry1A and 3A Bt endotoxins and viral coat proteins). However, in these cases, it important that the tests that are performed be rigorous, logical, and scientifically sound (see section 2.5.1, section 2.5.2, and section 3.1.3). Novel, or less familiar plant-pesticides (that is, in comparison to viral coat proteins and Bt toxins) may require additional toxicity testing.
In addition, given the difficulties with determining the potential allergenicity of proteins not currently in the food supply, the committee recommends that
Priority should be given to the development of improved methods for identifying potential allergens in pest-protected plants, specifically, the development of tests with human immune-system endpoints and of more reliable animal models.
Protocols for toxicity- and allergenicity-testing, whether of gene products or plants, should be reconciled across agencies (see section 4.3). Variations among EPA, FDA, and USDA toxicity-testing protocols, other than those dictated by legislative authority, should be minimized or abolished. It should also be noted that the use of “familiarity ” as a guideline to minimize testing can sometimes be inappropriate and warrants caution. Familiarity can be defined as indirect knowledge or experience obtained from similar gene products, plant varieties, or progenitor varieties grown under similar conditions and used for the same purposes in the same way.
2.6 POTENTIAL EFFECTS ON NONTARGET ORGANISMS
In addition to human health concerns, there is concern that gene products of some transgenic and conventional plants may be toxic to nontarget species in the ecosystem. This section reviews and discusses relevant data on such potential nontarget effects of transgenic and conventional pest-protected plants.
2.6.1 Definitions
It is useful to divide the effects of conventional and transgenic pest-protected plants on nontarget species into direct and indirect effects. Direct effects include the adverse effects of the toxic plant components on nontarget herbivores, omnivores, and microorganisms that feed on live plants (Hare 1992) and on detritivores that feed on dead plants (Horner et al. 1988). When toxic substances are on the surface of a plant, there could also be direct effects on organisms that do not feed on the plant solely caused by contact toxicity or repellence (Farrar and Kennedy 1993).
Indirect effects include adverse effects of genetically modified pest-protected (GMPP) plants through an intermediary species (Bergman and Tingey 1979; Hare 1992; Price et al. 1980). For example, if an herbivore is tolerant of toxic substances in a plant, these substances could be found in the herbivore's digestive system (Price et al. 1980) or even be sequestered in the herbivore's tissues (Brower et al. 1984; Duffey 1980; Tallamy et al. 1998). Such a herbivore can be unpalatable or toxic to some of its predators or parasites because of this biological magnification of plant-defense compounds (Brower et al. 1984; Ferguson and Metcalf 1985; Hoy et al. 1998). If a pest-protected plant causes dramatic decreases in some herbivore or omnivore populations, there will be less nutrient material for the next level in the food chain. It is theoretically possible that a specialized predator, parasite, or pathogen of an affected herbivore could become locally extinct (Riggin-Bucci and Gould 1997).
The few data sets on nontarget impacts of transgenic pest-protected plants come mostly from crops that express Bt toxins (Hoy et al. 1998). However, a considerable number of studies have examined direct and indirect effects of conventional pest-protected crops and of wild host plants that differ in their pesticidal properties. Some of these studies are reviewed in the following sections (2.6.2 and 2.6.3).
2.6.2 Direct Effects
Effects of Structural Changes
A number of studies have shown that the leaf hairs and leaf hair exudates found in resistant cultivars can kill predators and parasitoids of insect pests directly (Bottrell and Barbosa 1998; Price et al. 1980). This can result in a loss of biocontrol of the target pest. A classic study by Rabb and Bradley (1968) demonstrated that the sticky trichomes on tobacco leaves significantly decreased parasitism of the tobacco budworm (Heliothis virescens F.). A study by Kauffman and Kennedy (1989) demonstrated that allelochemicals in the trichomes of resistant tomatoes were toxic to a parasitoid (Campoletis sonorensis) of the corn earworm (Helicoverpa zea). The trichomes in these tomato lines also had an adverse effect on other parasites and predators (for example, Farrar and Kennedy 1993; Kashyap et al. 1991). A study of trichomes of potato plants showed that the impact of increased trichome density on natural enemies seen in the greenhouse was decreased under some field conditions (Obrycki and Tauber 1984); the study underscored the importance of creating experimental conditions similar to those experienced by insects in typical agro-ecosystems.
Changes in glossiness of leaves (for example, Eigenbrode et al. 1995) and general plant architecture (e.g., Kareiva and Sahakian 1990) can also affect the efficacy of natural enemies. Because plant-produced volatile substances attract many natural enemies, a new cultivar with an altered profile of insecticidal volatile chemicals could have reduced attraction to parasites or predators resulting in decreased biological control of the pest (Bottrell and Barbosa 1998; Dicke 1996).
Effects of Plant Ingestion
The chemical composition of forage plants can have a significant effect on livestock and bees (Keeler et al. 1978). Some chemicals can be passed on in the milk of cows and goats (Dickson and King 1978) and the honey of bees (Bull et al. 1968). Plant breeders have long recognized that some cultivars of forage crops can adversely affect livestock growth and
health (Reitz and Caldwell 1974). For example, reed canary grass varies in palatability as a forage crop: the least palatable cultivars have the highest alkaloid concentrations (Williams et al. 1971). Cultivars of alfalfa vary in content of saponin which can depress the growth of chickens (Hanson et al. 1973; Reitz and Caldwell 1974). The terpenoid gossypol makes cotton resistant to caterpillar pests (Shaver and Lukefahr 1969), but cottonseed meal from high gossypol cultivars is poisonous to swine and causes darkened yolks in eggs of chickens (Reitz and Caldwell 1974).
Some plant toxins similar to those in pest-protected plants are relatively stable and can be found in decaying plant tissues (Horner et al. 1988). Only a few studies have examined the effects of such compounds on detritivores (Horner et al. 1988; Paavolainen et al. 1998). One study of balsam poplar showed that the tannins and phenolic chemicals in fallen leaves could inhibit mineralization through two interactions with detritivores. The result can be reduced soil-nitrogen availability (Schimel et al. 1996).
Bt crops have not had an impact on honeybees in tests conducted for EPA approval of Bt crops (EPA 1998c). However, at high concentrations the Cry1Ab toxins engineered into crops have been shown to be toxic to Collembola that are part of the detritus food chain (EPA 1997a). Another Bt toxin, Cry9C, was not found to be toxic to the same Collembola species (EPA 1998c). Cry1Ab but not Cry9C was found to have some toxicity to Daphnia (EPA 1997a and 1998c).
Few studies have focused on measuring the impact of pest-protected plants on the population dynamics of insects (reviewed in Gould 1998). Likewise, few have examined the direct physiological effects of Bt on nontarget herbivores, but some nontarget lepidopterans feeding on crops that contain Cry1A Bt toxins are likely to be affected. These nontarget insects would include lepidopterans that are not pests (that is, do not provide significant damage to the crop) and lepidopteran pests that are not sufficiently affected enough by a pest-protected plant for pest-protection of this kind to be economically useful.
