3
Uses of Helium
The total amount of helium used in the United States grew from about 1.4 billion scf (36 million scm) in 1985 to about 2.6 billion scf (69 million scm) in 1996 and is expected to continue to grow in the foreseeable future (Figure 1.1 showed the trend in helium production and sales from 1960 to 1997). The remarkable fundamental properties of helium (Box 3.1) make it useful for many applications, but few people appreciate the broad range of crucial roles that it plays in industrial processes, military and civilian aerospace applications, medical technologies, and basic research. For example, research using liquid helium has been—and continues to be—at the forefront of science. Although some lines of investigation at the forefront of science may appear to have little impact on society, they could eventually become commercially or militarily important activities.
BOX 3.1 Unique Properties of Helium
|
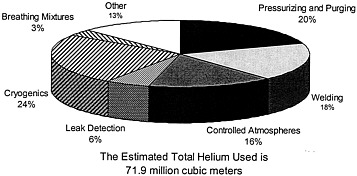
FIGURE 3.1 Estimated helium consumption in the United States by end use in 1996. SOURCE: U.S. Department of the Interior, U.S. Geological Survey. 1996. Mineral Industry Surveys: Helium. Reston, Va.: USGS.
This chapter examines the various current and future uses of helium. The current applications are divided into the main categories used by BLM to track domestic helium usage in 1996 (Figure 3.1): cryogenics, pressurizing and purging, welding, atmospheric control, leak detection, breathing mixtures, lifting, and other uses. Each section will discuss the application for which helium is used, the reason for its use, the volume of gas employed, and the prospects for conserving helium or using substitutes. The chapter concludes with a discussion of the potential future applications that may require helium and the overarching issues that surround the tracking of helium usage.
INADEQUACY OF DATA ON HELIUM USE
The U.S. Department of the Interior releases annual reports that discuss the supply and demand for helium. The domestic helium consumption portion of these reports is based on surveys, conducted every 5 years or so, of helium wholesalers. These surveys track domestic use in 18 categories. Although the gross amount of helium exported is known, there is only sparse information on its end uses. Even less is known about foreign production of helium. The last survey of domestic helium wholesalers was in 1995 and the one before that was in 1991. Helium use is estimated using the results of these surveys as a baseline.
One problem that the committee confronted in analyzing the trends in helium usage during the 1980s is that the Department of the Interior reduced the number of categories of helium use it reported from 13 to 7—even though it currently collects data on 18 categories. This aggregation of the data made it difficult for the committee to follow helium use over time within each category. A second problem with the data reported since 1994 is wild variation from year to year in the estimates of the percent of helium consumed in each category. For instance, the percent of helium in the "other" category was reported to be stable at about 11 percent from 1985 to 1993. It jumped to about 22 percent in 1994 and then declined to 18 percent in 1995 and 13 percent in 1996.
CURRENT USES
Cryogenics
The largest current use of helium is for cryogenics. The amount of helium used for cryogenics was about 620 million scf (17 million scm) in 1996. The main cryogenic applications to be discussed in this section are magnetic resonance imaging, semiconductor processing, and large-scale and small-scale fundamental research that requires helium temperatures. Unfortunately, no specific data are available on the quantities used for each of these applications.
It should be noted that the availability of safe, inexpensive helium resulted in the abandonment of liquid hydrogen as a coolant. In a situation of extreme shortage, hydrogen could be used as a refrigerant, with appropriate safety precautions. The lowest temperature attainable with hydrogen, however, is 9 K, which would require a change in magnet wire technology to a niobium-tin alloy or some other high-temperature material. Systems cooled only by liquid hydrogen would also not be able to achieve low enough temperatures to permit a second stage of refrigeration based on 3He. This problem might be resolved by using some of type of magnetic refrigeration as an intermediate stage. Any changes would require a large capital investment in infrastructure, however.
Magnetic Resonance Imaging
The main medical use of liquid helium is for magnetic resonance imaging (MRI) systems. Liquid helium is needed as a refrigerant for the superconducting magnets that are critical components in many of these devices. The availability of helium at a favorable price and the stability of the helium market have contributed to the rapid growth of MRI as a diagnostic tool and have allowed it to make a significant contribution to health care in the United States. There is a large, stable base of superconducting machines in the United States and abroad (>4,000), and this base is expected to grow at 15 percent per year. At least one reason for the abundance of superconducting MRI systems in the United States (approximately 80 percent of the worldwide installed base) is the ease with which liquid helium can be obtained and its relatively low cost.
