3
Research Opportunities and Functional Roles of Materials
This chapter describes research opportunities in materials science and engineering in terms of the functional roles of various classes of materials. Although such an approach emphasizes the connection between basic research and applications, the discussions in the following sections focus on the scientific and technological frontiers that lead to development of new materials and to processing of new and conventional materials. The power of materials science and engineering is most apparent when it combines intellectual, scientific, and technological opportunities at these frontiers, while also addressing societal needs.
The practical exploration of research on new materials and processes has grown increasingly effective as understanding of composition, microstructure, and properties has progressed, as the degree of control over these characteristics has increased, and as theoretical models have been applied to increasingly complex problems. Materials researchers can now analyze and manipulate the properties of materials in ways that were hard to imagine just a few years ago.
At the atomic level, instruments such as the scanning tunneling microscope and the atomic resolution transmission electron microscope can reveal, with atom-by-atom resolution, the structures of materials. Ion beam, molecular beam, and other types of equipment can build structures atom layer by atom layer. Instruments can monitor processes in materials on time scales so short that the various stages in atomic rearrangements and chemical reactions can be distinguished. Computers are becoming powerful enough to allow predictions of structures and of time-dependent processes, starting with nothing more than the atomic numbers of the constituents.
At higher levels of structure, researchers are beginning to understand, and build, structures with crystals or “grains” that contain only small groupings of atoms, in which as many atoms lie in the grain boundaries as in the grains themselves. Researchers are also finding new properties in “nanocomposites” —composites on the scale of nanometers. Element sizes in electronic chips are rapidly decreasing and are approaching the size of small groups of atoms.
The level of microstructure and macrostructure above the nanometer scale continues to have rich promise for research. Developments at this level include modern composites, directionally solidified high-temperature turbine blades, and flaw-tolerant ceramics. Development and application of modern fracture mechanics and design have also been important. Much of the innovation and development in modern processing is concerned with controlling structure at this level as well as at finer levels. Examples include new strip casting processes and near-net-shape forming. Major opportunities exist for computer modeling to aid development of new processes and more rapid introduction of new designs and novel production processes.
The following sections describe selected research opportunities for structural, electronic, magnetic, photonic, and superconducting materials and for biomaterials. The list is by no means intended to be complete, but rather to illustrate the vitality and rich promise of the field. The emphasis on function underscores the use of materials; it makes explicit the link between fundamental research and the applications of research; it highlights the opportunities for research to contribute to areas of societal need.
STRUCTURAL MATERIALS
The properties of structural materials—toughness, strength, hardness, stiffness, and weight, for example—are determined by the interaction of atoms by their arrangement as manifested in molecules, crystalline and non-crystalline arrays, and defects, and by higher levels of structure including flaws and other microscopic heterogeneities. Consequently, the ability to predict and control materials structure at all levels, from the lattice dimensions to the macroscopic level, is central to developing structural materials that achieve the level of performance needed to accomplish the nation’s technological, economic, and military goals.
Two examples bring this relationship into focus. Without major advances in propulsion technology, reducing the structural weight of launch vehicles is the only means of lowering the prohibitive cost of transporting heavy commercial, scientific, and military payloads into orbit. Reducing structural weight will require new materials with very high strength-to-weight ratios and the capacity to withstand high temperatures. Currently, the materials
used to make the structural components of the Space Shuttle and today’s expendable rockets are essentially improved versions of those used in the Apollo program. These materials are approaching their technical limits. Given that the gap between the discovery of a new high-performance material and its actual production may be as great as 15 years, today’s basic research should be acquiring the knowledge needed to design and fabricate the structural materials required for aerospace applications during the first decades of the next century.
Similarly, satisfactory disposal of high-level radioactive waste will depend, in large part, on R&D in materials science and engineering. Plans now call for incorporating waste materials into a relatively insoluble material, probably a glass or a ceramic. Then the integrated mass will be encased in a canister that, first, must withstand any potential accident during shipment to a waste repository and, second, must remain impervious for centuries. The canister and the waste form itself must be able to withstand the assaults of corrosion from the outside and the attacks of radiation from the inside. The search to identify and fabricate materials that can satisfy these requirements is under way, but fundamental understanding of the properties of materials and of the forces that degrade them and reduce their performance would advance these efforts.
Over recent decades, the development of structural materials has evolved from an activity guided almost entirely by empiricism to one in which theory is playing an increasingly important role. The design of alloys, for example, has benefited greatly from the application of fundamental principles. Yet theoretical inputs to these efforts and those addressed to other materials classes are largely in the form of qualitative guidelines. Given the complexity of most materials systems and the complexity of the mechanisms of deformation, fracture, and degradation, this is not surprising. The opportunity now exists, however, to fill important gaps in understanding and to develop theories that provide quantitative guidelines for the design of materials. With new instruments such as the scanning tunneling microscope and the atomic resolution transmission electron microscope, it is possible to view defects on an atomic scale. Supercomputers can now perform the many calculations required to determine the properties of a particular combination and arrangement of atoms. Further, process modeling and incorporation of novel processing techniques permit creation of some microstructures under reproducible conditions. Using these tools, the materials scientist is busily designing experiments that address fundamental questions about materials systems, the answers to which can provide the information necessary for erecting a unifying theoretical framework that fosters discovery.
The examples below highlight some important issues arising from basic research on structural materials, arranged according to materials class. The spectrum of structural materials includes metals, ceramics, polymers, and
composites. In a complex system, it is common to have niche applications for each of these.
Metals
The central role of processing in controlling and improving the performance of structural materials, first recognized in the late 1960s, has become a major focus in this area in the past decade. Processing is now viewed as complementary to the chemistry and physics of materials as a tool in controlling properties.
The advent of new materials, computer controls, and new sensing devices in combination with the use of process modeling and the concepts of artificial intelligence, as well as other new technologies, has permitted the recent development of a wide range of important and exciting new processing methods. These new processes have had an enormous impact on the cost and quality of materials. Rapid and efficient adoption of such processes has significantly affected the economic well-being of industries and countries that have adopted them, and continuing important developments in this area can be expected.
In the case of metals, continuous casting is an important example of an exciting new processing method developed in the past two decades, and extension of that technology to thin-slab and strip casting is the focus of much current effort. The broad area of application of magnetohydrodynamics to metal (and semiconductor) production and fabrication is still a largely unexplored field. Recent and past successes in the area include electromagnetic stirring, dampening of convection, moldless continuous casting, levitation, pumping, containment, and forming. New, important net shape forming processes for metals include injection molding, semisolid forming, innovative and economically promising investment casting techniques, hot chamber die casting, and spray forming. The last technique can combine the benefits of net shape processing and rapid solidification processing.
In the field of surface treatments and coatings, newer plasma processes permit large-area deposition of a wide range of coatings for decorative, protective, or functional purposes. Laser surface treatment (Figure 3.1) and laser cutting and shaping are being used more widely.
Important research opportunities exist in metal processing, and processing improvements are essential to reduce costs and maintain a viable U.S. metals industry. Critical processing needs of the industry derive from its role as a consumer of ores and other metal sources. Technology for efficiently producing high-quality metals and semifinished shapes from primary ores and from recycled scrap metal and metal-containing waste materials is clearly the most important processing need. To become cost-competitive in an
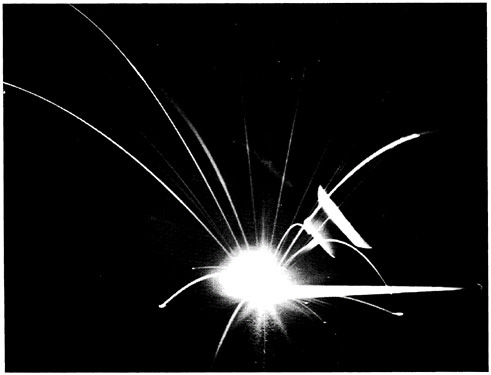
FIGURE 3.1 Laser and material interaction during welding. High-power carbon dioxide lasers provide a unique thermal energy source for processing materials. This energy source is precisely controllable in intensity and position. Laser beam welding is currently being used to fabricate components for naval structures and platforms, and its use is expanding rapidly. (Courtesy Naval Research Laboratory.)
international market, the steel, lead, and zinc industries need new processes to utilize fully their ore reserves in North America. The lead and zinc industries also need processing technology specifically related to the characteristics of their ore deposits. A significant opportunity exists to fully exploit the value of silver and other minor constituents in minerals.
Secondary processing operations such as rolling and shaping need attention throughout the metals industry. Process simulation modeling, sensing, and control improvements are needed to upgrade quality and reduce costs. New opportunities now exist in these areas as a result of developments in related technological fields.