Effects of Pollen Ingestion by Nontarget Herbivores
Pollen from wind-pollinated pest-protected plants can be deposited on nearby vegetation (figure 2.2) and inadvertently ingested by nontarget leaf-eating insects. For example, a small fraction of corn pollen is known to disperse up to180 ft from the edge of the crop (Raynor et al. 1972) and can be deposited on milkweed (Asclepias sp.), which are common in and along edges of corn fields in the midwestern United States where about half the population of US monarchs spend some of the summer (Wassenaar and Hobson 1998). Milkweed is the only food of monarch
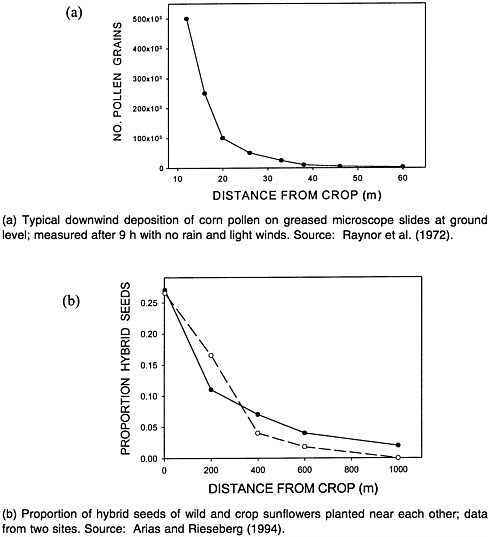
FIGURE 2.2 Examples of pollen and gene dispersal as a function of distance from edge of crop. Dispersal distances are expected to depend on local conditions and relative sizes of donor and recipient groups of plants.
butterfly larvae. Laboratory studies showed that young monarch larvae were killed by ingesting high doses of Bt corn pollen that had been experimentally dusted onto milkweed leaves (Losey et al. 1999). Another study by Hansen and Obrycki (1999c) obtained similar results when monarch larvae were placed on milkweed leaves that had been collected at the edges of Bt and non-Bt corn fields in Iowa and brought into the laboratory. Although both studies should be viewed as preliminary and actual
negative impact, if any, on the population densities of monarch butterflies is yet unknown, they have focused attention on the question of whether widespread dispersal of insecticidal pollen can occur if entire regions are planted with wind-pollinated Bt plants (such as corn, poplar, and pine). It should be noted, however, that some Bt corn cultivars apparently do not produce any Bt in their pollen (EPA 1998b). In those cases, Bt toxin would not be a potential hazard to nontargets even if pollen dispersed great distances.
One recent field test indicates that at least 500 Bt pollen grains per square centimeter is necessary to sicken monarch caterpillars and that milkweed plants growing adjacent to corn fields had only an average of 78 grains per square centimeter (Kendall 1999). Eighty-eight percent of milkweed within one meter fell below the level of toxicity to caterpillars. On the other hand, laboratory tests by Hansen and Obrycki (1999b) demonstrated that when monarch caterpillars consumed milkweed leaves experimentally dusted with 135 grains per square centimeter of pollen (comparable to pollen concentrations they found in the field), there was 46% mortality if the Bt pollen source was insertion event Bt11 and 65% if the source was event 176. Moreover, research suggests that wind direction, rainfall, and other factors can significantly affect pollen concentration (Weiss 1999). Further field-based research is needed to determine whether dispersed Bt pollen could have detectable effects on the population dynamics of nontarget organisms.
2.6.3 Indirect Effects
Studies in which predators were fed insects that developed on pest-protected cultivars have often produced adverse effects on the predator. For example, the compound DIMBOA is found in lepidopteran-resistant grain crops, including corn. Ladybugs that consumed aphids that had fed on diets containing DIMBOA had slower development (Martos et al. 1992). The tobacco hornworm parasitoid Cotesia congregata is adversely affected when the hornworm feeds on tobacco or artificial diets with high nicotine concentrations (Thorpe and Barbosa 1986). Cucumbers that are resistant to spider mites typically have higher concentrations of the triterpenoid cucurbitacin-C than nonresistant cultivars (DaCosta and Jones 1971; Gould 1978). Spotted cucumber beetles that feed on the resistant cultivars sequester cucurbitacin-C in their bodies and eggs (Ferguson and Mecalf 1985). The cucurbitacin-C in their eggs keeps the eggs from being killed by the entomopathogenic fungus Metarhizium anisopliae (Tallamy et al. 1998).
Although a pest-protected plant could have adverse effects on beneficial insects in the agroecosystem, the value of the cultivar is generally
based on the balance between adverse effects on beneficial and detrimental species. For example, Kauffman and Flanders (1985) found an adverse effect of pest-protected soybean on a parasitoid of the Mexican bean beetle, but the effect on the beetle pest was greater than on the parasitoid; it was therefore concluded that overall the cultivar would be useful. Hare (1992) reviewed the published data on overall effects of pest-protected host plants on biological control. Of the 16 case studies he reviewed, there were antagonistic interactions in six, positive synergistic interactions in two, neutral effects in five, and a concentration-sensitive relationship in three.
There have been a few published studies of the indirect effect of transgenic Bt-producing crops on natural enemies. Depending on the species involved some studies reported no significant effects (for example, Hough-Goldstein and Keil 1991), but others have found adverse effects. Giroux et al. (1994) reported that the ladybug predator of the Colorado potato beetle consumed fewer potato beetle eggs when potato Bt levels were high. Hillbeck et al. (1998a, b) found that when chrysopid larvae were reared on prey that were fed Bt-producing corn, they suffered 62% mortality. When they were reared on prey that were fed non-Bt corn, mortality was only 37%. Those results were found with two prey species, one sensitive to Bt and the other insensitive. The results indicate that it was the Bt toxin in the bodies of the prey, and not simply unhealthy prey, that caused the heightened mortality.
It is important to ask whether such indirect effects will have a harmful effect on the agroecosystem. In some cases, the use of conventional pest-protected plants has lead to decreased foraging efficiency of predators and parasitoids (Boethel and Eikenbary 1986). A few field studies have attempted to measure the effect of transgenic Bt potatoes on the diversity of insects in crop fields (Hoy et al. 1998). In one 4-year study at five locations in the Wisconsin potato-production system, it was found that populations of predators were, on the average, 63.8% lower in fields where non-Bt potatoes were managed with typical insecticides than in fields of transgenic Bt potatoes. Parasitoid populations in the chemically treated potato fields were, on the average, 58.4% lower than in Bt potato fields. Insecticidal treatment of potatoes to control Colorado potato beetles with conventional insecticides often results in secondary outbreaks of aphids because the aphids are released from biological control. In three of the four years of the study, aphid populations (which are not affected by Bt toxins) were lower in the transgenic Bt potato fields than in chemically treated potato fields, presumably because of greater biological control.
A study of potatoes in Ohio reported similar results (Hoy et al. 1998). In potato fields treated with pyrethroid insecticide to manage Colorado
potato beetles, natural enemies were reduced to undetectable levels. This resulted in aphid outbreaks that could be only marginally controlled by insecticides (253 aphids per meter of row in late July). In the transgenic Bt potatoes where natural enemies were always detectable (1 to 4 per meter), the aphid density was very low (below 10 per meter).
Those two studies compared the impacts of chemically-intensive potato farming and farming with transgenic Bt-potato. No comparison with a no-chemical control treatment was presented, but it might have been inappropriate. Another study provided to the committee indicated that there were no significant differences in beneficial arthropods between plots in which transgenic Bt potatoes or microbial Bt sprays were used (Feldman et al. 1994). Both had similar densities of beneficial arthropods, while plots in which chemical insecticides were used had much lower densities of some of these arthropods (Feldman et al. 1994).
In most of the areas where Bt potatoes will be used commercially, the crop is usually protected against the target Colorado potato beetle with conventional pesticides. In the corn system, most of the acreage has not been treated for the European corn borer, the major target pest of the Bt corn cultivars, because the insect feeds mostly within the plant stem where pesticides are typically not effective. In addition, pesticide treatment is more expensive than the yield losses due to the borer. In this case, the appropriate comparison of environmental impacts of the transgenic Bt cultivars would be with a system where non-Bt corn was not treated with pesticides aimed at the European corn borer.
It is useful to ask what will happen to the long-term biodiversity of agroecosytems if biotechnology provides us with crops that are constitutively toxic to almost all insect herbivores. A study of insecticide effects on quail populations in soybean fields provides interesting insight. Palmer et al. (1998) found no physiologic effects of the pesticide residues on the quail. However, quail in sprayed fields had lower weight gain and lower survival than those in control fields because there were fewer insects to feed on (Palmer 1995). If in the future we continue to commercialize pest-protected crops that constitutively express more diverse insect-specific toxins, we could inadvertantly produce crops that lower general herbivore abundance. This could result in lower biodiversity of species at higher trophic levels that depend on herbivorous insects as food.
2.6.4 Summary
Conventional and transgenic pest-protected crops can adversely affect nontarget organisms through direct contact with or ingestion of the plant or pollen by the nontarget organisms and through indirect contact when the pest-protective substances (or their effects) are passed to other
trophic levels that are consumed by nontarget organisms. However, the committee found that
Both conventional and transgenic pest-protected crops could have effects on nontarget species, but these potential impacts on nontarget organisms are generally expected to be smaller than the impacts of broad-spectrum synthetic insecticides, and therefore, the use of pest-protected plants could lead to greater biodiversity in agroecosystems where they replace the use of those insecticides.