There is no substitute for helium in this application. Use of high-temperature superconducting wire, while under investigation by several manufacturers, is not likely to be a viable alternative to conventional superconductors in MRI machines. High-Tc superconductors cost 500 times as much as conventional niobium-titanium superconducing wire. Although this cost might decrease in the future, both the mechanical and electrical properties of high-Tc would have to be improved before they could be used economically for this medical application.
However, even with the increasing medical importance of MRI systems, this application's demand for helium is expected to be flat or to grow only slowly. Because it is difficult for them to obtain reliable supplies of liquid helium in other parts of the world, MRI manufacturers have introduced new technologies that dramatically decrease the quantity of liquid helium required. These technologies include improved cryostats with superior thermal efficiency, cryocoolers that recondense the helium, and improved magnet wire and junctions that reduce the size and weight of materials that need to be cooled and lower the small heat load that the magnet itself generates. These systems, which often trade the low cost of electricity for the high price and relative inconvenience of cryogens, have dramatically decreased helium consumption in the superconducting MRI systems that are manufactured today. The newest generation of machines
uses 10 times less helium than earlier generations and can operate as long as 3 years between helium fills. Furthermore, while superconducting MRI systems are often the high-end performers, most of the growth in the current U.S. and world markets is for systems at lower magnetic field strengths, which use electromagnets or permanent magnets and require no cryogens.
Semiconductor Processing
Silicon wafer suppliers are the primary users of helium in the semiconductor industry. The process of pulling 12-in. (300-mm) crystals uses helium-cooled superconducting magnets to mechanically stabilize the hot boules of semiconductor material. Other activities in the industry that use helium are plasma etching and vacuum pumping. In the latter, liquid-helium-cooled surfaces cryopump gas contaminants. Recycling techniques could be introduced in each of these processes to reduce helium consumption.
The total volume of helium used by the semiconductor industry is unknown. Nevertheless, it is very important to this key industry. An increase in the price of helium could be accommodated, but if helium were to disappear altogether, semiconductor processing would be severely hampered.
Large-Scale Fundamental Research Requiring Helium Temperatures
The term "large scale" in the context of superconductor-based fundamental research refers to systems that are physically large (e.g., accelerators whose rings are many kilometers in circumference). Superconducting magnets and superconducting microwave and radio-frequency cavities are important components of charged-particle accelerators for nuclear and particle physics and the technology of choice for the highest energy colliding-beam accelerators. All such systems currently employ superconductors that must be cooled by liquid helium. In particular, significant amounts of helium are required to cool the magnets in large particle-storage rings (the circumferences of the Relativistic Heavy Ion Collider at Brookhaven National Laboratory, the Tevatron at Fermilab, and the ring at the Large Hadron Collider at CERN are 5 mi (8 km), 3.7 mi (6 km), and 17 mi (27 km), respectively). The cryogenic plants used at accelerator facilities must be able to provide tens of kilowatts of refrigeration and require tens of megawatts of electric power.
In some systems, especially those using radio-frequency or microwave resonators of large size, the uniquely high thermal conductivity of liquid helium also assures that the superconducting system is kept at the uniform temperatures critical to operation. Since there is no alternative material with comparably high thermal-conductivity properties, helium is the only cryogenic fluid that can be reliably used to reach the low temperatures required for all superconducting electromagnets and radio-frequency and microwave resonators currently in use in large-scale systems. Another use of large superconducting magnets is in specialized particle detectors, and helium is also used to cool accelerator targets to low temperatures.
Like the MRI community, the large-scale fundamental research community is taking steps to increase the efficiency of its systems. The use of closed-cycle systems that reliquefy helium is now commonplace and growing. For example, the new accelerators that are coming on line are extremely efficient, with most of the gas being reused and only small amounts being lost through
leakage, maintenance procedures, and failures. Losses should decline further with incremental improvements in recirculation technology.