Cooperative activities involving both users and producers are the most effective way of developing metal products. Product improvements will play an important role in maintaining market share in the competition of metals with ceramics, polymers, and composite materials. There is an excellent opportunity for innovative product research that will enable accurate predic-
tion of metallurgical phenomena and product properties. This ability should be as quantitative as possible, hence the need for emphasis on models to relate process variables and product properties. Product properties studied should include the conventional mechanical properties in addition to those related to modern coating and joining processes. Fabrication technology, with emphasis on the applications of robotization and computer-integrated manufacturing, also needs significant attention.
Researchers working on new metallic materials can now also focus on innovative processing techniques to produce “materials by design.” In this approach, the alloy designer can anticipate being able to engineer metals alone or in combination with ceramics, or in different chemical states, to optimize performance. Examples include the following:
-
Very fine grained, single-phase materials. These are achievable through advanced processing techniques, such as rapid solidification processing, permitting the attainment of structural refinement and control of metastable and equilibrium-phase transformation. Such materials can have excellent combinations of strength, ductility, and corrosion resistance.
-
Dispersion-strengthened and microduplex alloys. Again, advanced techniques such as mechanical alloying can create unique microstructural configurations leading, for example, to ideal multiaxial properties and microstructures that are stable at high temperatures.
-
Intermetallic compounds. Unique combinations of heretofore difficult-to-form compounds are now possible in polycrystalline, single-crystal, and amorphous forms by the use of novel processing techniques as well as compositional control and trace element additions. These promise to allow for higher-temperature, load-bearing applications.
-
Composites. Innovative combinations of metal matrix, intermetallic matrix, and ceramic matrix composites are clear examples of materials produced by design. Again, processing is the controlling parameter.
-
Thin films and layered structures. These are, perhaps, the ultimate examples of creating unique microstructures, literally by atom-by-atom layering.
-
Alloys resistant to radiation damage. Surface modification is one technique for developing such alloys. Other approaches based on ceramic or polymer matrices cannot be implemented until researchers learn how to design systems with less ductile materials and to incorporate them into structures. To accomplish this will require a multidisciplinary approach, including calculation (theory-assisted engineering) and modeling, particularly of micromechanical aspects.
Achieving the ideal microstructure for a particular materials system requires fundamental understanding of the many mechanisms of failure—brittle and ductile fracture, fatigue, creep, friction, and wear. Each is the product
of complex interactions, but mathematical models of failure mechanisms are limited to simple two-dimensional systems or are based on averages that mask many important details. These models must be extended, and new ones developed, to account for the interaction of atoms in three dimensions. The use of the whole arsenal of characterization tools and a complete understanding of synthesis-processing-structure-property relationships will also be needed. It is anticipated that the latter, in particular, will be aided by artificial intelligence and expert systems and by the development of reliable data bases.
Ceramics
Not so many years ago, ceramics processing comprised predominantly separation and crushing of naturally occurring minerals, followed by sintering. Today, many advanced and even conventional ceramics are produced chemically from pure materials with vapor processing, aqueous precipitation, or sol-gel techniques. Melt processing, melt alloying, and melt refinement—followed sometimes by rapid solidification—are used in producing a wide range of ceramic materials, including abrasives. Hot isostatic pressing, forging, and extrusion of ceramics are now, or will be, playing an important role in forming ceramics.
New processes developed specifically for advanced structure control include many of the above processes. Ultrafine grain sizes, useful in a wide range of materials, are obtained (depending on the part or material) by condensation from the vapor, controlled rolling, heterogeneous nucleation, and electromagnetic stirring. Amorphous or glassy structures are obtained by rapid solidification processing, vapor deposition, electrodeposition, and other solidification processes that operate far from equilibrium. Oriented grains or crystals are obtained by a wide range of processes to control crystallization or recrystallization behavior. Processes have also been developed to control structure at the level of the lattice spacing. Low-dislocation or dislocation-free single crystals are now commonly grown from the melt or are obtained through subsequent processing; much improvement in the quality of such crystals is needed and can be expected in the years ahead.
The appeal of ceramics as structural materials is easy to understand. Ceramics are light, but their compressive strength matches or exceeds that of metals; they can withstand extremely high temperatures; they are exceptionally hard, are resistant to abrasion, and are chemically inert; and they excel as electrical and thermal insulators. If research can add two other properties to this list—tensile fracture toughness and ease of processing—ceramics are likely to become ubiquitous in structural applications.
How rapidly ceramics will achieve their potential will be determined largely by research on processing techniques, which first must overcome the problem of brittleness and then must prove to be economical. One processing re-
quirement is either to eliminate defects—the voids, agglomerations, and chemical impurities from which cracks originate—or to toughen ceramics by devising ways to prevent cracks from spreading.
Several approaches yield pure, minute particles, the starting materials needed to achieve a void-free microstructure during synthesis. Attrition, melt atomization, and physical vapor deposition produce such particles without chemical change. Chemical precursors are involved in sol-gel and thermal degradation processes.
Dense ceramic bodies are usually obtained by pressing and sintering of fine particles. Modern processes include hot isostatic pressing and forging, which apply pressure during the sintering process. Dense bodies are also formed by direct casting of the melt and by processes that involve oxidation of a melt to obtain a ceramic or metal-ceramic composite. Infiltration of a ceramic precursor with a melt or vapor is another method used, and vapor forming is yet another. In self-propagating, high-temperature synthesis, reactive species are heated in a mold under pressure and are allowed to heat to a temperature at which they react and densify.
Developing tough, strong ceramics is another important, major goal of much ceramics research today. Transformation toughening is one important method, a practical example of which is seen in ceramics whose structure is composed of at least partly tetragonal zirconia. As a crack begins to grow in such ceramics, the tetragonal structure becomes monoclinic, with a resulting volume change of 3 to 5 percent that arrests crack growth. Fifteenfold increases in toughness have been achieved in such materials, and important structural ceramics and abrasive materials are built on these compositions. Other ways of improving the toughness of ceramics involve achieving and maintaining very small grain sizes within the ceramic body, incorporating controlled and dispersed voids, and synthesizing ceramic-ceramic composites. Toughening mechanisms in a ceramic are illustrated in Figure 3.2. An example of a ceramic composite (silicon carbide in alumina) is shown in Figure 3.3.
Research on new toughening mechanisms, as well as on new processing technologies, offers much promise for the future. Concomitant advances in nondestructive testing will also be needed. X-ray tomography, ultrasound, and other existing nondestructive testing methods must be refined to improve flaw detection, and new methods should be developed for ceramics systems. Probabilistic design methodology can play an important role in predicting the performance of ceramic parts. Modeling efforts, however, will require statistically valid data on crack propagation and the stress behavior of ceramics.
One exciting new development is the growth of diamond or diamondlike materials on the surface of various substrates. Whereas previously diamonds were grown at high pressures and temperatures, it is now possible to grow
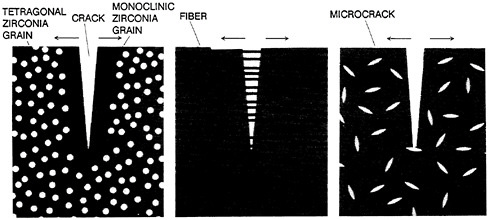
FIGURE 3.2 Toughening mechanisms, by which a crack in a ceramic can be arrested, complement new processing techniques that seek to eliminate crack-initiating imperfections. Transformation toughening (left) relies on a change in crystal structure that zirconia grains undergo when they are subjected to stresses at a crack tip. Ceramics can also be made crack-resistant by interlacing with fine ceramic fibers (middle), as is the case in composite materials. A third way to stop a crack is by spreading the stresses concentrated at its tip over a larger surface. This can be accomplished if minute cracks, called microcracks (right), are purposely created in the ceramic material during processing. (Reprinted, by permission, from H.Kent Bown, 1986, Advanced Ceramics, Sci. Am. 255:169–176. Copyright © 1986 by Scientific American, Inc. All rights reserved.)
crystalline diamond films by energetically assisted chemical vapor deposition processes at far lower temperatures and at low pressures. Diamond films may find application as wear-resistant coatings and as bearing surfaces. Their high thermal conductivity already has led to use of diamond materials as heat sinks in electronic applications, while their combined thermal conductivity, low expansion coefficient, and strength provide high resistance to thermal shock, a characteristic desirable for instrument windows. Applications for diamond films will expand rapidly in the future as the availability of large-area films increases.
Polymers
Polymers are notable for the unique combinations of properties that are possible. To the mechanical attributes of high strength, high flexibility, and light weight can be added thermal, electrical, and optical properties that make polymers especially well suited for specialized applications. In defense applications, for example, tough, lightweight polymers are likely to be used as armor, protecting against projectiles and lasers while offering the additional benefit of radar transparency. In the last decade, polymers have emerged
from their early role as primarily inexpensive commodity materials into the value-added, high-technology sector in which specific properties can be exploited for novel performance. Examples include piezoelectric polymers used for ultrasensitive sonar, high-temperature flame-retardant fabrics, nonstick surfaces, reusable pressure-sensitive adhesives, and encapsulants for drug delivery.