Current criteria for commercialization of transgenic pest-protected plants includes several laboratory toxicity tests for nontarget organisms (see appendix B and section 3.1.2). In light of the above discussions, more field evaluations should be conducted to determine the impacts of specific pest-protected crops on nontarget organisms, compared to impacts of standard and alternative agricultural practices. The committee recommends that
Criteria for evaluating the merit of commercializing a new transgenic pest-protected plant should include the anticipated impacts on nontarget organisms compared with those of currently used5 pest control techniques.
2.7 GENE FLOW FROM TRANSGENIC PEST-PROTECTED PLANTS
Genes from one crop plant may be spread to other plants of the same or related species when pollen is transported by wind, bees, or other animal pollinators. Genes have been flowing from crops to weedy relatives of crop plants for centuries. Now it is also possible for fitness-enhancing transgenes to spread to weed populations. In this report, the committee uses the terms “weedy” and “invasive” in reference to plants that are unwanted in human-dominated or natural habitats. Many people think of weeds primarily as undesirable plants that infest agricultural fields, tree plantations, lawns, and other managed areas. However, natural and semi-natural habitats such as wetlands, coastal dunes, and rangelands are also harmed by the spread of weedy species. Weedy plants quickly colonize open space and may displace non-weedy species, as has occurred with kudzu, Scotch broom, spotted knapweed, and purple loosestrife, for example. Once established, these types of plants are often difficult to eradicate. Annual species release large quantities of long-
5 |
Includes both chemical and non-chemical methods which are currently used. |
lived seeds that persist in the soil, while perennial species can be difficult to kill with herbicides, especially when they occur outside the realm of farmers' fields. Although only a small percentage of the weedy and invasive species in the United States are capable of crossing with cultivated plants, these species merit special attention with regard to crop-to-wild gene flow (NRC 1989). In addition, species that are not currently designated as weeds could potentially become more difficult to control if their populations are released from key ecological constraints (for example, herbivory, disease, or drought stress). This process is discussed further in the 1989 NRC book on field testing genetically engineered organisms.
The 1989 NRC report noted that “the potential for enhanced weediness is the major environmental risk perceived for introductions of genetically modified plants” (NRC 1989, p. 3). To evaluate problems possibly associated with gene flow from transgenic pest-protected plants, it would be useful to know how much pollen is dispersed from a crop, how far it travels, and whether its genes persist in wild populations. It would also be useful to know whether the spread of transgenes via pollen can lead to increased invasiveness in wild relatives of the crop.
Misinformation about these issues causes people to regard gene flow itself as a threat to the environment. Gene flow is indeed common, but the process of gene flow is important only if it leads to undesirable consequences, such as the spread of transgenes that are advantageous to weeds. In other words, the process of gene flow does not pose a hazard itself, but the consequences of gene flow might. Gene flow is the process by which exposure can occur (section 2.1). The consequences or potential hazards are dependent on the nature of the transferred trait, its level of expression in the recipient plant, and the biology and ecology of the recipient plant. The fact that the plant is genetically modified generally does not affect the process of gene flow or pollen dispersal.
In this section, the committee highlights the scientific dimensions of gene flow and its potential consequences. A few examples of transgenic pest-protected plants from which the consequences of gene flow are serious enough to merit special attention with regard to the weediness of wild relatives are also highlighted. The committee also describes the extent of gene flow from transgenic crops to organically grown crops of the same species. Scientific guidance as it relates to evaluating the consequence of gene flow and the review of gene flow for environmental safety assessments are presented in sections 3.1.4 and 3.3.
2.7.1 Pollen Dispersal and Outcrossing
The distance that pollen moves varies widely among species. Some crops—such as rice, wheat, and soybean—are mostly self-pollinated (selfed). In sorghum, most seeds result from self-pollination, and the rest
TABLE 2.1 Isolation distances required by USDA for producing foundation seed used for seed increase. Note that this is not a complete list of all crops
Crop Speciesa |
Distance, ft |
Maximal Proportion Contaiminated, %b |
No isolation required: |
||
Barley |
0 |
0.05 |
Bean, field and garden |
0 |
0.05 |
Broad bean |
0 |
0.05 |
Cotton |
0 |
0.03 |
Flax |
0 |
0.05 |
Millet, selfed |
0 |
0.05 |
Mung bean |
0 |
0.10 |
Oat |
0 |
0.02 |
Pea, field |
0 |
0.50 |
Peanut |
0 |
0.10 |
Soybean |
0 |
0.10 |
Triticale |
0 |
0.05 |
Wheat |
0 |
0.50 |
Isolation Required: |
||
Alfalfa |
600 |
0.10 |
Buckwheat |
660 |
0.05 |
Clover, < 2 ha |
600 |
0.10 |
Clover, > 2 ha |
900 |
0.10 |
Corn |
660 |
0.10 |
Crown vetch, < 2 ha |
200 |
0.10 |
Crown vetch, > 2 ha |
900 |
0.10 |
Grasses, cross-pollinated |
900 |
0.10 |
Grasses, selfed |
60 |
0.10 |
Lespedeza |
10 |
0.10 |
Millet, cross-pollinated |
1,320 |
0.005 |
Mustard |
1,320 |
0.05 |
Okra |
1,320 |
0.0 |
Onion |
5,280 |
0.0 |
Pepper |
200 |
0.0 |
Rape, cross-pollinated |
1,320 |
0.05 |
Rape, selfed |
660 |
0.05 |
Rice |
10 |
0.05 |
Rye |
660 |
0.05 |
Safflower |
1,320 |
0.01 |
Sorghum |
900 |
0.005 |
Sunflower |
2,640 |
0.02 |
Tobacco |
150 |
0.01 |
Tomato |
200 |
0.0 |
Trefoil, birdsfoot |
600 |
0.10 |
Vetch |
10 |
0.10 |
Vetch, milk |
600 |
0.05 |
Watermelon |
2,640 |
0.0 |
aCommon name. bMaximal percentage produced from pollen outside plot. Source: From regulations listed in table 5 of USDA (1994a). |
come from outcrossing or cross-fertilization among plants via wind pollination (Arriola and Ellstrand 1996; Ellstrand and Foster 1983). Oilseed rape (canola) also produces a mixture of selfed and outcrossed seeds, whereas corn, carrot, sunflower, poplar, radish, strawberry, clover, and many other species produce most of their seeds by outcrossing rather than selfing (for example, Brown et al. 1985; Free 1970; Richards 1986). By definition, outcrossers disperse pollen to other plants and therefore need greater isolation distances than selfing species in situations where pollen dispersal is considered undesirable ( table 2.1). Wind-pollinated outcrossers, such as many trees and grasses, tend to produce larger quantities of pollen (causing problems for people with pollen allergies) than similar-size plants that are pollinated mainly by insects and other animals. Pollen from animal-pollinated plants is not as buoyant in air currents, and very little is shed into the air; these types of pollen adhere to the pollinator's body. Pollen grains of a few species, such as sugar beet and oilseed rape, are dispersed by both wind and insects (Cresswell et al. 1995; Free et al. 1975; McCartney and Lacey 1991).
The amount of pollen dispersed from outcrossing plants declines rapidly as a function of distance from the source (section 2.6.2, figure 2.2). In the corn example, the amount of pollen deposited at 60 m was only 0.02% of the amount deposited 1 m from the edge of the crop, but this still represents 2,500 pollen grains per square meter (Raynor et al. 1972). Most pollen is deposited within a few meters of its source, but a small proportion can be carried more than 1 km away (for example, Arias and Rieseberg 1994; Kirkpatrick and Wilson 1988; Klinger et al. 1992). Such long-distance gene flow is not easy to measure, because very large samples are needed to detect low-probability events. In addition, the extent of long-distance gene flow is highly variable and depends on local conditions, the relative sizes of donor and recipient populations, and synchrony of flowering. Once pollen from a crop has spread to wild plants, further gene flow occurs in a ripple effect through both pollen and seed dispersal.