An important factor in the use of helium in large-scale applications is the possible development of substitutes for metallic alloy superconductors based on high-temperature superconducting compounds. Considerable progress has been made with superconducting transmission lines, to the extent that lines requiring liquid-helium temperatures are no longer seriously considered (e.g., prototype high-Tc lines are currently being installed in the power grid). Prototype superconducting magnets of a small laboratory scale, wound with high-temperature superconducting material, have also been produced, but they are extremely expensive. It would be premature, however, to say that it is inevitable that all large-scale superconducting systems will ultimately be made with high-temperature materials, given the extremely serious materials problems that would first have to be solved.
Large-scale accelerators could absorb a helium price increase because the cost of helium is a small part of their operating costs. However, an increase could reduce the amount of research conducted. If no helium were available, these facilities would cease operation.
The possible use of high-temperature superconductors in high-power radio-frequency applications is also under study at this time, but it is not likely to have any impact on accelerator technology for some time. However, high-temperature superconducting materials are close to commercialization in lower-power applications such as passive microwave filters for cellular telephone ground stations. These systems, which rely on liquid-nitrogen-based cryocoolers, must be able to run unattended for extended periods of time (i.e., years), so that complex, liquid-helium-based cryocoolers are not feasible.
Small-Scale Fundamental Research Requiring Helium Temperatures
Cryogenic-based research has broadened in recent years from its original focus on the properties of liquid and solid helium and metallic superconductors to include a very wide range of materials and phenomena. The fields most heavily involved in the use of helium are materials research, condensed-matter and materials physics, materials science, chemistry, and observational astronomy and astrophysics. The use of helium is absolutely critical to fundamental cutting-edge research in all of these areas, many of which (astrophysics, for one) are potential sources of future technologies.
In chemistry and materials research, both low-temperature measurements and measurements using instruments needing low temperatures for their operation are required to fully characterize new materials. For example, liquid helium is used to cool the superconducting magnets required for nuclear magnetic resonance (NMR), which is a critical tool in structural chemistry. Liquid helium is also frequently used to precool and then sustain the operation of 3He-4He dilution refrigerators, which are used to achieve ultralow temperatures (i.e., well below 1 K and down to 0.002 mK). These ultralow temperatures are unattainable by the evaporative cooling of either 4He or 3He. Helium is also used to cool samples for various kinds of electrical transport, magnetic, thermal, and spectroscopic studies. The largest steady magnetic fields, such as those available at the National High Magnetic Field Facility, employ combinations of resistive and superconducting magnets that require liquid helium. In many instances investigations conducted in strong magnetic fields involve materials that are also cooled using liquid helium. Many investigations at the frontier of materials research require the use of Superconducting Quantum Interference Device (SQUID) systems for magnetic measurements.
Two kinds of relatively sophisticated low-Tc SQUID systems have been very successful commercially. The first is the SQUID susceptometer, which has been used all over the world in an amazingly wide range of studies. The second is the multichannel system, each channel of which contains between 100 and 300 SQUID magnetometers, used to image magnetic signals produced by the brain. The magnetometers have noise levels as low as 2 × 10-15 T/root Hz. Under favorable circumstances they can locate a magnetic source to an accuracy of 2 to 3 mm, and they have been used for many different studies of the brain.
Astronomy and astrophysics, although not large users of helium, critically depend on liquid helium. Helium is used to cool infrared and other detectors to very low temperatures to reduce mechanical and electrical noise and permit the study of extremely weak signals. Some important research efforts in astrophysics (e.g., investigations of dark matter and the anisotropy of the cosmic background radiation) would not be possible without a supply of helium to cool the detectors.
Liquid helium is also itself a subject of great scientific interest. Liquid 4He undergoes a phase transition to a superfluid state when the temperature is taken below 2.2 K. The superfluid properties of liquid 4He are generally believed to be a manifestation of Bose-Einstein condensation, where the entire liquid exhibits macroscopic quantum properties such as quantization of the superfluid flow field. The properties of superfluid helium are unusual. For example, once set in circular flow, it will keep flowing perpetually. Moreover, it can flow up the walls of its container, meaning that a container being filled with superfluid helium can empty itself spontaneously.