Structural polymers are already in widespread use and account for a major portion of the $100 billion global market for polymers. However, improvements in existing materials and development of new polymers with significantly enhanced properties are possible and will increase the range of structural applications and the size of the market. Progress to date is well illustrated by the remarkable strength-to-density ratio of aromatic polyamide polymers; development of other impressively strong polymers of lower cost can be expected. Polymer adhesives are already replacing rivets in many joining applications, and polymer-fiber composites are replacing metals in many
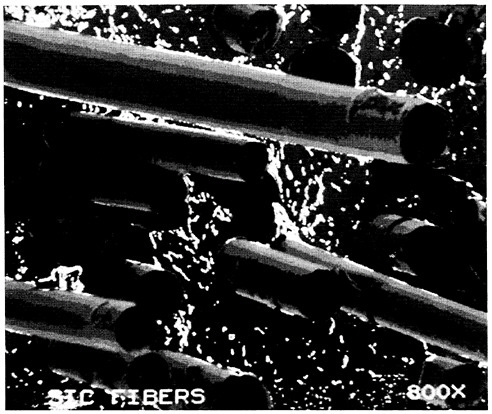
FIGURE 3.3 Fracture surface of silicon carbide-reinforced alumina. (Reprinted, by permission, from E.I.du Pont de Nemours & Co., Inc. Copyright © 1989 by E.I.du Pont de Nemours & Co., Inc.)
structural applications. Finally, polymer and polymer-fiber composite materials are finding extensive biomedical applications. All of these trends are expected to continue, and all present research challenges in synthesis and processing to achieve desired structures, properties, and performance.
Advances in computing power offer opportunities for substantial and badly needed improvements in the theoretical underpinning of polymer science. These include the modeling of stable and metastable structures of macro-molecules, of liquid crystallinity, of phase relations and phase-transition kinetics (including the now poorly understood glass transition in amorphous materials), and of nonlinear viscoelastic behavior in polymer melts (which is critical in polymer processing). The recently developed reptation concept is an interesting approach to the last problem; identification of the collapse transition of polymer solutions by use of computer-intensive Monte Carlo methods, combined with renormalization and scaling theories, illustrates the benefit of increasing computing power in modern theory.
The long-chain connectivity inherent in polymers imposes unusual constraints at or near interfaces, where abrupt changes in density, composition, and orientation can occur. Understanding polymeric interfacial problems requires sophisticated scientific studies; scaling up from analyses of small molecules will not suffice, because the behavior of polymer chains can be markedly different from that of low-molecular-weight compounds. Studies should address the physical and chemical dynamics of surfaces, interfacial regions of polymer blends and alloys, polymer melts in confined geometries, and crystal-amorphous interfaces in semicrystalline polymers. These interfaces control adhesion, wetting, colloidal stabilization, and mechanical properties, and they are intimately involved in the failure and deformation of polymers. Yet experimental and theoretical investigation of polymer interfaces has begun only recently.
Chemistry is important to all the materials classes, but none benefits more from close interaction with the field of chemistry than does polymers. The synthetic capabilities of the modern chemist can be advantageously applied in the rational design of molecules to achieve desired properties. Specificity in molecular composition, architecture, and size can be tailored with amazing precision. The attachment of functional groups at selected locations is readily accomplished. Moreover, control of chain length and composition provides for extremely well-defined materials that can self-assemble into intricate patterns sometimes mimicking those of biological systems.
Interfaces are also of crucial importance to the performance of composites (see below), many of which use polymers for the matrix, the reinforcing dispersed phase, or both. Defects or weaknesses at interfaces can severely limit the performance of composites. Figure 3.4 shows a carbon fiber-reinforced composite fracture surface. Recent research on polymers, however, suggests a route to eliminating interfaces while achieving many of the su-
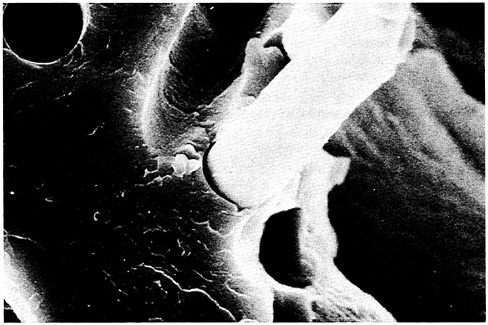
FIGURE 3.4 Micrograph of carbon fiber-reinforced composite fracture surface. (Reprinted, by permission, from E.I.du Pont de Nemours & Co., Inc. Copyright © 1989 by E.I.du Pont de Nemours & Co., Inc.)
perlative properties that composites can offer. Now under investigation are methods to achieve molecular mixing in which rigid backbone molecules are uniformly dispersed in a matrix of flexible-coil polymers. The result is a “molecular composite,” akin to a fiber-reinforced composite with the individual rigid macromolecules acting as the “fiber.” The uniform dispersion creates a polymer mixture of long-range molecular order and, thus, with no interfaces. Molecular composites are expected to have superior impact resistance, fracture toughness, and compressive strength, while offering opportunities for novel combinations of electrical and optical properties.
Research on molecular composites is in the very early stages. Progress will depend on finding answers to questions about the nature of liquid-crystalline polymers. The physics of the isotropic-anisotropic phase transition must be elucidated, and much more must be learned about chain conformations and intermolecular packing in mesomorphic states and about the effects of chemical structure and chemical reactions between chains on long-range order. Molecular composites are but one of many exciting technologies that are likely to emerge from fundamental research on polymers.
Composites
As should be apparent by now, the traditional categories for classifying materials can impose artificial distinctions. Although some research issues
are unique to one class of materials such as metals, many span the categories of materials or stem from technological applications that exploit the properties of a range of materials. Nowhere is this overlap of scientific interest and resultant benefits more apparent than in the area of composites.
Loosely defined, composites are hybrid creations made of two or more materials that maintain their identities when combined. The materials are chosen so that the properties of one constituent enhance the deficient properties of the other. Usually, a given property of a composite lies between the values for each constituent, but not always. Sometimes the property of a composite is dramatically better than that of either of the constituents. The potential for such synergy is one reason for the tremendous interest in composites for high-performance applications.
Understanding and control of the chemical changes that occur during creation of composites will be critical to achieving the enormous potential utility of these materials. This is a formidable challenge, requiring the contributions of experimentalists and theoreticians in metallurgy, polymer science, ceramics, organic and physical chemistry, rheology, and solid-state physics. Determining the molecular changes that occur in bulk materials during processing, which involves many steps, will be an extraordinarily difficult problem. The chemical dynamics of curing polymeric matrix composites is fairly well understood, at least in a qualitative sense, but very little is known about the reactions occurring during the production of metal matrix and ceramic matrix composites. Filling these serious gaps in understanding would advance efforts to develop fabrication methods to achieve the property improvements that are theoretically possible for a particular combination of materials.
Interfaces constitute a research area of obvious importance. Unusual physical and chemical processes occur at the boundaries between the reinforcing phase—particles, whiskers, or fibers—and the matrix. Reactions in interfacial regions can strengthen the bonding between the matrix and the reinforcing elements, or they can weaken bonding and also degrade the constituents of the composite. Moreover, weaknesses at interfaces, due to defects or poor chemical compatibility between the matrix and reinforcing elements, are major sources of stress- or impact-induced failure of composites. One way to solve the problem of chemical incompatibility, which is an obstacle to promising combinations of materials, is to develop chemical treatments and additives to strengthen interfaces.
Composites also pose problems of geometry. The geometry of the reinforcement is a critical determinant of a composite’s strength. Because fibers afford maximum control over the internal structure of a composite and because of their high aspect ratios (ratio of length to diameter), long, continuous fibers are the reinforcing elements of choice in high-performance composites (Figure 3.5). The need for more extended-chain polymers, such as the recently developed high-tensile-strength polyethylene fibers, is clear.
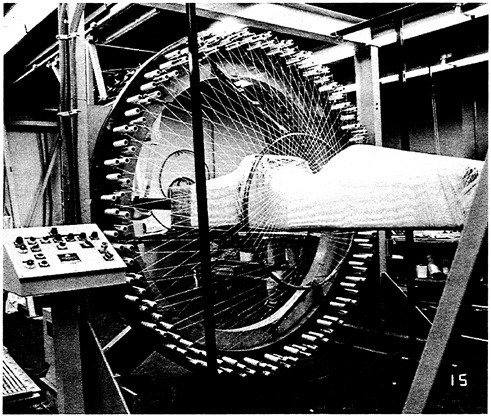
FIGURE 3.5 Computer-controlled 144 carrier braiding machine in the process of forming a fiberglass monocoque chassis for a composite racing car. (Courtesy Frank K.Ko, Fibrous Materials Research Center, Drexel University.)
