1
Properties and History
Helium is an element with many remarkable properties and a fascinating history. An accurate assessment of the possible effects of the Helium Privatization Act of 1996 is not possible without first understanding the reasons why helium is so important and the historical context of the legislation. This chapter presents and overview of the properties that make helium a unique resource and discusses the history of helium usage up to 1996, when the legislation was enacted.
UNIQUENESS OF HELIUM
The helium atom is smaller than that of any other element and second only to the hydrogen atom in lightness. It has a particularly stable and symmetrical structure. The nucleus of the atom consists of two protons and either one or two neutrons, depending on the isotope. The two electrons of the helium atom form a closed spherical shell that is tightly bound to the nucleus. The ionization potential for helium is higher than that for any other element. As a result, helium is chemically inert and does not form stable compounds with other elements. The attractive forces between helium atoms are also so weak that helium has the lowest liquefaction temperature of all the "permanent" gases and, unlike all other elements, does not freeze under its own vapor pressure as the temperature is lowered toward absolute zero. At one atmosphere pressure, 4He liquefies at 4.2 K, whereas hydrogen liquefies at 20.4 K and neon at 27.1 K.
Of the two stable helium isotopes, the lightest is 3He. This isotope is relatively rare and is obtained as a by-product of nuclear weapons production, following the radioactive decay of the hydrogen isotope tritium. The heavier helium isotope, 4He, is the more abundant form and available from a number of sources, including Earth's atmosphere. As will be discussed in Chapter 4, however, it is most economically obtained as a by-product of natural gas production.
The first large-scale use of helium for nonscientific purposes was its substitution for hydrogen as a lifting gas in lighter-than-air applications (e.g., balloons, zeppelins, and blimps). Although hydrogen provides about 7 percent more lift than helium, it is much more dangerous to
use, as was dramatically demonstrated when the zeppelin Hindenburg exploded and was then destroyed by fire at Lakehurst, New Jersey, in 1937. The inertness of helium firmly established it as the lifting gas of choice for most applications.
The properties of helium that make it desirable for application as a lifting gas (i.e., its chemical inertness and low mass) also underlie its use for many other commercial applications. There are, however, two other properties of helium that are important in some special industrial processes. First, the thermal conductivity of gaseous helium is five to six times greater than that of other gases (the exception is hydrogen, which is comparable in thermal conductivity). Second, helium atoms share with hydrogen the ability to diffuse with relative ease through many solid materials, especially at elevated temperatures. An example in which these properties play a vital role is the manufacture of optical fibers, where the high thermal conductivity is important during the heat-treatment phase of fabrication, and the rapid diffusion of helium through the glass ensures that there are no trapped bubbles that would destroy the desired properties of the fibers.
Helium's low liquefaction temperatures make it desirable for purging, pressurization, and cryogenic applications. The fact that it remains a gas at liquid hydrogen temperatures makes it especially useful in the purging and pressurization of liquid hydrogen rocket propulsion systems. Another important use for liquid helium is in the cooling of superconducting magnets, an application of increasing importance with the advent of such technologies as magnetic resonance imaging (MRI) and superconducting cavities for high-energy accelerators (see Chapter 3).
Liquid helium is also a subject of great scientific interest in itself. Liquid 4He undergoes a phase transition to a superfluid state when the temperature is lowered below 2.2 K. The superfluid properties of liquid 4He are generally believed to be a manifestation of the phenomenon known as the Bose-Einstein condensation, where the whole liquid exhibits macroscopic quantum properties such as quantization of the superfluid flow field. A practical aspect of superfluid helium is its extremely high thermal conductivity, which is orders of magnitude greater than that of other excellent thermal conductors (e.g., copper). Another important application for liquid helium is the 3He-4He dilution refrigerator, which employs both isotopes and allows routine access to temperatures within a few tens of millidegrees above absolute zero. Studies of materials at liquid helium temperatures or using liquid-helium-cooled apparatus play a central role in modern materials research. These cryogenic applications depend crucially on the unique properties of helium and can be expected to ensure a demand for helium for the foreseeable future.
HISTORY OF HELIUM USAGE
Helium was essentially unknown before the twentieth century. It was first detected in the spectra of solar prominences observed during the solar eclipse of August 18, 1868. The art of spectroscopic observations and interest in them had advanced sufficiently since the preceding total solar eclipse that at least six separate observers were able to identify a new spectroscopic line in the atmosphere of the Sun. The line was initially thought to be that of sodium, but it was concluded later that year that it was actually evidence of a new element. This element was named helium, after the Greek word for Sun.
Nearly 30 years passed before the element helium was detected from terrestrial sources. It was not until 1895 that Sir William Ramsay and Lord Rayleigh reported the spectroscopic observation of helium in gas evolved from uranium and thorium ores. Following this report,
BOX 1.1 Natural Gas Natural gas is a naturally occurring mixture of hydrocarbons and other compounds. Like petroleum, natural gas is generated by the deterioration of organic materials under anaerobic conditions. It consists primarily of light hydrocarbons (e.g., methane and ethane) with some heavier hydrocarbons (e.g., butane and propane). Impurities (e.g., sulfur dioxide, carbon dioxide, and helium) can also occasionally be present in various concentrations. |
helium was soon discovered in trace quantities from a variety of sources, including the atmosphere.
The supply situation changed dramatically in the early 1900s, when helium was found to exist in rather large quantities in the natural gas wells of the midcontinental United States (see Box 1.1 for a description of natural gas). The best known story concerns the exploratory well drilled in 1903 at Dexter, Kansas, which produced a gas that refused to burn. Subsequent analysis of the gases from this and neighboring wells by Cady and McFarland showed that the helium was at concentrations of around 1 percent by volume. Although geologists and oil producers reported a number of natural gas fields from which helium could potentially be produced, interest in commercial recovery did not develop until the onset of World War I. For example, Kamerlingh Onnes obtained the helium for his early 1908 experiments on the liquefaction of helium by a tedious extraction from a mineral source, monazite sands.