For the past 50 years scientists have conducted research on the fascinating behavior of superfluid helium, have used superfluidity to verify theoretical predictions of quantum physics, and have used superfluid helium as a trap for other particles under study. The total amount of helium used in these studies is not well known but is probably relatively small. Needless to say, there is no substitute for helium in studies of the properties of helium itself.
With a limited number of exceptions, liquid helium or helium-based cryocoolers are required for all of the systems discussed above. This instrumentation is either commercial or under development and has nurtured an industry that manufactures cryogenic research equipment in the United States and abroad and that is completely dependent on the use of liquid helium. The efficiency of these systems could be increased by a number of different means, however. For example, an increased use of closed-cycle refrigeration would reduce the helium wastage in some current practices in which helium boil-off gas is expended. For sufficiently large aggregations of research instruments and apparatus using liquid helium, such as might be found at major research universities, recovering and reliquefying helium boil-off gas could reduce helium consumption. This type of operation demands a substantial capital investment in a liquefier, a recovery system, and a helium storage facility, however, and at current helium prices, would be cost-effective only under special circumstances. Consequently, there is little incentive for most universities and laboratories to conserve helium by recovering it. An alternative to recovery and reliquefaction on a large scale would be the use of small closed-cycle coolers. Although this equipment uses helium as a cryogen and, because of inefficiencies and errors, may not eliminate helium loss entirely, a number of commercial suppliers of instrumentation have begun marketing systems equipped with these cryocoolers. For some of the more delicate experimental investigations, the current generation of cryocoolers is mechanically and electronically too noisy, however, and some applications involving very sensitive measurements may require the development of less noisy refrigeration systems.
Pressurizing and Purging
Helium plays a unique and critical role in the pressurizing and purging of primary rocket propulsion systems. It will continue to do so as long as the propellant is a combination of liquid hydrogen and liquid oxygen. First, helium gas is used to pressurize the propellant tanks for the engines. In this application, pressure is provided to prevent pump cavitation and must be sufficient to keep the tanks from collapsing under vehicle-imposed structural loads. Pressure-fed propulsion systems are also used to provide engine-chamber pressurization. Second, helium gas is used for the purging of the propellant feed systems for liquid-hydrogen engines. Helium is used because its normal boiling point is lower than that of hydrogen. Thus it is the only element that can be used without compromising propellant integrity or feed-system function. Other gases would freeze, producing particles that could clog equipment and seize an engine, or react with or dissolve in the liquid hydrogen, reducing engine efficiency, all with potentially disastrous results.
The use of liquid and gaseous helium for pressurizing and purging rocket propulsion systems by the aerospace industry and the National Aeronautics and Space Administration (NASA) amounted to about 470 million scf (13 million scm) of helium in 1996. Large-scale aerospace uses of helium are expected to increase over the next 20 years, as the space shuttle is used to construct and service the International Space Station. There is no question that past successes in space exploration and the associated technology have been partially enabled by access to substantial supplies of helium. Because aerospace use is such a large fraction of overall usage, attention must be paid to developing alternative approaches to helium-based pressurizing and purging or, where there are none, recycling procedures. There has been little incentive thus far to conserve helium in large-scale aerospace use. However, aerospace engineers are focusing their attention on future space access vehicles that do not depend on cryogenic propellants. If the uses of noncryogenic propulsion systems increase, the demand for helium as a pressurant will decrease.
It should be noted that the rocket industry also uses significant amounts of helium for welding, cooling scientific payloads, refrigerating fuel-handling systems to liquid-hydrogen test temperatures, and as a pneumatic control system fluid in spacecraft and other rocket-propelled systems. NASA's current combined use of liquid and gaseous helium for all purposes amounts to the equivalent of 150 million scf (4.2 million scm) of gaseous helium per year.
Welding
Figure 3.1 shows welding as one of the largest categories of helium usage. The amount of helium apparently used in this category was about 470 million scf (13 million scm) in 1996. These data may be misleading, however, because the suppliers of helium for welding also tend to be the leading suppliers of helium for most small-scale, incidental uses (the information on the suppliers of helium for welding is from a report provided to the committee by A. Francis in December 1998). Thus, it is uncertain how much helium is actually used each year for welding and whether this decline is the result of a decline in the use of helium in welding, a decline in other uses reported as welding, or a change in how the other uses are reported. The two uses of helium to be discussed in this section are arc welding and laser welding.