Unless manufacturing difficulties are overcome, many promising composites, especially ceramic and metal matrix composites, may never fulfill their commercial promise. Because manufacturing involves many steps and is labor intensive, composites may be too expensive to compete with metals and polymers, even if their properties are superior. New processing technologies are needed—and not only to reduce costs of production. The high temperatures necessary for processing many ceramic and metal matrices can damage and weaken reinforcing elements. As a result, current processing technologies do not permit combinations of certain materials with especially desirable properties.
Methods for joining composites to other materials represent a research area of practical importance. Because the chemical bonding in composites is unlike that of most other materials, parts made of composites are difficult to incorporate into complex assemblies. If properties of the joining material
do not equal those of the composite, the advantages of using a composite part can be nullified.
ELECTRONIC MATERIALS
Electronic materials can be semiconductors, polymers, ceramics, metals, or insulators. These materials and the processes used to fabricate them are being pushed to their limits by aggressive worldwide competition throughout the electronics industry. This has created a need for research on the fundamental limits of present technology, how these limits can be achieved by new processing methods, and what new materials or fundamental concepts will evolve to overcome these limits.
Optimization or development of individual electronic materials must be accompanied by fundamental research into how materials interact with one another. For example, information processing and communication to the external world take place through metal lines connecting semiconductor chips, logic and memory circuits, ceramic or polymer chip-carrier modules, and polymer-fiber composite cards and boards. Incompatibility between the various parts of a desired electronic component can often deter the implementation of new concepts. To enhance technology, research must take into consideration the entire materials and processing environment.
Semiconductors
Semiconductors—the source of transistors, integrated circuits, solid-state lasers, and detectors—are the central constituents of most electronic systems. Although other materials are finding an increasing range of uses, the dominant material in the electronics industry is still silicon. Among its attractive properties are its high mechanical strength, its high degree of crystalline perfection, the large diameters to which it can be grown, its natural abundance, the advantageous properties of its native oxide, and its low cost. By taking advantage of these and other capabilities of silicon, researchers have achieved high levels of circuit integration.
Competition is currently focused on dynamic memory chips, in which increased circuit density results in lower cost, higher data retrieval rates, and increased capabilities for small systems. Because devices are processed in surface arrays, lithography is of major importance, with a twofold reduction in the linear dimension of a memory cell resulting in approximately a fourfold increase in memory density. As feature sizes are further reduced, photoresist exposure to produce patterns of smaller dimensions must be accomplished with light of decreasing wavelengths. New light sources, such as synchrotron x-ray beams or deep-ultraviolet lasers, and new organic photoresist materials sensitive to the decreased wavelengths must be developed. Recent research
has shown that patterns written directly with an electron beam can produce working devices with dimensions as narrow as 0.1 µm, a development that illustrates the enormous future potential of efforts to transfer new lithographic tools to industry. Control of device processing at these dimensions is equally challenging, because diffusional processes that occur with normal high-temperature fabrication techniques spread dopant profiles and reduce performance. This is causing a redirection of research toward the low-temperature processing of materials and devices, with emphasis on the thermodynamics of reactions, laser- or plasma-enhanced processing, and a more atomistic approach to understanding the behavior of materials and interactions between materials.
The advent of high-vacuum techniques for depositing epitaxial layers at low temperatures, such as molecular beam epitaxy (MBE) and chemical vapor deposition (CVD), has opened a new world of opportunity in research as well as device fabrication. Discrete thin layers can be deposited to form narrow electronic transitions or superlattices, in which multiple layers with differing compositions are alternately deposited. These techniques have been applied to silicon electronic materials. Silicon-germanium alloy layers have been grown on silicon, and devices have been fabricated whose properties depend on the difference in electronic structure between the two materials. This new flexibility will greatly extend both the range of applications of silicon technology and the ultimate performance limits of devices. Figure 3.6 illustrates strained-layer superlattices formed through epitaxial techniques whereby electronic structure is tightly controlled.
Although silicon will dominate the electronics community for the foreseeable future, much research effort is being devoted to the development of III–V semiconductor compounds such as gallium arsenide (GaAs) for specific applications. Because of their electronic structures, their high electron mobilities, and the manner in which excited electrons in III–V compounds lose their energy by emitting light, III–V materials provide higher-frequency capabilities than silicon does, and they are optically active, being both laser sources and detectors. A wide range of technologies that use these features are being investigated, such as data transmission over optical fibers, holographic image transfer, and optical storage media on disks or tapes.
One of the most important features of these materials is the number of permutations possible between elements in the group III and group V families. For example, gallium, aluminum, or indium can be combined with phosphorus, arsenic, or antimony in ternary or quaternary compounds and in multilayers produced by MBE or CVD. The ranges of laser wavelengths and device structures that can be achieved are extensive, and their limits are unknown.
Compared with elemental silicon, application of GaAs is restricted by severe processing problems related to the different chemical reactivities of
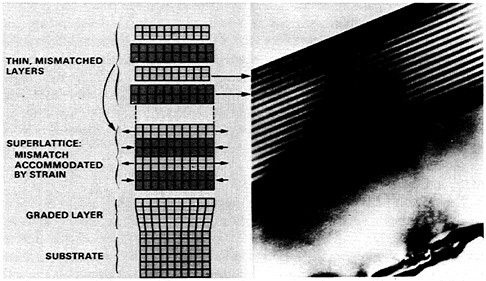
FIGURE 3.6 Strained-layer superlattices for band gap engineering. Transmission electron micrograph of a series of alternating 100-angstrom GaAs and InGaAs layers with the illustration depicting the distortion in the equilibrium lattice spacing for the two compounds that occurs in the stable structure. Stability criteria for these systems depend on the amount of lattice mismatch and the layer thicknesses, but typical cases have a 20 percent mismatch in 100-angstrom layers. Strained-layer materials are playing an increasing role in the rapidly emerging optoelectronic technologies and in a wide variety of electronic devices. By controlling the composition and thickness of the layers, researchers can optimize the electronic properties of devices. (Courtesy Sandia National Laboratories.)
the elements. Also, structural and chemical defects must be eliminated, contact fabrication is difficult, and native oxides are inadequate for use in devices. These and other materials issues must be overcome if more widespread applications of III–V compounds are to be realized.
As basic knowledge of surfaces and epitaxial layer formation has evolved, new emphasis has been given to heteroepitaxy of one material on another to achieve novel devices or to use the best features of each layer. For example, GaAs is being grown on silicon for integrated optics, in which laser or detector devices are directly associated with silicon circuitry. Vertically integrated structures containing epitaxial insulators and metals are also being studied—for example, calcium fluoride or cobalt silicide on silicon. Growth processes are difficult to control for these materials, requiring the development of new high-resolution techniques for interface studies. Until basic issues related to structural defects can be resolved, such materials will remain the subject of research. Figure 3.7 illustrates extremely small silicon structures prepared by lateral thermal oxidation processes.
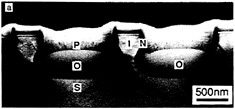
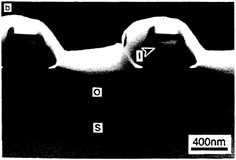
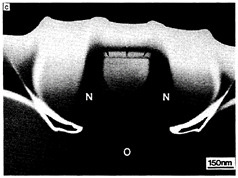
FIGURE 3.7 Artificially structured materials for microelectronics: isolated silicon islands. Future microelectronic circuits will require progressively smaller electrically isolated areas of single-crystal silicon. Panels a, b, and c show transmission electron microscope (TEM) images of isolated single-crystal silicon islands prepared by the lateral thermal oxidation of single-crystal silicon trench structures capped with silicon nitride. The patterns are produced by electron beam lithography, and the thin films are deposited by chemical vapor deposition processes, (a) TEM cross section showing silicon-on-insulator structures; the silicon islands have not been completely isolated. Labels indicate silicon substrate (S), silicon dioxide (O), polysilicon (P), silicon nitride (N), and silicon island (I). The dark lines are defects (dislocations) induced during processing, (b) TEM micrograph showing structures where the silicon islands have been completely isolated by encroaching oxide. Note reduction in defect density, (c) High-magnification TEM micrograph of an isolated silicon island shown in (b). The small island (outlined by arrows) is approximately 200 nm (1/500 the diameter of a human hair) on a side. (Courtesy N.C.MacDonald, School of Electrical Engineering, Cornell University.)