2.7.2 Crop-to-Wild Gene Flow
The escape of novel resistance traits into free-living populations of wild relatives is often cited as an undesirable consequence of growing transgenic crops on a commercial scale (for example, Bergelson et al. in press; Ellstrand and Hoffman 1990; NRC 1989; Parker and Kareiva 1996; Raybould and Gray 1998; Rissler and Mellon 1996; Snow and Morán-Palma 1997; Tiedje et al. 1989; Van Raamsdonk and Schouten 1997). Concerns arise mainly when novel, beneficial traits have the potential to spread to wild populations and cause them to become more invasive and difficult to control. Naturally-occurring damage by insects and diseases
can regulate the density and distribution of populations of wild relatives, so release from these pressures by genes from a cultivated relative could potentially make weeds more troublesome in agricultural fields. Moreover, many wild or weedy relatives also occur in other habitats, including rangeland, roadsides, forests, wetlands, and other natural areas where they are not controlled with weed-management techniques, such as the use of herbicides, mowing, and tilling.
Conventional crop genes have spread to wild populations in the past (for example, Arriola and Ellstrand 1996; Ellstrand et al. 1999; Linder et al. 1998; Small 1984), but this process has not been studied by weed scientists, and it is not known whether it has facilitated evolutionary adaptations in weedy relatives (Small 1984; Snow et al. 1998). Circumstantial evidence suggests that several weedy species such as Johnson grass have become more robust and abundant as a result of hybridization with crops (NRC 1989). Known problems have involved hybridization between a weed and a crop, often due to range expansions of one species or the other (Ellstrand et al. 1999; Rissler and Mellon 1996), rather than a transfer of few novel crop genes within an existing crop-weed complex. A well-documented example of a new weed problem occurred in France: a wild gene for early bolting spread from sugar beet seed nurseries. Seeds from those nurseries were then grown by farmers and new weed populations resulted. This led to serious economic losses (Boudry et al. 1993). Scientists have viewed wild relatives mainly as sources of genes that could affect crops, either as useful germplasm or as undesirable pollen contamination; thus, there is little empirical evidence of whether wild species have benefited or not from crop-to-wild gene flow.
Recently, weed scientists have redoubled their efforts to quantify genetic diversity in weed populations and to gain a better understanding of how weeds adapt to changing conditions. The commercialization of transgenic crops has provided added incentives for studies on crop-to-wild gene flow because some transgenic phenotypes have never occurred in wild relatives of certain crops. For example, wild sunflowers lack protection against their seed-eating insects and a fungal disease known as Sclerotinia rot (Seiler 1992). If transgenic methods introduced protectants against these pests into cultivated sunflowers, the genes that code for these protectants could move into wild sunflowers, perhaps increasing their ecological fitness and abundance. Likewise, genetic resistance to the herbicide glyphosate (RoundupTM) has not been found in the germplasm of crop relatives, but this trait could be transmitted to wild or weedy relatives of transgenic, glyphosate-resistant crops. The use of a wider and more effective variety of transgenic methods could increase the rate at which weedy species acquire novel types of strong, single-gene resistance via crop-wild hybridization.
A first step toward assessing the consequences of crop-to-wild gene flow is to determine which cultivated species are capable of crossing with wild relatives (table 2.2). Corn, soybean, tomato, and cotton do not escape cultivation, nor do they have many close wild relatives within the continental United States (except for cotton in southern Florida). In contrast, some cultivated species survive in feral populations or cross with wild plants of the same species (for example, carrot, sunflower, squash, rice, poplar, certain grasses, oilseed rape, radish, and beet). Many crops have weedy ancestors or occur as weeds in portions of their worldwide distribution (Colwell et al. 1985; Ellstrand et al. 1999; Small 1984). Hybridization between different species or genera is sometimes possible, especially if they share a close, common ancestry. Spontaneous hybridization can occur even when the two taxa have unequal chromosome numbers, which typically creates problems during cell division (for example, Mikkelsen et al. 1996; Zemetra et al. 1998). In fact, new molecular methods have shown that gene flow among related species is much more common than was previously thought (for example, Arnold 1997). Genes often spread among species without being detected by visible indicators, so genetic markers, such as unique DNA fragments are needed to identify current and historical patterns of crop-to-wild gene flow. Recent studies have augmented the number of plant groups in which interspecific hybridization is known to occur naturally, as in oaks, birches, orchids, irises, and wild gourds. The information derived from studies of ongoing gene flow could also be used to understand what types of genes and traits move between these species, thus providing a basis to predict whether specific traits would raise concern if the genes/traits were introduced and transferred.
Early generations of crop-wild hybrids generally have lower fertility than their parents, but there can be high variability among the fertility of the hybrids, with some having high fertility. Even those which produce few viable gametes can transmit crop genes to later generations (a process known as introgression). With each successive generation of backcrossing with wild genotypes, the crop's contribution to the plant's total genome is reduced by 50% (figure 2.3) and the progeny become more similar to wild genotypes. After two or three generations of backcrossing, plants with a crop ancestor can be just as competitive and successful as wild plants (for example, Snow et al. 1998). Moreover, the fitness of first-generation crop-wild hybrids is sometime as great as the fitness of wild plants (for example, Arriola and Ellstrand 1997; Klinger and Ellstrand 1994).
The frequency of a given crop gene in a wild population depends on many factors, including the rate at which it is introduced into the population; temporary fitness barriers, if any, in the first and early backcross generations; possible fitness costs associated with the gene itself; and
TABLE 2.2 Examples of Commercially Important Species That Can Hybridize with Wild Relatives in the Continental United States
Family and Cultivated Speciesa |
Wild Relativea |
||
Apiaceae |
|||
Apium graveolens (celery) |
Same species |
||
Daucus carota (carrot) |
Same species (wild carrot) |
||
Chenopodiaceae |
|||
Beta vulgaris (beet) |
B. vulgaris var. maritima (hybrid is a weed) |
||
Chenopodium quinoa (quinua, a grain) |
C. berlandieri |
||
Compositae |
|||
Chicorium intybus (chicory) |
Same species |
||
Helianthus annuus (sunflower) |
Same species |
||
Lactuca sativa (lettuce) |
L. serriola (wild lettuce) |
||
Cruciferae |
|||
Brassica napus (oilseed rape;canola)b |
Same species, B. campestris, B. juncea |
||
Brassica rapa (turnip) |
Same species (= B. campestris) |
||
Raphanus sativus (radish) |
Same species, R. raphanistrum |
||
Cucurbitaceae |
|||
Cucurbita pepo (squash) |
Same species (= C. texana, Wild squash) |
||
Ericaceae |
|||
Vaccinium macrocarpon (cranberry) |
Same species |
||
Vaccinium angustifolium (blueberry) |
Same species |
||
Fabaceae |
|||
Trifolium spp. (clover) |
Same species |
||
Medicago sativa (alfalfa) |
Same species |
||
Hamamelidaceae |
|||
Liquidambar styraciflua (sweetgum) |
Same species |
||
Juglandaceae |
|||
Juglans regia (walnut) |
J. hindsii |
||
Liliaceae |
|||
Asparagus officinalis (asparagus) |
Same species |
||
Pinaceae |
|||
Picea glauca (spruce) |
Same species |
||
Poaceae |
|||
Avena sativa (oat) |
A. fatua (wild oats) |
||
Cynodon dactylon (bermuda grass) |
Same species |
||
Oryza sativa (rice) |
Same species & others (red rice) |
||
Saccharum officinarum (sugar cane)c |
S. spontaneum (wild sugarcane) |
||
Sorghum bicolor (sorghum) |
S. halepense (johnsongrass) |
||
Same Speciesd (shattercane) |
|||
Triticum aestivum (wheat) |
Aegilops cylindrica (jointed goatgrass)e |
||
Rosaceae |
|||
Amelanchier laevis (serviceberry) |
Same species |
||
Fragaria sp. (strawberry) |
Fragaria virginiana |
||
Rubus spp. (raspberry, blackberry) |
Same species |
||
Salicaceae |
|||
Populus alba x P. grandidentata (poplar) |
Populus spp. |
||
Solanaceae |
|||
Nicotiana tabacum (tobacco) |
Same species |
||
Vitaceae |
|||
Vitis vinifera (grape) |
Vitis spp. (wild grape) |
||
a Wild relatives recognized as weeds (unwanted species in agricultural or other habitats) are underlined; those also included in the worst 100 weeds worldwide (Holm et al. 1997) or Federal Noxious Weed List are in boldface. This list is not exhaustive, especially for landscaping and forage species. For some cultivars the extent of hybridization has not been studied. b Also hybridizes with Raphanus raphanistrum, but evidence to date suggests that crop chromosomes do not recombine with wild genome and are lost after several generations (Chevre et al. 1997; Chevre et al. 1999). c Cultivated sugar cane does not need to flower before harvest, but hybrids can occur (Stevenson 1965). d From Burnside (1968). e From Zemetra et al. (1998). Source: Adapted from Snow and Morán-Palma (1997). |
The ecological and evolutionary benefits conferred by crop genes that
possible benefits of the gene for the plant's survival and reproduction. Fitness costs associated with transgenes may be small or absent when a seed is ready to be sold, because seed companies choose breeding lines with the best transformation events available (for example, Fredshavn et al. 1995). When several transgenes are inserted together as tightly linked traits (inherited as a unit), the combined ecological costs and benefits of these traits will determine whether and to what extent a wild relative's fitness is enhanced. If one transgene confers resistance to a common herbicide, such as glyphosate, selective pressures favoring plants with the transgene will be very strong when that particular herbicide is used, thereby increasing frequencies of the linked transgene in weed populations. Eventually, wild species will be able to acquire additional beneficial transgenes that are released in different cultivars, and the new traits could accumulate in wild populations.