Arc Welding
Gaseous helium is used for gas metal arc welding and gas tungsten arc welding. The helium serves mainly as a shielding gas, preventing atmospheric contamination of the molten metal and stabilizing the arc. It also serves the additional function in gas tungsten arc welding of shielding the red-hot tungsten electrode from the surrounding atmosphere. The unique characteristics of helium in these applications are its high ionization potential and high thermal conductivity, combined with its inertness. The benefits of its use include greater welding penetration in thick joints and in metals with very high thermal conductivity; higher travel speeds, permitting greater productivity; better shielding-gas coverage in vertical and overhead position welds; and flatter surface of root pass when used as a backing gas.
Some weld compositions can tolerate a wide variety of shielding gases, but others are quite demanding. For example, aluminum alloys and other reactive metals require inert shielding. While argon is used in some of the applications that require true inertness, helium or helium mixtures (often 20 percent helium and 80 percent argon) are used to increase the weld pool depth (e.g., penetration) or increase the travel speed. These argon-helium mixtures are quite popular in that they combine the good arc stability and lower cost of the argon with the deeper penetration of the helium. The recycling of helium would entail considerable cost in arc welding applications but would not be impossible. In those activities that are most technologically challenging in welding (i.e., aircraft engines and airframe manufacture), there probably is no substitute for helium. For other applications, argon cover gas might be substituted for helium, as is done in countries where helium gas is expensive, but there would be some loss in productivity.
Laser Processing
Processing with carbon dioxide (CO2) lasers is a growing activity in the United States. The amount of helium used in these operations is approximately 200 million scf (5.5 million scm) per year and is growing at about 20 percent per year. Helium serves several functions in laser processing: it is primarily a shielding gas for the molten pool in laser welding but is also an important component of the lasing gas in all CO2 lasers. Carbon dioxide lasers are also used for cutting, drilling, cladding, and heat treatment, although welding is the only process that also uses helium as a shielding gas.
The helium used as a shielding gas in CO2 laser welding protects the weld pool from oxidation (until the pool cools to temperatures where it does not react with the oxygen and nitrogen in the atmosphere), suppresses plasma formation, and protects the laser optics from damage. Other inert gases can replace helium in protecting the weld from oxidation and in protecting the optics, but the special characteristics of helium are quite important for plasma suppression at very high power densities. For laser powers above about 5 kW, helium is preferred because it is the gas that is least likely to ionize. Shielding and plasma suppression are open-loop processes where the gas flows through a nozzle toward the point at which the laser beam intersects the weldment. Recycling this shielding gas would require substantial investment in equipment to capture and refine the helium after it is contaminated with weld fumes and the surrounding atmosphere.
The smaller but perhaps more critical use of helium in laser processing is as a component of the lasing gas. The gas mixture in CO2 lasers consists of nitrogen, helium, and CO2, roughly in the ratio 3:2:1. Helium serves to cool the excited CO2 molecules in the laser cavity. In theory, it
should be easy to recycle the helium gas used in the lasers themselves, since the gas is confined within the laser and is replenished via a closed loop. In reality, recycling is difficult because the CO2 breaks down during the lasing process and must be replenished and because the reduced pressure in the laser cavity requires a vacuum pump, which can add contamination. Therefore, CO2 lasers require a regular supply of lasing gas to replenish the losses during operation and after the system is vented for maintenance. The annual operating cost for laser welding is typically dominated by the cost of electrical power, with shielding helium costs typically in second place at nearly 30 percent of the total and laser helium costs typically in third place at about 2 percent of the total. Obviously these percentages will change as helium cost fluctuates.
There are alternatives to laser processing. For example, electron-beam processing can be used, but a vacuum is required to achieve high performance. Nd:YAG lasers (see Appendix D) can also be used instead of CO2-based lasers, but they are not desirable for most cutting and thick welding processes because they cannot yet achieve the same power densities and they have a much lower operating efficiency.