Ceramics and Polymers
Electronic packages, consisting mainly of wiring and interconnections, provide the interface between active semiconductor devices and the external world. The levels of packaging technology involved are related primarily to the size and to the performance requirements of the application, ranging from one or a few semiconductor chips to massively integrated, large computer systems. The requirements for a substrate material onto which chips can be attached are easily understood, but in practice these materials generate a complex set of interactions that need to be resolved. Ceramic substrates are currently used when high circuit density and multiple chips are needed and when mechanical stability is important. Substrates are fabricated by screening
metal patterns onto thin, prefired ceramic layers, stacking the layers, and firing at high temperatures to densify and strengthen the mass. For alumina, which is the ceramic presently used, the choice of conductor metal is restricted to molybdenum because of the need for excessive firing temperatures. However, the dielectric constant of alumina is too high and molybdenum is too resistive, resulting in relatively poor electric characteristics for the transmission lines. Also, the thermal coefficient of expansion of alumina is not the same as that for silicon, leading to high stress and failure when chips are attached and powered.
New substrate materials must be developed that have lower fabrication temperatures, lower dielectric constants, high strength, and thermal expansion coefficients matched for those of silicon. Compatibility during processing between the substrate matrix, the metal conductors, and the semiconductor chips has been elusive and will require more research into interface interactions, fracture propagation, and thermal relaxation mechanisms. If ceramics are to remain competitive for chip substrates, dielectric constants must be reduced by techniques such as microscopic porosity, and surface roughness must be eliminated to facilitate lithographic processing of metal lines.
There is a significant trend away from ceramics and toward polymers for substrate packaging materials. Polymer layers have intrinsically lower dielectric constants and preparation temperatures, can be applied onto a surface with controllable flatness, and are compatible with lithographic processing of metal transmission lines. Mechanical stability and heat dissipation are areas requiring further research, but hybrid ceramic-polymer layers are now being produced in which the polymer layers are processed on top of or adjacent to chips when metal lines of the highest density and highest performance are required. New polymeric materials will be needed in which thermal expansion coefficients are reduced to match the chip and ceramic, strength is increased to prevent cracking, and dielectric constants are further reduced, perhaps by the use of fluorocarbon-like chemistries. Adding moisture resistance, thermal stability, and perhaps photosensitivity to the list illustrates the complex combination of requirements that apply to new polymer materials.
The printed circuit boards onto which chip-carrier modules are mounted have been made of epoxy-filled glass cloth for some time. The reinforced structure has a thermal expansion coefficient characteristic of the composite, with strength being provided by the glass. Research on this material is seeking to lower the dielectric constant by using aromatic polyamide polymers to replace the glass, and to replace the epoxy with a more compatible material. Structural integrity and compatibility with high wiring densities are further areas for research.
Many other important electronic applications for ceramics and polymers are the subjects of research, including capacitor coatings for substrates and chips, piezoelectrics, ferrite recording-head elements, bistable switching, photosensitive polymers for lithography, polymer substrates for optical storage, and high-strength polymers spun from liquid crystal solutions. Impressive advances have been made in recent years in the field of electroactive polymers. Conjugated polymers (polymers that have overlapping π orbitals along their backbone) have been reported with conductivity approaching that of copper at room temperature. Continuing work in this field is needed in processability and in performance, especially with respect to environmental stability.
Metals
Metallic components serve a number of functions in electronic systems, from transmission lines within and between packing levels to magnetooptic or magnetic films for information storage. Because high device densities in semiconductor chips cannot be achieved without providing the necessary wiring between devices or circuits on the chip, wires have to be reduced in size to the point at which issues of reliability become overriding. Wiring is also distributed vertically over many layers processed on the chip, not unlike the multilayered wiring processed on substrates, except with much finer dimensions. Research issues include the stability of the contacts made to the semiconductors; interdiffusion between, on the one hand, metal layers, contacts, and conductor lines, and, on the other hand, the structures on the chip and substrate; reliability at the high current densities resulting from finer lithography; structural and chemical compatibility with the insulating materials that separate the wiring layers; and the effects of environmental attack. New metal deposition processes using vapor transport and radiation enhancement are being developed to expand the list of candidate metals and diffusion barriers that can be implemented. Specific metallurgy questions may vary between silicon and GaAs, but the fundamental scientific issues are similar. Figure 3.8 shows multilayer ceramic packages with metallic conductor lines deposited on the ceramic substrate providing complex interconnections among active devices.
Developing new materials and processes and performing extraordinary research and laboratory work are necessary but are not sufficient to ensure the competitiveness of the electronics industry. Engineering of needed advances into manufactured products is one of the biggest challenges facing the materials community. Those companies that are proficient at this step of the innovation process will be leading edge competitors.
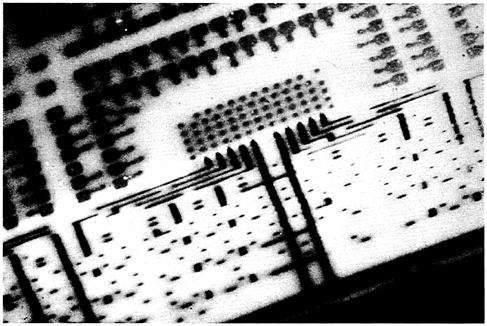
FIGURE 3.8 Multilayer ceramic packages. (Courtesy IBM Corporation.)
MAGNETIC MATERIALS
Magnetic materials have received less public attention during recent decades than have semiconducting or superconducting materials. Nevertheless, the intrinsic scientific issues and opportunities in the study of magnetic materials are equally interesting, and the existing and potential economic impacts of magnetic materials are comparably great.
The economic impact is perhaps easiest to see. Magnetic materials underlie applications in information storage, audio and video recording, microwave communications, and permanent magnets for products ranging from motors to wall clips. Of these applications, the largest by far is the magnetic storage industry. The entire field of data processing stands on two legs—semiconductor logic and memory to process data, and magnetic devices to store data. Not surprisingly, the total yearly sales of magnetic storage systems, totaling several tens of billions of dollars, are comparable to those for the semiconductor logic and memory systems in computers.
For years, the magnetic storage industry has used standard materials—iron and chromium oxides for storage media and permalloy or ferrites for heads. The bottleneck to increasing the size and efficiency of these systems was never the magnetic materials but rather the mechanical components required to fly recording heads faster and closer to the magnetic medium. But now the magnetic materials themselves are beginning to limit further progress, because of recent developments such as thin-film heads; the demand
for higher-density storage, requiring new storage media; and the emergence of competitive new technologies, such as magnetooptic storage, that call for entirely new classes of magnetic materials. As never before, magnetic materials are the key to the future of the storage industry.
Scientific opportunities in magnetic materials, exciting in themselves, also dovetail in many cases with the technical demands of magnetic applications. An example is the study of magnetic surfaces and interfaces. Control and study of surfaces on an atomic level have proved to be more challenging for magnetic materials than for semiconducting materials. One reason is that detection of magnetic properties requires the use of so-called spin-sensitive measurement techniques, which add extra complexity to the already very sophisticated surface analytical tools used to study semiconductors and other nonmagnetic materials. However, this technology is now largely in place in many laboratories throughout the world, opening the door to an understanding of magnetic surfaces that was never before possible.
Magnetic surfaces and interfaces can give rise to anisotropies and effective fields that become an ever larger factor in the behavior of the bulk material as its thickness decreases, which will be a major consideration in future storage technologies. A specific example concerns the peculiar internal field, called exchange anisotropy, that arises at interfaces between a ferromagnet and an antiferromagnet, causing the ferromagnet to behave as if it were subject to an external field. Such an effect could potentially find application where it is awkward or costly to apply a field with an external power source. This long-puzzling phenomenon is just beginning to be understood in terms of the randomness of the interfacial magnetic structure. Synthesis of more perfect interfaces would offer a conclusive test of the mechanism.
Control of magnetic surfaces and interfaces is also the basis for synthesis of new artificial magnetic materials—multilayers and superlattices. Early experiments have already shown complex couplings between the different magnetic layers, which could be the basis for new properties not available in bulk materials. There is hope for achieving larger anisotropies, coercivities, galvanomagnetic effects, and magnetooptic effects, and there are tremendous varieties of systems to explore. Interest in this area has grown dramatically in the last few years in research groups throughout the world.
Another example of scientific opportunities comes from progress in the band theory of magnetic materials. Using novel statistical techniques and Monte Carlo calculations, researchers are now calculating the Curie temperature of iron with increasing accuracy. This had been a long-standing problem, and its solution opens the door to theoretical prediction of a variety of other magnetic properties. Many of these fall under a common umbrella, the so-called spin-orbit interaction, which gives rise to anisotropy, galvanomagnetism, and magnetooptic rotations. All of these properties have been poorly understood in the past and are decisive for many applications.