The British government became interested in helium as a lifting gas early in War World I. Although not as buoyant as hydrogen, helium would not burn and thus could withstand enemy fire. The British initiated a research program at the University of Toronto, Ontario, in 1915, and a small experimental plant was operating near Hamilton, Ontario, by 1918. No Canadian helium was actually used in warfare, but the separation technology was demonstrated at Hamilton and later at Calgary, Alberta, in 1919 and 1920.
The task of establishing a domestic source of helium was given to the U.S. Bureau of Mines (BOM) when the United States entered World War I. BOM contracted the construction of three helium extraction plants: two at Fort Worth and one at Petrolia, Texas. The three produced about 200,000 scf (5,500 scm) of helium in experimental runs, and 140,000 scf (4,000 scm) of compressed gas was awaiting shipment to Europe at the war's end. It is not surprising that there was no thought given to long-term storage of helium at this point, except as compressed gas in cylinders ready for immediate use.
Cognizant of Germany's relative success with military zeppelins, the U.S. Navy began a program immediately after the war to develop rigid airships as naval weapons. The first full-scale U.S. helium production plant, completed near Fort Worth in April 1921, was based on the experimental wartime plant designed by Linde Air Products. The plant was operated for the Navy under contract by Linde until 1925, when it was turned over to BOM. With the Petrolia gas field nearing exhaustion, the plant was mothballed in 1929, by which time it had produced some 47 million scf (1.3 million scm) of helium. As before, no thought was given to long-term storage in a reserve.
In anticipation of the Fort Worth closure, BOM decided to build a new plant, the first unit of which went into production in April 1929, followed by a second unit in May 1930. This plant, near Amarillo, Texas, subsisted on natural gas from the Cliffside field, which was connected by a 12-mi (19-km) pipeline. The methane produced was pipelined about 7.5 mi (12 km) to Amarillo, where it was used as fuel. The plant could produce as much as 25 million scf (700,000 scm) of helium per year but never operated at full capacity.
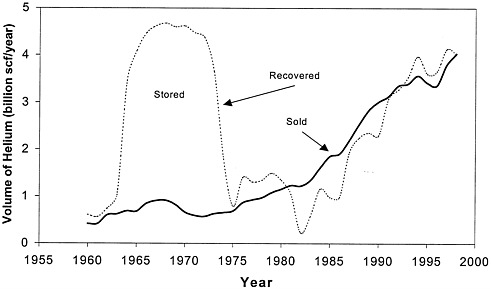
FIGURE 1.1 Annual helium recovery and sales, 1960-1997. The area between the curves is the net amount that was added to or removed from the reserve (courtesy of the Bureau of Land Management).
Although helium in the ground was owned by the federal government and could only be purchased—unlike oil and gas, which could be leased—the Girdler Company built two commercial helium plants in 1927-1928: one at Dexter, Kansas, and the other at Thatcher, Colorado. These plants made Girdler the only private helium producer in the world at the time. Over the next 10 years, Girdler produced some 14 million scf (380,000 scm), almost 90 percent of which went to the Navy airship program. The Girdler plants were sold to the U.S. government in 1937, returning helium to the status of a federal monopoly. Later, BOM built a fourth plant, the Exell facility in Texas, which eventually became its last operating plant.
Considerable optimism about future markets for helium developed in the 1950s, and helium began to be considered as a resource for the Cold War. As a result, Congress enacted the Helium Act Amendments (P.L. 86-777) in 1960, which in essence directed the secretary of the Interior to (1) accomplish the acquisition and conservation of helium, using the partially depleted Bush Dome reservoir in the Cliffside field for storage and (2) buy commercial crude helium, using funds borrowed from the Treasury. The act also permitted private helium production, so that BOM would become a buyer of last resort. As a direct result of the 1960 Act and on the basis of 22-year contracts with BOM, several private oil and gas producers built five new helium extraction plants. It soon became apparent, however, that the optimistic market projections were not going to be met. BOM had 35 billion scf (970 million scm) of crude helium in storage by 1973 (see Figure 1.1), plus a large and growing debt to the Treasury. Under the circumstances, the government cancelled the contracts, touching off several years of litigation, and most private helium production was suspended during that period. What had begun as an effort to assure the government of sources of helium at a time when there was no private production evolved first into a program to promote helium conservation by encouraging private companies to extract it
from natural gas, then into a provision for storage to deal with the expected periodic imbalances in supply and demand, and finally into a situation that threatened the viability of the entire industry.
Private consumption of helium began to recover from this low point in the 1970s, and private production was again healthy by the mid-1980s, although BOM was still producing. By 1988, the Office of Management and Budget proposed that all helium production belonged in the private sector. This proposal was opposed by the Congress, however. In 1992, the administration suggested a compromise under which BOM's facilities would not be sold but government agencies would be free to buy helium from the private sector.
In the meantime, two major changes occurred. First, Congress eliminated BOM in 1997 and transferred responsibility for the Federal Helium Reserve to the Bureau of Land Management (BLM) of the Department of the Interior. Second, the government raised its helium prices to levels significantly above those of the private producers. As a result, just before BLM ceased helium production in 1998, more than 90 percent of domestic demand was being satisfied from private sources—up from nothing before passage of the 1960 amendments. Somewhat ironically, it was the Bush Dome reservoir and its associated pipelines that were facilitating this change and making a unique contribution to the conservation of U.S. domestic helium. In Congress, however, initial reluctance to leave the helium business had switched to eagerness to get out, provided that the complex at Cliffside field could be preserved.