Atmospheric Control
Helium is used to create an inert atmosphere in many industrial fabrication processes. The amount of helium used in atmospheric control was about 410 million scf (11 million scm) in 1996. This section will examine helium usage in optical fiber manufacture, plasma-arc coating, plasma-arc melting, and heat treatment.
Optical Fiber Manufacture
Fiber-optic technology is a burgeoning field that is becoming increasingly important in modern communications. The manufacture of optical fibers critically depends on the use of helium gas. It involves a number of steps in which a large rod is eventually pulled to form a fiber. The fiber is also coated with several different claddings during the drawing process, which are applied using a modified chemical vapor deposition (MCVD) process. Helium is mixed with the MCVD reactive gases to enhance the thermal gradient and improve the uniformity of the claddings. Helium is also used in the chilling of freshly drawn fiber, where it serves as a nonreacting thermal contacting agent. An additional property of helium that is critical to this activity is that it does not form bubbles in the glass, which would destroy the fiber's transmission properties.
Helium currently accounts for about 1 percent of the total cost of fiber production. The total amount of helium consumed in optical fiber manufacture is not definitively known but is at least 20 million scf (550,000 scm) and is expected to more than double by 2003.
Helium vented during this process could be recycled without much difficulty. The only contaminant is chlorine, which can be removed from the gas by cooling it until the chlorine condenses. However, the current price of helium is too low to make recycling an attractive option. (The information on fiber optics production is from a presentation to the committee by S.-P. Hong in December 1998.)
Plasma-Arc Coating
Plasma-arc coating is a process used in the aerospace industry and other industries to apply wear-resistant coatings to critical components. A mixture of 10 percent helium and 90 percent argon is typically employed. Plasma-arc coatings applied in 100 percent argon atmospheres are not as adherent. Hydrogen could be used as a substitute for helium in this application, but there are concerns about both safety and hydrogen embrittlement of the material being coated.
Plasma-Arc Melting
Plasma-arc melting is used to make specialty metal billets, such as titanium billets for jet-engine components. The process provides superior uniformity and composition control and has effectively replaced electron-beam melting, which used to be the main method. Furnaces operate in a helium atmosphere, with a helium plasma melting the titanium. There are no known substitutes for helium in this application. Argon does not have sufficient specific heat to produce the desired depth of melt. In this process the helium is recirculated during furnace operation but is vented at the end of a run because it is highly contaminated. In principle it should be possible to recover and purify the helium after a run.
Heat Treatment
Some industrial heat treatments are conducted in helium atmospheres because helium's high thermal conductivity allows the cooling of thick sections. For example, nickel-base superalloys are rapidly cooled in helium atmospheres. Helium can be replaced with argon in all but special applications.
Leak Detection
The use of helium for leak detection is a relatively small but critical industrial application. Helium is an excellent leak detector because of its low viscosity and large diffusion coefficient. The amount of helium used for leak detection was about 140 million scf (3.9 million scm) in 1996.
Leak testing using helium-tuned mass spectrometers is the most sensitive method of detecting leaks before they reach a critical stage and thus is ubiquitous in science and technology. It is critical in the manufacture of large rocket engines; the manufacture and maintenance of vacuum equipment in all aspects of industrial processing, including the electronics industry and the advanced materials industry; and in scientific research. Indeed, helium leak detection is the standard in any activity requiring leak-tight systems. Helium leak detectors were developed during World War II and can detect and measure leaks that are thousands of times smaller than the leaks that can be located by other procedures. Equipment can be calibrated to detect leaks that are smaller in volume than the equivalent of one drop of water per year. The usual procedure in leak testing is to spray the area on the outside of the system being tested with helium and then try to detect its presence on the inside, using a vacuum environment attached to a mass spectrometer.
The development of any replacement technology for helium is problematic because its use is based on its combination of unique size and inertness. Inert gases other than argon, which is excluded because it exists in the atmosphere at the 1 percent level, could be used in principle, but comparable sensitivity might require more elaborate mass spectrometers than are currently employed in helium leak-detection systems. Some degree of conservation through gas recovery might be possible using ''sniffer" technology. In this approach, helium is introduced under pressure into the device being tested, and a sniffer, connected to a helium-tuned mass spectrometer, detects leaks by sensing helium leaking out of the helium-pressurized apparatus. Helium used in this manner could be recovered.