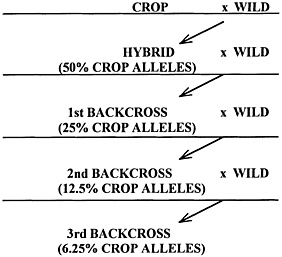
FIGURE 2.3 Dilution of crop alleles in plant genotypes after spontaneous hybridization and backcrossing with wild plants. Two parent plants are shown on each line (for example, crop x wild), with an arrow pointing to their offspring.
enter wild populations are difficult to evaluate. This is an issue that lies at the crux of concerns about gene flow from transgenic and conventional crops. We know little about the extent to which insects and diseases limit wild, weedy populations that are sexually compatible with cultivated species. Critics of biotechnology argue that the spread of beneficial traits could quickly lead to the spread of weeds; advocates of transgenic crops maintain that this risk is small or nonexistent. Empirical data with which to address the question are lacking. Many publications describe proposed methods for evaluating the effects of beneficial crop genes on the dynamics of wild, weedy populations (for example, Crawley et al. 1993; Kareiva et al. 1996; Rissler and Mellon 1996). However, inadequate funding and restrictions on trial releases of transgenic pest-protected plants have hampered opportunities to carry out this important research before commercialization (Wrubel et al. 1992; Purrington and Bergelson 1995). A few studies have focused on how novel genes affect plant fitness, (that is, the relative survival and reproduction of new genotypes) (for example, Bartsch et al. 1996; Stewart et al. 1997), but it is much more difficult to determine how new traits will affect the geographic distribution and local abundances of a given species. The latter requires a combination of field studies, field experiments, and mathematical modeling (for example, Rees and Paynter 1997).
Until better data are available, it will be necessary to rely on general ecological and agricultural knowledge to predict the consequences of com-
mercial-scale, crop-to-wild gene flow from pest-protected plants. The common perception that most wild plants are adapted to tolerate or resist pests is misleading in that even species that appear to be protected by mechanical or chemical defenses can be decimated by specialist herbivores or new diseases. Many studies have demonstrated that herbivores, especially insects that specialize on flowers and seeds, can regulate wild plant populations (for example, Guretzky and Louda 1997; Rees and Paytner 1997; Waloff and Richards 1977). In contrast, much less is known about the extent to which diseases affect the population dynamics of wild plants (Burdon 1987; Burdon and Jarosz 1988). The impact of diseases is much more obvious in cultivated species, especially genetically uniform plants grown at high densities. However in natural populations, where nonspecific plants are often scattered and genetically diverse, disease could still be important. Wild plants are susceptible to many native, introduced, and newly evolved diseases, but we know little about how often they are exposed to these pathogens and whether such exposure constrains population growth. Although general surveys of disease frequencies in wild populations are helpful, they might miss infections that occur inconspicuously (in seeds, seedlings, or roots) or infrequently, and those which are so virulent that they cause local extinctions.
Because of the uncertainties described above, it is premature to predict the ecological impacts of gene flow from transgenic pest-protected plants. Meanwhile, regulatory decisions must be made in a timely fashion. It seems unlikely that the transfer of one or two novel crop genes for pest-protection would transform a wild species into a problematic weed, although in some cases unwanted population increases of weedy species could result. Moreover, the cumulative effects of beneficial crop genes could potentially lead to expensive and ecologically damaging problems in weeds that are already difficult to control, such as Johnson grass (Sorghum halepense). In the future, additional phenotypic traits might include broad-spectrum resistance to insects or diseases and greater tolerance of cold, drought, salinity, nutrient scarcity, or acidic soils. Such traits could be more advantageous to wild relatives than those now in use.
Consequences of gene flow other than weediness are also perceived to be detrimental to preserving biodiversity. For example, the spread of transgenes to wild relatives that are rare or endangered is sometimes considered as a potential ecological risk, especially in regions that are centers of diversity for crop relatives (for example, Rissler and Mellon 1996). However, if a portion of wild species's genome is being exchanged for new genetic material by hybridization, this process will occur whether or not the crop is transgenic. The transfer of resistance traits to rare wild relatives is unlikely to exacerbate population declines or lower genetic diversity in these species, given that transfer of traits between crops and
wild relatives is an ongoing process. However, researchers and regulators should be aware of these and other potential unintended consequences of gene flow from transgenic pest-protected plants (for example, the hypothetical spread of a Bt gene from an ornamental species to the only host plant of a rare beetle).
Eventually, it could be possible to reduce gene flow from cultivated plants with various containment methods. If a transgene is inserted into the chloroplast genome, which is usually not transmitted by pollen, spread of the transgene into natural populations could be delayed (Daniell et al. 1998) but would not be avoided entirely (Stewart and Prakesh 1998). Gressel (1999) suggested linking transgenes to genes for traits that are detrimental to weeds but not crops (for example, a gene for lack of seed dormancy). Again, this would slow down the process of introgression but would not necessarily provide complete containment of ecologically beneficial transgenes. A more effective method of confining gene flow may be to use a system in which transgenic seeds are killed just before they mature, so that they can still be harvested but will not germinate (US Patent No. 5,723,765, “Control of plant gene expression”). Popularly known as “terminator” technology, this potential method of containment is highly controversial and has not yet been used commercially. In the future, biotechnology companies might develop transgenic plants with inducible pest resistance traits that require a chemical spray to be “turned on.” If these systems work as predicted, resistance genes that spread to wild species would not be expressed in the wild species unless the spray was used, and therefore, would be extremely unlikely to contribute to the wild species' invasiveness. Because of the concerns raised about using chemicals to turn on genes in the environment, it is unclear whether or not such technology will be acceptable. However, if this strategy is pursued on a commercial scale, it could greatly reduce the need to regulate transgenic crops based on weedy-relative considerations.
2.7.3 Crop-to-Crop Gene Flow
Crop breeders try to minimize gene flow into their breeding lines by using crop-specific isolation distances (for example, Bateman 1947a, b, c). Seeds used for commercial sale are often mandated to have less than 0.1% contamination from phenotypically different sources (table 2.1). Less stringent genetic isolation is required for producing certified seeds to sell to farmers, and only rarely is any isolation required when farmers grow a crop for harvest. Now, however, organic farmers are concerned about gene flow from transgenic plants as organic standards may forbid the use of transgenic products. Under proposed standards, organic farmers are not allowed to sell transgenic food, and a tolerance for very low levels of
contamination by transgenic DNA has not been established (NOSB 1996). The committee knows of no scientific evidence that crop-to-crop gene flow has caused health or environmental risks to date, but we recognize that consumer choices are involved. Nonorganic farmers might also face problems with crop-to-crop gene flow and undesirable seed characteristics —for example, if transgenic crops are commercially developed for pharmaceutical or industrial compounds, or when farmers' fields are inspected in search of the unauthorized use of patented transgenic genotypes. Clearly, these hypothetical concerns apply to a variety of transgenic crops. Contamination with pollen from other farms is likely to be very low in most cases, as illustrated in figure 2.2 and table 2.1 and described further below.