Major advances in magnetic materials have been appearing at an accel-
erating pace. New neodymium-iron-boron materials have been discovered that significantly increase the energy product of permanent magnets, thus increasing the volumetric efficiency in applications such as motors. Record magnetooptic rotations, larger by a factor of 20 than those presently used in magnetooptic systems under development, have been discovered in a new class of uranium chalcogenides. During the last decade, a whole new class of magnetic materials has been identified, called spin glasses, in which random magnetic interactions lead to complex magnetic behavior unlike any known in standard ferromagnets, ferrimagnets, and antiferromagnets. The theory of these systems constitutes a major revolution in statistical mechanics, introducing new and sophisticated concepts like distributed order parameters and nonergodic phase transitions. The analogy of random spin glass interactions and the seemingly random neuronal couplings in the brain have led to the concept of neural nets, resulting in a new theoretical approach to distributed data storage that is now being actively explored in the computer science community.
These and many other remarkable developments point to a remarkable richness of research opportunities and a large impact on applications in the field of magnetic materials.
PHOTONIC MATERIALS
Photonic materials are now where electronic materials were in the early 1950s—at the very beginning of a steep growth curve. In information technology, a photonic or optical material is any material with which light interacts for the purposes of information generation, transmission, detection, conversion, display, storage, or processing. Like electronic technology, optical technology ultimately depends on the discovery, development, and manufacture of reliable and economical materials. The past 20 years have brought unprecedented advances across all fronts. A few of the most important are briefly mentioned below.
Before the early 1970s, the most transparent glass that could be manufactured had an optical attenuation of over 1000 dB/km. Today, optical fibers are being manufactured with loss coefficients nearly 10,000 times lower. This advance has transformed the field of optical communications from a visionary dream to a commercial reality in just over a decade.
Other important advances include the invention of room-temperature semiconductor lasers and the development of ultrasensitive, low-noise detectors based on III–V semiconductor materials. The bit rate×distance product, a figure of merit for optical communications systems, increased a thousandfold in a single decade.
Epitaxial deposition of films has led to the generation of totally new semiconductor devices, called multiquantum wells, that exhibit phenomena
not found in any natural material. This discovery makes possible virtually unlimited flexibility in the design of integrated optical and optoelectronic circuits.
A whole range of new nonlinear phenomena has been discovered, and an active search is now under way for materials that optimize these phenomena for specific applications. Optical bistability provides the key to all-photonic switching and logic and quite possibly will provide the basis for an all-optical computer. Self-induced transparency and a host of related nonlinear optical propagation phenomena have recently been demonstrated in rare-earth-doped optical fibers. The photorefractive effect is a form of dynamic hologram and may form the basis for optical storage, processing, and display technologies of the future. Only a small fraction of the materials exhibiting these effects have been investigated, and the basic physics is not well understood in all cases.
Advances that bear on the display of information include the intrinsic photo-ferroelectric effect, which may be used to store high-quality images in transparent ferroelectric ceramic materials. In addition, the discovery of ferroelectric liquid crystals has led to new electrooptical devices that respond extremely rapidly to external electrical signals.
Although this section focuses on applications related to information technologies, optical materials also have uses in many other areas that are being actively investigated. Recent materials research has improved the conversion efficiencies and lowered the production costs of solar cells made from amorphous silicon, bringing this technology to the threshold of large-scale commercial viability. Ultraviolet lasers are being developed for laser fusion, while other lasers are producing very short pulses of light that can be used to study chemical reactions and other dynamic processes.
Substantial advances have been made in many other areas of photonics, such as optical phase conjugation, two-wave mixing and energy exchange, erasable optical memories, high-power optical materials, gradient-index coatings, phase transition materials, flat panel displays, and optical window materials. The development of suitable high-performance materials is the key to success in all these areas.
Virtually all of the discoveries discussed above suggest directions for further research. Listed below are a few of the obvious opportunities within the major categories.
For fibers or other transmission systems, it is important to devise new materials that have lower optical losses. Probably required are transparency windows at wavelengths longer than 1.5 µm. For use in power transmission or medical applications such as surgery, a modest-loss glass operating with a transparency window near 10 µm is important.
Important possibilities exist for the use of fibers in nonlinear optical processing—that is, in the regeneration, amplification, detection, and modu-
lation of pulsed signals. Such applications require active glasses such as silica doped with rare-earth ions.
The theory of the nonlinear propagation of novel optical modes (e.g., solitons), the interactions between these modes, and the optimization of waveguides designed for these nonlinear effects deserve increased attention.
If the speed advantage offered by photonics over electronics is ever to be matched at least by parity in the spatial domain, materials or structures must be found that permit optical modulation over micron rather that millimeter distances. Increasing the nonlinear optical response of photonic materials is thus a fundamental challenge to materials scientists and theoretical physicists.
The search for optical silicon, although difficult, is nevertheless important. Optoelectronic semiconductor materials, in addition to the group IV and III-V systems, require increased attention; II–VIs and I–III–Vs, both in bulk and superlattice forms, provide intriguing possibilities.
In the materials science of crystal growth, European and Japanese research efforts dominate U.S. efforts. There is a growing need for bulk crystals of various kinds as well as for specific magnetooptic, acoustooptic, and electrooptic materials. The scarcity of such materials often limits the scope and pace of research on new phenomena, devices, and systems. Figure 3.9 illustrates growth of high-purity lithium niobate crystals for electrooptic applications in telecommunications.
Another challenge of great significance is the extension to two and even three dimensions of the kind of control in materials fabrication that vapor deposition provides in one dimension. The extension from quantum wells to quantum wires and then to quantum dots is but one example of the power that such an advance in fabrication technology would represent.
Serious consideration should be given to a research program aimed at what might be called microprogrammable materials. The phenomena of spectral hole burning and photorefractive effects represent limited examples of this idea. It might be possible to use energetic beams to make localized changes in the chemical bonds or the structure of a material in a way that will change its function at desired points in space. For example, it might be possible to convert an insulator into a semiconductor or a semiconductor into a superconductor in spatially controlled regions.
Finally, as materials become purer and more microscopically controlled, the requirements for materials characterization become even more stringent. Monitoring the amount, identity, and location of each chemical or even each atomic constituent in situ in real time, and often during the fabrication process itself, will require substantial research in characterization. The tools and technological sophistication required are complex, and the costs of the equipment are high. Nevertheless, characterization is essential to successful materials developments, and the investment should be made.
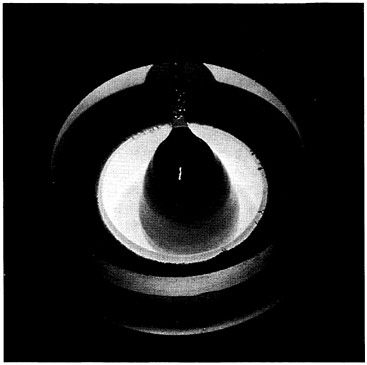
FIGURE 3.9 View of Czochralski crystal growth furnace preparing lithium niobate. Czochralski growth in which a seed is dipped into molten material contained in a crucible and slowly extracted is the principal technique for the preparation of silicon, III–V semiconductors, and refractory materials used for lasers and electrooptic modulators. Lithium niobate is an electrooptic material used for switching light signals at high speed. The melt is at a temperature above 1000°C. (Courtesy AT&T Bell Laboratories.)
SUPERCONDUCTING MATERIALS
The recent discovery of high-temperature superconductivity in ceramic oxides is one of the most dramatic breakthroughs in physics and materials science in recent decades. It has focused world attention on the field of superconductivity; more broadly, it has quickly come to symbolize the opportunity and challenge in the overall field of materials science and engineering.
Nevertheless, high-temperature superconductivity is only one part of the story of superconducting materials. Throughout the decades following H. Kamerlingh Onnes’s discovery, in 1911, of superconductivity in mercury at 4.2 K, materials with ever higher transition temperatures were steadily discovered, until 23 K in an intermetallic compound of niobium-germanium was reached in 1973. At the same time, theories developed in the middle to
late 1950s and early 1960s provided a comprehensive understanding of the existing experiments based on the formation of electron pairs mediated by lattice vibrations. With the development of superconducting magnetic materials such as niobium-titanium and niobium-tin, which can carry large currents in the presence of large magnetic fields and remain superconducting, and of Josephson devices such as superconducting quantum interference devices (SQUIDs), sales of complete systems based on superconducting components have approached $1 billion annually.
In spite of the long hiatus in increasing transition temperatures beyond 23 K, the field of superconducting materials has not remained static. In fact, since 1973 a series of novel materials and systems have been discovered and studied. Magnetic superconductors have been found in which superconductivity and magnetism coexist (normally, superconducting materials expel magnetic fields), superconductors containing organic materials have been developed, artificially structured arrays have been shown to exhibit complex behavior known as frustration, ultrasmall junctions have displayed novel quantum behavior not seen in standard Josephson junctions, and heavy-fermion superconductors have demonstrated novel types of pairing. These studies have raised fundamental issues about the nature of the microscopic pairing mechanisms in highly correlated electron systems, the effects of disorder, and the underlying quantum description of dissipative systems.