Breathing Mixtures
Mixtures of helium and oxygen are used as breathing gases for deep-sea divers and for individuals working under high atmospheric pressures for extended periods of time. The advantage of helium over nitrogen in these mixtures is that it is absorbed and released by human tissue faster than nitrogen, making longer dives possible with shorter decompression times. The amount of helium used was about 56 million scf (1.6 million scm) in 1996. Given the diving industry's increasing reliance on robot replacements for humans, however, the amount of helium used for diving is expected to remain relatively stable, or even decline. Each dive consumes very little helium because "rebreathers," which recirculate the gas, are commonly employed.
Lifting
One obvious use of helium is as a lifting gas. Unfortunately, this usage is no longer reported separately, making the amount of helium used for this purpose unknown. Because it is the most visible use of helium, however, it deserves mention in a separate section of this report.
Hydrogen is the lightest gas, but helium's chemical inertness makes it the safest lifting gas. Helium replaced hydrogen for blimps in the 1930s after a number of tragic accidents involving hydrogen-filled airships. Nowadays, party balloons are probably the application with which most people are familiar. Helium is used as well in blimps that bear advertising (e.g., the Goodyear blimp), to detect low-flying cruise missiles, and to carry radar equipment to detect drug smugglers along the nation's borders. Helium-filled balloons are also used in various types of atmospheric and astrophysical research. One future use of helium is as a lifting gas in devices to lift heavy loads for construction.
Other Uses of Helium
Other uses of helium include minor medical uses and uses in lasers not covered in previous sections. The total amount of helium used for these purposes was about 320 million scf (8.9 million scm) in 1996.
POTENTIAL FUTURE USES
This section discusses potentially important applications that could rely on the availability of helium at a low price: magnetic levitation, superconducting magnetic energy storage, energy conversion systems, cryogenic wind tunnels, and superconducting electronics.
Magnetic Levitation
Superconducting magnets could be a central feature of a transport technology involving magnetic levitation (MAGLEV). In this technology, trains are levitated above their tracks, eliminating wheels and permitting very high-speed operation without frictional losses. The Japanese version of MAGLEV is based on the magnetic repulsion between a conducting track and high-power, helium-cooled, superconducting magnets on the vehicle. The highest speed achieved by a full-size test vehicle was 250 mph. A scaled-down, non-passenger-carrying vehicle attained 321 mph (517 kph) in 1979. Although superconducting MAGLEV technology was pioneered in the United States, there has been almost no domestic effort to develop it over the past 30 years.
Superconducting Magnetic Energy Storage
Superconducting magnetic energy storage (SMES) devices store energy in magnetic fields. These systems are highly efficient and could be used for equalizing energy distribution in power systems on small scales, e.g., to keep high-security computer systems running. The devices consist of closed coils of superconducting wires. The coils can be fed and discharged by means of a switch that connects the winding with the power grid. Superconductors are the only appropriate materials for SMES devices because they have no electrical resistance and thus can be operated in a persistent current mode without being connected to a power supply. A SMES device is the only method of storing electrical energy without first converting it to mechanical or chemical energy. The energy density in superconducting coils is comparable with that in flywheels (i.e., higher than that for capacitor banks, but less than that for batteries). Their short cycle times make them competitive with batteries, however. SMES units can be large scale (i.e., 1 MWh to more than 1 GWh) or small-to-medium scale (i.e., a few watt-hours to 1 MWh).
Energy Conversion Systems
Superconducting technology is considered to be an important secondary technology for plasma confinement fusion. Helium would play a critical role in cooling the superconducting magnets that would provide the magnetic containment environment. This kind of fusion technology would become widespread only in the very distant future, if ever. Nevertheless, it is essential to mention it as a potential user of helium as a refrigerant for superconducting magnets, which would probably require liquid-helium temperature refrigeration even if they were made from high-temperature superconducting wire.