Crop-to-crop gene flow is unlikely to occur over long distances in species with high selfing rates, although even rice, sorghum, and wheat are known to hybridize occasionally with nearby plants (Arriola and Ellstrand 1996; Langevin et al. 1990; Seefeldt et al. 1998; Zemetra et al. 1998). Pollen from outcrossing species could easily move 600 ft or more among plantings (table 2.1). In a study of oilseed rape fields in Scotland, researchers documented a small amount of pollen dispersal as far as 3 km from the crop, indicating that farm-to-farm spread of transgenes was common (Thompson et al. 1999). In that region, over 60% of the crop plantings occurred within 100 m of another crop planting. Gene flow into row crops can also occur via volunteer crop plants that survive to reproduce and via feral plants that start new populations, for example, when sunflower or oilseed rape seeds are inadvertently spilled along roadsides. Even for selfing species, total containment of crop genes is not considered to be feasible when seeds are distributed and grown on a commercial scale. The inevitability of gene escape has been recognized by federal agencies, and regulatory decisions are based on it.
In some situations, unwanted levels of contamination can be avoided by altering the distances among plots, using border rows to intercept unwanted pollen, or planting large acreages to minimize edge effects (for example, figure 2.2 and table 2.1; Hokanson et al. 1997; Morris et al. 1994). These approaches have been discussed and recommended by the USDA for field testing of transgenic plants products prior to the regulatory approval of these products (USDA 1993; USDA 1996a). Figure 2.4 illustrates the general principle that small populations are more susceptible to contamination from long-distance pollen sources than are large populations (for example, Ellstrand and Elam 1993; but see Klinger et al. 1992). For outcrossing cultivated plants, contamination of less than 1% of the seeds in a given crop can often be prevented by isolation distances of over 1 km, but the threshold distance depends on the species, the relative sizes of donor and recipient plantings, use of border rows, and overlap in flowering times.
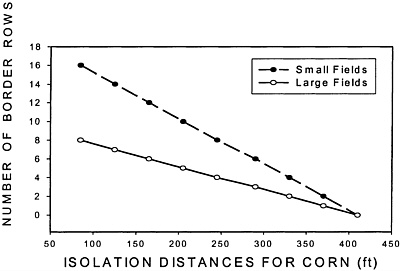
FIGURE 2.4. Number of border rows recommended to keep “off-type” seeds in corn fields under 0.5% as a function of isolation distance from other sources in corn fields. These requirements apply to contaminating pollen from sources with kernels that closely resemble the crop; therefore, “under 0.5%” does not refer to actual gene flow, but rather to acceptable levels of gene flow for producing certified seeds of standard quality. Small fields are defined as less than 20 acres; large fields are more than 20 acres. Source: From seed certification regulations listed in USDA 1994a.
2.7.4 Summary
In light of the above discussions, the committee found that
Pollen dispersal can lead to gene flow among cultivars and from cultivars to wild relatives, but only trace amounts of pollen are typically dispersed further than a few hundred feet.
The transfer of resistance traits to weedy relatives could potentially exacerbate weed problems, but such problems have not been observed or adequately studied.
The committee recommends that
Criteria for evaluating the merit of commercializing a new transgenic pest-protected plant should include whether gene flow to feral plants or wild relatives is likely to have a significant impact on these populations.
In order to study the effects of gene flow, the committee recommends areas for research in chapter 3 and the executive summary (section 3.4 and section ES.5.2).
2.8 AGRONOMIC RISKS ASSOCIATED WITH VIRUS-RESISTANT CROPS
Agronomic risks are defined as those related to quality or productivity of a modified crop. A number of issues and concerns emerge when agronomic consequences of using transgenic pest-protection strategies against viruses are considered. These center on emergence of new or novel viral strains, introduction of new transmission characteristics, and changes in susceptibility to heterologous viruses. Some of the concerns, such as the question of new virus emergence, also have relevance to ecological risks.
2.8.1 Evolution of Resistance to Pest-Protected Crops
The emergence of strains of pathogens that overcome plant-genetic resistance or other disease-control methods has been and probably always will be a problem in agriculture. Indeed, this problem is common to all plant and animal hosts for which pathogens exist. Traditionally the problem has been managed by development of multiple strategies for disease control (genetic and other control measures), surveillance of pathogen activity and strain development, deployment of new pest-protected germplasm in response to emerging pest strains, and development and use of longer-lasting forms of pest-protection. With transgenic pest-protected plants that express a pest-protection gene transferred from another plant, the selective pressure for development of resistance-breaking strains should be qualitatively similar to the selective pressure associated with conventionally bred pest-protected plants. In section 2.9, these issues are discussed.
2.8.2 Risks Posed by Virus-Derived Transgenes
Recombination Between Transgenes and Viral Pathogens
Recombination between a virus-derived transgene and a virus during plant infection has been suggested as a potential source of novel virus strains with enhanced virulence characteristics. From an evolutionary perspective, new viruses emerge through gradual accumulation of point mutations and major acquisition or deletion of genetic material. New genetic material can be incorporated by recombination with nucleic acids
from the host or from other viruses during coinfections (Koonin and Dolja 1993). Several laboratory studies have shown that viruses can recombine with homologous transgene sequences (Borja et al. 1999; Greene and Allison 1994; Wintermantel and Schoelz 1996). Those studies required moderate or heavy selection pressure to detect the recombinant viruses. In all cases examined, only homologous sequences were exchanged. No experimental data indicate that recombination can occur between virus genomes and transgene sequences that are derived from distantly related or unrelated viruses.
Two points should be considered in assessing risks that may be posed by virus-transgene recombination. First, will large-scale plantings of transgenic material increase the risk of recombination above the preexisting risk due to the widespread occurrence of mixed infections? Mixed infections by related and unrelated viruses are common in natural and agricultural ecosystems. For example, mixed infections by two or more viruses were detected in 64% and 90% of peppers surveyed in three California counties in 1984 and 1985, respectively (Abdalla et al. 1991). Mixed infections provide a continuous opportunity for intervirus recombination. Either because intervirus recombination occurs with such low frequency or because new recombinants so rarely have a competitive advantage, the new viruses have not been detected in agricultural settings. One could argue that past and current agricultural practices have provided a fertile environment to spawn novel recombinant viruses with virulent properties, but these viruses have not been observed.
Second, can transgenes be engineered to reduce or eliminate the risk that recombination will spawn new pathogens? Evidence suggests that elimination of genome replication-control sequences from transgenes can limit recombination and therefore risk (Greene and Allison 1996). Furthermore, strategies to produce resistance-mediating transgenes that encode nonfunction proteins or no protein can be used effectively against viruses. For example, resistant plants that express nontranslatable RNA can confer immunity through induction of post-transcriptional gene silencing (Kasschau and Carrington 1998; Lindbo and Dougherty 1992).
Transcapsidation and Gain-of-Transmission Characters
In the process of encapsidation, a virus genome is packaged in a shell of self-encoded coat proteins after it is replicated. Although encapsidation of one virus genome by the coat protein from a different virus (transcapsidation) is well documented (Matthews 1991), it is highly unlikely that functional coat proteins expressed in transgenic plants pose a significant risk of expansion of host range to new crop or non-crop hosts. Transcapsidation does not involve exchange of genetic material, meaning
that any unique insect vectoring properties of a transcapsidated virus genome will not be inherited.
Synergism Between Viral Transgenes and Heterologous Viruses
Mixed infections by viruses can sometimes lead to a synergistic disease syndrome that is more severe than that caused by either virus individually (Matthews 1991). In cases of synergism involving the potyvirus family of viruses, the region of the viral genome that causes exacerbation of disease codes for a protein termed HC-Pro (Pruss et al. 1997). The synergism effect appears due to the natural role of HC-Pro as a suppressor of the gene-silencing response (Scheid 1999). Thus, plants are unable to mount an effective defense response to infection. Indeed, transgenic plants that express potyvirus genome segments that include HC-Pro exhibit more severe symptoms when inoculated with heterologous viruses (Pruss et al. 1997). No data indicate that expression of viral coat protein or replicase proteins enhances the virulence of heterologous viruses.