These superconducting materials, which so far have shown low to modest transition temperatures, have not captured the public or wider scientific imagination. However, their underlying physics and the materials issues associated with them are of great complexity and interest. At present, the questions they raise are by no means resolved and deserve further attention. And the possibility for setting new transition temperature records should not be discounted: there has been steady progress, for example, in raising the transition temperatures of organic superconductors to a level approaching that of “pre-Bednorz-Muller” oxides.
Building on the earlier discovery of superconductivity at up to about 13 K in certain oxides, J.Georg Bednorz and Karl Alex Muller in early 1986 found superconductivity at over 30 K in a class of ceramic oxides containing lanthanum, barium, and copper. Shortly after this result was verified in December 1986, superconductivity at up to about 95 K was discovered in a compound of yttrium-barium-copper-oxygen with a structure now dubbed “123.”
More recently, zero resistance at up to 107 K has been reported in layered structures of bismuth-strontium-calcium-copper-oxygen. Related structures of thallium-barium-calcium-copper-oxygen have pushed the zero-resistance point to 125 K. It is noteworthy that the key breakthroughs in new materials have come both from small laboratories and from larger centers in different
countries, demonstrating the importance of a balanced national and international research effort.
There have been many reports of higher transition temperatures. So far these results have been difficult to reproduce, and there is no consensus at present about their validity. Nevertheless, there is widespread expectation that new phases will be discovered, and a frenetic search for them continues in many laboratories worldwide.
Synthesizing and characterizing these new materials have been multidisciplinary enterprises involving contributions from many fields. Unlike most earlier superconductors, the new materials are ceramics, so that many techniques of ceramic processing have been applied to their synthesis. The new superconductors have already been prepared in the form of wires, thin films, and single crystals, in addition to sintered granular material. Yet many challenges remain: the ceramic or polycrystalline material suffers from low critical current densities (the highest current the material can carry before losing its superconductivity), and the single crystals are still generally too small for decisive neutron-scattering studies, which would allow their magnetic correlations to be determined. Electron pairing also has yet to be controlled in single-crystal synthesis.
The problems in synthesis have also pointed to a variety of chemistry issues, including the interactions of the new high-temperature superconductors with environmental factors such as water and carbon dioxide. The large number of elemental constituents and the critical role of oxygen in the structures make their chemistry both complex and interesting. Formation of the superconducting phase seems to require high-temperature annealing, but proper control of the oxygen, at least in the “123” compounds, requires a lower temperature. Whether these temperatures can be lowered further will be vital to many applications that cannot tolerate high-temperature anneals. This is particularly true of thin-film applications, and studies have already revealed significant interdiffusion of the superconducting films with most substrates.
The nature of superconductivity in these materials and the nature of the materials in their nonsuperconducting states have rapidly become central problems in modern condensed-matter physics. There is growing evidence that new pairing mechanisms are required that go beyond the conventional electron-phonon coupling believed to account for most earlier superconductors. There is also growing evidence that earlier theories must be generalized to include effects arising from both large anisotropy and fluctuation phenomena enhanced by the short coherence length in these materials. The effects of different oxygen defect structures, of twin boundaries, and of copper oxide planes and chains on the superconductivity are all yet to be understood. The structure of a superconducting material with one of the highest transition temperatures yet achieved is shown in Figure 3.10.
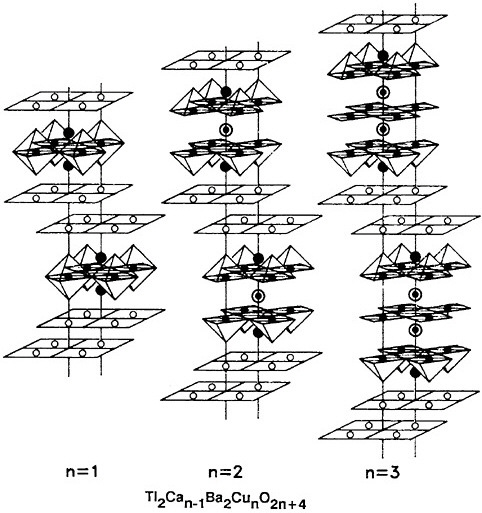
FIGURE 3.10 Structure of a superconducting material. As the number of copper layers (n) increases from one to three, the superconducting transition termperature rises. (Courtesy Stuart Pankin, IBM Corporation.)
A vast number of experiments have been conducted to elucidate the microscopic mechanisms of the new superconductors. But basic information on high-quality single crystals has only begun to appear, and vital experiments such as neutron scattering still await the availability of large enough crystals. Although many theories have been proposed, most theorists agree that more experimental information is needed before a consensus will begin to emerge.
Many other physically interesting properties bear on the practical application of these materials. Foremost is the critical current density, which appears to be controlled by the presence of grain boundaries in polycrystalline
material. The nature of those grain boundaries and the control of impurity phases segregated at them are just beginning to be studied. In single crystals, the nature of the mechanism that permits large current densities is as yet unknown. There has been steady but largely empirical progress in increasing current densities for practical application. More work is needed before such applications as magnetic wire will become feasible.
There are numerous structural issues as well. In ceramics, various superconducting structures have already been synthesized, with different degrees of orientation. Again, this work has been done on a largely empirical basis, but a detailed understanding is vital for control of mechanical and electrical properties. In thin films, epitaxial growth has been used to demonstrate the high critical currents possible in these materials. These findings need to be demonstrated in polymeric films.
BIOMATERIALS
Biomaterials can be broadly defined as the class of materials suitable for biomedical applications. They may be synthetically derived from nonbiological or even inorganic materials, or they may originate in living tissues. The products that incorporate biomaterials are extremely varied and include artificial organs; biochemical sensors; vascular grafts; artificial hearts; disposable materials and commodities; drug-delivery systems; hybrid artificial organs; dental, plastic surgery, ear, and ophthalmological devices; orthopedic replacements; prostheses and repairs; wound management aids; and packaging materials for biomedical and hygienic uses.
The success of already developed materials, notably of implants and joint replacements in older patients, has helped create an increased demand for these devices in all age groups. A corollary of this new demand is that the in vivo lifetime of devices will have to be increased from a current value of approximately 20 years to possibly 80 years.
The search for new biomaterials has accelerated enormously in the past two decades, spurred on in part by rapid advances in biomedical research and by the development of new synthetic materials, particularly polymers. A primary direction of biomaterials research must be toward understanding the interaction between synthetic substrates and biological tissues to meet the needs of clinical applications. Much basic research is currently directed toward finding new materials for reconstructive surgery and for other applications. However, the anticipated rapid increase in the demand for biomaterials will require that applied research also focus on cutting the costs of substance manufacture, clinical procedures, and rehabilitative systems.
Biomaterials Categories
Synthetic Nondegradable Polymers
One of the most important research issues related to synthetic nondegradable polymers is their stability and compatibility in the presence of biological materials, since they are often used in applications requiring moderate to long lifetimes. Their very broad range of applications includes prosthetic devices, vascular grafts, sutures, packaging, soft tissue augmentation, drug-delivery systems, synthetic oxygen carriers, contact lenses, and orthopedic replacements, as well as packaging, syringes, and diapers. For each material, stability and compatibility issues include compatibility with tissues (including blood), hydrolytic stability, reactivity with drugs and medications, calcification, long-term functionality, mutagenic or carcinogenic properties, and sterilizability.
Concentrated efforts should be directed to investigating in vivo reactions with tissues, the effects of surface chemistry and morphology on thrombogenesis, protein and cell consumption, and the formation of emboli. Additional concerns include assessing the medical risks of additives in materials, low-molecular-weight components, in vivo degradation products, and sterilization products, and constructing a data base to help assess these biomedical factors.
Synthetic materials used in drug-delivery systems should be compatible with a number of different drugs and other excipients and should be stable during processing and during sterilization by gamma radiation.
Bioabsorbable and Soluble Polymers
Unlike the nondegradable polymers, bioabsorbable compounds depend for their functionality on a capacity to be absorbed by living tissue and/or dispersed in a biological solvent such as blood serum. Applications for this class of synthetic biomaterials include sutures, vascular grafts, artificial ligament scaffolds, drug-delivery systems, environmentally degradable materials, and edible food casings. Figure 3.11 illustrates the growth of endothelial cells on a vascular graft.
Some of the key issues for these materials are absorbability and its measurement and definition, the effects of absorption on the tissue site, the effects of enzymes and other biologically active materials on the absorbability of the polymers, the biological effects of degradation products, the effects of sterilization on functionality and degradability, the behavior of labile release agents incorporated into the polymer, and the effects of substances on wound healing.