A possible nearer-term use for gaseous helium in the energy conversion enterprise is in the high-temperature gas-cooled reactor. This type of fission reactor is fueled with a mixture of graphite and fuel-bearing elements. The coolant consists of helium gas pressurized to about 100 atm. Helium, which is radiologically inert, passes through interstices in the array of fuel and graphite elements. These reactors can operate at extremely high temperatures, as graphite has a high sublimation temperature and helium is chemically inert. The hot helium can then be directly used either as the working fluid in a high-temperature gas turbine or as the heat source to generate steam. The advantages of such a reactor are that it is meltdown-proof, nearly 50 percent more efficient than current water-cooled reactors, more proliferation-resistant since it uses ceramic fuel, and an efficient plutonium burner, and also that it produces less high-level waste. A joint U.S./Russian program is developing and constructing reactors for the destruction of Russian weapons-grade plutonium and is looking at their possible commercialization and marketing. Each reactor would require an inventory of 100,000 scf (2,800 scm) of helium and a reserve of 200,000 scf (5,600 scm). The inventory is expected to be drawn down at 25 percent per year. Depending on scenarios for deployment, the cumulative requirement for helium by 2020 could be as high as 75 million scf (2.1 million scm).
Cryogenic Wind Tunnels
Other potentially important uses for helium are in the operation of high-Reynolds-number wind tunnels, which would facilitate testing the behavior of aircraft and ships, and high-Rayleigh-number Bénard cells, which could lead to the realistic modeling of convective behavior in weather patterns and other studies of turbulent and convective phenomena having astrophysical and geophysical significance. These facilities would require liquefiers of the scale used by the Relativistic Heavy Ion Collider at Brookhaven National Laboratory, so they could become large-scale users of helium.
The Reynolds number is a figure of merit that characterizes the flow of a fluid around an object such as an airplane or a ship. It is proportional to the product of a length of the object, the density of the fluid, and its velocity, and is inversely proportional to the fluid's viscosity. When a scale model is tested in a wind tunnel, the test is realistic only if the flow over the model is the same as the one the real plane would experience in flight. To achieve this, the Reynolds numbers of the real object and the test object must be the same. This can be difficult if the real object is much larger than the model, because the Reynolds number depends on the size of the object under test. One solution to the problem has been to increase the speed of the gas passing over the model. Indeed there are supersonic wind tunnels that use helium gas. There are limits to this approach, however. Building larger models and larger wind tunnels is too expensive, and thus the highest number achieved is typically 10 million. A submarine moving in water can have a Reynolds number as high as a billion. Liquid helium just above its superfluid transition has a very low viscosity and can be used to achieve very high Reynolds numbers.
Standard wind tunnels achieve Reynolds numbers of 106. A liquid-helium flow tunnel can achieve values of 109. Using cold, gaseous helium, Rayleigh numbers of 1016 to 1020 can be achieved, depending on the scale. For purposes of comparison, the Reynolds number of a Boeing 747 fuselage is 5 × 108 and that of a nuclear submarine is 109. The Rayleigh numbers of the atmosphere, ocean, and Sun are 109, 1020, and 1021, respectively.
The Rayleigh number is a figure of merit for convection. Supercold gaseous helium (i.e., helium just above its liquid-gas critical point) can be used in a structure known as a Bénard cell to study convection phenomena. This is possible because buoyancy forces dominate viscous forces in low-viscosity helium gas, leading to high Rayleigh numbers and convective behavior.
Superconducting Electronics
Superconducting electronics systems are being developed for use in radar and electronic warfare. Various switches and analog-to-digital and digital-to-analog converter systems are also being developed for communications applications. Superconducting systems using Rapid Single-Flux Quantum Logic are currently the leading technology under consideration for petaflop computing.
With a limited number of exceptions, liquid helium or cryocoolers using helium are required for all of the systems that are currently commercial or under development. Closed-cycle refrigeration is an alternative to current practices in which helium boil-off gas is expended. After the discovery of high-temperature superconductors, their use in tunneling junctions appeared to be very promising. It was believed that they could lead to a superconducting electronics technology that would not require helium. This may ultimately be possible, but probably not in large computer systems and other applications, such as ultrahigh-speed analog-to-digital and digital-to-analog converters that require large numbers of tunneling junctions on a single chip. The junctions in these systems must have tightly controlled parameters to meet operating margins. It is likely to be a very long time before chips using high-temperature superconductors can be made, and these complicated systems, if they are ever built, will probably require liquid-helium temperatures.