The problem of synergism is manageable through avoiding the use of functional transgenes that encode defense-suppressor substances or pathogenicity-enhancer substances. In addition, the normal process of testing in breeding programs that seek to incorporate natural or transgenic resistance traits will reveal the extent to which the virulence of heterologous viruses is exacerbated. Thus, it is highly unlikely that transgenic plants with general hyper susceptibility characteristics will pass through a breeding program to commercialization.
2.8.3 Summary
In light of the above analysis, the committee found that
Most virus-derived resistance genes are unlikely to present unusual or unmanageable problems that differ from those associated with traditional breeding for virus resistance.
Case studies of virus resistant squash and papaya are presented in chapter 3 (section 3.1.4).
2.9 PEST RESISTANCE TO PEST-PROTECTED PLANTS AND RESISTANCE MANAGEMENT
In this section, the ability of pests to adapt and develop resistance to transgenic or conventional pest-protected plants will be discussed, and resistance management strategies to abate this development and their scientific basis will be presented.
2.9.1 Pest Resistance to Control Tactics
The history of agricultural pest management has demonstrated that insects, weeds, and microbial pathogens have the evolutionary potential to overcome or circumvent most control tactics imposed by farmers (Barrett 1983; Green et al. 1990; Gould et al. 1991). That over 400 insect species have become resistant to at least one insecticide (Georghiou 1986) is often cited as evidence of the genetic ability of arthropods to evolve resistant strains. In addition, weeds and pathogens also have an impressive record of successful adaptation to control measures (Green et al. 1990). Although the number of cases of resistance by weeds to herbicides is smaller than that of insects, the percentage of weed species that has developed resistance is greater than that of insects (Gould 1995). It is well documented that microbial pathogens can successfully adapt to crop cultivars that are bred to resist specific diseases (Lamberti et al. 1981). In examining a random selection of 63 cases of viruses that live in association with specific plant hosts, Fraser (1990) found that in 28 of the cases there were good data indicating that adapted isolates of the virus existed (in only five cases was there good evidence that there had been no adaptation by the virus). Fungal and bacterial adaptation to pest-protected cultivars has caused serious crop losses. In many cases, pathogen resistance has occurred less than 5 years after a classically bred resistant cultivar was released for commercial use (see section 3.1.1). Experience with both insect and pathogen adaptation to genetically modified pest-protected (GMPP)6plants indicates that the more intensively a control tactic is used, the more rapidly pests will adapt to it (Gould et al. 1991). History also indicates that pests adapt more rapidly to some types of GMPP plants than to others (Lamberti et al. 1981).
If a GMPP cultivar is lost because the target pest adapts to the cultivar, replacing the cultivar with a new GMPP cultivar can have a number of associated costs. Even if new GMPP genes are available, moving those genes into a modern cultivar is expensive. Although the health and environmental safety of the plant protectant in the new cultivar can be tested in laboratory experiments, the new cultivar will need to be monitored for impacts that could not have been detected in the laboratory experiments. If new pest-protection genes are not available, farmers might need to move back to reliance on broad-spectrum pesticides. Decreasing the rate at which target pests adapt to GMPP cultivars can therefore produce societal benefits.
6 |
As a reminder to the reader, GMPP plants include both transgenic and conventional pest-protected plants. See section ES.3. |
2.9.2 Decrease in the Rate of Resistance Evolution
Concern over the risk of pest resistance to conventional pesticides led to development of a relatively new field of applied science, called pest-resistance management (NRC 1986). The goal of this field is to determine approaches for developing and deploying pest control tactics in ways that maximize long-term benefits. Pest-resistance management is grounded in concepts and empirical findings from the basic sciences of quantitative genetics and population genetics (NRC 1986). In this regard, it is very similar to the applied science of classical crop breeding. These fields of inquiry rely heavily on statistical inference. A theoretical population geneticist or a crop breeder is therefore unlikely to make a deterministic prediction about the outcome of a natural evolutionary event or the exact characteristics of his or her next new cultivar. For the same reason, scientists investigating pest-resistance management tactics are reluctant to provide regulatory officials or farmers with exact predictions about how many years it will take for a specific pest to adapt to overcome a proposed resistance management plan. However, they can provide information on which of a number of approaches to development and deployment of transgenic and conventional pest-protected plants is likely to be most successful in decreasing the rate of pest evolution to adapt to those plants.
Quantitative comparisons of resistance management approaches for crops protected against insect damage began in 1986 (Cox and Hatchett 1986; Gould 1986a, b). A list of potential approaches has since been developed (Gould 1988a; McGaughey and Whalon 1992; Roush 1997; Tabashnik 1994). Some of the general approaches for resistance management for insect pests are as follows:
-
High dose of a single contained toxin in most plants, with some plants producing no toxin at all and thus serving as a refuge (approach 1).
-
Multiple toxins at high (or in some cases moderate) doses in most plants, with some nontoxic plants serving as a refuge (approach 2).
-
GMPP plants with low doses of a toxin that only slow the growth of the pest, so that pest population growth decreases and natural enemies can become more effective (approach 3).
-
Development of GMPP plants that produce the toxin only when and where it is most critical to protecting the plant (approach 4).
Much of the research aimed at developing these approaches and assessing their expected impacts has focused on transgenic pest-protected plants that produce Bt toxins.
A high dose of a toxin has been defined by the EPA Science Advisory
Panel (SAP 1998) as an amount that is 25 times the amount needed to kill 99% of susceptible insects. Empirical information on development of insect genetic adaptation to Bt toxins indicates that such a high dose will kill most partially adapted insects in a pest population (such as heterozygotes). The result is interruption of the typical stepwise process of evolving from susceptible populations, to one that is partially adapted, to fully adapted to the GMPP plant. An analogy can be made to the use of antibiotics to treat human pathogens. The utility of decreasing the survival of partially adapted human pathogens has long been recognized by medical researchers and physicians who routinely recommend that their patients continue to take antibiotics past the period when most of the infectious organisms have been killed. The prolonged treatment period ensures that partially adapted target pathogens will also be killed and so not be transmitted to other people.
Many researchers have examined field and laboratory insect populations in an attempt to understand the mechanisms of insect adaptation to Bt toxins (Tabashnik 1994; Gould and Tabashnik 1998). Results indicate that either multiple genes or single recessive genes are needed to confer full adaptation to a high dose of Bt although partial resistance can be confered by a dominant gene. A very low proportion (1 out of a million, to 1 out of 1000) of fully adapted insects is expected to exist in a population before the population is exposed to Bt. If all host plants on a farm produced the high dose of Bt, only those few insects with the right gene combinations would survive. If they then mated with each other, their offspring would be fully adapted, and the pest population would no longer be affected by the GMPP crop. The planting of nontoxic host plants (refuges) is designed to make sure that a relatively large number of totally susceptible insects are produced on each farm, compared with the few fully adapted insects produced. As long as this refuge is maintained, almost all fully adapted insects produced in the Bt crop are expected to mate with susceptible insects. The offspring of these matings will not have the proper combination of genes needed to be fully adapted, so the evolutionary process is again interrupted. Many researchers expect use of the refuge in combination with plants that produce a high dose of the toxin to increase the time needed for insect adaptation by a factor of 10 (for example, from eight years to more than 80 years) if properly implemented (Gould 1998; Roush 1997; Tabashnik 1994).
By using transgenic or conventional pest-protected plants that contain high levels of multiple toxins with high doses (approach 2), the chance that insects will have the proper gene combination to be fully adapted is further decreased compared to the case where only one toxin is produced by the plant. It also increases the efficiency with which refuge-produced insects can break up combinations of resistance genes from the few pests
that happen to carry the proper gene combination (Gould 1986a, b; Roush 1997). The development of resistance may be delayed by the use of several toxins with different modes of action (Zhu et al. 1994; Jach et al. 1995). The toxins could arise from a combination of conventional breeding and transgenic techniques. However, even if the target sites of two toxins differ, there is still the possibility of cross resistance if the two toxins can be detoxified by the same enzymes. The new high-dose refuge approach (approach 1) has been the most widely accepted tactic for resistance management of target pests of transgenic or conventional pest-protected plants. Approaches 3 and 4 also have potential applicability. Approach 3, which relies on an interaction between the GMPP plant and natural enemies, is expected to decrease the rate of evolution of adaptation because it does not result in a major decrease in the fitness of either susceptible or adapted pests. Companies have not embraced this approach, because it cannot always be relied on to protect the crop, and they may have liability for control failures. There have also been concerns that the approach might not always inhibit evolution of adaptation to the pest-protected plant (for example, Johnson et al. 1997a, b). Approach 4 would also decrease the rate of evolution of resistance because only the fraction of the pest population that feeds on the protected-plant parts would be killed. This general approach could be useful if correctly implemented, but technological and ecological problems must be solved before it can be used (Roush 1997).