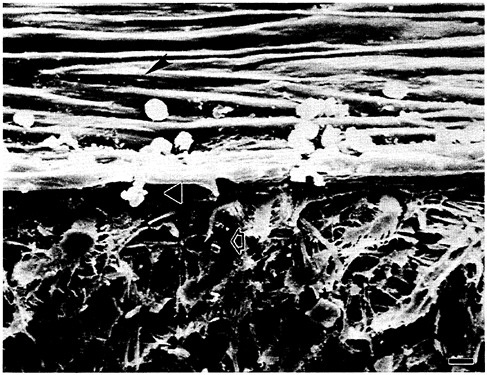
FIGURE 3.11 Scanning electron microscope micrograph of a vascular graft “seeded” with autologous microvessel endothelial cells and implanted in a canine artery for 5 weeks. The arrowhead points to the endothelial cell lining that was created. The triangle shows the thickness of the new cellular lining. The open arrow points to the vascular graft. Bar=11 µm. (Reprinted, by permission, from Bruce Jarrell and Stuart Williams. Copyright © 1989 by Bruce Jarrell and Stuart Williams.)
Polymeric Tissue Adhesives
The strength and durability of surfaces attached by polymers are clearly of essential importance, as are the chemical and mechanical compatibility of tissue adhesives with biological materials. The problem of tissue-to-tissue adhesion will require careful study. In addition, research will be required to enable understanding of the biological effects of degradation products of adhesives, the biological pathways that interact with degradation products and the effects of degradation products on those pathways, the effects of processing and sterilization on biodegradation, and the effects and control of wound healing.
Metals, Ceramics, and Glass
Metals and metal alloys are broadly used in biomedical applications, such as in orthopedic replacements, plastic surgery, and sutures. For metals to
meet criteria for biomedical use, they must be well characterized with regard to electrochemical corrodibility, the durability of passive layers, other degradative mechanisms, ability to elicit immune and hypersensitive reactions, and interactions with biological pathways.
For ceramics and glassy materials, durability, fracture toughness, surface activity, and degradation are properties requiring in-depth investigation. The biological effects of degradation, the effects of biological materials such as enzymes, and the effects of processing and sterilization on the behavior of synthetic biomaterials are also important issues.
For bioabsorbable ceramics, which are projected for use in orthopedics, research should focus on the measurement of bioabsorption; the influence of particular tissue sites; the effects of the ceramics on calcification, bone formation, and wound healing; and the ramifications of incorporating labile release agents into the ceramics.
Fundamental research on bioactive ceramics should be directed to bioactive mechanisms. Specific issues include the compositional dependence of bioactive bonding to bone and soft tissues, the effects of mismatches of elasticity modulus on stress transfer, bioelectric potentials and interfacial bonding properties, and the stability and fatigue resistance of bioactive interfacial bonding, coatings, and composites.
Carbons
Carbon in various forms—glassy, pyrolytically deposited, in fibers, and in composites—is a candidate material for biomedical applications. Like other proposed synthetic biomaterials, carbons must be characterized regarding properties affecting biological activity. The strength and fracture resistance of carbon materials are salient properties for biological use.
Biologically Derived Materials
Biological materials from both human and nonhuman sources have medical applications. Such materials include processed tissue from porcine heart valves, bovine carotid arteries, human umbilical veins, reconstituted collagen and elastin, hyaluronic acid, chondroitin sulfate, and chitosans. Applications include cardiovascular and ophthalmic applications as well as soft tissue augmentation.
Whether human or nonhuman, biological materials tend to provoke anti-genic rejection responses, which need to be characterized. The materials noted require isolation and purification, and standards for purity must be defined. Durability of materials and their calcification effects are also important issues.
Surface Modifications
Numerous techniques of coating and surface derivatization are under investigation in a variety of medical applications, including the control of tissue adhesion, the reduction of friction (e.g., in catheters), thrombolytic and anticoagulant agents, antibiotics, immobilized enzymes or other agents for chemotherapy, and removal of certain proteins or cells. Central issues for each of these processes must be the complete biological characterization of the coatings or surfaces, measurement and definition of in vitro and in vivo biological activity, and the effects of sterilization on the functionality of the coatings.
Major progress in extracorporeal procedures could be achieved if device surfaces could be rendered nonthrombogenic at reasonable cost. The most significant barrier to success at the moment is lack of an appropriate model for human use, as platelet aggregation and the clotting cascade mechanism appear to be unique to humans; hence adequate animal models are not available.
Surface effects are also crucial to determining immunoactive or immunocompatible properties. Creation of surfaces with affinities for specific proteins will probably be a major focus for the development of new polymers.
Long-Term Opportunities Related to Biomaterials
The incorporation of biochemical and pharmacological activity into medical devices is an area that is already undergoing rapid development. Particular areas of interest include devices for controlled and sustained drug delivery and devices with active anticoagulant behavior and antibacterial properties.
Totally synthetic or artificial devices will not be used for very long in medicine. The field is now moving toward use of what can be called hybrid devices and organs. A good example is the use of materials as a scaffold on which to culture, organize, and grow cells for specific functions. This is happening, for example, in the creation of the artificial pancreas. Today’s vascular grafts and artificial blood vessels depend, in part, on cellular linings for their function.
The biotechnology industry also depends on the growing or culturing of cells on suitable supports for various purposes. This field is undergoing rapid development, due largely to the discovery and increased understanding of cellular growth factors, which allow cell growth and proliferation to be controlled.
An extension of hybrid devices and organs will be the capacity to induce cells and tissues to fully duplicate and regenerate themselves. There is some evidence for this in nonmammalian species. Developing a means to literally grow a new heart or kidney from a piece of biopsy tissue would be far more
desirable and perhaps less costly than attempting to maintain an individual on an artificial kidney or heart indefinitely.
With regard to basic research opportunities in biomaterials, a number of recent developments in surface chemistry and surface characterization, as well as in microengineering and microprocessing, can be expected to lead to major breakthroughs and innovations in the near future. Specific examples are the scanning tunneling and scanning force microscopes, which provide for the study, analysis, and understanding of surfaces at the subnanometer scale. These devices are only beginning to be applied to solids in an aqueous environment and to biological macromolecules, including proteins and viruses. In principle, they permit imaging of individual atoms on the surface of, for example, protein molecules deposited on an appropriate support.
Of particular interest and potential application is the scanning force microscope, which images intermolecular and interatomic forces of attraction with subnanometer lateral resolution. If such an instrument can indeed be applied to biological macromolecules, viruses, and cell surfaces, it will transform research and understanding in these areas.
There has been considerable progress in adapting micron- and submicronscale processes commonly used in the electronics industry to biological materials. Thin-film and integrated optics, micromachining, and microarea chemistry and biochemistry are all being applied extensively to the development of biosensors, including miniature multichannel biosensors, which eventually will be incorporated into medical devices. This will permit regulation of function and will enable the multivariate testing and evaluation of medical devices in a variety of complex biological environments.
Microengineering processes are already being used in the cell culture field to deposit cells on appropriate substrates in microscopic arrays and patterns. As this technology evolves, it will be possible to envision cells of the same or different types being deposited and maintained in precisely designed morphologies and complex three-dimensional architectures. One could, in principle, begin to assemble synthetic tissues, using individual cellular and macromolecular building blocks.
FINDINGS
Over the past 10 decades, enormous improvements in materials and in their ability to perform useful functions for society have changed the world we live in—our transportation, communications, health, safety, and quality of life. One might reasonably ask, are we nearing an end to all this? Are we approaching a leveling off, a period of diminishing returns? The findings of this committee, to the contrary, are that the ripeness of the field is undiminished and that new materials and functional improvements of materials will continue to come at a remarkable rate. Of course, that rate will depend
on resources devoted to the task. Those nations that deploy their resources wisely can be expected to reap a rich technological and economic harvest in the decades ahead.
Several common themes have been discussed in this chapter. Foremost is the role of synthesis and processing in developing structural, electronic, magnetic, photonic, and superconducting materials and biomaterials. From strip casting of metals through the synthesizing of new nonlinear optical media in photonic materials to improving the critical current requirements of the new high-temperature superconductors, synthesis and processing are crucial for the continuing advancement of those technologies that depend on these functional properties.
Numerical simulations, modeling, and calculation of properties from first principles are increasingly used by scientists and engineers as means to shorten the time to develop understanding and applications of materials. It is almost inevitable that computers will be used increasingly to simulate the properties of new assemblies of atoms or to simulate a new process to shape structural materials, for instance. This trend needs to be encouraged in materials science and engineering both at the level of practice as well as at the level of teaching.
The enormous utility of the functional properties of the materials described in this chapter is well known. Previous studies dealing with particular classes of functional materials, such as electronic materials, have been carried out both in the United States and abroad. This study, however, emphasizes to a much greater degree the importance of synthesis and processing as the key, top-priority area of opportunity in materials science and engineering today. This theme is further developed in the section “Synthesis and Processing” in Chapter 4. To a very large extent, the relative advantage an organization or a nation will have in the competitive race ahead will be determined by the speed with which ideas such as those presented here can be exploited.