The high-dose approach is feasible with Bt toxins because even at high doses no health or environmental problems have been reported in commercially grown varieties. Also, crop yield has not been reduced by production of high doses. That might not be the case with some other plant-protection mechanisms.
Much of the theory of resistance management for GMPP plants has been developed for diploid, sexually reproducing organisms (NRC 1986; Roush and Tabashnik 1990). The theory is therefore only partially applicable to viruses, bacteria, and even a large group of insects that have different means of reproduction.
Plant pathologists have long been concerned with viral, fungal, and bacterial adaptation to conventional pest-protected plants. In the 1950s they developed the concept of GMPP plants having either vertical or horizontal resistance to pathogens (Van der Plank 1963). Vertical resistance typically involved single plant genes that were initially very effective at mitigating a disease but were expected to be evolutionarily overcome by rapid genetic shifts in the pathogen (Lamberti et al. 1981). Horizontal resistance was typically controlled by many genes, offered lower but adequate suppression of the target pathogen, and was expected to be more durable (recalcitrant to pathogen adaptation). This system for
stereotyping GMPP plants had some predictive power, but later investigators found that there were too many exceptions (Lamberti et al. 1981). Durability of a specific GMPP plant is now typically judged in retrospect on the basis of its long term performance (Johnson 1981).
There have been some recent attempts to use population-genetics theory for developing and deploying conventional pest-protected plants in ways that slow pathogen adaptation (Burdon et al. 1994; Lannou and Mundt 1996 and 1997; Mundt 1990; Zeigler 1998), but it has not become common practice. Instead, many current pathology programs for production of GMPP plants emphasize continual discovery of new resistance genes so that breeding programs can stay a step ahead of an evolving pathogen (McIntosh and Brown 1997).
Researchers developing engineered pathogen-resistant plants have also been concerned with pest adaptation (Beachy 1997). Although new molecular approaches could lead to plants that offer a greater evolutionary challenge to pathogens (Beachy 1997; Bendahmane et al. 1997), little empirical or theoretical work has been aimed at determining how to produce durable engineered pathogen resistance (but see Qiu and Moyer 1999).
2.9.3 Future of Resistance Management for GMPP Plants
EPA has been active in developing resistance management plans for Bt crops. It has developed an internal group of staff to work on the issue and has consulted formally and informally with researchers (Matten et al. 1996; Matten 1998). Researchers and EPA regulatory officials will probably learn a lot of general principles about how to develop and implement resistance management of transgenic pest-protected plants from the continuing work on Bt crops. Much has already been learned from the Bt system regarding theoretical and practical aspects of developing and implementing a resistance management program, but the EPA policy is still evolving (Matten 1998). Each year, new empirical results should provide information on better ways to optimize resistance management for these crops. Therefore, plans implemented today will need to be periodically reviewed for their continued usefulness.
Although EPA has instituted programs and regulations that demonstrate serious concern about insect adaptation to Bt crops (Matten 1998; SAP 1998), it has not indicated concern about virus adaptation to transgenic pest-protected plants with plant-produced viral coat protein. In general, EPA has not commented in formal documents about when it considers pest adaptation to pest-protected plants to be an important public or social problem and when it considers resistance to be only a business problem or an insignificant public or social problem.
There has been considerable public debate about this issue. One opinion is that there is no more reason to institute resistance management for transgenically produced Bt than there is to institute resistance management for conventional pesticides. Others argue that the use of Bt toxins in transgenic pest-protected crops is fundamentally different from the use of chemical pesticides, for a combination of the following reasons:
-
Insecticides are typically used only when pest populations increase to the point where substantial yield-losses could occur, so refuges are already present. With transgenic Bt crops, the toxin is selecting for resistance all season long, even during weeks when the pest cannot feed on plant parts that affect crop yield, or during years when pest number are too low to cause yield loss.
-
Bt toxins are seen as benign to the environment and public health, and no equally benign replacement product is available.
-
Bt toxins are the active component in Bt spray formulations that have been used sustainably by organic and conventional farmers for many years, and this tool could be lost if transgenic Bt crops are not managed correctly.
Many transgenic pest-protected plants of the future may be protected by novel mechanisms and therefore not compromise the utility of plant protectants that are already being used by farmers. In such cases, the company that produces the plant protectant can be seen as the major party affected by pest evolution of adaptation to the company 's product. However, there could be cases in which a new transgenic pest-protected plant cultivar is produced by transferring a plant protectant that is already in use to another crop species. The new use could increase the risk that pests will involve adaptation to the plant protectant in all uses. An example might be moving a pathogen-resistance gene from tomato into cotton. If the same pathogen is now controlled by this resistance-mechanism in both crops, the intensity of selection for adaptation could be substantially increased. A resistance management program could be developed in such a situation to ensure that adaptation does not evolve at a rate or in a manner that causes environmental, economic, or health problems.
2.9.4 Summary
In light of the above discussion, the committee found that
Evolution of pest strains that can overcome the pest-protection mechanisms of plants can have a number of potential environmental and health impacts.
For example, adaptation of a pest to an environmentally pesticidal substance produced by a transgenic plant may cause farmers to return to or begin the use of a conventional chemical pesticide with toxic effects on nontarget organisms. Also, adaptation of a pest to one type of transgenic pest-protected plant could result in its replacement with a novel transgenic pest-protected plant for which there is less information regarding health and environmental impacts.
Our understanding of the evolution of adaptation to pest-protected plants is still limited, but there is reasonable expectation that specific approaches to the development and deployment of transgenic pest-protected crops can substantially delay the evolution of pest adaptation. The committee found that
Although EPA has worked actively to develop useful resistance management plans for crops containing Bacillus thuringiensis (Bt) toxins, the agency has not articulated a general policy indicating when it believes it should require the development of resistance management plans for specific transgenic pest-protected crops.
The committee recommends that EPA continue to deal seriously with Bt resistance management (section 1.6.1), and it should also begin to consider resistance management strategies for other transgenic pest-protected plants. Specifically,
If a pest protectant or its functional equivalent is providing effective pest control, and if growing a new transgenic pest-protected plant variety threatens the utility of the existing uses of the pest-protectant or its functional equivalent, implementation of resistance management practices for all uses should be encouraged (for example, Bt proteins used both in microbial sprays and in transgenic pest-protected plants).
2.10 RECOMMENDATIONS
-
When the active ingredient of a transgenic pest-protected plant is a protein and when health effects data are required, both short-term oral toxicity and potential for allergenicity should be tested. Additional categori.es of health effects testing (such as carcinogenicity) should not be required unless justified.
-
The EPA should provied clear, scientifically justifiable criteria for establishing biochemical and functional equivalency when registrants request permission to test non plant-expressed proteins in lieu of plant-expressed proteins.
-
Priority should be given to the development of improved methods for identifying potential allergens in pest-protected plants, specifically, the development of tests with human immune-system endpoints and of more reliable animal models.
-
Criteria for evaluating the merit of commercializing a new transgenic pest-protected plant should include the anticipated impacts on nontarget organisms compared with those of currently used pest control techniques 7 and whether gene flow to feral plants or wild relatives is likely to have a significant impact on these populations.
-
If a pest protectant or its functional equivalent is providing effective pest control, and if growing a new transgenic pest-protected plant variety threatens the utility of existing uses of the pest protectant or its functional equivalent, implementation of resistance management practices for all uses should be encouraged (for example, Bt proteins used both in microbial sprays and in transgenic pest-protected plants).
7 |
Includes both chemical and non-chemical methods which are currently used. |